CDK5_Pathway信号通路
kenpaullone通过gsk-3cdk-5通路改善ad大鼠记忆功能
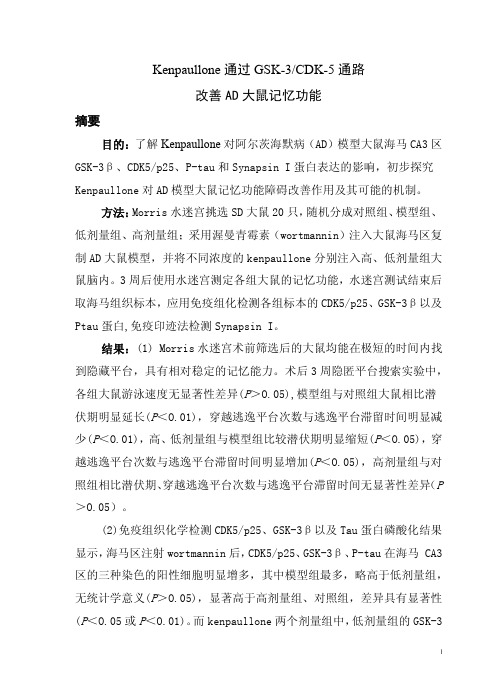
Kenpaullone通过GSK-3/CDK-5通路改善AD大鼠记忆功能摘要目的:了解Kenpaullone对阿尔茨海默病(AD)模型大鼠海马CA3区GSK-3β、CDK5/p25、P-tau和Synapsin I蛋白表达的影响,初步探究Kenpaullone对AD模型大鼠记忆功能障碍改善作用及其可能的机制。
方法:Morris水迷宫挑选SD大鼠20只,随机分成对照组、模型组、低剂量组、高剂量组;采用渥曼青霉素(wortmannin)注入大鼠海马区复制AD大鼠模型,并将不同浓度的kenpaullone分别注入高、低剂量组大鼠脑内。
3周后使用水迷宫测定各组大鼠的记忆功能,水迷宫测试结束后取海马组织标本,应用免疫组化检测各组标本的CDK5/p25、GSK-3β以及Ptau蛋白,免疫印迹法检测Synapsin I。
结果:(1) Morris水迷宫术前筛选后的大鼠均能在极短的时间内找到隐藏平台,具有相对稳定的记忆能力。
术后3周隐匿平台搜索实验中,各组大鼠游泳速度无显著性差异(P>O.05),模型组与对照组大鼠相比潜伏期明显延长(P<O.01),穿越逃逸平台次数与逃逸平台滞留时间明显减少(P<O.01),高、低剂量组与模型组比较潜伏期明显缩短(P<O.05),穿越逃逸平台次数与逃逸平台滞留时间明显增加(P<O.05),高剂量组与对照组相比潜伏期、穿越逃逸平台次数与逃逸平台滞留时间无显著性差异(P >O.05)。
(2)免疫组织化学检测CDK5/p25、GSK-3β以及Tau蛋白磷酸化结果显示,海马区注射wortmannin后,CDK5/p25、GSK-3β、P-tau在海马 CA3区的三种染色的阳性细胞明显增多,其中模型组最多,略高于低剂量组,无统计学意义(P>O.05),显著高于高剂量组、对照组,差异具有显著性(P<O.05或P<O.01)。
而kenpaullone两个剂量组中,低剂量组的GSK-3Iβ、P-tau高于对照组,具有统计学意义(P<O.05);高剂量组的CDK5/p25、GSK-3β、P-tau与对照组比较无显著性(P>O.05),表明kenpaullone能减轻wortmannin导致的CDK5/p25、GSK-3β升高,防止Tau蛋白过度磷酸化。
肿瘤细胞信号转导通路
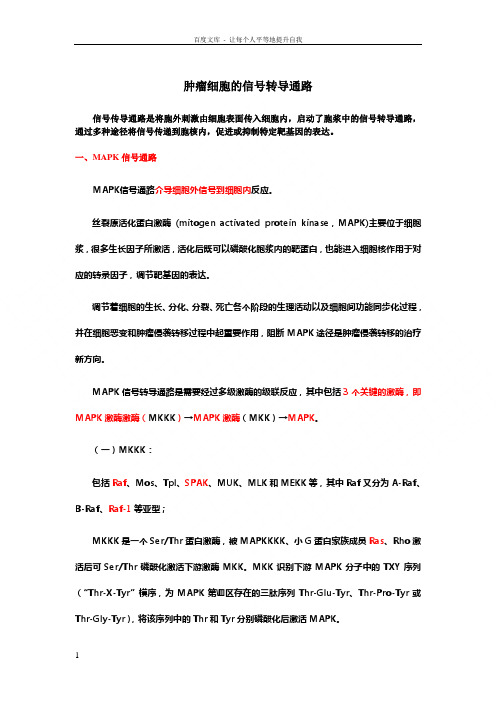
肿瘤细胞的信号转导通路信号传导通路是将胞外刺激由细胞表面传入细胞内,启动了胞浆中的信号转导通路,通过多种途径将信号传递到胞核内,促进或抑制特定靶基因的表达。
一、MAPK信号通路MAPK信号通路介导细胞外信号到细胞内反应。
丝裂原活化蛋白激酶(mitogen activated protein kinase,MAPK)主要位于细胞浆,很多生长因子所激活,活化后既可以磷酸化胞浆内的靶蛋白,也能进入细胞核作用于对应的转录因子,调节靶基因的表达。
调节着细胞的生长、分化、分裂、死亡各个阶段的生理活动以及细胞间功能同步化过程,并在细胞恶变和肿瘤侵袭转移过程中起重要作用,阻断MAPK途径是肿瘤侵袭转移的治疗新方向。
MAPK信号转导通路是需要经过多级激酶的级联反应,其中包括3个关键的激酶,即MAPK激酶激酶(MKKK)→MAPK激酶(MKK)→MAPK。
(一)MKKK:包括Raf、Mos、Tpl、SPAK、MUK、MLK和MEKK等,其中Raf又分为A-Raf、B-Raf、Raf-1等亚型;MKKK是一个Ser/Thr蛋白激酶,被MAPKKKK、小G蛋白家族成员Ras、Rho激活后可Ser/Thr磷酸化激活下游激酶MKK。
MKK识别下游MAPK分子中的TXY序列(“Thr-X-Tyr”模序,为MAPK第Ⅷ区存在的三肽序列Thr-Glu-Tyr、Thr-Pro-Tyr或Thr-Gly-Tyr),将该序列中的Thr和Tyr分别磷酸化后激活MAPK。
注:TXY序列是MKK活化JNK的双磷酸化位点,MKK4和MKK7通过磷酸化TXY 序列的第183位苏氨酸残基(Thr183)和第185位酪氨酸残基(Tyr185)激活JNK1。
(二)MKK:包括MEK1-MEK7,主要是MEK1/2;(三)MAPK:MAPK是一类丝氨酸/苏氨酸激酶,是MAPK途径的核心,它至少由4种同功酶组成,包括:细胞外信号调节激酶(Extracellular signal Regulated Kinases,ERK1/2)、C-Jun 氨基末端激酶(JNK)/应激激活蛋白激酶(Stress-activated protein kinase,SAPK)、p38(p38MAPK)、ERK5/BMK1(big MAP kinase1)等MAPK亚族,并根据此将MAPK 信号传导通路分为4条途径。
细胞周期依赖性激酶CDKs介绍
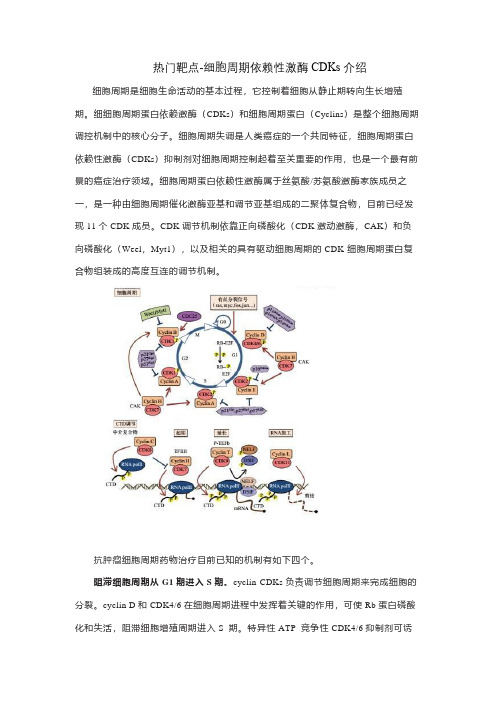
热门靶点-细胞周期依赖性激酶CDKs介绍细胞周期是细胞生命活动的基本过程,它控制着细胞从静止期转向生长增殖期。
细细胞周期蛋白依赖激酶(CDKs)和细胞周期蛋白(Cyclins)是整个细胞周期调控机制中的核心分子。
细胞周期失调是人类癌症的一个共同特征,细胞周期蛋白依赖性激酶(CDKs)抑制剂对细胞周期控制起着至关重要的作用,也是一个最有前景的癌症治疗领域。
细胞周期蛋白依赖性激酶属于丝氨酸/苏氨酸激酶家族成员之一,是一种由细胞周期催化激酶亚基和调节亚基组成的二聚体复合物,目前已经发现11个CDK成员。
CDK调节机制依靠正向磷酸化(CDK激动激酶,CAK)和负向磷酸化(Weel,Myt1),以及相关的具有驱动细胞周期的CDK-细胞周期蛋白复合物组装成的高度互连的调节机制。
抗肿瘤细胞周期药物治疗目前已知的机制有如下四个。
阻滞细胞周期从G1期进入S期。
cyclin-CDKs负责调节细胞周期来完成细胞的分裂。
cyclin D和CDK4/6在细胞周期进程中发挥着关键的作用,可使Rb蛋白磷酸化和失活,阻滞细胞增殖周期进入S 期。
特异性ATP 竞争性CDK4/6抑制剂可诱导Rb 蛋白阳性肿瘤不可逆的细胞周期G1期阻滞。
选择性CDK4/6抑制剂包括ribociclib、abemaciclib和palbociclib,阻滞细胞从G1期进入S期,提高抗肿瘤效果。
诸多的细胞周期治疗药物中,批准临床应用只有CDK4/6抑制剂ribociclib、abemaciclib、palbociclib,阻滞细胞周期从G1期进入S期,激活抗肿瘤免疫,控制代谢功能和调节转录水平。
调节转录水平有丝分裂期间细胞转录保持在低水平状态,只有当丝分裂结束后转录才会重新激活。
CDK9 和CDK12可调控细胞转录。
细胞周期治疗领域的研究主要是寻找转录CDKs的抑制剂。
激活抗肿瘤免疫研究证实,CDK4/6抑制剂不仅能诱导肿瘤细胞周期阻滞,还促抗肿瘤免疫:首先,CDK4/6抑制剂激活肿瘤细胞内源性逆转录病毒成分表达,增加细胞内双链RNA水平,进而刺激产生Ⅲ 型干扰素并增加肿瘤抗原递呈;其次,CDK4/6抑制剂显著抑制调节性T细胞的增殖,促进细胞毒性T细胞对肿瘤细胞的清除。
细胞信号通路大全

1 PPAR信号通路:过氧化物酶体增殖物激活受体( PPARs) 是与维甲酸、类固醇和甲状腺激素受体相关的配体激活转录因子超家族核激素受体成员。
它们作为脂肪传感器调节脂肪代谢酶的转录。
PPARs由PPARα、PPARβ和PPARγ 3种亚型组成。
PPARα主要在脂肪酸代谢水平高的组织,如:肝、棕色脂肪、心、肾和骨骼肌表达。
他通过调控靶基因的表达而调节机体许多生理功能包括能量代谢、生长发育等。
另外,他还通过调节脂质代谢的生物感受器而调节细胞生长、分化与凋亡。
PPARa同时也是一种磷酸化蛋白,他受多种磷酸化酶的调节包括丝裂原激活蛋白激酶( ERK-和p38.M APK) ,蛋白激酶A和C( PKA,PKC) ,AM PK和糖原合成酶一3( G SK3) 等调控。
调控PPARa生长信号的酶报道有M APK、PKA和G SK3。
PPARβ广泛表达于各种组织,而PPAR γ主要局限表达在血和棕色脂肪,其他组织如骨骼肌和心肌有少量表达。
PPAR-γ在诸如炎症、动脉粥样硬化、胰岛素抵抗和糖代谢调节,以及肿瘤和肥胖等方面均有着举足轻重的作用,而其众多生物学效应则是通过启动或参与的复杂信号通路予以实现。
鉴于目前人们对PPAR—γ信号通路尚不甚清,PPARs 通常是通过与9-cis维甲酸受体( RXR)结合实现其转录活性的。
2 MAPK信号通路:mapk简介:丝裂原激活蛋白激酶(mitogen—activated protein kinase,MAPK)是广泛存在于动植物细胞中的一类丝氨酸/苏氨酸蛋白激酶。
作用主要是将细胞外刺激信号转导至细胞及其核内,并引起细胞的生物化学反应(增殖、分化、凋亡、应激等)。
MAPKs家族的亚族 :ERKs(extracellular signal regulated kinase):包括ERK1、ERK2。
生长因子、细胞因子或激素激活此通路,介导细胞增殖、分化。
JNKs(c-Jun N-terminal kinase)包括JNK1、JNK2、JNK3。
细胞常见信号通路图片合集
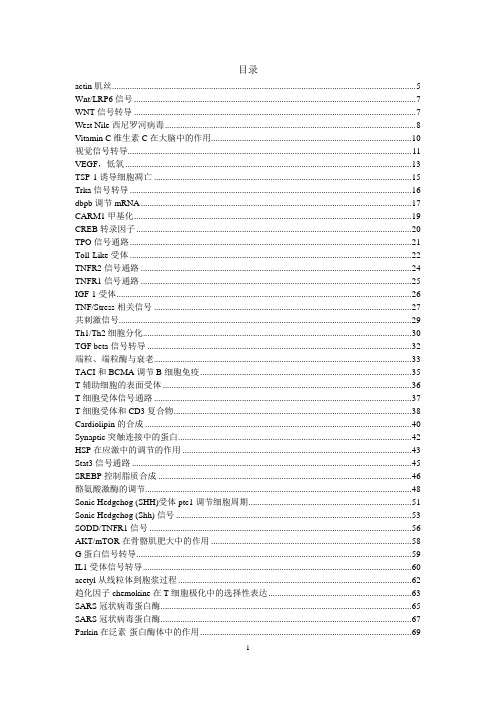
目录actin肌丝 (5)Wnt/LRP6 信号 (7)WNT信号转导 (7)West Nile 西尼罗河病毒 (8)Vitamin C 维生素C在大脑中的作用 (10)视觉信号转导 (11)VEGF,低氧 (13)TSP-1诱导细胞凋亡 (15)Trka信号转导 (16)dbpb调节mRNA (17)CARM1甲基化 (19)CREB转录因子 (20)TPO信号通路 (21)Toll-Like 受体 (22)TNFR2 信号通路 (24)TNFR1信号通路 (25)IGF-1受体 (26)TNF/Stress相关信号 (27)共刺激信号 (29)Th1/Th2 细胞分化 (30)TGF beta 信号转导 (32)端粒、端粒酶与衰老 (33)TACI和BCMA调节B细胞免疫 (35)T辅助细胞的表面受体 (36)T细胞受体信号通路 (37)T细胞受体和CD3复合物 (38)Cardiolipin的合成 (40)Synaptic突触连接中的蛋白 (42)HSP在应激中的调节的作用 (43)Stat3 信号通路 (45)SREBP控制脂质合成 (46)酪氨酸激酶的调节 (48)Sonic Hedgehog (SHH)受体ptc1调节细胞周期 (51)Sonic Hedgehog (Shh) 信号 (53)SODD/TNFR1信号 (56)AKT/mTOR在骨骼肌肥大中的作用 (58)G蛋白信号转导 (59)IL1受体信号转导 (60)acetyl从线粒体到胞浆过程 (62)趋化因子chemokine在T细胞极化中的选择性表达 (63)SARS冠状病毒蛋白酶 (65)SARS冠状病毒蛋白酶 (67)Parkin在泛素-蛋白酶体中的作用 (69)nicotinic acetylcholine受体在凋亡中的作用 (71)线粒体在细胞凋亡中的作用 (73)MEF2D在T细胞凋亡中的作用 (74)Erk5和神经元生存 (75)ERBB2信号转导 (77)GPCRs调节EGF受体 (78)BRCA1调节肿瘤敏感性 (79)Rho细胞运动的信号 (81)Leptin能逆转胰岛素抵抗 (82)转录因子DREAM调节疼敏感 (84)PML调节转录 (86)p27调节细胞周期 (88)MAPK信号调节 (89)细胞因子调节造血细胞分化 (91)eIF4e和p70 S6激酶调节 (92)eIF2调节 (93)谷氨酸受体调节ck1/cdk5 (94)BAD磷酸化调节 (95)plk3在细胞周期中的作用 (96)Reelin信号通路 (97)RB肿瘤抑制和DNA破坏 (98)NK细胞介导的细胞毒作用 (99)Ras信号通路 (100)Rac 1细胞运动信号 (101)PTEN依赖的细胞生长抑制和细胞凋亡 (103)蛋白激酶A(PKA)在中心粒中的作用 (104)notch信号通路 (106)蛋白酶体Proteasome复合物 (108)Prion朊病毒的信号通路 (109)早老素Presenilin在notch和wnt信号中的作用 (110)淀粉样蛋白前体信号 (112)mRNA的poly(A)形成 (113)PKC抑制myosin磷酸化 (114)磷脂酶C(PLC)信号 (115)巨噬细胞Pertussis toxin不敏感的CCR5信号通路 (116)Pelp1调节雌激素受体的活性 (117)PDGF信号通路 (118)p53信号通路 (120)p38MAPK信号通路 (121)Nrf2是氧化应激基本表达的关键基因 (122)OX40信号通路 (123)hTert转录因子的调节作用 (124)hTerc转录调节活性图 (125)AIF在细胞凋亡中的作用 (126)Omega氧化通路 (127)核受体在脂质代谢和毒性中的作用 (129)NK细胞中NO2依赖的IL-12信号通路 (131)TOR信号通路 (133)NO信号通路 (134)NF-kB信号转导通路 (135)NFAT与心肌肥厚的示意图 (137)神经营养素及其表面分子 (139)神经肽VIP和PACAP防止活化T细胞凋亡图 (141)神经生长因子信号图 (142)细胞凋亡信号通路 (144)MAPK级联通路 (144)MAPK信号通路图 (145)BCR信号通路 (146)蛋白质乙酰化示意图 (147)wnt信号通路 (148)胰岛素受体信号通路 (149)细胞周期在G2/M期的调控机理图 (151)细胞周期G1/S检查点调控机理图 (152)Jak-STAT关系总表 (153)Jak/STAT 信号 (155)TGFbeta信号 (156)NFkappaB信号 (157)p38 MAPK信号通路 (159)SAPK/JNK 信号级联通路 (160)从G蛋白偶联受体到MAPK (161)MAPK pathwayMAPK级联信号图 (162)eIF-4E和p70 S6激酶调控蛋白质翻译 (163)eif2蛋白质翻译 (164)蛋白质翻译示意图 (165)线粒体凋亡通路 (167)死亡受体信号通路 (168)凋亡抑制通路 (170)细胞凋亡综合示意图 (171)Akt/Pkb信号通路 (172)MAPK/ERK信号通路 (174)哺乳动物MAPK信号通路 (175)Pitx2多步调节基因转录 (176)IGF-1R导致BAD磷酸化的多个凋亡路径 (177)多重耐药因子 (179)mTOR信号通路 (180)Msp/Ron受体信号通路 (181)单核细胞和其表面分子 (182)线粒体的肉毒碱转移酶(CPT)系统 (183)METS影响巨噬细胞的分化 (184)Anandamide,内源性大麻醇的代谢 (186)黑色素细胞(Melanocyte)发育和信号 (187)DNA甲基化导致转录抑制的机理图 (188)蛋白质的核输入信号图 (190)PPARa调节过氧化物酶体的增殖 (192)对乙氨基酚(Acetaminophen)的活性和毒性机理 (194)mCalpain在细胞运动中的作用 (196)MAPK信号图 (198)MAPK抑制SMRT活化 (200)苹果酸和天门冬酸间的转化 (201)低密度脂蛋白(LDL)在动脉粥样硬化中的作用 (202)LIS1基因在神经细胞的发育和迁移中的作用图 (204)Pyk2与Mapk相连的信号通路 (205)galactose代谢通路 (206)Lectin诱导补体的通路 (207)Lck和Fyn在TCR活化中的作用 (208)乳酸合成图 (209)Keratinocyte分化图 (210)离子通道在心血管内皮细胞中的作用 (211)离子通道和佛波脂(Phorbal Esters)信号 (213)内源性Prothrombin激活通路 (214)Ribosome内化通路 (216)整合素(Integrin)信号通路 (217)胰岛素(Insulin)信号通路 (218)Matrix Metalloproteinases (219)组氨酸去乙酰化抑制剂抑制Huntington病 (220)Gleevec诱导细胞增殖 (222)Ras和Rho在细胞周期的G1/S转换中的作用 (224)DR3,4,5受体诱导细胞凋亡 (225)AKT调控Gsk3图 (226)IL-7信号转导 (227)IL22可溶性受体信号转导图 (229)IL-2活化T细胞图 (230)IL12和Stat4依赖的TH1细胞发育信号通路 (232)IL-10信号通路 (233)IL 6信号通路 (234)IL 5信号通路 (236)actin肌丝Mammalian cell motility requires actin polymerization in the direction of movement to change membrane shape and extend cytoplasm into lamellipodia. The polymerization of actin to drive cell movement also involves branching of actin filaments into a network oriented with the growing ends of the fibers near the cell membrane. Manipulation of this process helps bacteria like Salmonella gain entry into cells they infect. Two of the proteins involved in the formation of Y branches and in cell motility are Arp2 and Arp3, both members of a large multiprotein complex containing several other polypeptides as well. The Arp2/3 complex is localized at the Y branch junction and induces actin polymerization. Activity of this complex is regulated by multiple different cell surface receptor signaling systems, activating WASP, and Arp2/3 in turn to cause changes in cell shape and cell motility. Wasp and its cousin Wave-1 interact with the Arp2/3 complex through the p21 component of the complex. The crystal structure of the Arp2/3 complex has revealed further insights into the nature of how the complex works.Activation by Wave-1, another member of the WASP family, also induces actinalterations in response to Rac1 signals upstream. Wave-1 is held in an inactive complex in the cytosol that is activated to allow Wave-1 to associate with Arp2/3. While WASP is activated by interaction with Cdc42, Wave-1, is activated by interaction with Rac1 and Nck. Wave-1 activation by Rac1 and Nck releases Wave-1 with Hspc300 to activate actin Y branching and polymerization by Arp2/3. Different members of this gene family may produce different actin cytoskeletal architectures. The immunological defects associated with mutation of the WASP gene, the Wiskott-Aldrich syndrome for which WASP was named, indicates the importance of this system for normal cellular function.Cory GO, Ridley AJ. Cell motility: braking WAVEs. Nature. 2002 Aug15;418(6899):732-3. No abstract available.Eden, S., et al. (2002) Mechanism of regulation of WAVE1-induced actin nucleation by Rac1 and Nck. Nature 418(6899), 790-3Falet H, Hoffmeister KM, Neujahr R, Hartwig JH. Normal Arp2/3 complex activation in platelets lacking WASp. Blood. 2002 Sep 15;100(6):2113-22.Kreishman-Deitrick M, Rosen MK, Kreishman-Deltrick M. Ignition of a cellular machine. Nat Cell Biol. 2002 Feb;4(2):E31-3. No abstract available.Machesky, L.M., Insall, R.H. (1998) Scar1 and the related Wiskott-Aldrich syndrome protein, WASP, regulate the actin cytoskeleton through the Arp2/3 complex. Curr Biol 8(25), 1347-56Robinson, R.C. et al. (2001) Crystal structure of Arp2/3 complex. Science 294(5547), 1679-84Weeds A, Yeoh S. Structure. Action at the Y-branch. Science. 2001 Nov23;294(5547):1660-1. No abstract available.Wnt/LRP6 信号Wnt glycoproteins play a role in diverse processes during embryonic patterning in metazoa through interaction with frizzled-type seven-transmembrane-domain receptors (Frz) to stabilize b-catenin. LDL-receptor-related protein 6 (LRP6), a Wnt co-receptor, is required for this interaction. Dikkopf (dkk) proteins are both positive and negative modulators of this signalingWNT信号转导West Nile 西尼罗河病毒West Nile virus (WNV) is a member of the Flaviviridae, a plus-stranded virus family that includes St. Louis encephalitis virus, Kunjin virus, yellow fever virus, Dengue virus, and Japanese encephalitis virus. WNV was initially isolated in 1937 in the West Nile region of Uganda and has become prevalent in Africa, Asia, and Europe. WNV has rapidly spread across the United States through its insect host and causes neurological symptoms and encephalitis, which can result in paralysis or death. Since 1999 about 3700 cases of West Nile virus (WNV) infection and 200 deaths have been recorded in United States. The viral capsid protein likely contributes to theWNV-associated deadly inflammation via apoptosis induced through the mitochondrial pathway.WNV particles (50 nm in diameter) consist of a dense core (viral protein C encapsidated virus RNA genome) surrounded by a membrane envelope (viral E and M proteins embedded in a lipid bilayer). The virus binds to a specific cell surface protein (not yet identified), an interaction thought to involve E protein with highly sulfated neperan sulfate (HSHS) residues that are present on the surfaces of many cells and enters the cell by a process similar to that of endocytosis. Once inside the cell, the genome RNA is released into the cytoplasm via endosomal release, a fusion process involving acidic pH induced conformation change in the E protein. The RNA genome serves as mRNA and is translated by ribosomes into ten mature viral proteins are produced via proteolytic cleavage, which include three structural components and seven different nonstructural components of the virus. These proteins assemble and transcribe complimentary minus strand RNAs from the genomic RNA. The complimentary minus strand RNA in turns serves as template for the synthesis of positive-stranded genomic RNAs. Once viral E, preM and C proteins have accumulated to sufficient level, they assemble with the genomic RNA to form progeny virions, which migrate to the cell surface where they are surrounded with lipid envelop and released.Vitamin C 维生素C在大脑中的作用Vitamin C (ascorbic acid) was first identified by virtue of the essential role it plays in collagen modification, preventing the nutritional deficiency scurvy. Vitamin C acts as a cofactor for hydroxylase enzymes that post-translationally modify collagen to increase the strength and elasticity of tissues. Vitamin C reduces the metal ion prosthetic groups of many enzymes, maintaining activity of enzymes, also acts as an anti-oxidant. Although the prevention of scurvy through modification of collagen may be the most obvious role for vitamin C, it is not necessarily the only role of vitamin C. Svct1 and Svct2 are ascorbate transporters for vitamin C import into tissues and into cells. Both of these transporters specifically transport reduced L-ascorbic acid against a concentration gradient using the intracellular sodium gradient to drive ascorbate transport. Svct1 is expressed in epithelial cells in the intestine, upregulated in cellular models for intestinal epithelium and appears to be responsible for the import ofdietary vitamin C from the intestinal lumen. The vitamin C imported from the intestine is present in plasma at approximately 50 uM, almost exclusively in the reduced form, and is transported to tissues to play a variety of roles. Svct2 imports reduced ascorbate from the plasma into very active tissues like the brain. Deletion in mice of the gene for Svct2 revealed that ascorbate is required for normal development of the lungs and brain during pregnancy. A high concentration of vitamin C in neurons of the developing brain may help protect the developing brain from free radical damage. The oxidized form of ascorbate, dehydroascorbic acid, is transported into a variety of cells by the glucose transporter Glut-1. Glut-1, Glut-3 and Glut-4 can transport dehydroascorbate, but may not transport significant quantities of ascorbic acid in vivo.视觉信号转导The signal transduction cascade responsible for sensing light in vertebrates is one of the best studied signal transduction processes, and is initiated by rhodopsin in rodcells, a member of the G-protein coupled receptor gene family. Rhodopsin remains the only GPCR whose structure has been resolved at high resolution. Rhodopsin in the discs of rod cells contains a bound 11-cis retinal chromophore, a small molecule derived from Vitamin A that acts as the light sensitive portion of the receptor molecule, absorbing light to initiate the signal transduction cascade. When light strikes 11-cis retinal and is absorbed, it isomerizes to all-trans retinal, changing the shape of the molecule and the receptor it is bound to. This change in rhodopsin抯shape alters its interaction with transducin, the member of the G-protein gene family that is specific in its role in visual signal transduction. Activation of transducin causes its alpha subunit to dissociate from the trimer and exchange bound GDP for GTP, activating in turn a membrane-bound cyclic-GMP specific phosphodiesterase that hydrolyzes cGMP. In the resting rod cell, high levels of cGMP associate with a cyclic-GMP gated sodium channel in the plasma membrane, keeping the channels open and the membrane of the resting rod cells depolarized. This is distinct from synaptic generation of action potentials, in which stimulation induces opening of sodium channels and depolarization. When cGMP gated channels in rod cells open, both sodium and calcium ions enter the cell, hyperpolarizing the membrane and initiating the electrochemical impulse responsible for conveying the signal from the sensory neuron to the CNS. The rod cell in the resting state releases high levels of the inhibitory neurotransmitter glutamate, while the release of glutamate is repressed by the hyperpolarization in the presence of light to trigger a downstream action potential by ganglion cells that convey signals to the brain. The calcium which enters the cell also activates GCAP, which activates guanylate cyclase (GC-1 and GC-2) to rapidly produce more cGMP, ending the hyperpolarization and returning the cell to its resting depolarized state. A protein called recoverin helps mediate the inactivation of the signal transduction cascade, returning rhodopsin to its preactivated state, along with the rhodopsin kinase Grk1. Phosphorylation of rhodopsin by Grkl causes arrestin to bind, helping to terminate the receptor activation signal. Dissociation and reassociation of retinal, dephosphorylation of rhodopsin and release of arrestin all return rhodopsin to its ready state, prepared once again to respond to light.VEGF,低氧Vascular endothelial growth factor (VEGF) plays a key role in physiological blood vessel formation and pathological angiogenesis such as tumor growth and ischemic diseases. Hypoxia is a potent inducer of VEGF in vitro. The increase in secreted biologically active VEGF protein from cells exposed to hypoxia is partly because of an increased transcription rate, mediated by binding of hypoxia-inducible factor-1 (HIF1) to a hypoxia responsive element in the 5'-flanking region of the VEGF gene. bHLH-PAS transcription factor that interacts with the Ah receptor nuclear translocator (Arnt), and its predicted amino acid sequence exhibits significantsimilarity to the hypoxia-inducible factor 1alpha (HIF1a) product. HLF mRNA expression is closely correlated with that of VEGF mRNA.. The high expression level of HLF mRNA in the O2 delivery system of developing embryos and adult organs suggests that in a normoxic state, HLF regulates gene expression of VEGF, various glycolytic enzymes, and others driven by the HRE sequence, and may be involved in development of blood vessels and the tubular system of lung. VEGF expression is dramatically induced by hypoxia due in large part to an increase in the stability of its mRNA. HuR binds with high affinity and specificity to the VRS element that regulates VEGF mRNA stability by hypoxia. In addition, an internal ribosome entry site (IRES) ensures efficient translation of VEGF mRNA even under hypoxia. The VHL tumor suppressor (von Hippel-Lindau) regulates also VEGF expression at a post-transcriptional level. The secreted VEGF is a major angiogenic factor that regulates multiple endothelial cell functions, including mitogenesis. Cellular and circulating levels of VEGF are elevated in hematologic malignancies and are adversely associated with prognosis. Angiogenesis is a very complex, tightly regulated, multistep process, the targeting of which may well prove useful in the creation of novel therapeutic agents. Current approaches being investigated include the inhibition of angiogenesis stimulants (e.g., VEGF), or their receptors, blockade of endothelial cell activation, inhibition of matrix metalloproteinases, and inhibition of tumor vasculature. Preclinical, phase I, and phase II studies of both monoclonal antibodies to VEGF and blockers of the VEGF receptor tyrosine kinase pathway indicate that these agents are safe and offer potential clinical utility in patients with hematologic malignancies.TSP-1诱导细胞凋亡As tissues grow they require angiogenesis to occur if they are to be supplied with blood vessels and survive. Factors that inhibit angiogenesis might act as cancer therapeutics by blocking vessel formation in tumors and starving cancer cells. Thrombospondin-1 (TSP-1) is a protein that inhibits angiogenesis and slows tumor growth, apparently by inducing apoptosis of microvascular endothelial cells that line blood vessels. TSP-1 appears to produce this response by activating a signaling pathway that begins with its receptor CD36 at the cell surface of the microvascular endothelial cell. The non-receptor tyrosine kinase fyn is activated by TSP-1 through CD36, activating the apoptosis inducing proteases like caspase-3 and p38 protein kinases. p38 is a mitogen-activated kinase that also induces apoptosis in some conditions, perhaps through AP-1 activation and the activation of genes that lead to apoptosis.Trka信号转导Nerve growth factor (NGF) is a neurotrophic factor that stimulates neuronal survival and growth through TrkA, a member of the trk family of tyrosine kinase receptors that also includes TrkB and TrkC. Some NGF responses are also mediated or modified by p75LNTR, a low affinity neurotrophin receptor. Binding of NGF to TrkA stimulates neuronal survival, and also proliferation. Pathways coupled to these responses are linked to TrkA through association of signaling factors with specific amino acids in the TrkA cytoplasmic domain. Cell survival through inhibition of apoptosis is signaled through activation of PI3-kinase and AKT. Ras-mediated signaling and phospholipase C both activate the MAP kinase pathway to stimulate proliferation.dbpb调节mRNAEndothelial cells respond to treatment with the protease thrombin with increased secretion of the PDGF B-chain. This activation occurs at the transcriptional level and a thrombin response element was identified in the promoter of the PDGF B-chain gene. A transcription factor called the DNA-binding protein B (dbpB) mediates the activation of PDGF B-chain transcription in response to thrombin treatment. DbpB is a member of the Y box family of transcription factors and binds to both RNA and DNA. In the absence of thrombin, endothelial cells contain a 50 kD form of dbpB that binds RNA in the cytoplasm and may play a role as a chaperone for mRNA. The 50 kD version of dbpB also binds DNA to regulate genes containing Y box elements in their promoters. Thrombin activation results in the cleavage of dbpB to a 30 kD form. The proteolytic cleavage releases dbpB from RNA in the nucleus, allowing it to enter the nucleus and binds to a regulatory element distinct from the site recognized by the full length 50 kD dbpB. The genes activated by cleaved dbpB include the PDGF B chain. Dephosphorylation of dbpB also regulates nuclear entry and transcriptional activation.RNA digestion in vitro can release dbpB in its active form, suggesting that the protease responsible for dbpB may be closely associated in a complex. Identification of the protease that cleaves dbpB, the mechanisms of phosphorylation and dephosphorylation, and elucidation of the signaling path by which thrombin induces dbpB will provide greater understanding of this novel signaling pathway.CARM1甲基化Several forms of post-translational modification regulate protein activities. Recently, protein methylation by CARM1 (coactivator-associated arginine methyltransferase 1) has been observed to play a key role in transcriptional regulation. CARM1 associates with the p160 class of transcriptional coactivators involved in gene activation by steroid hormone family receptors. CARM1 also interacts with CBP/p300 transcriptional coactivators involved in gene activation by a large variety of transcription factors, including steroid hormone receptors and CEBP. One target of CARM1 is the core histones H3 and H4, which are also targets of the histone acetylase activity of CBP/p300 coactivators. Recruitment of CARM1 to the promoter region by binding to coactivators increases histone methylation and makes promoter regions more accessible for transcription. Another target of CARM1 methylation is a coactivator it interacts with, CBP. Methylation of CBP by CARM1 blocks CBP from acting as a coactivator for CREB and redirects the limited CBP pool in the cell to be available for steroid hormone receptors. Other forms of post-translational protein modification such as phosphorylation are reversible in nature, but as of yet a protein demethylase is not known.CREB转录因子The transcription factor CREB binds the cyclic AMP response element (CRE) and activates transcription in response to a variety of extracellular signals including neurotransmitters, hormones, membrane depolarization, and growth and neurotrophic factors. Protein kinase A and the calmodulin-dependent protein kinases CaMKII stimulate CREB phosphorylation at Ser133, a key regulatory site controlling transcriptional activity. Growth and neurotrophic factors also stimulate CREB phosphorylation at Ser133. Phosphorylation occurs at Ser133 via p44/42 MAP Kinase and p90RSK and also via p38 MAP Kinase and MSK1. CREB exhibit deficiencies in spatial learning tasks, while flies overexpressing or lacking CREB show enhanced or diminished learning, respectively.TPO信号通路Thrombopoietin (TPO) binds to its receptor inducing aggregation and activation. TPO signals its growth regulating effects to the cell through several major pathways including MAPK (ERK and JNK), Protein Kinase C, and JAK/Stat.Toll-Like 受体The innate immune response responds in a general manner to factors present in invading pathogens. Bacterial factors such as lipopolysaccharides (LPS, endotoxin), bacterial lipoproteins, peptidoglycans and also CpG nucleic acids activate innate immunity as well as stimulating the antigen-specific immune response and triggering the inflammatory response. Members of the toll-like receptor (TLR) gene family convey signals stimulated by these factors, activating signal transduction pathways that result in transcriptional regulation and stimulate immune function. TLR2 is activated by bacterial lipoproteins, TLR4 is activated by LPS, and TLR9 is activated by CpG DNA; peptidoglycan recognition protein (PGRP) is activated bypeptidoglycan (PGN). The downstream signaling pathways used by these receptors are similar to that used by the IL-1 receptor, activating the IL-1 receptor associated kinase (IRAK) through the MyD88 adaptor protein, and signaling through TRAF-6 and protein kinase cascades to activate NF-kB and Jun. NF-kB and c-Jun activate transcription of genes such as the proinflammatory cytokines IL-1 and IL-12. Several recent reports have suggested that the functional outcomes of signaling via TLR2, TLR4 and PGRP are not equivalent. For example, while the LPS-induced,p38-dependent response was dependent upon PU.1 binding, the PGN-induced, p38 response was not. The intracelular receptor for PGN, PGRP is conserved from insects to mammals. In insects, PGRP activates prophenoloxidase cascade, a part of the insect antimicrobial defense system. Because mammals do not have the prophenoloxidase cascade, its function in mammals is unknown. However, it was suggested that an identical protein Tag7 was a tumor necrosis factor-like (TNF-like) cytokine.PGRP/Tag7 possesses cytotoxicity and triggers intranucleosomal DNA fragmentation in target cells in the same way as many known members of the TNF family. Fragmentation of DNA is one of the characteristics of apoptosis. The possibility that in another system, PGRP/Tag7 would induce NF-kB activation, as observed for TRAIL (TNF-related apoptosis-inducing ligand) receptors canot be ruled out.TNFR2 is the receptor for the 171 amino acid 19 kD TNF(beta) (a.k.a. lymphotoxin). TNF(beta) is produced by activated lymphocytes and can be cytotoxic to many tumor and other cells. In neutrophils, endothelial cells and osteoclasts TNF(beta) can lead to activation while in many other cell types it can lead to increased expression of MHC and adhesion molecules.TNFR1 (a.k.a. p55, CD120a) is the receptor for TNF(alpha) and also will bind TNF(beta). Upon binding TNF(alpha) a TNFR1+ cell is triggered to undergo apoptosis. This critical regulatory process is accomplished by activating the proteolytic caspase cascade that results in the degradation of many critical cellular proteinsIGF-1受体TNF/Stress相关信号TNF acts on several different signaling pathways through two cell surface receptors, TNFR1 and TNFR2 (See TNFR1 and TNFR2 Signaling Pathways) to regulate apoptotic pathways, NF-kB activation of inflammation, and activate stress-activated protein kinases (SAPKs). Interaction of TNFR1 with TRADD leads to activation of NF-kB and apoptosis pathways, while interaction with TRAF2 has generally been thought to be involved in stress kinase and NF-kB activation but is not required for TNF to induce apoptosis. Activation of NF-kB is mediated by TRAF2 through the NIK kinase and also by RIP but the observation that TNF activates NF-kB in mice lacking TRAF2 indicates that TRAF-2 does not play an essential role in this process. Stress-activated protein kinases, also called JNKs, are a family of map kinases activated by cellular stress and inflammatory signals. Binding of TNF to the TNFR1 receptor activates the germinal center kinase (GCK) through the TNF adaptor Traf2, activating the map kinase MEKK1. Both GCK and MEKK1 interact with Traf2, andGCK is required for MEKK1 activation by TNF, but GCK kinase activity does not appear to be required for MEKK1 activation. Instead, GCK activates MEKK1 by causing MEKK1 oligomerization and autophosphorylation. Tank increases the affinity of Traf2 for GCK to increase Map kinase activation by TNF. Once activated, MEKK1 stands at the top of a map kinase pathways leading to transcriptional regulation, including JNK phosphorylation of c-Jun to stimulate transcriptional activation by AP-1, a heterodimer of c-jun and fos or ATF proteins. The activation of the p38 Map kinase also contributes to AP-1 activation leading to the transcriptional activation of many stress and growth related genes. RIP has been suggested as a component of the p38 pathway in addition to playing a role in NF-kB activation. MEKK1 knockout mice support the role of MEKK1 in JNK activation in some cells but did not support MEKK1 dependent activation of NF-kB. Alternative redundant mechanisms may obscure the role of MEKK1 in NF-kB mechanisms. TNF activation of stress kinase pathways and downstream transcription factors may help to modulate the apoptotic pathways also activated by TNF.共刺激信号For a T cell to be activated by a specific antigen, the T cell receptor must recognize complexes of MHCI with the antigen on the surface of an antigen-presenting cell. T cells and the T cell receptor complex do not respond to antigen in solution, but even for the specific antigen they only respond to antigen-MHC-1 complexes on the cell surface. This interaction is necessary for T cell activation, but it is not sufficient. T cell activation also requires a co-stimulatory signal involving interaction of CD28 on the T cell with CD80 or CD86 (B7 family genes) on the antigen-presenting cell.CD28 activates a signal transduction pathway acting through PI-3K, Lck andGrb-2/ITK to provide its co-stimulatory signal for T cell activation. Another means to control T cell activation is by expressing factors that down-regulate T cell activation. Signaling by activated T cell receptors induces expression of CTLA-4, a receptor that opposes T cell activation. CTLA-4 has a higher affinity than CD28 for B7 proteins, terminating T cell activation. ICOS is a protein related to CD28 that is only expressed on activated T cells, and that provides another important co-stimulatory signal. The requirement for co-stimulatory signals provides additional control mechanisms that prevent inappropriate and hazardous T cell activation.。
肿瘤细胞信号转导通路

肿瘤细胞的信号转导通路信号传导通路是将胞外刺激由细胞表面传入细胞内,启动了胞浆中的信号转导通路,通过多种途径将信号传递到胞核内,促进或抑制特定靶基因的表达。
一、MAPK信号通路MAPK信号通路介导细胞外信号到细胞内反应。
丝裂原活化蛋白激酶(mitogen activated protein kinase,MAPK)主要位于细胞浆,很多生长因子所激活,活化后既可以磷酸化胞浆内的靶蛋白,也能进入细胞核作用于对应的转录因子,调节靶基因的表达。
调节着细胞的生长、分化、分裂、死亡各个阶段的生理活动以及细胞间功能同步化过程,并在细胞恶变和肿瘤侵袭转移过程中起重要作用,阻断MAPK途径是肿瘤侵袭转移的治疗新方向。
MAPK信号转导通路是需要经过多级激酶的级联反应,其中包括3个关键的激酶,即MAPK激酶激酶(MKKK)→MAPK激酶(MKK)→MAPK。
(一)MKKK:包括Raf、Mos、Tpl、SPAK、MUK、MLK和MEKK等,其中Raf又分为A-Raf、B-Raf、Raf-1等亚型;MKKK是一个Ser/Thr蛋白激酶,被MAPKKKK、小G蛋白家族成员Ras、Rho激活后可Ser/Thr磷酸化激活下游激酶MKK。
MKK识别下游MAPK分子中的TXY序列(“Thr-X-Tyr”模序,为MAPK第Ⅷ区存在的三肽序列Thr-Glu-Tyr、Thr-Pro-Tyr或Thr-Gly-Tyr),将该序列中的Thr和Tyr分别磷酸化后激活MAPK。
注:TXY序列是MKK活化JNK的双磷酸化位点,MKK4和MKK7通过磷酸化TXY 序列的第183位苏氨酸残基(Thr183)和第185位酪氨酸残基(Tyr185)激活JNK1。
(二)MKK:包括MEK1-MEK7,主要是MEK1/2;(三)MAPK:MAPK是一类丝氨酸/苏氨酸激酶,是MAPK途径的核心,它至少由4种同功酶组成,包括:细胞外信号调节激酶(Extracellular signal Regulated Kinases,ERK1/2)、C-Jun 氨基末端激酶(JNK)/应激激活蛋白激酶(Stress-activated protein kinase,SAPK)、p38(p38MAPK)、ERK5/BMK1(big MAP kinase1)等MAPK亚族,并根据此将MAPK 信号传导通路分为4条途径。
细胞信号通路大全
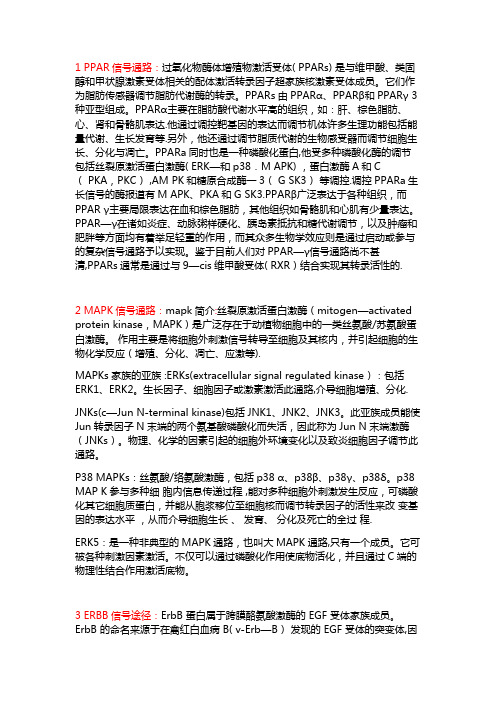
1 PPAR信号通路:过氧化物酶体增殖物激活受体( PPARs) 是与维甲酸、类固醇和甲状腺激素受体相关的配体激活转录因子超家族核激素受体成员。
它们作为脂肪传感器调节脂肪代谢酶的转录。
PPARs由PPARα、PPARβ和PPARγ 3种亚型组成。
PPARα主要在脂肪酸代谢水平高的组织,如:肝、棕色脂肪、心、肾和骨骼肌表达.他通过调控靶基因的表达而调节机体许多生理功能包括能量代谢、生长发育等.另外,他还通过调节脂质代谢的生物感受器而调节细胞生长、分化与凋亡。
PPARa同时也是一种磷酸化蛋白,他受多种磷酸化酶的调节包括丝裂原激活蛋白激酶( ERK—和p38.M APK) ,蛋白激酶A和C( PKA,PKC) ,AM PK和糖原合成酶一3( G SK3)等调控.调控PPARa生长信号的酶报道有M APK、PKA和G SK3.PPARβ广泛表达于各种组织,而PPAR γ主要局限表达在血和棕色脂肪,其他组织如骨骼肌和心肌有少量表达。
PPAR—γ在诸如炎症、动脉粥样硬化、胰岛素抵抗和糖代谢调节,以及肿瘤和肥胖等方面均有着举足轻重的作用,而其众多生物学效应则是通过启动或参与的复杂信号通路予以实现。
鉴于目前人们对PPAR—γ信号通路尚不甚清,PPARs通常是通过与9—cis维甲酸受体( RXR)结合实现其转录活性的.2 MAPK信号通路:mapk简介:丝裂原激活蛋白激酶(mitogen—activated protein kinase,MAPK)是广泛存在于动植物细胞中的一类丝氨酸/苏氨酸蛋白激酶。
作用主要是将细胞外刺激信号转导至细胞及其核内,并引起细胞的生物化学反应(增殖、分化、凋亡、应激等).MAPKs家族的亚族 :ERKs(extracellular signal regulated kinase):包括ERK1、ERK2。
生长因子、细胞因子或激素激活此通路,介导细胞增殖、分化. JNKs(c—Jun N-terminal kinase)包括JNK1、JNK2、JNK3。
细胞常见信号通路图片合集
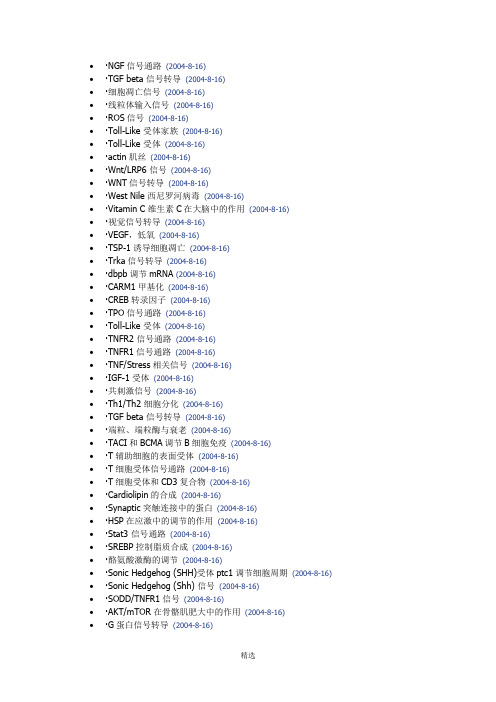
•·NGF信号通路(2004-8-16)•·TGF beta 信号转导(2004-8-16)•·细胞凋亡信号(2004-8-16)•·线粒体输入信号(2004-8-16)•·ROS信号(2004-8-16)•·Toll-Like 受体家族(2004-8-16)•·Toll-Like 受体(2004-8-16)•·actin肌丝(2004-8-16)•·Wnt/LRP6 信号(2004-8-16)•·WNT信号转导(2004-8-16)•·West Nile 西尼罗河病毒(2004-8-16)•·Vitamin C 维生素C在大脑中的作用(2004-8-16)•·视觉信号转导(2004-8-16)•·VEGF,低氧(2004-8-16)•·TSP-1诱导细胞凋亡(2004-8-16)•·Trka信号转导(2004-8-16)•·dbpb调节mRNA (2004-8-16)•·CARM1甲基化(2004-8-16)•·CREB转录因子(2004-8-16)•·TPO信号通路(2004-8-16)•·Toll-Like 受体(2004-8-16)•·TNFR2 信号通路(2004-8-16)•·TNFR1信号通路(2004-8-16)•·TNF/Stress相关信号(2004-8-16)•·IGF-1受体(2004-8-16)•·共刺激信号(2004-8-16)•·Th1/Th2 细胞分化(2004-8-16)•·TGF beta 信号转导(2004-8-16)•·端粒、端粒酶与衰老(2004-8-16)•·TACI和BCMA调节B细胞免疫(2004-8-16)•·T辅助细胞的表面受体(2004-8-16)•·T细胞受体信号通路(2004-8-16)•·T细胞受体和CD3复合物(2004-8-16)•·Cardiolipin的合成(2004-8-16)•·Synaptic突触连接中的蛋白(2004-8-16)•·HSP在应激中的调节的作用(2004-8-16)•·Stat3 信号通路(2004-8-16)•·SREBP控制脂质合成(2004-8-16)•·酪氨酸激酶的调节(2004-8-16)•·Sonic Hedgehog (SHH)受体ptc1调节细胞周期(2004-8-16)•·Sonic Hedgehog (Shh) 信号(2004-8-16)•·SODD/TNFR1信号(2004-8-16)•·AKT/mTOR在骨骼肌肥大中的作用(2004-8-16)•·G蛋白信号转导(2004-8-16)•·肝细胞生长因子受体信号(2004-8-16)•·IL1受体信号转导(2004-8-16)•·acetyl从线粒体到胞浆过程(2004-8-16)•·趋化因子chemokine在T细胞极化中的选择性表(2004-8-16)•·SARS冠状病毒蛋白酶(2004-8-16)•·Parkin在泛素-蛋白酶体中的作用(2004-8-16)•·nicotinic acetylcholine受体在凋亡中的作用(2004-8-16)•·线粒体在细胞凋亡中的作用(2004-8-16)•·MEF2D在T细胞凋亡中的作用(2004-8-16)•·Erk5和神经元生存(2004-8-16)•·ERBB2信号转导(2004-8-16)•·GPCRs调节EGF受体(2004-8-16)•·BRCA1调节肿瘤敏感性(2004-8-16)•·Rho细胞运动的信号(2004-8-16)•·Leptin能逆转胰岛素抵抗(2004-8-16)•·转录因子DREAM调节疼敏感(2004-8-16)•·PML调节转录(2004-8-16)•·p27调节细胞周期(2004-8-16)•·MAPK信号调节(2004-8-16)•·细胞因子调节造血细胞分化(2004-8-16)•·eIF4e和p70 S6激酶调节(2004-8-16)•·eIF2调节(2004-8-16)•·谷氨酸受体调节ck1/cdk5 (2004-8-16)•·plk3在细胞周期中的作用(2004-8-1)•·BAD磷酸化调节(2004-8-1)•·Reelin信号通路(2004-8-1)•·RB肿瘤抑制和DNA破坏(2004-8-1)•·NK细胞介导的细胞毒作用(2004-8-1)•·Ras信号通路(2004-8-1)•·Rac 1细胞运动信号(2004-8-1)•·PTEN依赖的细胞生长抑制和细胞凋亡(2004-8-1)•·notch信号通路(2004-8-1)•·蛋白激酶A(PKA)在中心粒中的作用(2004-8-1)•·蛋白酶体Proteasome复合物(2004-8-1)•·Prion朊病毒的信号通路(2004-8-1)•·早老素Presenilin在notch和wnt信号中的作用(2004-8-1)•·mRNA的poly(A)形成(2004-8-1)•·淀粉样蛋白前体信号(2004-8-1)•·PKC抑制myosin磷酸化(2004-8-1)•·磷脂酶C(PLC)信号(2004-8-1)•·巨噬细胞Pertussis toxin不敏感的CCR5信号通(2004-8-1)•·Pelp1调节雌激素受体的活性(2004-8-1)•·PDGF信号通路(2004-8-1)•·p53信号通路(2004-8-1)•·p38MAPK信号通路(2004-8-1)•·Nrf2是氧化应激基本表达的关键基因(2004-8-1)•·OX40信号通路(2004-8-1)•·hTerc转录调节活性图(2004-8-1)•·hTert转录因子的调节作用(2004-8-1)•·AIF在细胞凋亡中的作用(2004-8-1)•·Omega氧化通路(2004-8-1)•·核受体在脂质代谢和毒性中的作用(2004-8-1)•·NK细胞中NO2依赖的IL-12信号通路(2004-8-1)•·TOR信号通路(2004-8-1)•·NO信号通路(2004-8-1)•·NF-kB信号转导通路(2004-8-1)•·NFAT与心肌肥厚的示意图(2004-8-1)•·神经营养素及其表面分子(2004-8-1)•·神经肽VIP和PACAP防止活化T细胞凋亡图(2004-8-1)•·神经生长因子信号图(2004-8-1)•·线虫和哺乳动物的MAPK信号比较(2004-7-17)•·细胞内信号总论(2004-7-17)•·细胞凋亡信号通路(2004-7-17)•·MAPK级联通路(2004-7-17)•·MAPK信号通路图(2004-7-17)•·BCR信号通路(2004-7-17)•·蛋白质乙酰化示意图(2004-7-17)•·wnt信号通路(2004-7-17)•·胰岛素受体信号通路(2004-7-17)•·细胞周期在G2/M期的调控机理图(2004-7-17)•·细胞周期G1/S检查点调控机理图(2004-7-17)•·Jak-STAT关系总表(2004-7-17)•·Jak/STAT 信号(2004-7-17)•·TGFbeta信号(2004-7-17)•·NFkappaB信号(2004-7-17)•·p38 MAPK信号通路(2004-7-17)•·SAPK/JNK 信号级联通路(2004-7-17)•·从G蛋白偶联受体到MAPK (2004-7-17)•·MAPK级联信号图(2004-7-17)•·eIF-4E和p70 S6激酶调控蛋白质翻译(2004-7-17)•·eif2蛋白质翻译(2004-7-17)•·蛋白质翻译示意图(2004-7-17)•·线粒体凋亡通路(2004-7-17)•·死亡受体信号通路(2004-7-17)•·凋亡抑制通路(2004-7-17)•·细胞凋亡综合示意图(2004-7-17)•·Akt/Pkb信号通路(2004-7-17)•·MAPK/ERK信号通路(2004-7-17)•·哺乳动物MAPK信号通路(2004-7-17)•·Pitx2多步调节基因转录(2004-7-17)•·IGF-1R导致BAD磷酸化的多个凋亡路径(2004-7-17)•·多重耐药因子(2004-7-17)•·mTOR信号通路(2004-7-17)•·Msp/Ron受体信号通路(2004-7-17)•·单核细胞和其表面分子(2004-7-17)•·线粒体的肉毒碱转移酶(CPT)系统(2004-7-17)•·METS影响巨噬细胞的分化(2004-7-17)•·Anandamide,内源性大麻醇的代谢(2004-7-17)•·黑色素细胞(Melanocyte)发育和信号(2004-7-17)•·DNA甲基化导致转录抑制的机理图(2004-7-17)•·蛋白质的核输入信号图(2004-7-17)•·PPARa调节过氧化物酶体的增殖(2004-7-17)•·对乙氨基酚(Acetaminophen)的活性和毒性机(2004-7-17)•·mCalpain在细胞运动中的作用(2004-7-17)•·MAPK信号图(2004-7-17)•·MAPK抑制SMRT活化(2004-7-17)•·苹果酸和天门冬酸间的转化(2004-7-17)•·低密度脂蛋白(LDL)在动脉粥样硬化中的作用(2004-7-17)•·LIS1基因在神经细胞的发育和迁移中的作用图(2004-7-17)•·Pyk2与Mapk相连的信号通路(2004-7-17)•·galactose代谢通路(2004-7-17)•·Lectin诱导补体的通路(2004-7-17)•·Lck和Fyn在TCR活化中的作用(2004-7-17)•·乳酸合成图(2004-7-17)•·Keratinocyte分化图(2004-7-17)•·离子通道在心血管内皮细胞中的作用(2004-7-17)•·离子通道和佛波脂(Phorbal Esters)信号(2004-7-17)•·内源性Prothrombin激活通路(2004-7-17)•·Ribosome内化通路(2004-7-17)•·整合素(Integrin)信号通路(2004-7-17)•·胰岛素(Insulin)信号通路(2004-7-17)•·Matrix Metalloproteinases (2004-7-17)•·组氨酸去乙酰化抑制剂抑制Huntington病(2004-7-17)•·Gleevec诱导细胞增殖(2004-7-17)•·Ras和Rho在细胞周期的G1/S转换中的作用(2004-7-17)•·DR3,4,5受体诱导细胞凋亡(2004-7-17)•·AKT调控Gsk3图(2004-7-17)•·IL-7信号转导(2004-7-17)•·IL22可溶性受体信号转导图(2004-7-17)•·IL-2活化T细胞图(2004-7-17)•·IL12和Stat4依赖的TH1细胞发育信号通路(2004-7-17)•·IL-10信号通路(2004-7-17)•·IL 6信号通路(2004-7-17)•·IL 5信号通路(2004-7-17)•·IL 4信号通路(2004-7-17)•·IL 3信号通路(2004-7-17)•·IL 2 信号通路(2004-7-17)•·IL 18信号通路(2004-7-17)•·IL 17信号通路(2004-7-17)•·IGF-1信号通路(2004-7-17)•·IFN gamma信号通路(2004-7-17)•·INF信号通路(2004-7-17)•·低氧诱导因子(HIF)在心血管中的作用(2004-7-17)•·低氧和P53在心血管系统中的作用(2004-7-17)•·人类巨细胞病毒和MAP信号通路(2004-7-17)•·孕酮如何促进卵细胞成熟?(2004-7-17)•·How does salmonella hijack a cell (2004-7-17)•·Hop通路在心脏发育中的作用(2004-7-17)•·HIV-I Nef:负性调节fas和TNF (2004-7-17)•·HIV-1防止宿主细胞耐受的机理(2004-7-17)•·HIV诱导T细胞凋亡图(2004-7-17)•·血红素的伴侣分子(2004-7-17)•·g-Secretase介导ErbB4信号通路(2004-7-17)•·生物激素信号(2004-7-17)•·Granzyme A介导的凋亡信号通路(2004-7-17)•·G蛋白偶联信号需要Tubby支持(2004-7-17)•·糖酵解通路(2004-7-17)•·Ghrelin:食物吸收和能量平衡的调控者(2004-7-17)•·PS1能产生beta淀粉样蛋白导致老年性痴呆(2004-7-17)•·GATA3部分参与TH2细胞因子基因的表达(2004-7-17)•·GABA受体的代谢图(2004-7-17)•·FXR和LXR调节胆固醇代谢(2004-7-17)•·SLRP在骨骼中的作用(2004-7-17)•·自由基诱导细胞凋亡信号(2004-7-17)•·FOSB与药物成瘾(2004-7-17)•·fMLP诱导趋化因子基因表达(2004-7-17)•·Fibrinolysis通路(2004-7-17)•·糖酵解通路(2004-7-17)•·Fc Epsilon Receptor I信号(2004-7-17)•·FAS信号通路(2004-7-17)•·外源性Prothrombin激活通路(2004-7-17)•·真核细胞蛋白质翻译示意图(2004-7-17)•·雌激素反应蛋白EFP控制乳腺癌细胞的细胞周期(2004-7-17)•·EPO介导神经保护作用与NF-kB相关(2004-7-17)•·Erythrocyte分化通路(2004-7-17)•·Erk1/Erk2 Mapk 信号通路(2004-7-17)•·Erk和PI-3K在细胞外间质中的作用(2004-7-17)•·内质网相关的蛋白质降解通路示意图(2004-7-17)•·EPO售转导机制图(2004-7-17)•·血小板凝聚示意图(2004-7-17)•·NDK动力学(2004-7-17)•·线粒体的电子传递链示意图(2004-7-17)•·Eicosanoid代谢(2004-7-17)•·EGF信号通路(2004-7-17)•·calcineurin对Keratinocyte分化的影响(2004-7-17)•·E2F1信号通路(2004-7-17)•·MTA-3在雌激素不敏感性乳腺癌中下调(2004-7-17)•·双链RNA诱导基因表达示意图(2004-7-17)•·Dicer信号通路(RNAi机理)(2004-7-17)•·CDK5在老年性痴呆中的调节作用(2004-7-17)•·树突状细胞调节TH1和TH2发育示意图(2004-7-17)•·RAR和RXR被蛋白酶体降解通路(2004-7-17)•·D4-GDI信号通路示意图(2004-7-17)•·细胞因子和炎症反应示意图(2004-7-9)•·细胞因子网络调控图(2004-7-9)•·CFTR和beta 2肾上腺素受体通路(2004-7-9)•·Cyclin和细胞周期调控图(2004-7-9)•·Ran核质循环转运图(2004-7-9)•·Cyclin E降解通路图(2004-7-9)•·CXCR4信号通路图(2004-7-9)•·CTL介导的免疫反应图(2004-7-9)•·CTCF:第一个多价核因子(2004-7-9)•·皮质激素和心脏保护(2004-7-9)•·骨骼肌的成肌信号图(2004-7-9)•·VitD调控基因表达信号图(2004-7-9)•·补体信号通路(2004-7-9)•·线粒体和过氧化物酶体中β氧化的比较图(2004-7-9)•·经典的补体信号通路图(2004-7-9)•·心律失常的分子机制图(2004-7-9)•·hSWI/SNF ATP依赖的染色体重塑(2004-7-9)•·碳水化合物和cAMP调节ChREBP图(2004-7-9)•·分子伴侣调节干扰素信号图(2004-7-9)•·Ceramide信号图(2004-7-9)•·局部急性感染的细胞与分子信号(2004-7-9)•·细胞与细胞粘附信号(2004-7-9)•·细胞周期G2/M调控点信号调节(2004-7-9)•·细胞周期 G1/S调控点信号图(2004-7-9)•·CDK调节DNA复制(2004-7-9)•·cdc25和chk1在DNA破坏中的作用图(2004-7-9)•·CD40L信号通路图(2004-7-9)•·CCR3信号图(2004-7-9)•·CBL下调EGF受体的信号转导图(2004-7-9)•·一些氨基酸的代谢通路图 3 (2004-7-9)•·一些氨基酸的代谢通路图 2 (2004-7-9)•·一些氨基酸的代谢通路图(2004-7-9)•·Catabolic pathway for asparagine and asp (2004-7-9)•·Caspase 信号级联通路在细胞凋亡中的作用(2004-7-9)•·CARM1和雌激素的信号转导调控(2004-7-9)•·抗氧自由基的心脏保护作用信号转导图(2004-7-9)•·乙肝病毒中的钙信号调控(2004-7-9)•·镉诱导巨噬细胞的DNA合成和增殖(2004-7-9)•·Ca2+/CaM依赖的激活(2004-7-9)•·B细胞活化机理图(2004-6-9)•·BTG家族蛋白和细胞周期的调节(2004-6-9)•·BRCA1作用机理(2004-6-9)•·骨重塑示意图(2004-6-9)•·Botulinum Toxin阻断神经递质释放示意图(2004-6-9)•·缬氨酸的生物合成图(2004-6-9)•·Tryptophan在植物和细菌内的生物合成(2004-6-9)•·苏氨酸和蛋氨酸的体内合成示意图(2004-6-9)•·sphingolipids生物合成(2004-6-9)•·spermidine和spermine生物合成(2004-6-9)•·细菌体内合成脯氨酸的示意图(2004-6-9)•·苯丙氨酸和酪氨酸的生物合成(2004-6-9)•·神经递质的合成示意图(2004-6-9)•·赖氨酸生物合成图(2004-6-9)•·亮氨酸的体内生物合成图(2004-6-9)•·异亮氨酸的生物合成图(2004-6-9)•·甘氨酸和色氨酸的生物合成(2004-6-9)•·Cysteine在哺乳动物中的合成图(2004-6-9)•·Cysteine在细菌和植物内生物合成图(2004-6-9)•·Chorismate在细菌和植物内的生物合成(2004-6-9)•·Arginine在细菌内的生物合成(2004-6-9)•·生物活性肽诱导的通路(2004-6-9)•·脂肪酸的β氧化通路(2004-6-9)•·BCR信号通路示意图(2004-6-9)•·SUMOylation基本机理(2004-6-9)•·PPAR影响基因表达的基本信号机制图(2004-6-9)•·B淋巴细胞表面分子示意图(2004-6-9)•·B细胞生存信号通路(2004-6-5)•·B细胞信号通路的复杂性(2004-6-5)•·GPCR信号的衰减的机理(2004-6-4)•·ATM信号通路(2004-6-4)•·阿斯匹林的抗凝机理(2004-6-4)•·细胞凋亡信号调节DNA片段化(2004-6-4)•·细胞凋亡DNA片段化与组织稳态的机理(2004-6-4)•·反义核酸的作用机理---RNA polymerase III (2004-6-4)•·抗原递呈与处理信号图(2004-6-4)•·Antigen依赖的B细胞激活(2004-6-4)•·Anthrax Toxin Mechanism of Action (2004-6-4)•·血管紧张素转换酶2调节心脏功能(2004-6-4)•·Angiotensin II 介导JNK信号通路的激活(2004-6-4)•·Alternative Complement Pathway (2004-6-4)•·Alpha-synuclein和Parkin在怕金森病中的作用(2004-6-4)•·ALK在心肌细胞中的功能图(2004-6-4)•·AKT信号通路(2004-6-4)•·AKAP95在有丝分裂中的作用图(2004-6-4)•·Ahr信号转导图(2004-6-4)•·Agrin突触后的功能图(2004-6-4)•·ADP-Ribosylation 因子(2004-6-4)•·淋巴细胞粘附分子信号图(2004-6-4)•·Adhesion and Diapedesis of Lymphocytes (2004-6-4)•·Adhesion and Diapedesis of Granulocytes (2004-6-4)•·急性心肌梗死信号转导图(2004-6-4)•·src蛋白质激活图(2004-6-4)•·PKC与G蛋白耦联受体的关系(2004-6-4)•·cAMP依赖的CSK抑制T细胞功能示意图(2004-6-4)•·PKA功能示意图(2004-6-4)•·一氧化氮(NO)在心脏中的功能示意图(2004-6-4)•·RelA 在细胞核内乙酰化和去乙酰化(2004-6-4)actin肌丝Mammalian cell motility requires actin polymerization in the direction of movement to change membrane shape and extend cytoplasm into lamellipodia. The polymerization of actin to drive cell movement also involves branching of actin filaments into a network oriented with the growing ends of the fibers near the cell membrane. Manipulation of this process helps bacteria like Salmonella gain entry into cells they infect. Two of the proteins involved in the formation of Y branches and in cell motility are Arp2 and Arp3, both members of a large multiprotein complex containing several other polypeptides as well. The Arp2/3 complex is localized at the Y branch junction and induces actin polymerization. Activity of this complex is regulated by multiple different cell surface receptor signaling systems, activating WASP, and Arp2/3 in turn to cause changes in cell shape and cell motility. Wasp and its cousin Wave-1 interact with the Arp2/3 complex through the p21 component of the complex. The crystal structure of the Arp2/3 complex has revealed further insights into the nature of how the complex works.Activation by Wave-1, another member of the WASP family, also induces actin alterations in response to Rac1 signals upstream. Wave-1 is held in an inactive complex in the cytosol that is activated to allow Wave-1 to associate with Arp2/3. While WASP is activated by interaction with Cdc42, Wave-1, is activated by interaction with Rac1 and Nck. Wave-1 activation by Rac1 and Nck releases Wave-1 with Hspc300 to activate actin Y branching and polymerization by Arp2/3. Different members of this gene family may produce different actin cytoskeletal architectures. The immunological defects associated with mutation of the WASP gene, theWiskott-Aldrich syndrome for which WASP was named, indicates the importance of this system for normal cellular function.Cory GO, Ridley AJ. Cell motility: braking WAVEs. Nature. 2002 Aug 15;418(6899):732-3. No abstract available.Eden, S., et al. (2002) Mechanism of regulation of WAVE1-induced actin nucleation by Rac1 and Nck. Nature 418(6899), 790-3Falet H, Hoffmeister KM, Neujahr R, Hartwig JH. Normal Arp2/3 complex activation in platelets lacking WASp. Blood. 2002 Sep 15;100(6):2113-22.Kreishman-Deitrick M, Rosen MK, Kreishman-Deltrick M. Ignition of a cellular machine. Nat Cell Biol. 2002 Feb;4(2):E31-3. No abstract available.Machesky, L.M., Insall, R.H. (1998) Scar1 and the related Wiskott-Aldrich syndrome protein, WASP, regulate the actin cytoskeleton through the Arp2/3 complex. Curr Biol 8(25), 1347-56Robinson, R.C. et al. (2001) Crystal structure of Arp2/3 complex. Science 294(5547), 1679-84Weeds A, Yeoh S. Structure. Action at the Y-branch. Science. 2001 Nov 23;294(5547):1660-1. No abstract available.Wnt/LRP6 信号Wnt glycoproteins play a role in diverse processes during embryonic patterning in metazoa through interaction with frizzled-type seven-transmembrane-domain receptors (Frz) to stabilize b-catenin. LDL-receptor-related protein 6 (LRP6), a Wnt co-receptor, is required for this interaction. Dikkopf (dkk) proteins are both positive and negative modulators of this signalingWNT信号转导West Nile 西尼罗河病毒West Nile virus (WNV) is a member of the Flaviviridae, a plus-stranded virus family that includes St. Louis encephalitis virus, Kunjin virus, yellow fever virus, Dengue virus, and Japanese encephalitis virus. WNV was initially isolated in 1937 in the West Nile region of Uganda and has become prevalent in Africa, Asia, and Europe. WNV has rapidly spread across the United States through its insect host and causes neurological symptoms and encephalitis, which can result in paralysis or death. Since 1999 about 3700 cases of West Nile virus (WNV) infection and 200 deaths have been recorded in United States. The viral capsid protein likely contributes to the WNV-associated deadly inflammation via apoptosis induced through the mitochondrial pathway.WNV particles (50 nm in diameter) consist of a dense core (viral protein C encapsidated virus RNA genome)surrounded by a membrane envelope (viral E and M proteins embedded in a lipid bilayer). The virus binds to a specific cell surface protein (not yet identified), an interaction thought to involve E protein with highly sulfated neperan sulfate (HSHS) residues that are present on the surfaces of many cells and enters the cell by a process similar to that of endocytosis. Once inside the cell, the genome RNA is released into the cytoplasm via endosomal release, a fusion process involving acidic pH induced conformation change in the E protein. The RNA genome serves as mRNA and is translated by ribosomes into ten mature viral proteins are produced via proteolytic cleavage, which include three structural components and seven different nonstructural components of the virus. These proteins assemble and transcribe complimentary minus strand RNAs from the genomic RNA. The complimentary minus strand RNA in turns serves as template for the synthesis of positive-stranded genomic RNAs. Once viral E, preM and C proteins have accumulated to sufficient level, they assemble with the genomic RNA to form progeny virions, which migrate to the cell surface where they are surrounded with lipid envelop and released.Vitamin C 维生素C在大脑中的作用Vitamin C (ascorbic acid) was first identified by virtue of the essential role it plays in collagen modification, preventing the nutritional deficiency scurvy. Vitamin C acts as a cofactor for hydroxylase enzymes thatpost-translationally modify collagen to increase the strength and elasticity of tissues. Vitamin C reduces the metal ion prosthetic groups of many enzymes, maintaining activity of enzymes, also acts as an anti-oxidant. Although the prevention of scurvy through modification of collagen may be the most obvious role for vitamin C, it is not necessarily the only role of vitamin C. Svct1 and Svct2 are ascorbate transporters for vitamin C import into tissues and into cells. Both of these transporters specifically transport reduced L-ascorbic acid against a concentration gradient using the intracellular sodium gradient to drive ascorbate transport. Svct1 is expressed in epithelial cells in the intestine, upregulated in cellular models for intestinal epithelium and appears to be responsible for the import of dietary vitamin C from the intestinal lumen. The vitamin C imported from the intestine is present in plasma at approximately 50 uM, almost exclusively in the reduced form, and is transported to tissues to play a variety of roles. Svct2 imports reduced ascorbate from the plasma into veryactive tissues like the brain. Deletion in mice of the gene for Svct2 revealed that ascorbate is required for normal development of the lungs and brain during pregnancy. A high concentration of vitamin C in neurons of the developing brain may help protect the developing brain from free radical damage. The oxidized form of ascorbate, dehydroascorbic acid, is transported into a variety of cells by the glucose transporter Glut-1. Glut-1, Glut-3 and Glut-4 can transport dehydroascorbate, but may not transport significant quantities of ascorbic acid in vivo.视觉信号转导信息来源:本站原创生物谷网站The signal transduction cascade responsible for sensing light in vertebrates is one of the best studied signal transduction processes, and is initiated by rhodopsin in rod cells, a member of the G-protein coupled receptor gene family. Rhodopsin remains the only GPCR whose structure has been resolved at high resolution. Rhodopsinin the discs of rod cells contains a bound 11-cis retinal chromophore, a small molecule derived from Vitamin A that acts as the light sensitive portion of the receptor molecule, absorbing light to initiate the signal transduction cascade. When light strikes 11-cis retinal and is absorbed, it isomerizes to all-trans retinal, changing the shape of the molecule and the receptor it is bound to. This change in rhodopsin抯shape alters its interaction with transducin, the member of the G-protein gene family that is specific in its role in visual signal transduction. Activation of transducin causes its alpha subunit to dissociate from the trimer and exchange bound GDP for GTP, activating in turn a membrane-bound cyclic-GMP specific phosphodiesterase that hydrolyzes cGMP. In the resting rod cell, high levels of cGMP associate with a cyclic-GMP gated sodium channel in the plasma membrane, keeping the channels open and the membrane of the resting rod cells depolarized. This is distinct from synaptic generation of action potentials, in which stimulation induces opening of sodium channels and depolarization. When cGMP gated channels in rod cells open, both sodium and calcium ions enter the cell, hyperpolarizing the membrane and initiating the electrochemical impulse responsible for conveying the signal from the sensory neuron to the CNS. The rod cell in the resting state releases high levels of the inhibitory neurotransmitter glutamate, while the release of glutamate is repressed by the hyperpolarization in the presence of light to trigger a downstream action potential by ganglion cells that convey signals to the brain. The calcium which enters the cell also activates GCAP, which activates guanylate cyclase (GC-1 and GC-2) to rapidly produce more cGMP, ending the hyperpolarization and returning the cell to its resting depolarized state. A protein called recoverin helps mediate the inactivation of the signal transduction cascade, returning rhodopsin to its preactivated state, along with the rhodopsin kinase Grk1. Phosphorylation of rhodopsin by Grkl causes arrestin to bind, helping to terminate the receptor activation signal. Dissociation and reassociation of retinal, dephosphorylation of rhodopsin and release of arrestin all return rhodopsin to its ready state, prepared once again to respond to light.VEGF,低氧信息来源:本站原创生物谷网站Vascular endothelial growth factor (VEGF) plays a key role in physiological blood vessel formation and pathological angiogenesis such as tumor growth and ischemic diseases. Hypoxia is a potent inducer of VEGF in vitro. The increase in secreted biologically active VEGF protein from cells exposed to hypoxia is partly because of an increased transcription rate, mediated by binding of hypoxia-inducible factor-1 (HIF1) to a hypoxia responsive element in the 5'-flanking region of the VEGF gene. bHLH-PAS transcription factor that interacts with the Ah receptor nuclear translocator (Arnt), and its predicted amino acid sequence exhibits significant similarity to the hypoxia-inducible factor 1alpha (HIF1a) product. HLF mRNA expression is closely correlated with that of VEGF mRNA.. The high expression level of HLF mRNA in the O2 delivery system of developing embryos and adult organs suggests that in a normoxic state, HLF regulates gene expression of VEGF, various glycolytic enzymes, and others driven by the HRE sequence, and may be involved in development of blood vessels and the tubularsystem of lung. VEGF expression is dramatically induced by hypoxia due in large part to an increase in the stability of its mRNA. HuR binds with high affinity and specificity to the VRS element that regulates VEGF mRNA stability by hypoxia. In addition, an internal ribosome entry site (IRES) ensures efficient translation of VEGF mRNA even under hypoxia. The VHL tumor suppressor (von Hippel-Lindau) regulates also VEGF expression at a post-transcriptional level. The secreted VEGF is a major angiogenic factor that regulates multiple endothelial cell functions, including mitogenesis. Cellular and circulating levels of VEGF are elevated in hematologic malignancies and are adversely associated with prognosis. Angiogenesis is a very complex, tightly regulated, multistep process, the targeting of which may well prove useful in the creation of novel therapeutic agents. Current approaches being investigated include the inhibition of angiogenesis stimulants (e.g., VEGF), or their receptors, blockade of endothelial cell activation, inhibition of matrix metalloproteinases, and inhibition of tumor vasculature. Preclinical, phase I, and phase II studies of both monoclonal antibodies to VEGF and blockers of the VEGF receptor tyrosine kinase pathway indicate that these agents are safe and offer potential clinical utility in patients with hematologic malignancies.TSP-1诱导细胞凋亡信息来源:本站原创生物谷网站As tissues grow they require angiogenesis to occur if they are to be supplied with blood vessels and survive. Factors that inhibit angiogenesis might act as cancer therapeutics by blocking vessel formation in tumors and starving cancer cells. Thrombospondin-1 (TSP-1) is a protein that inhibits angiogenesis and slows tumor growth, apparently by inducing apoptosis of microvascular endothelial cells that line blood vessels. TSP-1 appears to produce this response by activating a signaling pathway that begins with its receptor CD36 at the cell surface of the microvascular endothelial cell. The non-receptor tyrosine kinase fyn is activated by TSP-1 through CD36, activating the apoptosis inducing proteases like caspase-3 and p38 protein kinases. p38 is a mitogen-activated kinase that also induces apoptosis in some conditions, perhaps through AP-1 activation and the activation of genes that lead to apoptosis.Trka信号转导信息来源:本站原创生物谷网站Nerve growth factor (NGF) is a neurotrophic factor that stimulates neuronal survival and growth through TrkA, a member of the trk family of tyrosine kinase receptors that also includes TrkB and TrkC. Some NGF responses are also mediated or modified by p75LNTR, a low affinity neurotrophin receptor. Binding of NGF to TrkA stimulates neuronal survival, and also proliferation. Pathways coupled to these responses are linked to TrkAthrough association of signaling factors with specific amino acids in the TrkA cytoplasmic domain. Cell survival through inhibition of apoptosis is signaled through activation of PI3-kinase and AKT. Ras-mediated signaling and phospholipase C both activate the MAP kinase pathway to stimulate proliferation.dbpb调节mRNA信息来源:本站原创生物谷网站Endothelial cells respond to treatment with the protease thrombin with increased secretion of the PDGF B-chain. This activation occurs at the transcriptional level and a thrombin response element was identified in the promoter of the PDGF B-chain gene. A transcription factor called the DNA-binding protein B (dbpB) mediates the activation of PDGF B-chain transcription in response to thrombin treatment. DbpB is a member of the Y box family of transcription factors and binds to both RNA and DNA. In the absence of thrombin, endothelial cells contain a 50 kD form of dbpB that binds RNA in the cytoplasm and may play a role as a chaperone for mRNA. The 50 kD version of dbpB also binds DNA to regulate genes containing Y box elements in their promoters. Thrombin activation results in the cleavage of dbpB to a 30 kD form. The proteolytic cleavage releases dbpB from RNA in the nucleus, allowing it to enter the nucleus and binds to a regulatory element distinct from the site recognized by the full length 50 kD dbpB. The genes activated by cleaved dbpB include the PDGF B chain. Dephosphorylation of dbpB also regulates nuclear entry and transcriptional activation.RNA digestion in vitro can release dbpB in its active form, suggesting that the protease responsible for dbpB may be closely associated in a complex. Identification of the protease that cleaves dbpB, the mechanisms of phosphorylation and dephosphorylation, and elucidation of the signaling path by which thrombin induces dbpB will provide greater understanding of this novel signaling pathway.CARM1甲基化信息来源:本站原创生物谷网站Several forms of post-translational modification regulate protein activities. Recently, protein methylation by CARM1 (coactivator-associated arginine methyltransferase 1) has been observed to play a key role in transcriptional regulation. CARM1 associates with the p160 class of transcriptional coactivators involved in gene activation by steroid hormone family receptors. CARM1 also interacts with CBP/p300 transcriptional coactivators involved in gene activation by a large variety of transcription factors, including steroid hormone receptors and CEBP. One target of CARM1 is the core histones H3 and H4, which are also targets of the histone acetylase activity of CBP/p300 coactivators. Recruitment of CARM1 to the promoter region by binding to coactivators increases histone methylation and makes promoter regions more accessible for transcription. Another target of CARM1 methylation is a coactivator it interacts with, CBP. Methylation of CBP by CARM1 blocks。
帕金森病中的CDK5相关通路
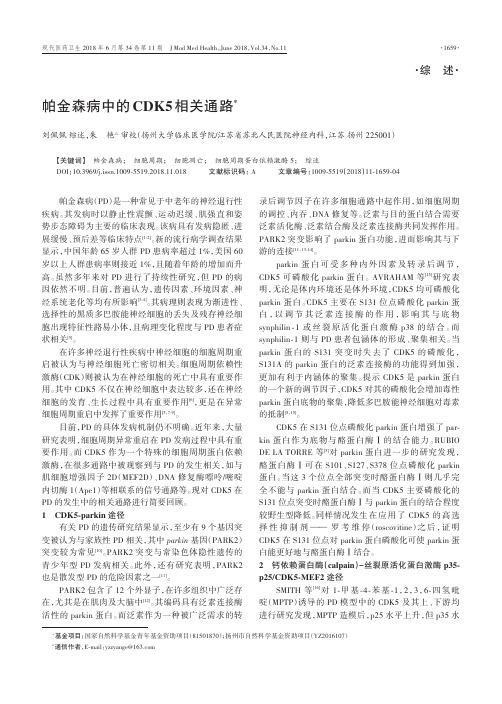
录后调节因子在许多细胞通路中起作用,如细胞周期 的调控、内吞、DNA 修复等。泛素与目的蛋白结合需要 泛素活化酶、泛素结合酶及泛素连接酶共同发挥作用。 PARK2 突变影响了 parkin 蛋白功能,进而影响其与下 游的连接[11,13⁃14]。
parkin 蛋 白 可 受 多 种 内 外 因 素 及 转 录 后 调 节 , CDK5 可 磷 酸 化 parkin 蛋 白 。AVRAHAM 等[15]研 究 表 明,无论是体内环境还是体外环境,CDK5 均可磷酸化 parkin 蛋白。CDK5 主要在 S131 位点磷酸化 parkin 蛋 白 ,以 调 节 其 泛 素 连 接 酶 的 作 用 ,影 响 其 与 底 物 synphilin ⁃ 1 或 丝 裂 原 活 化 蛋 白 激 酶 p38 的 结 合 。而 synphilin⁃1 则与 PD 患者包涵体的形成、聚集相关。当 parkin 蛋 白 的 S131 突 变 时 失 去 了 CDK5 的 磷 酸 化 , S131A 的 parkin 蛋白的泛素连接酶的功能得到加强, 更加有利于内涵体的聚集。提示 CDK5 是 parkin 蛋白 的一个新的调节因子,CDK5 对其的磷酸化会增加毒性 parkin 蛋白底物的聚集,降低多巴胺能神经细胞对毒素 的抵制[5,15]。
DOI:10.3969/j.issn.1009⁃5519.2018.11.018
文献标识码:A
文章编号:1009⁃5519(2018)11⁃1659⁃04
帕金森病(PD)是一种常见于中老年的神经退行性 疾病。其发病时以静止性震颤、运动迟缓、肌强直和姿 势步态障碍为主要的临床表现。该病具有发病隐匿、进 展缓慢、预后差等临床特点[1⁃2]。新的流行病学调查结果 显示,中国年龄 65 岁人群 PD 患病率超过 1%,美国 60 岁以上人群患病率则接近 1%,且随着年龄的增加而升 高。虽然多年来对 PD 进行了持续性研究,但 PD 的病 因依然不明。目前,普遍认为,遗传因素、环境因素、神 经系统老化等均有所影响[3⁃4]。其病理则表现为渐进性、 选择性的黑质多巴胺能神经细胞的丢失及残存神经细 胞出现特征性路易小体,且病理变化程度与 PD 患者症 状相关[5]。
细胞周期信号转导通路
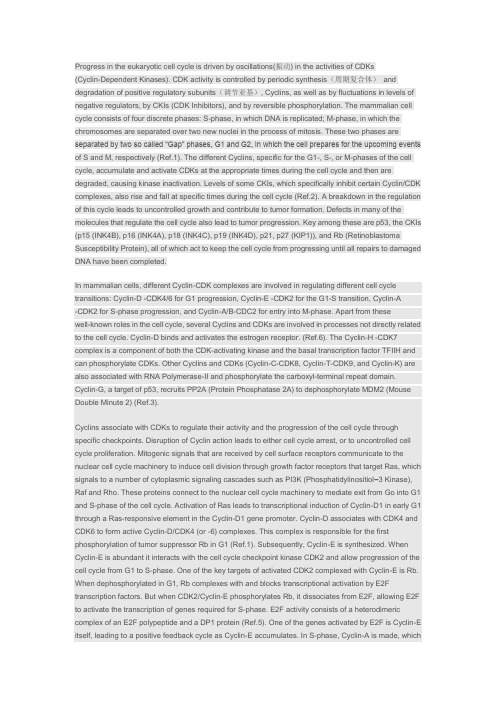
Progress in the eukaryotic cell cycle is driven by oscillations(振动) in the activities of CDKs(Cyclin-Dependent Kinases). CDK activity is controlled by periodic synthesis(周期复合体)and degradation of positive regulatory subunits(调节亚基), Cyclins, as well as by fluctuations in levels of negative regulators, by CKIs (CDK Inhibitors), and by reversible phosphorylation. The mammalian cell cycle consists of four discrete phases: S-phase, in which DNA is replicated; M-phase, in which the chromosomes are separated over two new nuclei in the process of mitosis. These two phases are separated by two so called “Gap” phases, G1 and G2, in which the cell prepares for the upcoming events of S and M, respectively (Ref.1). The different Cyclins, specific for the G1-, S-, or M-phases of the cell cycle, accumulate and activate CDKs at the appropriate times during the cell cycle and then are degraded, causing kinase inactivation. Levels of some CKIs, which specifically inhibit certain Cyclin/CDK complexes, also rise and fall at specific times during the cell cycle (Ref.2). A breakdown in the regulation of this cycle leads to uncontrolled growth and contribute to tumor formation. Defects in many of the molecules that regulate the cell cycle also lead to tumor progression. Key among these are p53, the CKIs (p15 (INK4B), p16 (INK4A), p18 (INK4C), p19 (INK4D), p21, p27 (KIP1)), and Rb (Retinoblastoma Susceptibility Protein), all of which act to keep the cell cycle from progressing until all repairs to damaged DNA have been completed.In mammalian cells, different Cyclin-CDK complexes are involved in regulating different cell cycle transitions: Cyclin-D -CDK4/6 for G1 progression, Cyclin-E -CDK2 for the G1-S transition, Cyclin-A-CDK2 for S-phase progression, and Cyclin-A/B-CDC2 for entry into M-phase. Apart from thesewell-known roles in the cell cycle, several Cyclins and CDKs are involved in processes not directly related to the cell cycle. Cyclin-D binds and activates the estrogen receptor. (Ref.6). The Cyclin-H -CDK7 complex is a component of both the CDK-activating kinase and the basal transcription factor TFIIH and can phosphorylate CDKs. Other Cyclins and CDKs (Cyclin-C-CDK8, Cyclin-T-CDK9, and Cyclin-K) are also associated with RNA Polymerase-II and phosphorylate the carboxyl-terminal repeat domain.Cyclin-G, a target of p53, recruits PP2A (Protein Phosphatase 2A) to dephosphorylate MDM2 (Mouse Double Minute 2) (Ref.3).Cyclins associate with CDKs to regulate their activity and the progression of the cell cycle through specific checkpoints. Disruption of Cyclin action leads to either cell cycle arrest, or to uncontrolled cell cycle proliferation. Mitogenic signals that are received by cell surface receptors communicate to the nuclear cell cycle machinery to induce cell division through growth factor receptors that target Ras, which signals to a number of cytoplasmic signaling cascades such as PI3K (Phosphatidylinositiol–3 Kinase), Raf and Rho. These proteins connect to the nuclear cell cycle machinery to mediate exit from Go into G1 and S-phase of the cell cycle. Activation of Ras leads to transcriptional induction of Cyclin-D1 in early G1 through a Ras-responsive element in the Cyclin-D1 gene promoter. Cyclin-D associates with CDK4 and CDK6 to form active Cyclin-D/CDK4 (or -6) complexes. This complex is responsible for the first phosphorylation of tumor suppressor Rb in G1 (Ref.1). Subsequently, Cyclin-E is synthesized. When Cyclin-E is abundant it interacts with the cell cycle checkpoint kinase CDK2 and allow progression of the cell cycle from G1 to S-phase. One of the key targets of activated CDK2 complexed with Cyclin-E is Rb. When dephosphorylated in G1, Rb complexes with and blocks transcriptional activation by E2F transcription factors. But when CDK2/Cyclin-E phosphorylates Rb, it dissociates from E2F, allowing E2F to activate the transcription of genes required for S-phase. E2F activity consists of a heterodimeric complex of an E2F polypeptide and a DP1 protein (Ref.5). One of the genes activated by E2F is Cyclin-E itself, leading to a positive feedback cycle as Cyclin-E accumulates. In S-phase, Cyclin-A is made, whichin complex with DK2 adds further phosphates to Rb. Cyclin-B is made in G2 and M-phases of the cellcycle (Ref.4). It combines with CDK1 (also called CDC2 or CDC28) to form the major mitotic kinase MPF (M-phase Promoting Factor). MPF causes entry of cells into mitosis and, after a lag, activates the system that degrades its Cyclin subunit. MPF inactivation, caused by the degradation of Cyclin-B, is required forexit from mitosis (Ref.2). 14-3-3s bind to the phosphorylated CDC2–Cyclin-B kinase and exports it fromthe nucleus. During G2-phase, CDC2 is maintained in an inactive state by the kinases Wee1 and Myt1 (Myelin Transcription Factor 1). As cells approach M-phase, the phosphatase CDC25 is activated by PLK (Polo-Like Kinase). CDC25 then activates CDC2, establishing a feedback amplification loop thatefficiently drives the cell into mitosis.All Cyclins are degraded by ubiquitin-mediated processes, and the mode by which these systems are connected to the cell-cycle regulatory phosphorylation network, are different for mitotic and G1 Cyclins (Ref.2). The decision by the cell to either remain in G1 or progress into S-phase is the result in part of the balance between Cyclin-E production and proteolytic degradation in the proteosome. Cyclin-E is targetedfor destruction by the proteosome through ubiquitination when associated with a complex of proteinscalled the SCF or F box complex. During G1-phase, the Rb-HDACs (Histone Deacetylases) repressor complex binds to the E2F-DP1 transcription factors, inhibiting the downstream transcription. Manydifferent stimuli exert checkpoint control including TGF-Beta, DNA damage, contact inhibition, replicative senescence and growth factor withdrawal. The first four act by inducing members of the INK4A family orKIP/CIP families of cell cycle kinase inhibitors. TGF-Beta additionally inhibits the transcription of CDC25A,a phosphatase that activates the cell cycle kinases. DNA damage activates the DNA-PK/ATM/ATR kinases, initiating cascades that inactivate CDC2–Cyclin-B.Both synthesis and destruction of Cyclins are important for cell cycle progression. The destruction of Cyclin-B by Anaphase-Promoting Complex/cyclosome is essential for metaphase-anaphase transition, and expression of indestructible Cyclin-B traps cells in mitosis (Ref.3). Cyclins-E and A have been implicated in the DNA replication initiation process in mammalian cells. In embryonic systems, Cyclin-E regulates replication in the absence of Cyclin-A. For centrosome duplication, in somatic cells Cyclin-A is required to induce DNA replication and it has also been implicated in activation of DNA synthesis, because of its appearance time relative to the onset time of DNA synthesis and its localization to sites of nuclear DNA replication. Cyclin-E regulates the transcription of genes that encode the replication machinery but has also been implicated in the initiation process in mammalian cells (Ref.1). Similarly, expression of indestructible Cyclin-A arrests cells in late mitosis. Overexpression of Cyclin-F also causes an accumulation of the G2/M (Ref.3).。
细胞周期蛋白依赖性蛋白激酶5在中枢神经系统发育和神经退行性疾病中的作..
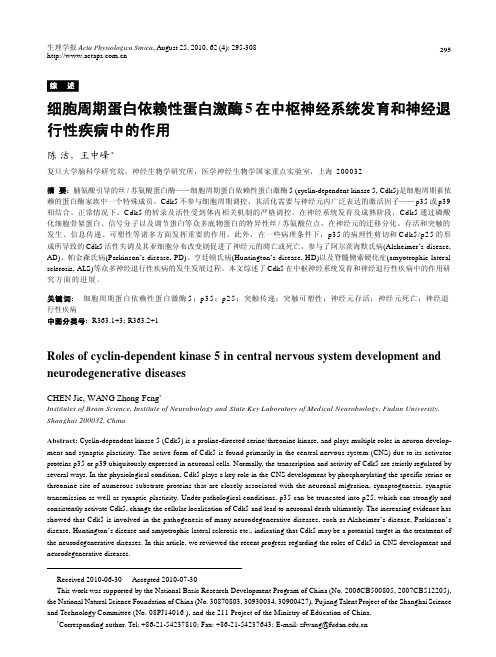
细胞周期蛋白依赖性蛋白激酶5在中枢神经系统发育和神经退行性疾病中的作用陈洁,王中峰*复旦大学脑科学研究院,神经生物学研究所,医学神经生物学国家重点实验室,上海 200032摘要: 脯氨酸引导的丝/苏氨酸蛋白酶——细胞周期蛋白依赖性蛋白激酶5 (cyclin-dependent kinase 5, Cdk5)是细胞周期素依赖的蛋白酶家族中一个特殊成员。
Cdk5不参与细胞周期调控,其活化需要与神经元内广泛表达的激活因子——p35或p39相结合。
正常情况下,Cdk5的转录及活性受到体内相关机制的严格调控。
在神经系统发育及成熟阶段,Cdk5通过磷酸化细胞骨架蛋白、信号分子以及调节蛋白等众多底物蛋白的特异性丝/苏氨酸位点,在神经元的迁移分化、存活和突触的发生、信息传递、可塑性等诸多方面发挥重要的作用。
此外,在一些病理条件下,p35的病理性剪切和Cdk5/p25的形成所导致的Cdk5活性失调及其亚细胞分布改变则促进了神经元的凋亡或死亡,参与了阿尔茨海默氏病(Alzheimer’s disease, AD)、帕金森氏病(Parkinson’s disease, PD)、亨廷顿氏病(Huntington’s disease, HD)以及脊髓侧索硬化症(amyotrophic lateral sclerosis, ALS)等众多神经退行性疾病的发生发展过程。
本文综述了Cdk5在中枢神经系统发育和神经退行性疾病中的作用研究方面的进展。
关键词:细胞周期蛋白依赖性蛋白激酶5;p35;p25;突触传递;突触可塑性;神经元存活;神经元死亡;神经退行性疾病中图分类号: R363.1+3; R363.2+1Roles of cyclin-dependent kinase 5 in central nervous system development and neurodegenerative diseasesCHEN Jie, WANG Zhong-Feng*Institutes of Brain Science, Institute of Neurobiology and State Key Laboratory of Medical Neurobiology, Fudan University, Shanghai 200032, ChinaAbstract: Cyclin-dependent kinase 5 (Cdk5) is a proline-directed serine/threonine kinase, and plays multiple roles in neuron develop-ment and synaptic plasticity. The active form of Cdk5 is found primarily in the central nervous system (CNS) due to its activator proteins p35 or p39 ubiquitously expressed in neuronal cells. Normally, the transcription and activity of Cdk5 are strictly regulated by several ways. In the physiological condition, Cdk5 plays a key role in the CNS development by phosphorylating the specific serine or threonine site of numerous substrate proteins that are closely associated with the neuronal migration, synaptogenesis, synaptic transmission as well as synaptic plasticity. Under pathological conditions, p35 can be truncated into p25, which can strongly and consistently activate Cdk5, change the cellular localization of Cdk5 and lead to neuronal death ultimately. The increasing evidence has showed that Cdk5 is involved in the pathogenesis of many neurodegenerative diseases, such as Alzheimer’s disease, Parkinson’s disease, Huntington’s disease and amyotrophic lateral sclerosis etc., indicating that Cdk5 may be a potential target in the treatment of the neurodegenerative diseases. In this article, we reviewed the recent progress regarding the roles of Cdk5 in CNS development and neurodegenerative diseases.Received 2010-06-30 Accepted 2010-07-30This work was supported by the National Basic Research Development Program of China (No. 2006CB500805, 2007CB512205), the National Natural Science Foundation of China (No. 30870803, 30930034, 30900427), Pujiang Talent Project of the Shanghai Science and Technology Committee (No. 08PJ14016 ), and the 211 Project of the Ministry of Education of China.*Correspondingauthor.Tel:+86-21-54237810;Fax:+86-21-54237643;E-mail:****************.cnKey words: cyclin-dependent kinase 5; p35; p25; synaptic transmission; synaptic plasticity; neuronal survival; neuronal death; neurodegenerative diseases1 引言细胞周期蛋白依赖性蛋白激酶5 (cyclin-depen-dent kinase 5, Cdk5)从1992年开始被多个实验室先后发现,并被予以不同的命名。
氧化应激相关kegg通路
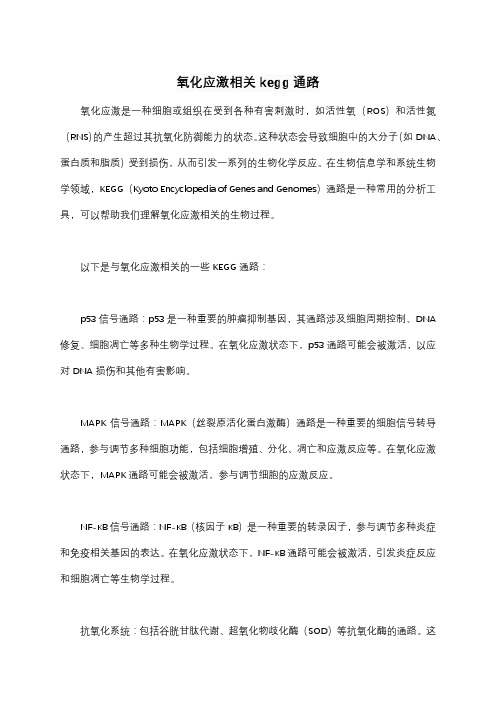
氧化应激相关kegg通路
氧化应激是一种细胞或组织在受到各种有害刺激时,如活性氧(ROS)和活性氮(RNS)的产生超过其抗氧化防御能力的状态。
这种状态会导致细胞中的大分子(如DNA、蛋白质和脂质)受到损伤,从而引发一系列的生物化学反应。
在生物信息学和系统生物学领域,KEGG(Kyoto Encyclopedia of Genes and Genomes)通路是一种常用的分析工具,可以帮助我们理解氧化应激相关的生物过程。
以下是与氧化应激相关的一些KEGG通路:
p53信号通路:p53是一种重要的肿瘤抑制基因,其通路涉及细胞周期控制、DNA 修复、细胞凋亡等多种生物学过程。
在氧化应激状态下,p53通路可能会被激活,以应对DNA损伤和其他有害影响。
MAPK信号通路:MAPK(丝裂原活化蛋白激酶)通路是一种重要的细胞信号转导通路,参与调节多种细胞功能,包括细胞增殖、分化、凋亡和应激反应等。
在氧化应激状态下,MAPK通路可能会被激活,参与调节细胞的应激反应。
NF-κB信号通路:NF-κB(核因子κB)是一种重要的转录因子,参与调节多种炎症和免疫相关基因的表达。
在氧化应激状态下,NF-κB通路可能会被激活,引发炎症反应和细胞凋亡等生物学过程。
抗氧化系统:包括谷胱甘肽代谢、超氧化物歧化酶(SOD)等抗氧化酶的通路。
这
些通路通过清除ROS和RNS等有害物质,维护细胞的氧化还原平衡。
需要注意的是,这些通路并不是孤立的,它们之间可能存在复杂的交互和调控关系。
因此,在研究氧化应激相关的生物学过程时,需要综合考虑这些通路之间的相互作用和影响。
中间纤维与细胞信号通路的研究进展
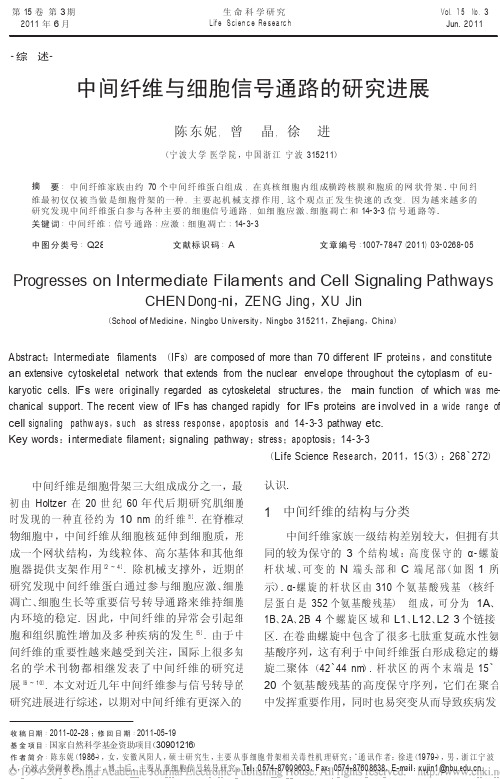
第15 卷第3 期2011 年6 月生命科学研究Life Science Re sea rchVol.15 No.3Jun. 2011·综述·中间纤维与细胞信号通路的研究进展陈东妮,曾晶,徐进(宁波大学医学院,中国浙江宁波315211)摘要:中间纤维家族由约70 个中间纤维蛋白组成,在真核细胞内组成横跨核膜和胞质的网状骨架.中间纤维最初仅仅被当做是细胞骨架的一种,主要起机械支撑作用.这个观点正发生快速的改变,因为越来越多的研究发现中间纤维蛋白参与各种主要的细胞信号通路,如细胞应激、细胞凋亡和14-3-3信号通路等.关键词:中间纤维;信号通路;应激;细胞凋亡;14-3-3中图分类号:Q28文献标识码:A文章编号:1007-7847(2011)03-0268-05 Progresses on Intermediat e Filament s and Cell Signaling PathwaysCHEN Don g-n i,ZENG Jing,XU Jin(School of Medicine,Ningbo University,Ningbo 315211,Zhejiang,Chin a)Abstract:Intermediate filaments (IF s)ar e composed of more than 70 different IF pr o te i ns,and co nstitute an extensive cytoskeleta l network that extends from the nuclear envelope throughout the cytoplasm of e u-karyotic cells. IFs were o r i g i nally regarded as cytoskeletal structures,the main function of which was m e- chanical support. The recent view of IFs has changed rapidly for IFs proteins are i nvolved in a wide rang e o f cell signaling pathw ays,s uch as stress resp onse,apo ptosis and 14-3-3pathway etc.Key w ord s:i ntermedi ate filament;signaling pathway;str ess;ap o ptosi s;14-3-3(Li f e Science Rese ar ch,2011,15(3):268~272)中间纤维是细胞骨架三大组成成分之一,最初由Holtzer 在20 世纪60 年代后期研究肌细胞时发现的一种直径约为10 nm 的纤维[1].在脊椎动物细胞中,中间纤维从细胞核延伸到细胞质,形成一个网状结构,为线粒体、高尔基体和其他细胞器提供支架作用[2 ~ 4].除机械支撑外,近期的研究发现中间纤维蛋白通过参与细胞应激、细胞凋亡、细胞生长等重要信号转导通路来维持细胞内环境的稳定. 因此,中间纤维的异常会引起细胞和组织脆性增加及多种疾病的发生[5].由于中间纤维的重要性越来越受到关注,国际上很多知名的学术刊物都相继发表了中间纤维的研究进展[6 ~ 10].本文对近几年中间纤维参与信号转导的研究进展进行综述,以期对中间纤维有更深入的认识.中间纤维的结构与分类中间纤维家族一级结构差别较大,但拥有共同的较为保守的3 个结构域:高度保守的α-螺旋杆状域、可变的N 端头部和C 端尾部(如图1 所示). α-螺旋的杆状区由310 个氨基酸残基(核纤1层蛋白是352 个氨基酸残基)组成,可分为1A、1B、2A、2B 4 个螺旋区域和L1、L12、L2 3 个链接区. 在卷曲螺旋中包含了很多七肽重复疏水性氨基酸序列,这有利于中间纤维蛋白形成稳定的螺旋二聚体(42~44nm). 杆状区的两个末端是15~20 个氨基酸残基的高度保守序列,它们在聚合中发挥重要作用,同时也易突变从而导致疾病发收稿日期:2011-02-28;修回日期:2011-05-19基金项目:国家自然科学基金资助项目(30901216)作者简介:陈东妮(1986-),女,安徽凤阳人,硕士研究生,主要从事细胞骨架相关毒性机理研究;* 通讯作者:徐进(1979-),男,浙江宁波il生. 中间纤维蛋白的头尾是非螺旋且高度可变是异聚体结构中的重要组分,同时也是中间纤维的的N 端和C 端,头尾的高度灵活可变使得中间纤维表现出了结构的多样性,同时也是这些蛋白质有特定生理功能的基础[8].N 端和C 端区域蛋白与细胞糖脂和接头蛋白等蛋白相互作用的主要部位[11].a -helica l rod domain1A2A2B1B图1 中间纤维结构示意图图中1A 左端和2B 右端的黑影部分表示杆状区具有高度保守序列两个末端.Fig.1 Schematic diagram of interm ediat e filamentThe regions indicated by shading are the highly conserved boundaries of rod domain.表1 中间纤维蛋白分类Table 1 The classification of interm e diat e filam entSubtype Protein Tissue distributionK8-K28 epithelia;K31-K40 hair and nailK1-K8,K71-K74 epithelia;K81-K86 hairMesenchyma l cellsMuscle cellsDiverse neurona l cellsStellate liver cellsNeuronsNeuroepithelia l stem cells;muscle cellsMuscle cellsMuscle cellsNeuronsAll cell typesAll cell typeslenslensTypeⅠTypeⅡTypeⅢAcidic keratinsBasic keratinsVimentinDesminPeripherinGFAPN F-L,-M,-HNestinSyneminSyncoilinα-internexinLamin A/CLamin B1/2FilensinPhakininTypeⅣTypeⅤTypeⅥ中间纤维蛋白是由约70 个基因编码的,其中3 个基因编码细胞核的核纤层蛋白,其余编码胞质蛋白[12].不同来源的细胞有着不同类型的中间纤维蛋白,如上皮细胞主要含角蛋白(ker-at i n);肌细胞含结蛋白(d esm i n);间充质细胞含波形蛋白(viment i n);神经元含神经微丝(neur o-f il am ent)[13].此外,有些细胞可能在发育的不同阶段表达不同的中间纤维蛋白,如肌细胞早期发育阶段可检测到viment i n高表达,而终末期分化的肌细胞中则没有[14].正因如此,无法根据细胞特异性来对中间纤维蛋白进行分类,只能按照α-螺旋杆状域氨基酸序列的不同,将其分为6 种类型(表1). Ⅰ型是酸性角蛋白,Ⅱ型是中性角蛋白,二聚体的角蛋白中间纤维. Ⅲ型中间纤维蛋白包括viment i n和desm i n,主要形成同二聚体的中间纤维[15].Ⅳ型中间纤维蛋白包括神经微丝、nest i n、synem i n等. Ⅰ~Ⅳ型蛋白位于细胞质中;Ⅴ型核纤层蛋白位于细胞核中,是核膜和核质的重要组成部分[4].Ⅵ型蛋白则位于晶状体[16].中间纤维家族一般是一个基因编码一个蛋白质,但也存在一些mRNA 经剪切加工后翻译多种蛋白质的情况,如l am i n A/C、GFA P、per i pher i n和synem i n等[3].中间纤维与应激中间纤维蛋白具有应激蛋白的功能,当应激反应发生时,中间纤维蛋白的表达显著上调,转2270生命科学研究2011 年而导致中间纤维溶解性的变化(解聚或聚合)[9]. K8/18 通过磷酸化改变参与多种细胞应激反应,尤其是生长因子和环境应激[17].小鼠实验证实,K18 在毒物应激时通过磷酸化对细胞起保护作用. 而K18 Ser52Ala 突变时,该氨基酸残基磷酸化水平显著下降,无法应对毒物的应激反应,最终引起肝脏损伤[6].这种角蛋白磷酸化保护作用的另一个范例是K8 Gly61Cys(G61C)突变. 转基因小鼠中该突变的过度表达比野生型小鼠更容易导致细胞凋亡和肝脏损伤[18]. K8 G61C 突变能抑制S er73位置的磷酸化,而该位置正是p38、JNK 和p42 等应激活化蛋白激酶的结合位点[18].与K8 Ser73 磷酸化模序相似的氨基酸序列在其他的Ⅱ型角蛋白(K4、K5、K6 等)中也存在,并且在应激反应中也能磷酸化[19].因此,在应激反应中,角蛋白如同“海绵”一样吸收蛋白激酶的活性而使组织免受损伤[6]. K8/18 能与应激相关的伴侣分子(如H SP70、GR P78、MR J)等结合,进而调控中间纤维的组成和聚集,以此保护肝细胞免受代谢性应激损伤[20].此外,vimentin、d es min、peripherin、GFA P、ph- akinin、filensin 等中间纤维蛋白能与热休克蛋白家族的H SP27、αβ-cry s ta llin 等作用以应对各种应激[20].各种中间纤维与HSP 的结合具有选择性,如K8、K18 分别与MRJ 和HSP70 结合[21,22],GFAP 和vimentin 可与HSP27 或αβ-cry s ta llin 结合[23].在热应激的条件下这种结合会显著增加[22,24],如晶状体暴露于热应激环境中可导致αβ-cry s-tallin 和中间纤维的结合增加[25].显微注射MR J抗体可导致K8/18 纤维解聚,提示中间纤维和H SP 的相互作用有利于中间纤维的正常组装[21],但这种结合参与应激的作用机制还不清楚. 同时在非应激条件下这种相互作用也处于一个较高的水平,因而提示中间纤维和HSP 的相互结合可能还存在其他的功能,尚待进一步研究[9].在氧化应激时,角蛋白表现出抗氧化特性[26]. 用基因芯片法筛选K18 Ar g89Cy s(R89C)突变小鼠的转录水平,发生改变的绝大多数是氧化代谢基因和氧化还原信号通路相关的基因,如细胞色素P450、谷胱甘肽硫转移酶、S-腺苷高半胱氨酸水解酶、半胱氨酸亚磺酸脱羧酶等. 与野生型小鼠相比,K18 R89C 转基因小鼠肝脏的细胞色素P450 的水平要升高3.9 倍,脂质过氧化物(丙二转导通路有关[27].内皮细胞在缺氧诱导的应激反应时,vimentin 的磷酸化水平显著上升,并与HSP27 相互作用,使vimentin 蛋白可溶性降低,形成更广泛的网络结构,以此增强内皮细胞间的粘附能力及屏障功能,保护细胞免受应激损伤[28].中间纤维与细胞凋亡中间纤维对细胞的保护除了它们的机械作用和消除应激外,也来自于中间纤维蛋白参与细胞凋亡信号通路. 如nestin 能与Cdk5 形成支架,进而维持胞浆内Cdk5/p53 复合体稳定,增加细胞内p53 稳定性,减少p25 的产生,抑制细胞凋亡的发生. 同时P25 的产生可介导cdk5 在细胞核中的定位,这也是cdk5 的细胞毒性的基础. 当氧3化损伤诱导细胞凋亡时,nestin 被下调表达,Cdk5 的活性随之增加,促进细胞凋亡[29].与之相似,序列比对分析发现,K8 和K18 具有与J NK 底物相类似的JNK 停泊位点,因此k8 可直接或通过与K18 聚合间接的与JNK 相互作用,当细胞凋亡的死亡受体通路激活时,K8 在ser-73 位置上被JNK 磷酸化[30],并减少c-jun 等转录生长因子被泛素化[31].中间纤维还可以通过运输信号分子来保护细胞. 例如,当神经细胞损伤时,ERK1 和ERK2 被磷酸化,伴随着vimentin 表达增加,可溶性的vimentin 亚基与磷酸化的ERK1/ 2 相结合,vimentin 通过与轴浆中输入蛋白β直接结合将vimentin-ERK1/2 复合体从轴突损伤位置运送到神经细胞体内,以此避免细胞死亡的发生[32].K8/18 通过Fas/TNF 受体途径来调控细胞凋亡. 如果K8/18 缺失,上皮细胞用TNF/放线菌酮诱导时,细胞凋亡增强100 倍[33].K18 可以阻止TRADD(TNF Receptor-Associated Death D o m a i n)与TNF 受体结合,抑制死亡诱导信号复合物(death-inducing signaling c o mplex,D I S C)的形成,从而调控TNF 诱导的细胞凋亡[33 ~ 35].K8 可以降低Fas 受体与细胞表面的结合,以此保护细胞免受Fas 介导的细胞凋亡[35].此外,肝细胞和乳腺细胞中,K8 缺失会下调c-FL I P(F as-asso ciated d ea th domain-like I L-1-c o nverting enzyme-like inhibitory protein)的表达[36].c-FL I P可以阻止caspase-8 聚集到Fas 受体,所以K8 的正常表达可以使细胞降低对Fas 介导细胞凋亡的敏感性. K17 参与的控 .展望中间纤维蛋白家族成员众多,广泛分布于人体的各个组织脏器. 它们不仅仅起支架作用,大量维持细胞稳态和正常功能的酶、分子马达、膜受5 大部分Ⅰ型角蛋白的杆状域存在 c as pa se -6 (或 c as pa se -3)的剪切序列,而Ⅱ型角蛋白则不会 被 caspase 降解[38]. K18 在 C 端还存在另一个 c as - pase-9 剪切的位点. K18 称为 caspase 底物的机理 可 能 与 DEDD (death effector domain c o ntaining DNA binding pr o te i n ) 有关. DEDD 虽然主要存在 于胞质中,但具有核定位信号和 DNA 结合功能. 在 HeLa 、MCF-7 和 A549 等 细 胞 系 中 ,胞 质 DEDD 以双泛素化形式存在,并与 K18、pr o c as - pase-3 和 procaspase-9 联系在一起. 当细胞凋亡 发生时,DE DD 的空间构象发生改变,与 K18 和 procaspase-9 形成丝状结构. DEDD 介导的 pr o - caspase-9 在角蛋白支架上的聚集,会导致其浓度 增加,并发生寡聚化和自身活化. 激活的 c as - pase-9 作用于 K18 及下游 caspase 成员,最终导 致角蛋白网络的崩解[39, 40]. 其他类型的中间纤维蛋 白,如 vimentin 、desmin 和 lamin 也是 caspase 的 底物,剪切后的片段会促进凋亡的级联反应[20].体、接头蛋白等都能直接与中间纤维发生作用,并影响胞内的主要信号转导通路[20]. 越来越多的 研究揭示中间纤维蛋白如何参与磷酸化及相关的信 号 通 路 ,除 本 文 中 已 经 介 绍 的 ERK 、cdk5、cdc25 等,这类蛋白还能与许多激酶相互作用,如 P KC 、P KA 、J NK 、Ca MK Ⅱ、Akt 、Rh o 、R a f-1[44],也包括 PP2A 和 PP 1C 两个磷酸酶[45, 46]. 因此,有不少学者将中间纤维称为“信号平台”,并认为这个 平台的范围及作用机理将是细胞生物学研究的重 点之一[3, 6]. 鉴定与中间纤维蛋白结合的信号分子依 旧是此项研究的核心,因为这将有助于我们了解哪 些信号通路可能依赖这个“信号平台”产生作用.此外,已知的中间纤维功能几乎都来自于转基因小鼠模型,而其他的模式生物或许能提供更多的信息. 例如,线虫有许多中间纤维基因,部分基因还与胚胎发育后期的线虫生存直接相关而这些中间纤维基因在决定线虫生死命运中的作用机理却仍不清晰[47]. 斑马鱼基因组中有与哺乳动物相类似的中间纤维家族基因,非常适合用定点突变法来鉴定某个中间纤维基因的作用及表型筛选[48]. 此外,这两种模式生物还非常适合实时活体成像,能更好地研究中间纤维动态变化及作用机理[3]. 因此,在今后的研究中像线虫、斑马鱼等模式生物的应用将会有助于我们更好地了解中间纤维.参考文献(References ):中间纤维与 14-3-3 信号通路14-3-3 家族是一类高度保守且高丰度的胞质蛋白,通过与其他信号分子磷酸化依赖的相互作 用而调控胞内主要的信号转导通路[41]. 中间纤维 蛋白具有调控 14-3-3 的作用,细胞实验发现在有 丝分裂期,K18 ser33 选择性磷酸化能促进 K18 与 14-3-3ξ 的相互作用,并促进 K18 从胞浆向细 胞核转移,改变 K18 在胞内的分布[42]. K8 缺失时, 无法形成 K8/18 异二聚体中间纤维,从而改变 14-3-3ξ 的分布,使之聚集于细胞核内,最终导致 细胞周期在 S/G 2 期阻滞[43]. K18 与 14-3-3 的相互 作用,可以抑制 14-3-3 与磷酸化 cdc25 的结合, 而 cdc25 的主要功能是清除细胞周期依赖蛋白激 酶上的抑制性磷酸残基,若 cdc25 与 14-3-3 结合 则抑制 cdc25 与特定的细胞周期依赖蛋白激酶相 互作用,最终导致细胞周期阻滞[43]。
杨梅黄酮药理作用机制的研究进展
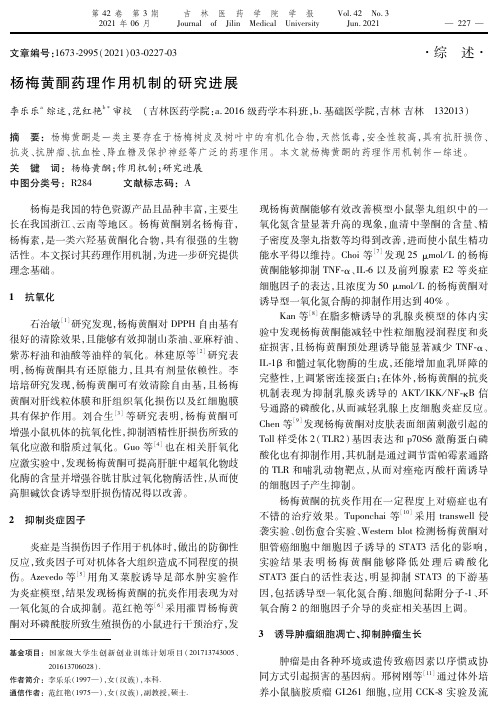
文章编号:1673 2995(2021)03 0227 03·综 述·杨梅黄酮药理作用机制的研究进展李乐乐a综述,范红艳b 审校 (吉林医药学院:a.2016级药学本科班,b.基础医学院,吉林吉林 132013)摘 要:杨梅黄酮是一类主要存在于杨梅树皮及树叶中的有机化合物,天然低毒,安全性较高,具有抗肝损伤、抗炎、抗肿瘤、抗血栓、降血糖及保护神经等广泛的药理作用。
本文就杨梅黄酮的药理作用机制作一综述。
关 键 词:杨梅黄酮;作用机制;研究进展中图分类号:R284 文献标志码:A 杨梅是我国的特色资源产品且品种丰富,主要生长在我国浙江、云南等地区。
杨梅黄酮别名杨梅苷,杨梅素,是一类六羟基黄酮化合物,具有很强的生物活性。
本文探讨其药理作用机制,为进一步研究提供理念基础。
1 抗氧化石治敏[1]研究发现,杨梅黄酮对DPPH自由基有很好的清除效果,且能够有效抑制山茶油、亚麻籽油、紫苏籽油和油酸等油样的氧化。
林建原等[2]研究表明,杨梅黄酮具有还原能力,且具有剂量依赖性。
李培培研究发现,杨梅黄酮可有效清除自由基,且杨梅黄酮对肝线粒体膜和肝组织氧化损伤以及红细胞膜具有保护作用。
刘合生[3]等研究表明,杨梅黄酮可增强小鼠机体的抗氧化性,抑制酒精性肝损伤所致的氧化应激和脂质过氧化。
Guo等[4]也在相关肝氧化应激实验中,发现杨梅黄酮可提高肝脏中超氧化物歧化酶的含量并增强谷胱甘肽过氧化物酶活性,从而使高胆碱饮食诱导型肝损伤情况得以改善。
2 抑制炎症因子炎症是当损伤因子作用于机体时,做出的防御性反应,致炎因子可对机体各大组织造成不同程度的损伤。
Azevedo等[5]用角叉菜胶诱导足部水肿实验作为炎症模型,结果发现杨梅黄酮的抗炎作用表现为对一氧化氮的合成抑制。
范红艳等[6]采用灌胃杨梅黄酮对环磷酰胺所致生殖损伤的小鼠进行干预治疗,发基金项目:国家级大学生创新创业训练计划项目(201713743005、201613706028).作者简介:李乐乐(1997—),女(汉族),本科.通信作者:范红艳(1975—),女(汉族),副教授,硕士.现杨梅黄酮能够有效改善模型小鼠睾丸组织中的一氧化氮含量显著升高的现象,血清中睾酮的含量、精子密度及睾丸指数等均得到改善,进而使小鼠生精功能水平得以维持。
P25CDK5-p53通路在BaP致神经细胞凋亡中的作用研究的开题报告
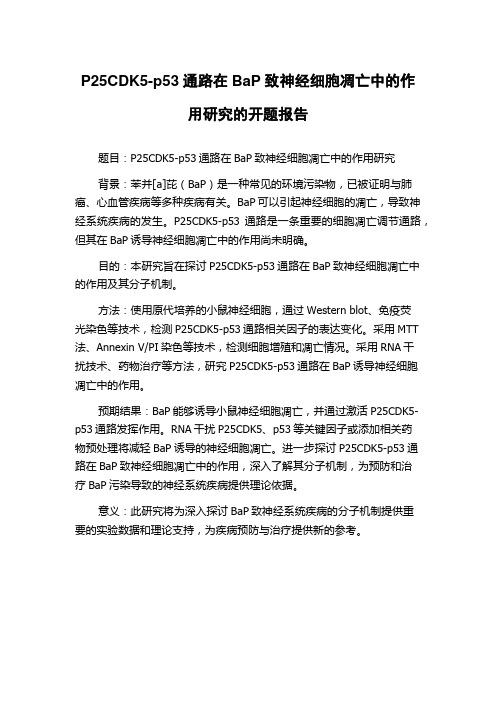
P25CDK5-p53通路在BaP致神经细胞凋亡中的作
用研究的开题报告
题目:P25CDK5-p53通路在BaP致神经细胞凋亡中的作用研究
背景:苯并[a]芘(BaP)是一种常见的环境污染物,已被证明与肺癌、心血管疾病等多种疾病有关。
BaP可以引起神经细胞的凋亡,导致神经系统疾病的发生。
P25CDK5-p53通路是一条重要的细胞凋亡调节通路,但其在BaP诱导神经细胞凋亡中的作用尚未明确。
目的:本研究旨在探讨P25CDK5-p53通路在BaP致神经细胞凋亡中的作用及其分子机制。
方法:使用原代培养的小鼠神经细胞,通过Western blot、免疫荧
光染色等技术,检测P25CDK5-p53通路相关因子的表达变化。
采用MTT 法、Annexin V/PI染色等技术,检测细胞增殖和凋亡情况。
采用RNA干
扰技术、药物治疗等方法,研究P25CDK5-p53通路在BaP诱导神经细胞凋亡中的作用。
预期结果:BaP能够诱导小鼠神经细胞凋亡,并通过激活P25CDK5-p53通路发挥作用。
RNA干扰P25CDK5、p53等关键因子或添加相关药
物预处理将减轻BaP诱导的神经细胞凋亡。
进一步探讨P25CDK5-p53通路在BaP致神经细胞凋亡中的作用,深入了解其分子机制,为预防和治
疗BaP污染导致的神经系统疾病提供理论依据。
意义:此研究将为深入探讨BaP致神经系统疾病的分子机制提供重
要的实验数据和理论支持,为疾病预防与治疗提供新的参考。
免疫和炎症相关信号通路

免疫与炎症相关信号通路一、Jak/Stat Signaling:IL-6 Receptor FamilyJak和Stat是许多调节细胞生长、分化、存活和病原体抵抗信号通路中的关键部分。
就有这样一个通路涉及到IL-6(gp130)受体家族,它帮助调节B细胞的分化,浆细胞生成和急性期反应。
细胞因子结合引起受体的二聚化同时激活受体结合的Jak蛋白,活化的Jak蛋白对受体和自身进行磷酸化。
这些磷酸化的位点成为带有SH2结构的Stat蛋白和接头蛋白的结合位置,接头蛋白将受体和MAP激酶,PI3激酶/Akt还有其他的通路联系在一起。
受体结合的Stat蛋白被Jak磷酸化后形成二聚体,转移进入细胞核调节目的基因的表达。
细胞因子信号传导抑制分子(SOCS)家族的成员通过同源或异源的反馈减弱受体传递的信号。
Jak或Stat参与其他受体蛋白的信号传导,在下面Jak/Stat使用表格中有这方面的列举。
研究人员已经发现Stat3和Stat5在一些实体肿瘤中被酪氨酸激酶而不是Jaks组成性激活。
JAK/STAT途径介导细胞因子的效应,如促红细胞生成素,血小板生成素,G-CSF,这些细胞因子分别是用于治疗贫血,血小板减少症和中性粒细胞减少症的蛋白质类药物。
该途径也通过干扰素介导信号通路,干扰素可以用来作为抗病毒和抗增殖剂。
研究人员发现,失调的细胞因子信号有助于癌症的发生。
异常的IL-6的信号或导致自身免疫性疾病,炎症,癌症,如前列腺癌和多发性骨髓瘤的发生。
Jak抑制剂目前正在多发性骨髓瘤模型中进行测试。
Stat3具有潜在促癌性(原癌基因),在许多癌症中持续的表达。
在一些癌细胞中,细胞因子信号传导和表皮生长因子受体(EGFR)家族成员之间存在交流。
Jak激活突变是恶性血液病中主要的分子机制。
研究人员已经在Jak2假激酶域中发现一个特有的体细胞突变(V617F),这个突变常常发生于真性红细胞增多症,原发性血小板增多症和骨髓纤维化症患者。