jp101661t ABS文献
日本文献标引对照表(08年9月更新)
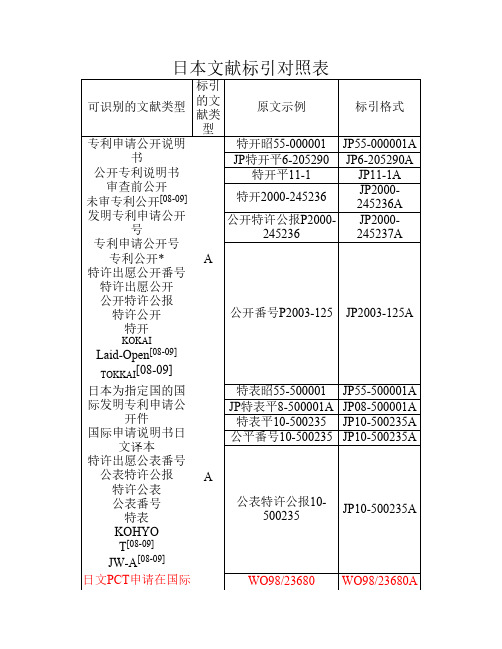
用新型登录公报”(Y2),采用连续编号方式,取消前缀年,起号自 2500001Y2开始。连续编号号段为JP2500001~JP2607898,标为 JP2500001Y~JP2607898Y。 自1号始顺序号排列。
专利申请公告号
特许番号25000001 JP25000001B
特许出愿公告番号
JP特公平7-26
JP7-26B
特许公告公报 特许公告 特许公报
特许登录[08-09] 特许 特公
特公平7-1
JP7-1B
B
特许番号第2500006 号
JP2500006B
特许第3123756号 JP3123756B
特许公报P3123756 JP3123756B
开件 国际申请说明书日
文译本 特许出愿公表番号
特表昭55-500001 JP55-500001A JP特表平8-500001A JP08-500001A
特表平10-500235 JP10-500235A 公平番号10-500235 JP10-500235A
公表特许公报 特许公表 公表番号 特表
A
公表特许公报10500235
为96年后出版,此阶段发明文献申请后包括公开和授权两阶段,B类文 献为授权阶段文献,形式以序号连续编号形式体现(为不包含年号的表 示法),号码起号为JP2500001B。 附:1996年3月29日,依据旧专利法出版的日本公告说明书全部出版完 毕,最后一件专利的公告号为JP平8-034772B2。此后9周内没有再出版 标识代码为B的文献,直到1996年5月29日,根据新的专利法第一次出版 了授权专利说明书,其起始号为2500001,即JP2500001B。 C类文件(特许明细)为1950-1996年前的专利授权公告号,其号段为 JP000001~JP216017,标为JP000001C~JP216017C。 U类文件(实开、实表、注册实用新型)标号类型有三种:1、本国纪 年+本年度公表顺序号,实开、实表为该表示法,实公本国纪年后面跟 的数字为1-4,实表后面跟的数字为5、6;94年1月1日前申请的实用新 型专利沿用此类编号方式予以公开,此时实用新型文献经过实质审查, 文件申请后经过公开、公告、授权三阶段和公告,此U类文献对应此阶 段公开文献类型;2、94年1月1日后申请的实用新型公告不经实质审查 直接进行授权公告,此时使用“登录实用新案公报”,采用连续编号方 式,取消前缀纪年,起号自3000001U开始,号段为 JP3000001~JP3129095,标为JP3000001U~JP3129095U;3、1996年日本 专利法修改,取消公告阶段,在94年1月1日前申请,截止到1995年底已 公开但还未实审的实用新型案件,依据旧法采取实审制,公开文件编号 采用原“本国纪年+本年度公开顺序号”编号形式,但编号从JP8-000001 开始计号。
日本小学理科新教材中生物实验设计精度分析

力。
关 键 词: 日本; 教科书; 生物; 实验设计; 精度
中图分类号: G 624 文献标识码: A 文章编号: 1671-0916( 2011) 12-0125-03
1947 年,日本制定了第一套《学习指导要领》,理科篇的 第七章在对小学生学习态度进行考察时以观察为例: 提出观 察的考查尺度包括: “观察兴趣、发现问题、观察精度、观察正 确度、观察毅力、发现关系、观察记录七个维度”[1]。可见日 本小学理科在“观察和实验”上有重视精度的传统。2008 年 3 月新的小学指导要领出台后,经过三年过渡期,日本小新 理科教材于 2011 年 4 月全面实施。新日本小学理科中的实 验设计体现日本传 统 文 化 中 的“花 道”、“茶 道”、“剑 道 ”中 “道”的精髓,这种“科学之道”值得我们借鉴。以期改善我 国小学科学实验设计粗糙单一,只有比对实验的现状。
时打开塑料袋用 成长情况
雄花授粉再封上
葫芦瓜雌花未开放前 控制组
用塑料袋套着
不处理
观察葫芦 成长情况
( 表 5) 为控制组实验组后测设计,由于实验组接受授粉
处理,控制组不接受授粉处理,从而控制了生物成熟因素( 果
实生长的原因为植物自然生长) 的影响,另外这个实验采用
了塑料袋控制了外部因素的干扰,此外实验选用了两种实验
遮光处理
第二天早上 取叶子测 含不含淀粉
第二天早上 取叶子测 含不含淀粉
第二天阳光 照射植物
第二天继续 遮光处理
第二天午后 取叶片测 含不含淀粉
第二天午后 取叶片测 含不含淀粉
( 表 3) 为控制组实验组前后测设计,采用前测控制了历 史事件的影响,从而排除叶片原本就含有淀粉。控制组与实 验组对照消除了植物成长因素的影响,得出实验的结果差异 是由于不同的实验处理( 受不受阳光照射) 引起的。
高固含量聚丙烯酸酯微乳液的研究[文献综述]
![高固含量聚丙烯酸酯微乳液的研究[文献综述]](https://img.taocdn.com/s3/m/a76e04ad19e8b8f67d1cb92a.png)
文献综述高固含量聚丙烯酸酯微乳液的研究一、前言丙烯酸酯乳液由丙烯酸酯类单体与功能单体共聚而成。
其成膜具有良好的耐候性和保光性,广泛应用与涂料、纺织整理、纸加工处理、皮革整饰以及胶黏剂等领域。
通常的丙烯酸酯乳液粒径较大(一般在几百纳米左右),涂膜效果较差。
近年来,粒径在几十纳米的微乳液已成为国内外研究的热点。
为此,人们试图通过改进聚合工艺和寻找合成新的高效乳化剂来克服上述缺点。
自从1943 年Schulman 首次报道微乳液以来,提高它的固含量一直是人们追求的目标。
目前,降低体系中乳化剂用量和提高固含量仍然是微乳液聚合的研究热点。
二正文1.聚丙烯酸酯微乳液的特点纳米级粒子乳液(即微乳液)成膜性能较好,可在保证成膜性能的同时赋予其较高的硬度,形成的胶膜致密、光洁爽滑、光泽好以及透明度高,因而在追求原色的木器涂料市场中具有广阔的应用前景。
根据微乳液的性质,以微胶乳作为涂料印花粘合剂已经广泛应用,常规微乳液聚合体系存在比较突出的问题是乳化剂用量大、单体含量低;乳化剂含量太高则胶膜耐水性差,而固含量太低又使得乳胶膜的丰满度不能满足要求,强度下降,因而限制了微乳液在涂料和胶粘剂工业上的应用。
超微粒子聚合物乳液,其粒径一般在50 nm以下,为透明或半透明的胶体分散液。
由于雷利(Rayleigh)散射,其反射光呈青白色,透过光呈黄红色,粘度较高。
由于粒径很小,系几介于溶液和胶体之间的分散体系,故其皮膜致密性、光洁性近似于溶剂型胶。
在合成超微粒子乳液时添加一定量的水溶性醇类,既有利于胶粒的超微化,又可以在成膜过程中起成膜助剂的作用,使之在低于MFT的室温下也可成膜。
采用含亲水基团的自乳化型单体代替表面活性剂,则可完全避免由于后者的存在而引起的表面“不爽”感。
羧基的存在,又为交联成膜时提供一定量的交联点,有利于膜强度和耐水压性能的提高。
2.2 几种丙烯酸酯乳液制备研究近年来,国内外对丙烯酸及其酯类的研究相当活跃,通过改性,采用新的工艺,制备了多种性能优异丙烯酸酯乳液产品。
日本有关纤维、织物、人造皮革的专利文献题录

楮 布 的 制 法
特 开 昭 6 ~2 7 3 1 066
23 3
聚 酯 系 无 纺 布 特开平 1 4—20 7 275
21 8
防 爆 防 尘 服
特 开 昭 6 ~12 0 1 36 2
23 4
超 细 纤 维 无 纺 布 特 开 平 1 2 5 4 4~ 7 7 8
21 9
特开昭 5 9~14 73
231
能超 细化 的长 纤 维 无 纺 布 特开平 1 5—13 7 15 0
21 6
可变 色纱 和可 变花 纹 编 织 物
特 开 昭 6 ~7 05 1 0 5
23 2
起绒状无纺布的制法以及使用它的纤维制品
特开平 1 4—32 6 081
21 7
211
丝 绒状 绒 布 的 制法
特开昭 5 7—17 7 64
22 8
耐 热 性 无 纺布 特开平 1 4—60 3 16
21 2
天 鹅 绒 状 织 物
特开昭 5 9—7 9 7 6 4
22 9
可作上光剂 和纤维整理剂 使用 的保护膜 形
成 型 聚 硅 氧烷 乳 液 组 成 物 特 开 平 4~ 5 5 4 14
防弹 衣 用 的 耐 弹 织 布
特 开 平 1 5 9 —9 27
23 5
干式 无 纺 布 特 开平 1 2 2 7 4— 18 0
2 20
防 弹用 织 物
特 开平 1—16 4 55 1
23 6
阻 燃 性 无 纺布 特开平 1 4~1 16 64 1
221
丝 绒 织 物 的 机织 方法
223
窗 帘 布 的 织 造 方 法 特 开 平 1 2 2 4 ~ 787
聚丙烯腈-丁二烯-苯乙烯ABS的应变率敏感性及缺口敏感性实验研究
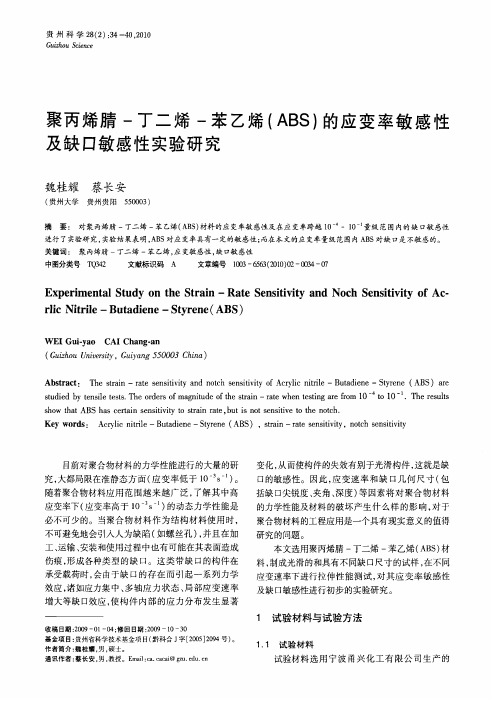
目前对 聚合物 材 料 的力学 性 能进行 的大量 的研 究 , 大都局 限在准 静态 方 面 ( 应 变 率低 于 10 一 一) 随着聚合 物材料 应 用范 围越 来 越 广 泛 , 了解其 中高 应 变率 下 ( 应 变率高 于 10 一S一) 的动 态 力学性 能是
变化 , 从 而使 构件 的失效 有别 于光滑 构 件 , 这就 是缺 口的敏感 性 因此 , 应 变 速 率 和 缺 口几 何 尺 寸 ( 包
必不可少的 当聚合物材料作 为结构材料使用时 ,
不可避 免地会 引入人 为 缺 陷 ( 如螺 丝 孔 ) , 并 且 在加 工 运输 安装 和使用 过 程 中也 有 可能 在其表 面造成 伤痕 ,形 成 各种类 型 的 缺 口 这 类 带 缺 口的构 件在
承受载 荷时 , 会 由于缺 口的 存 在 而 引起 一 系 列 力学 效应 ,诸 如应 力集 中 多 轴应 力状 态 局 部应 变 速率 增大 等缺 口效 应 , 使 构 件 内部 的应 力 分 布发 生 显著
t o s t ai n ra t e , b u t 15 n ot se n s i ti v e t r oΒιβλιοθήκη K ey wor d s :
Ae叮lie nitr le 一Bu tadiene 一Styrene ( ABS) , str in 一rate sensitivity , noteh sensitivity i a
试 验 材 料 与试 验 方 法
收稿 日期 :2(X珍一0 一0 ; 修 回 日期 :2 0 一10 一 1 4 0 30 基金项 目 : 贵州省科学 技术基金项 目( 黔科合 J 字 #20 5 20 4 号) 0 9 作者简 介 : 魏桂雄 , 男 , 硕士 通讯作者 : 蔡长安 , 男 , 教授 Email : ea. eaeai@ g % !e u ! cn d
聚丙烯腈纤维的文献综述
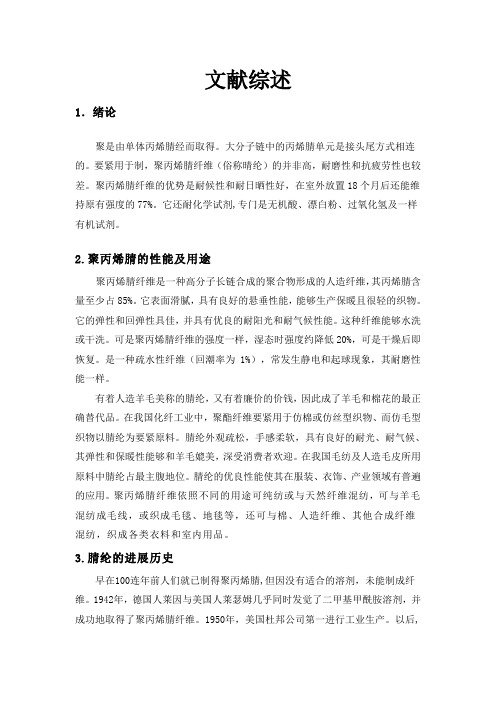
文献综述1.绪论聚是由单体丙烯腈经而取得。
大分子链中的丙烯腈单元是接头尾方式相连的。
要紧用于制,聚丙烯腈纤维(俗称晴纶)的并非高,耐磨性和抗疲劳性也较差。
聚丙烯腈纤维的优势是耐候性和耐日晒性好,在室外放置18个月后还能维持原有强度的77%。
它还耐化学试剂,专门是无机酸、漂白粉、过氧化氢及一样有机试剂。
2.聚丙烯腈的性能及用途聚丙烯腈纤维是一种高分子长链合成的聚合物形成的人造纤维,其丙烯腈含量至少占85%。
它表面滑腻,具有良好的悬垂性能,能够生产保暖且很轻的织物。
它的弹性和回弹性具佳,并具有优良的耐阳光和耐气候性能。
这种纤维能够水洗或干洗。
可是聚丙烯腈纤维的强度一样,湿态时强度约降低20%,可是干燥后即恢复。
是一种疏水性纤维(回潮率为1%),常发生静电和起球现象,其耐磨性能一样。
有着人造羊毛美称的腈纶,又有着廉价的价钱,因此成了羊毛和棉花的最正确替代品。
在我国化纤工业中,聚酯纤维要紧用于仿棉或仿丝型织物、而仿毛型织物以腈纶为要紧原料。
腈纶外观疏松,手感柔软,具有良好的耐光、耐气候、其弹性和保暖性能够和羊毛媲美,深受消费者欢迎。
在我国毛纺及人造毛皮所用原料中腈纶占最主腹地位。
腈纶的优良性能使其在服装、衣饰、产业领域有普遍的应用。
聚丙烯腈纤维依照不同的用途可纯纺或与天然纤维混纺,可与羊毛混纺成毛线,或织成毛毯、地毯等,还可与棉、人造纤维、其他合成纤维混纺,织成各类衣料和室内用品。
3.腈纶的进展历史早在100连年前人们就已制得聚丙烯腈,但因没有适合的溶剂,未能制成纤维。
1942年,德国人莱因与美国人莱瑟姆几乎同时发觉了二甲基甲酰胺溶剂,并成功地取得了聚丙烯腈纤维。
1950年,美国杜邦公司第一进行工业生产。
以后,又发觉了多种溶剂,形成了多种生产工艺。
1954年,联邦德国法本拜耳公司用丙烯酸甲酯与丙烯腈的共聚物制得纤维,改良了纤维性能,提高了有效性,增进了聚丙烯腈纤维的进展。
腈纶工业从2O世纪5O年代在美国、德国和日本实现了工业化生产,6O年代和7O年代世界腈纶工业快速进展,到8O年代由于服用纤维转向棉和天然纤维,而且原料价钱上涨,本钱增加,致使腈纶增加减缓。
- 1、下载文档前请自行甄别文档内容的完整性,平台不提供额外的编辑、内容补充、找答案等附加服务。
- 2、"仅部分预览"的文档,不可在线预览部分如存在完整性等问题,可反馈申请退款(可完整预览的文档不适用该条件!)。
- 3、如文档侵犯您的权益,请联系客服反馈,我们会尽快为您处理(人工客服工作时间:9:00-18:30)。
Dynamics of Phase Separation in Poly(acrylonitrile-butadiene-styrene)-Modified Epoxy/DDS System:Kinetics and Viscoelastic EffectsP.Jyotishkumar,†Ceren O¨zdilek,‡Paula Moldenaers,‡Christophe Sinturel,§Andreas Janke,|Ju¨rgen Pionteck,|and Sabu Thomas*,†,⊥School of Chemical Sciences,Mahatma Gandhi Uni V ersity,Priyadarshini Hills,Kottayam,Kerala686560,India;Department of Chemical Engineering,Catholic Uni V ersity of Leu V en,de Croylaan,46,B-3001,Leu V en,Belgium;Centre de Recherche sur la Matie`re Di V ise´e,UMR6619CNRS Uni V ersite´d’Orle´ans,1B Rue de la Fe´rollerie,FR45071,Orle´ans Cedex2,France;Leibniz Institute of PolymerResearch Dresden,Hohe Str6,DE01069,Dresden,Germany;and Centre for Nanoscience andNanotechnology,Mahatma Gandhi Uni V ersity,Priyadarshini Hills,Kottayam,Kerala686560,IndiaRecei V ed:February24,2010;Re V ised Manuscript Recei V ed:September19,2010The dynamics of phase separation andfinal morphologies of poly(acrylonitrile-butadiene-styrene)(ABS)-modified epoxy system based on diglycidyl ether of bisphenol A(DGEBA)cured with4,4′-diaminodiphe-nylsulfone(DDS)have been monitored in situ throughout the entire curing process by using optical microscopy(OM),differential scanning calorimetry(DSC),rheometry,and small-angle laser light scattering(SALLS).The evolution of phase separation andfinal morphologies with substructures were explored by OM.Thefinalmorphologies of the blend cured at150and165°C are of phase-inverted type and are quite different fromthefinal morphologies of the same blend cured at180°C,in which thefinal morphologies are cocontinuous.AFM observations of the fully cured sample confirmed the existence of three different phases,the epoxycontinuous phase,SAN(styrene/acrylonitrile)continuous phase,and PB droplets at the interface,with a strongtendency to stay at SAN continuous phase.Furthermore,the continuous epoxy phase contains SAN particlesand the continuous SAN phase contains epoxy particles.Cure kinetics and rheological results correspondwell with the viscoelastic phase separation revealed by OM.The SALLS results display clearly that thephase separation takes place according to nucleation and growth mechanism followed by spinodaldecomposition.The development of light scattering patterns during the second stage phase separation followsthe Cahn-Hilliard model of spinodal demixing.Furthermore,the evolution of the scattering vector followsa Maxwell-type relaxation equation establishing the viscoelastic behavior of phase separation.The relaxationtime of phase separation can be described by the Williams-Landel-Ferry equation for viscoelasticity.As awhole,the dependence of phase separation on cure temperature and the development offinal morphologiesand the associated mechanisms were explored in detail for the complex epoxy/ABS system.1.IntroductionThe viscoelastic effects on phase separation and the evolution of phase morphology in thermoset-thermoplastic blends have attracted increased attention due to their great significance to optimize the mechanical properties.Systems such as polysul-fone-modified epoxy,1polyoxymethylene-modified epoxy,2etc. have been studied.On mixing thermoplastic with epoxy resin, one can obtain a homogeneous or heterogeneous mixture.Upon curing,phase separation starts immediately due to the increase in molecular weight of the epoxy resin,which results in a thermodynamically unstable system.3Tanaka and Araki reported that viscoelastic effects play a crucial role in phase separation for the blends with dynamic asymmetry.4-6In other words, viscoelastic effects on phase separation originate from the dynamic asymmetry in the relaxation and diffusion of molecular chains,which may be due to large difference in the T g and molecular weight of the blend components.7,8The phase separation may take place through two main mechanisms,which are nucleation and growth(NG)or spinodal decomposition (SD).9-14SALLS,OM,DSC,and rheology are the most imperative techniques to explore the phase separation behavior of polymer blends.15-17Apart from the evolution of phase separation,the curing reaction in epoxy/DDS/ABS blends is accompanied by chemical reactions and physical transformations from gelation to vitrifica-tion and hence results in a complex kinetic behavior and deserves a detailed exploration.In comparison with thermo-plastics,the processing of thermosets and thermoset matrix composites is more complicated and less controlled because of their reactivity.In these processes,polymer synthesis and shaping take place in a single operation:this involves the conversion of liquid monomers or polymers into solid cross-linked polymers.18The mechanism and kinetics of cure deter-mine the network morphology,which in turn decides the physical and mechanical properties of the cured product.The viscosity is a very important parameter to characterize accurately the process conditions and to visualize the gelation and vitrification transition state.19Thus,the knowledge of rheological*To whom correspondence should be addressed.Phone:+91-481-2730003.Fax:+91-481-2731002.E-mail:sabupolymer@,sabuchathukulam@.†School of Chemical Sciences,Mahatma Gandhi University.‡Catholic University of Leuven.§UMR6619CNRS Universite´d’Orle´ans.|Leibniz Institute of Polymer Research Dresden.⊥Centre for Nanoscience and Nanotechnology,Mahatma GandhiUniversity.J.Phys.Chem.B2010,114,13271–132811327110.1021/jp101661t 2010American Chemical SocietyPublished on Web10/06/2010and curing behavior of the resin system is particularly necessary to control the processing and subsequent engineering design.20 Moreover,the rheological analysis is indispensable tofind out the relationship between conversion,phase separation,and viscosity evolution for the process simulation.19For thermoset-ting systems in industrial applications,it is important to establish relations of transition phenomena to the reaction time at different cure temperature so that desired properties can be controlled.21 Furthermore,the study of mechanism of phase separation and the evolution of scattering vector(q)are very important to establish the effect of viscoelastic behavior on the resulting morphologies and properties.The cure temperature and polymer molecular weight are important factors influencing the thermodynamic and kinetic aspects of phase separation and thus thefinal morphology of the epoxy blends.However,the influence of cure temperature and polymer molecular weight on the phase separation has not been extensively studied to date.Moreover,the mechanism of phase separation is not fully understood for epoxy/ABS thermoplastic blends.In this paper,our focus is to understand the mechanism of phase separation in epoxy/ABS blends by considering all the above points.As shown in our previous publications,there is a high dependence of the cure kinetics andfinal morphologies on the thermoplastic concentration.13,22 Recently,we have also recognized that,for the epoxy blend system containing12.9wt%ABS,phase separation takes place by the combination of both NG and SD mechanism with a well-defined phase inversion process.14The main objective of this work is to investigate the affect of temperature and viscoelastic effects on the dynamics of phase separation of an ABS-modified epoxy system.For that purpose,a time-resolved study of the phase separation was carried out by OM,DSC,rheology,and SALLS.2.Experimental Section2.1.Materials Used.The matrix material used in the experiments consists of diglycidyl ether of bisphenol A(DGE-BA)(Lapox L-12,Atul Ltd.,India.)and4,4′-diaminodiphenyl-sulfone(DDS)(Lapox K-10,Atul Ltd.,India).The epoxy content in Lapox L-12varies between5.25and5.40eq/kg. Lapox L-12has a viscosity of about1.15-1.2g/cm3.DDS is a white powder with a melting point of178°C.It has a pot life of about75-115min at25°C.The toughener ABS(Poly lac PA-757K)was manufactured by Chi Mei Corp.,Taiwan.The used poly(acrylonitrile-butadiene-styrene)(ABS)is a com-mercially available thermoplastic polymer consisting of70wt %polystyrene(PS),25wt%acrylonitrile(AN),and5wt% polybutadiene(PB).The molecular weight of the soluble part of ABS was determined to be M n)51300g/mol and M w) 125200g/mol(PDI)2.44,GPC,PS standard)and the density was determined to be1.051g/cm3by means of an helium pycnometer.2.2.Preparation of Blends.The epoxy/ABS blend contain-ing12.9wt%ABS was prepared by mixing20g of ABS with 100g of DGEBA at180°C under constant stirring.After proper mixing,35g of DDS was added to epoxy/ABS mixture corresponding to a stoichiometric epoxy:amine ratio of2:1.The freshly prepared mixtures were immediately used for the analysis.2.3.Characterization Techniques.2.3.1.Optical Micros-copy.A few milligrams of the epoxy/ABS system placed between two glass slides were observed by a Leitz laborlux12 Pols optical microscope,while the sample was being cured in a Linkam CSS450shearing cell.The Linkam cell was only used for heating;no shear was applied.Digital micrographs were taken at several times by a Hamamatsu TSU digital camera controller C4742-95.2.3.2.Differential Scanning Calorimetry.The calorimetric measurements were performed on a Perkin-Elmer Pyris DSC6 differential scanning calorimeter.The instrument was calibrated with indium and dry cyclohexane standards.Dry nitrogen was used as purge gas and samples of15-20mg were analyzed. Dynamic DSC was done at2.5,5,7.5,and10°C/min from20 to350°C for the neat epoxy resin/DDS mixture.Isothermal measurements were done at150,165,and180°C.The curing was assumed to be completed when the isothermal curve leveled off to a straight line.The areas under the peaks during the isothermal cure were used to determine the conversion R at various times.The conversion R)∆H t/∆H tot,where∆H t is the heat of cure at time t,calculated by the integration of the DSC isothermal signal,and∆H tot is the total heat of cure of the neat epoxy resin calculated by the integration of DSC dynamic signal.2.3.3.Oscillatory Shear Rheology.The viscoelastic proper-ties of the epoxy blends during isothermal curing were determined by oscillatory shear measurements performed on an ARG2rheometer(TA Instruments).Each sample was placed on aluminum parallel plates of25mm in diameter,which were of disposable type.Temperature control was achieved by an ETC system(environmental testing chamber)suitable for studying the polymer melts in the temperature range-160to 600°C under a N2atmosphere.The experiments were carried out with5%strain and an angular frequency of1Hz and were used to determine G′,G′′,tanδ,and complex viscosity.2.3.4.Small-Angle Laser Light Scattering(SALLS).The small-angle light scattering setup(Newport model ULM-TILT) consists of a5mW He-Ne laser(λ)638.2nm)as the scattering light source.The sample is placed between two glass slides being cured in a Linkam cell(CSS450).The distance between the sample and screen was adjusted as29cm.The laser beam passing through the Linkam cell was examined by a Pulnix camera,which was used to record the change in scattering patterns.The processing of the data was performed using the special SALLS software developed in Catholic University,Leuven,Belgium.2.3.5.Atomic Force Microscopy.The AFM measurements were done in the tapping mode by a Dimension3100Nanoscope V(Veeco,USA).We used silicon-SPM sensors(Budget Sensors,Bulgaria)with a spring constant of ca.40N/m and resonance frequency of ca.280kHz;the tip radius is lower than 10nm.2.3.6.Field Emission Scanning Electron Microscopy.The morphology of the cross-linked epoxy blend was examined using a ULTRA FESEM(model ULTRA plus,Carl Zeiss NTS GmbH,Germany).The fractured samples were smoothed with an ultramicrotome and the SAN-rubber phase(SAN)styrene/ acrylonitrile)was etched out by immersing the cut in chloroform for2h.The samples were coated with platinum by vapor deposition using a SCD500sputter coater(BAL-TEC AG, Liechtenstein).3.Results3.1.Evolution of Phase Separation by OM.Figures1,2, and3demonstrate the phase separation and evolution of phase morphologies in the ABS-modified epoxy/DDS blends cured at different temperatures,illustrating different stages of the phase separation process from the initially formed matrix droplet morphology to thefinal complex structures.Before going into the detailed mechanism of phase separation in the ABS-modified13272J.Phys.Chem.B,Vol.114,No.42,2010Jyotishkumar et al.epoxy system,it is important to mention that the T g of SAN and PB are110and-80°C,respectively,while that of epoxy monomer is-20°C and hence there is a strong dynamic asymmetry.As mentioned in the Introduction,the other important factor that contributes to the dynamic asymmetry is the difference in molecular weight.The number-average mo-lecular weights of ABS and the epoxy resin(DGEBA)were M n)51300and355g/mol,respectively.This dynamic asymmetry can play a very important role in the dynamics of phase separation and thefinal morphology.Thefinal morphologies of12.9wt%ABS-modified epoxy blends cured at150and165°C are of phase-inverted type and are quite different from thefinal morphologies of ABS-modified epoxy blends cured at180°C,in which thefinal morphology is cocontinuous.This indicates that,at temperatures higher than 165°C,the rate of phase separation is faster and,as a result, the generated epoxy domains grow faster.In later stages,the growing epoxy domains start to agglomerate,resulting in the final cocontinuous morphology,in which both SAN phase and epoxy phase are continuous.The time at which the formation of the new epoxy phase due to phase separation could be detected and is listed in Table1.The effect of temperature on the phase separation was also carefully examined using DSC, rheology,and SALLS and the results are elaborated in detail in the coming text.From the evolution of morphology and resultant final morphologies at different temperatures,it was obvious that the temperature plays a prominent role for the growth rate of phase-separated structures and the resultingfinal phase morphologies.3.2.Calorimetric Analysis.The epoxy/ABS blend was cured isothermally at three different temperatures,i.e.,150,165,and 180°C.Figure4a shows the isothermal DSC curves of neat epoxy system and the ABS-modified epoxy blend cured at150°C.A rapid increase in the reaction rate was observed whichpasses through a maximum in the exothermic heatflow.In the presence of ABS the reaction rate is reduced.However,a second increase in reaction rate was observed in the DSC curve of the ABS-containing blend.The time at which this second increment in reaction rate occurs(appearance of the shoulder)is listed in Table 1.A similar kind of acceleration phenomenon was observed by Pascault and co-workers for the blends based on polystyrene or polyetherimide in DGEBA/MCDEA(4,4′-methylenebis(3-chloro-2,6-diethylaniline))systems3and poly-(methyl methacrylate)in a DGEBA/DDS system.23To study the effect of temperature on the rate and phase separation of the blend,a comparison of curing rate vs time in the ABS-modified epoxy cured at150,165,and180°C is shown in Figure4b.The peak maximum and the shoulder are slightly shifted toward shorter cure times with increase in temperature. This reflects an increase in the reaction rate as well as the rate of phase separation at higher cure temperatures supporting the OM micrographs.This is because of the increased molecular mobility that favors the chemical interactions such that a higher number of chains are involved in the curing process and,as a consequence,both the rate of the reaction and rate of phase separation are increased.3.3.Rheological Behavior.The profile of change in complex viscosity of ABS-modified epoxy blends with time at different temperatures(150-180°C)is shown in Figure5.In general, the rheology on polymer blends is an accepted method to provide valuable information on the structure development during curing.At the beginning of the cure,the viscosity slowly increases with time and then,at a certain point,a very rapid increase is observed.Moreover,this increase in complex viscosity curves is shifted to shorter times with increase in temperature;this is because the reactions between epoxy and DDS are thermally accelerated.The reaction between epoxyFigure1.Evolution of phase morphologies of ABS-modified epoxy blends at150°C:(a)360s,(b)2220s,(c)2420s,(d)2600s,(e) 2900s,(f)3680s,and(g)9000s(800×800µm2).Figure2.Evolution of phase morphologies of ABS-modified epoxy blends at165°C:(a)480s,(b)1440s,and(c)6000s(800×800µm2).Figure3.Evolution of phase morphologies of ABS-modified epoxy blends at180°C:(a)480s,(b)960s,and(c)6000s(800×800µm2).TABLE1:Time for the Generation of New Epoxy Phase Due to Complex Phase Separationtemp(°C)time for theappearance ofsecond increasein reaction rate(shoulder)fromDSC(s)time for thegeneration of newepoxy phasefrom OM(s)time for thegeneration ofnew epoxy phasefrom rheology(s) 150244024002106 165122013801021 180760920662Phase Separation of an ABS-Modified Epoxy System J.Phys.Chem.B,Vol.114,No.42,201013273and DDS induces various chemical and physical phenomena. In fact,the resin viscosity increases and cross densification advances.The curing reaction proceeds until vitrification or until the transformation of the rubbery network into a glassy solid takes place.24-26It is well-known that the phase separation has a profound effect on the rheological behavior of the blends.It is important to mention that phase separation is triggered by the NG mechanism,which takes place immediately after mixing the curing agent into the epoxy monomer/ABS blend.14This means that phase separation may affect the curing reaction even at very low conversion but this may not be prominent.On the other hand,we have an increase in reaction rate of the epoxy amine system during the second stage of phase separation by spinodal decomposition.This means that the phase separation affects the reaction rate and hence may influence the gelation times of the epoxy blends.However,we have difficulties to predict the exact gelation times of the epoxy blends since they are always coupled with the phase separation process.We have investigated the evolution of rheological parameters such as tanδ,G′,and G′′at different temperatures;the results seem to be identical irrespective of temperature.For avoiding overlapping of the results,we are giving only the representative rheological profile obtained at150°C.Figure6reveals the rheological parameter as a function of real time/conversion scale in case of epoxy/ABS-modified epoxy cured at150°C.The rheological curves reveal a reduction in the tanδcurve during the early stages of the reaction,which may be due to dominance of SAN-rich phase(the generation of thermoplastic-rich con-tinuous phase)and is accompanied by a gradual increase in viscosity;hence,this drop in tanδcurve supports the results from OM micrographs.During the early stages of the reaction, G′is below G′′,indicating the liquid nature of the material; after some time,both G′and G′′increase rapidly.As mentioned before,this phenomenon may be due to the formation of continuous SAN-rich phase;this is followed by a crossover point where G′equals G′′which means the system acts as both elastic and viscous,storing and dissipating an identical amount of energy at this point.Pascault et al.observed a similar kind of behavior for the blends based on polystyrene(PS)or polyether-imide(PEI)in DGEBA/MCDEA systems3and poly(methyl methacrylate)(PMMA)in the DGEBA/DDS system.233.4.Phase Separation Dynamics by SALLS.The phase separation process of all modified systems was monitored in situ by SALLS.The change in scattered intensity and scattering vector(q)was recorded at appropriate time intervals during isothermal curing at135,140,150,160,165,170,and180°C. At all curing temperatures,the light scattering profile remains the same irrespective of the temperature.Figure4.(a)Curing rate against conversion plot for neat resin and epoxy/ABS blends cured at150°C.(b)Curing rate against time plot for epoxy/ABS blends cured at150,165,and180°C.plex viscosity of ABS-modified epoxy blends at150, 165,and180°C.Figure6.Storage modulus,loss modulus,and tanδvs time curves of modified epoxy blends at150°C.13274J.Phys.Chem.B,Vol.114,No.42,2010Jyotishkumar etal.Figure 7a shows the scattering intensity vs scattering vector (q )for with 12.9wt %ABS-modified blends cured at 135°C during the very early stage of phase separation.Here the scattering peaks at low q values are observable at the beginning of cure,implying the presence of microphase separation.The following factors are possible for the initiation of microphase separation:the first one could be the presence of SAN-grafted PB particles,and the PB phase in SAN-grafted PB is cross-linked and hence they are insoluble in epoxy resin.The presence of insoluble SAN-grafted PB may initiate the phase separation process.The second reason for phase separation probably from the thermal treatment of samples at the temperature as high as 180°C.These initial scattering patterns during early stages of phase separation exactly match with our previous observationsFigure 7.Scattering intensity vs scattering vector profile for the ABS-modified epoxy system cured at (a,b)135,(c)150,(d)165,and (e)180°C.Phase Separation of an ABS-Modified Epoxy System J.Phys.Chem.B,Vol.114,No.42,201013275for NG mechanism.14Figure7b shows the scattering intensity vs scattering vector(q)during the second-stage phase separation. Scattering peaks are at larger q values originating at the beginning of the second stage phase separation and are typical for SD.These peaks shift rapidly to lower q values due to epoxy phase coarsening.A clear shift of the main peak in the intermediate to late stages establishes an SD mechanism. Furthermore,a sharp increase in intensity of scattered light from the beginning of second-stage phase of separation was observed. Figure7c-e shows the scattering intensity vs scattering vector during the second-stage phase separation by SD mechanism at 150,165,and180°C,respectively.From the light scattering profiles at different temperatures,we observe a rapid shift in the scattering vector to lower values at180°C as compared to light scattering profiles at lower temperatures,establishing rapid epoxy phase coarsening by coalescence at high temperatures. This supports the facts observed by OM,DSC,and rheological measurements.Even though thefinal morphologies are different at different temperatures,the shape of the scattering profiles looks almost identical.3.5.Final Morphology by AFM and FESEM.AFM investigations were made to understand thefinal morphology in detail.According to Magonow et al.,27we choose the scan conditions(free amplitude>100nm,set-point amplitude ratio 0.8)in order to get stiffness contrast in the phase image,which means bright features in the phase image are stiffer than dark areas.Parts a and b of Figure8show the AFM micrograph of neat ABS and completely cured ABS-modified epoxy/DDS system at180°C.The AFM micrograph of ABS shows that the cross-linked PB phase is dispersed in the SAN continuous phase,indicating two-phase morphology.The dispersed PB domains consist of small agglomerates of original PB(dark phase)particles containing some(bright phase)grafted SAN. On the other hand,the AFM micrographs of the completely cross-linked ABS/epoxy blend show a very interesting morphol-ogy with three different phases:two continuous phases forming a cocontinuous structure with substructures(epoxy continuous phase containing dispersed SAN particles and the SAN continu-ous phase in which epoxy particles are dispersed)and the most important feature,the PB phase appears as dispersed small agglomerates at the blend interface between the cocontinuous structures.The driving force for the segregation at the interface between the SAN and epoxy continuous phases is the minimiza-tion of the specific interfacial energy of the system,but there is still a tendency to locate more near to the SAN phase due to the better compatibility caused by the grafted SAN part.A minor quantity of PB is dispersed in the SAN continuous phase. For the better understanding of thefinal generated complex morphology of ABS-modified epoxy/DDS system at180°C, the blend was carefully examined by FESEM.FESEM micro-graph of epoxy/ABS blend after ultramicrotome cutting followed by etching in chloroform for2h is shown in Figure8c.During the etching process,the SAN phase has been dissolved away by chloroform.FESEM micrograph of the12.9wt%ABS-containing cross-linked epoxy specimen exhibits a cocontinuous structure in which both the epoxy phase as well as the SAN phase are continuous.In the continuous epoxy phase,SAN particles are dispersed,while in the continuous SAN phase clusters of big epoxy particles were observed.These particles result from viscoelastic phase separation as described below.4.DiscussionIn general,the phase separation phenomenon in the modified epoxy blends may be interpreted as follows based on OM analysis.The system was partially miscible initially(before curing).There were only the small insoluble SAN-graft-PB particles present in the homogeneous DGEBA/DDS/SAN mixture.Because of the thermal treatment of the samples,these SAN-graft-PB particles dispersed in the homogeneous epoxy/ SAN mixture may initiate the phase separation through nucle-ation and growth mechanism.During the initial stages of phase separation,spherical SAN nuclei are found to develop in the epoxy matrix by the nucleation process.More and more SAN particles may form by NG as the curing time advances(Figures 1a,2a,3a).After a few minutes,the system transforms into a cocontinuous structure,like the one in Figure1b.The cocon-tinuous structure may come from the SD mechanism,in which both SAN-rich phase with PB particles and epoxy-rich phase are continuous.After this point,the cocontinuous structure ruptures rapidly and the SAN-rich phase with PB particles becomes the continuous phase while the epoxy phase becomes the dispersed phase,indicating a clear phase inversion at which epoxy particles develop in the SAN continuous matrix as shown in Figure1c.This transition in the phase behavior could be explained as follows.The enhancement of the concentration fluctuation makes the SAN-rich phase more elastic(due to its high molecular weight)than epoxy-rich phase such that these epoxy droplets subsequently grow by the diffusion process (Figure1d-g).During the fast growth of the epoxy phase,there may be some miscible SAN chains get separated out of the SAN-rich phase.These original miscible SAN chainsfinally phase separate due to the reduced miscibility caused by the epoxy network formation resulting in the formation of SAN substructures in the epoxy-rich matrix.On the other hand,during the phase inversion process,some epoxy will not reach the growing epoxy-rich domains since the diffusion is hampered by the highly viscous SAN surrounding.This part of the epoxy resin trapped in the SAN-rich phase separates in later stages to spherical epoxy substructures in the SAN-rich continuous phase. The immiscible PB particles are driven during this process to the interface of the bulky epoxy phase and SAN phase,with a tendency to have more contacts with the SAN phase due to the better compatibility caused by the grafted SAN part.Kyu et al. reported similar kind of complex crossover behavior of reaction-induced phase separation from NG to SD.28These observations are completely different from the simple NG mechanism advocated by Pascault et al.8,29-32and the SD process described by Inoue.12A schematic model describing the evolution of phase separa-tion based on OM,AFM,and FESEM investigations is given in Figure9.Figure9a shows the schematic representation of immiscible SAN-graft-PB particles dispersed in a homogeneous epoxy/SAN mixture.In Figure9b,the NG mechanism possibly initiated by SAN-graft-PB particles is depicted,which results in SAN particles with PB inclusions dispersed in the epoxy matrix.Figure9c shows that the change from the NG mecha-nism to the SD mechanism results in the formation of cocon-tinuous structures of both the epoxy phase and the SAN phase. Obviously,the PB will be in the SAN phase.Figure9d shows a rapid phase inversion in which the SAN phase with PB particles becomes the continuous phase and epoxy becomes the droplet.Eventually,Figure9e represents thefinal morphological structures obtained at different curing temperatures.As mentioned in the Results,thefinal morphology of ABS/ epoxy blends cured at150and165°C is of phase-inverted type and is different from thefinal morphology of blends cured at 180°C,in which thefinal morphology is cocontinuous.This effect of temperature on thefinal morphologies can be explained13276J.Phys.Chem.B,Vol.114,No.42,2010Jyotishkumar et al.。