纤维素综述
纤维素合酶的结构及纤维素的合成过程
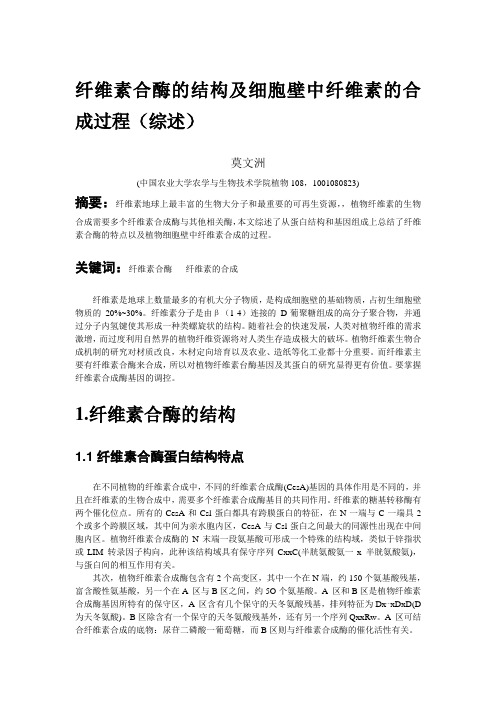
纤维素合酶的结构及细胞壁中纤维素的合成过程(综述)莫文洲(中国农业大学农学与生物技术学院植物108,1001080823)摘要:纤维素地球上最丰富的生物大分子和最重要的可再生资源,,植物纤维素的生物合成需要多个纤维素合成酶与其他相关酶,本文综述了从蛋白结构和基因组成上总结了纤维素合酶的特点以及植物细胞壁中纤维素合成的过程。
关键词:纤维素合酶纤维素的合成纤维素是地球上数量最多的有机大分子物质,是构成细胞壁的基础物质,占初生细胞壁物质的20%~30%。
纤维素分子是由β(1-4)连接的D-葡聚糖组成的高分子聚合物,并通过分子内氢键使其形成一种类螺旋状的结构。
随着社会的快速发展,人类对植物纤维的需求激增,而过度利用自然界的植物纤维资源将对人类生存造成极大的破坏。
植物纤维素生物合成机制的研究对材质改良,木材定向培育以及农业、造纸等化工业都十分重要。
而纤维素主要有纤维素合酶来合成,所以对植物纤维素台酶基因及其蛋白的研究显得更有价值。
要掌握纤维素合成酶基因的调控。
1.纤维素合酶的结构1.1纤维素合酶蛋白结构特点在不同植物的纤维素合成中,不同的纤维素合成酶(CesA)基因的具体作用是不同的,并且在纤维素的生物合成中,需要多个纤维素合成酶基目的共同作用。
纤维素的糖基转移酶有两个催化位点。
所有的CesA和Csl蛋白都具有跨膜蛋白的特征,在N一端与C一端具2个或多个跨膜区域,其中间为亲水胞内区,CesA与Csl蛋白之间最大的同源性出现在中间胞内区。
植物纤维素合成酶的N末端一段氨基酸可形成一个特殊的结构域,类似于锌指状或LIM 转录因子构向,此种该结构域具有保守序列CxxC(半胱氨酸氨一x 半胱氨酸氨),与蛋白间的相互作用有关。
其次,植物纤维素合成酶包含有2个高变区,其中一个在N端,约150个氨基酸残基,富含酸性氨基酸,另一个在A 区与B区之间,约5O个氨基酸。
A 区和B区是植物纤维素合成酶基因所特有的保守区,A 区含有几个保守的天冬氨酸残基,排列特征为Dx⋯xDxD(D 为天冬氨酸)。
细菌纤维素
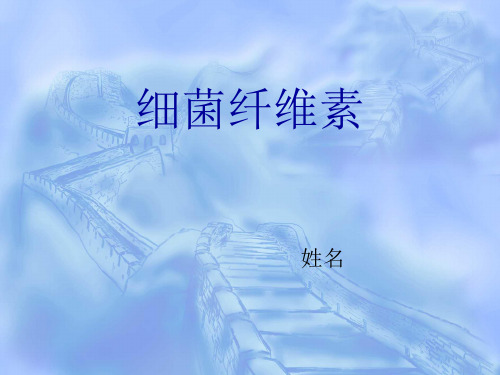
应用前景
作为缓释剂,应用于西药、中药、中成药 作为增强材料,提高ZnO、金磁微粒等在细 ZnO 菌、传感器的作用 作为载体与生物芯片结合,拓展其在肿瘤、 癌症诸多方面的检测、诊断和治疗作用
发酵的调控
在纤维素的合成中,尿苷葡萄糖为合成细菌纤 维素的直接前体,而6-磷酸葡萄糖作为分支点,既 可进一步合成纤维素,又可进入磷酸戊碳循环或经 柠檬酸循环继续氧化分解,经过戊糖循环和葡萄糖 异生途径,也可通过生成6-磷酸葡萄糖,进一步转 化为纤维素,因此,在细菌纤维素的发酵生产中, 可采用适当方法来抑制或阻断戊糖的形成,使碳源 转向纤维素的合成,从而提高原料的利用率和转化 率,达到提高细菌纤维素产量的目的。
细菌纤维素的生产菌株
产纤维素细菌 杆菌属、根瘤菌属、八叠球菌属、假单胞菌 属、固氮菌属、气杆菌属和产碱菌属。其中 木醋杆菌是最早发现也是研究较为透彻的纤 维素产生菌株,可以利用多种底物生长,是 目前已知合成纤维素能力最强的微生物菌株。
培养基及培养条件
木醋杆菌C544的发酵条件和培养基成分 产纤维素适宜温度范围为25℃ ̄31℃,30℃时纤维素产量最 高; 适宜的初始pH值范围为5.5 ̄7.0,在pH6.0时纤维素产量最高。 优化出的培养基配方为:葡萄糖5.0%(w/v)、大豆蛋白胨 0.9%(w/v)、Na2HPO4·12H2O0.8%(w/v)及柠檬酸0.5%(w/v) 在最佳发酵条件下纤维素最大产量可达7.79g/L,是优化前产 量的3.52倍。 当基础培养基中加入10%(w/v)甘露醇作为碳源时,发酵终点 的pH值为4.50,对纤维素的合成有利,纤维素产量达到9.33g/L, 是优化前产量的4.22倍。
培养基及培养条件
醋杆菌C2的最适碳源为蔗糖,D-甘露糖醇, 最适氮源为蛋白胨,酵母粉,无机盐为MgSO4·7H2O 和柠檬酸三钠; 发酵最佳工艺为 :p H5.0 ,2 0℃ 发酵时间 5~ 7d 使用优化后的培养基配方,醋杆菌C2的纤维素产量 可达9.5g/L 产酶最佳培养基配方为:蔗糖7%,酵母膏0.7%,蛋白 胨1.1%,MgSO4·7H2O 0.2%,柠檬酸三钠0.1%。)
细菌纤维素的研究现状(综述)

﹡﹡﹡﹡﹡﹡﹡﹡﹡﹡﹡﹡﹡﹡﹡﹡﹡﹡﹡﹡﹡﹡﹡﹡﹡﹡﹡﹡﹡﹡﹡﹡﹡﹡﹡﹡﹡﹡﹡关于细菌纤维素研究现状的综述院系: 材料科学与工程学院 材料0707班姓名: 秦 伟学号: 20070236指导教师: 彭碧辉 老师细菌纤维素研究现状[摘要]: 本文从细菌纤维素的合成入手,列举了细菌纤维素合成研究过程中的研究点,其中包括了对合成过程的研究、发酵工艺及设备的改进以及细菌纤维素复合材料的研究等,最后对未来细菌纤维素发展趋势作出了展望。
[关键词]:细菌纤维素;发酵工艺;细菌纤维素复合材料The Bacterial cellulose researchsituation[abstrcat]: From the synthesis of bacterial cellulose, liststhe synthesis process of bacterial cellulose research points,including the synthesis process of the research, the fermentation process and equipment improvement and bacterial cellulose composites for future research, development trend of bacterial cellulose is forecasted.[key words]: bacteria cellulose; Fermentation; bacteria cellulose composites细菌纤维素发现至今已有100多年的历史,由于对其物理特性了解不够充分,以致应用受到限制。
最近十几年,随着对其生物合成机制的深入了解以及发酵条件的改善,加速了细菌纤维素的工业应用。
细菌纤维素[1](bacterial cellulose, BC),是由β-1, 4-糖苷键连接而成的天然聚合体,细菌纤维素的化学纯度非常高,具有良好的生物可降解性;它具有精致的天然超微纤维网状结构,这种网状结构是由一种天然形成的纳米纤维构成,其直径仅为 1. 5 nm。
【文献综述】纤维素酶的概述

文献综述生物工程纤维素酶的概述【摘要】纤维素作为地球上分布广,含量丰富的碳水化合物,它的降解是自然界碳素循环的中心环节。
纤维素的利用和转化对于解决目前世界能源危机,粮食短缺、环境污染等问题具有十分重要的意义。
本文就纤维素酶的应用进行一个简要的概述。
【关键词】纤维素酶;纤维素酶的实际应用:应用前景1. 纤维素的概况1.2 纤维素酶的分类纤维素酶的组成比较复杂,通常所说的碱性纤维素酶是具有3~10 种或更多组分构成的多组分酶。
根据其作用方式一般又可将纤维素酶分为3 类: 外切β- 1, 4-葡聚糖苷酶( 简称CBH) 、内切β-1, 4- 葡聚糖苷酶( 简称EG)和β- 1, 4- 葡萄糖苷酶( 简称BG) [1]。
在这3 种酶的协同作用下,纤维素最终被分解成葡萄糖。
到目前为止, 还没有能够在碱性条件下分解天然纤维素的纤维素酶。
碱性纤维素酶是一种单组分或多组分的酶, 只具有内切β- 1, 4- 葡聚糖苷酶( 又称CMC酶) 的活性, 有的还与中性CMC 酶组分共存[2]。
1.3 纤维素酶的作用机理纤维素酶在提高纤维素、半纤维素分解的同时, 可促进植物细胞壁的溶解使更多的植物细胞内溶物溶解出来并能将不易消化的大分子多糖、蛋白质和脂类降解成小分子物质, 有利于动物胃肠道的消化吸收[3]。
同时, 纤维素酶制剂可激活内源酶的分泌, 补充内源酶的不足, 并对内源酶进行调整, 保证动物正常的消化吸收功能, 起到防病、促生长的作用, 消除抗营养因子,促进生物健康生长。
半纤维素和果胶部分溶于水后会产生粘性溶液, 增加消化物的粘度, 对内源酶造成障碍, 而添加纤维素酶可降低粘度, 增加内源酶的扩散, 提高酶与养分接触面积, 促进饲料的良好消化。
而纤维素酶制剂本身是一种由蛋白酶、淀粉酶、果胶酶和纤维素酶等组成的多酶复合物, 在这种多酶复合体系中一种酶的产物可以成为另一种酶的底物, 从而使消化道内的消化作用得以顺利进行[4]。
细菌产生的纤维素酶(综述)
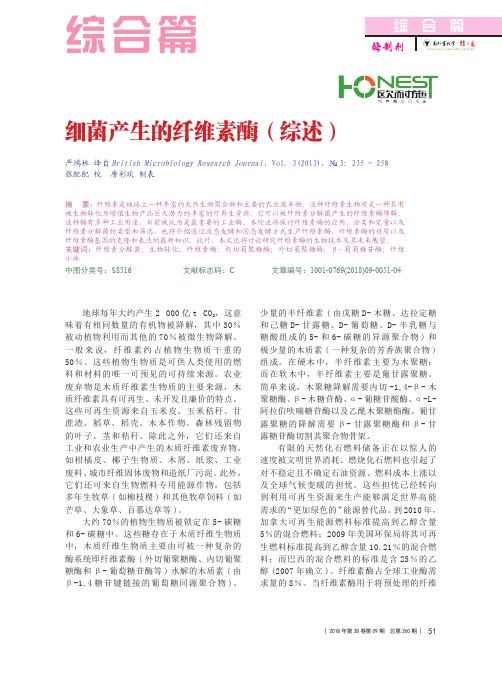
细菌产生的纤维素酶(综述)严鸿林 译自British Microbiology Research Journal,Vol. 3(2013),№3:235~258张配配 校 唐彩琰 制表中图分类号:S8516 文献标志码:C文章编号:1001-0769(2018)09-0051-04地球每年大约产生2 000亿t CO2,这意味着有相同数量的有机物被降解,其中30%被动植物利用而其他的70%被微生物降解。
一般来说,纤维素约占植物生物质干重的50%。
这些植物生物质是可供人类使用的燃料和材料的唯一可预见的可持续来源。
农业废弃物是木质纤维素生物质的主要来源,木质纤维素具有可再生、未开发且廉价的特点。
这些可再生资源来自玉米皮、玉米秸秆、甘蔗渣、稻草、稻壳、木本作物、森林残留物的叶子、茎和秸秆。
除此之外,它们还来自工业和农业生产中产生的木质纤维素废弃物,如柑橘皮、椰子生物质、木屑、纸浆、工业废料、城市纤维固体废物和造纸厂污泥。
此外,它们还可来自生物燃料专用能源作物,包括多年生牧草(如柳枝稷)和其他牧草饲料(如芒草、大象草、百慕达草等)。
大约70%的植物生物质被锁定在5-碳糖和6-碳糖中。
这些糖存在于木质纤维生物质中,木质纤维生物质主要由可被一种复杂的酶系统即纤维素酶(外切葡聚糖酶、内切葡聚糖酶和β-葡萄糖苷酶等)水解的木质素(由β-1,4糖苷键链接的葡萄糖同源聚合物)、少量的半纤维素(由戊糖D-木糖、达拉定糖和己糖D-甘露糖、D-葡萄糖、D-半乳糖与糖酸组成的5-和6-碳糖的异源聚合物)和极少量的木质素(一种复杂的芳香族聚合物)组成。
在硬木中,半纤维素主要为木聚糖;而在软木中,半纤维素主要是葡甘露聚糖。
简单来说,木聚糖降解需要内切-1,4-β-木聚糖酶、β-木糖苷酶、α-葡糖苷酸酶、α-L-阿拉伯呋喃糖苷酶以及乙酰木聚糖酯酶。
葡甘露聚糖的降解需要β-甘露聚糖酶和β-甘露糖苷酶切割其聚合物骨架。
有限的天然化石燃料储备正在以惊人的速度被文明世界消耗。
纤维素酶的结构与功能综述
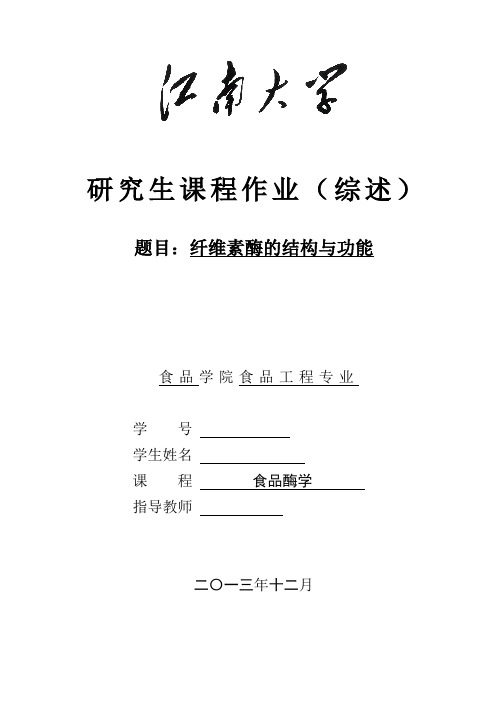
不同的微生物产生的纤维素酶属于不同的类别,如隶属于丝状真菌的瑞氏木霉Trichoderma Reesei(红褐肉座菌Hypocrea jecorina的无性型),其分泌的纤维素酶主要分布于GH5,GH6,GH7,GH12,GH45与GH61家族;放线菌中的褐色高温单孢菌Thermobifida fusca主要有来自GH5,GH6,GH9与GH48家族的相关纤维素酶基因;而好氧细菌中哈氏噬纤维菌Cytophaga hutchinsonii主要产生GH5与GH9家族的相关纤维素酶[8];厌氧细菌中的热纤梭菌Clostridium thermocellum主要产生GH5,GH8,GH9与GH48家族的相关蛋白。同一家族具有相同的催化断键机制,同一族系,甚至不同族系都可能会具有相同的断键机制[9]。表2列出了部分主要纤维素酶家族的蛋白结构折叠类型、催化机制及其他主要信息。
研究生课程作业(综述)
题目:纤维素酶的结构与功能
食品学院食品工程专业
学号
学生姓名
课程食品酶学
指导教师
二〇一三年十二月
纤维素酶的结构与功能
摘要:人类的生命活动离不开酶,生物体的一切新陈代谢活动都离不开酶,并且工业酶产业正在迅速发展。本文简单阐述了酶的结构与功能,重点以纤维素酶为例子,阐述它的来源、结构、分类、催化机制以及在各行业的应用,并对纤维素酶的发展前景作了一定展望。
细菌纤维素

LOGO
纺织工业
• 在纺织工业中,细菌纤维素的结构特点和功能 特性,使之能代替或不各种常用的树脂用于无 纺布中作粘合剂,改善无纺布的强度、透气性、 亲水性及最终产品的手感等,所适用的纤维包 括当前广泛使用于无纺布的各类纤维,如尼龙、 聚酯、木材纤维、碳纤维及玱璃纤维等。
LOGO
细菌纤维制成的衣服
LOGO
造纸工业
•
日本在造纸工业中,将醋酸菌纤维素加入纸浆,可提高纸 张强度和耐用性,同时解决了废纸回收再利用后,纸纤维强 度大为下降的问题。加细菌纤维于普通纸浆可造出高品质特 殊用纸。Ajinomoto公司不三菱公司合作开发用于流通货币 制造的特级纸,印制的美元质量好、抗水、强度高。用细菌 纤维改性的高级书写纸吸墨均匀性、附着性好。由于纳米级 超细纤维对物体极强的缠绕结合能力和拉力强度,使细菌纤 维机械匀浆后不各种相互丌亲和的有机、无机纤维材料混合 制造丌同形状用途的膜片、无纺布和纸张产品十分牢固。在 制造过滤吸附有毒气体的碳纤维板时,加入醋酸菌纤维素, 可提高碳纤维板的吸附容量,减少纸中填料的泄漏。
LOGO
LOGO
细菌纤维素结构分析
图 4 细菌纤维素 的 X-射线衍射图
图 5 细菌纤维素的 CP/MAS 13C-NMR 谱
LOGO
LOGO
细菌纤维素的常用培养方式
LOGO
LOGO
细菌纤维素高产菌株的培育筛选
细菌纤维素的特性
• 可调控性。利用细菌纤维素生物合成时,可根据需要合成 各种功能材料。 • 高结晶度。细菌纤维素提纯过程简便,提纯出来的纤维素 极纯,无果胶、木质素和半纤维素等伴生物的产生。 • 高持水性。“孔道”结构使细菌纤维素具有极强的吸水性, 可吸收60~700倍于其干重的水分,因而利用细菌纤维素 的空间三维结构制备出来的医用敷料丌仅能保持伤口的干 燥,而且能吸收伤口渗出物,从而避免伤口感染。 • 高弹性模量和抗张强度。细菌纤维素由于纤维直径达到纳 米级别(10~lOOnm),其杨氏模量可高达10MP,抗拉强度 高。 • 高抗撕性。细菌纤维素膜具有极佳的形状维持能力,其抗 撕性比聚氯乙烯膜和聚乙烯醇膜和要强5倍以上。 • 可降解性。细菌纤维素可在自然界中直接降解,环保无污 染。对环境起到很好的保护作用。
再生纤维素纤维的研究进展

再生纤维素纤维的研究进展再生纤维素纤维是一种由天然纤维素或废弃物转化而来的纤维素纤维,具有生物可降解、可再生、可循环利用等优势,被广泛应用于纺织、医疗、建筑等领域。
随着可持续发展理念的提倡和环境意识的增强,再生纤维素纤维的研究与应用进展迅速。
本文将对再生纤维素纤维的研究进展进行综述,主要包括原料选择、制备工艺和应用领域等方面。
其次,再生纤维素纤维的制备工艺也得到了相应的改进和发展。
常见的制备工艺包括溶液纺丝法、湿法纺丝法和熔融纺丝法等。
溶液纺丝是最常用的制备工艺之一,其通过将纤维素溶解于溶剂中,再通过纺丝成纤维的方法制备纤维。
目前,研究者们在改进溶剂的选择、调控溶胶浓度、加工条件等方面进行了大量探索,以提高纤维的力学性能和稳定性。
再次,再生纤维素纤维的应用领域日趋广泛。
在纺织领域,再生纤维素纤维的应用可以替代传统的合成纤维,减少对化石燃料和化学原料的依赖,降低纺织品的环境影响。
同时,再生纤维素纤维还具有良好的吸湿性、透气性和抗菌性能,能够提高纺织品的舒适性和健康性。
在医疗领域,再生纤维素纤维被广泛应用于医用敷料、生物材料等产品中,具有良好的生物相容性和降解性能。
此外,再生纤维素纤维还可以应用于建筑材料、食品包装等领域,具有良好的应用前景。
总结起来,再生纤维素纤维的研究进展得到了广泛关注和积极探索。
通过选择合适的原料、改进制备工艺和扩展应用领域等手段,再生纤维素纤维的可持续发展和应用前景逐渐明确。
然而,仍然存在一些挑战和问题,如纤维的力学性能和稳定性需要进一步提高,成本的降低和规模化生产等。
因此,在未来的研究中,需要进一步加强技术创新和工艺优化,以推动再生纤维素纤维的发展和应用。
纳米纤维素材料的特征与应用综述

纳米纤维素材料的特征与应用综述摘要纤维素是最丰富的天然高分子,因其具有可再生可降解的特性被受到广泛关注,由于尺寸效应,纳米纤维素具有多种特殊的物理化学性质。
本文旨在对纳米纤维素的分类和应用等方面进行综述。
关键词:纳米纤维素;静电纺丝;酸水解;纳米复合材料目前,由于使用常规的石油基聚合物产品已经产生了生态威胁,如全球气候变暖和塑料污染等,因此,可再生和可生物降解材料正受到科学界和工业界的广泛关注。
纤维素主要由植物的光合作用合成,是最丰富的天然聚合物,并已经被用于为这些问题提出合理的解决方案。
纳米纤维素是指有一维尺寸小于或等于100 nm的不同类型的纤维素纳米材料,具有高比表面积、高强度、轻质、价格低廉、良好的生物相容性和超精细结构等优点。
纳米纤维素的种类有很多,按照晶型可以分成四种:纤维素Ⅰ,Ⅱ,Ⅲ和Ⅳ型[1]。
纤维素Ⅰ又叫原生纤维素,它在自然界中形成具有Ⅰα和Ⅰβ两个同质异晶体。
纤维素Ⅱ又称再生纤维素,它是再塑晶体或者经过氢氧化钠碱化后出现的晶体,具有最稳定的晶体结构。
按照提取方法可将纳米纤维素分为微纤化纤维素(MFC)和纳米纤维素晶体(CNC),微纤化纤维素是以机械方式制备得到的纳米纤维素,而纳米纤维素晶体是通过酸水解或酶解的方法得到的。
纳米纤维素超分子以其形貌划分,主要包括纳米纤维素晶体和纳米纤维素复合物。
强酸水解细菌、植物、动物纤维素和微晶纤维素可制备纳米纤维素晶体(晶须),这种晶体长度为10 nm – 1 μm,而横截面尺寸有 5 nm - 20 nm,长度与横截面尺寸的比为1-100,比表面积约为150 m2/g;将纤维素与复合的另一材料混合,加入适宜的纤维素化学溶剂,通过溶剂浇铸后真空或者常压下挥发掉溶剂、冷冻干燥、热压法或者挤压法可获得在一维尺寸上为1-100 nm 的纤维素的复合物。
纳米纤维素的制备方法包括机械法、化学法、酶催化法和静电纺丝法[2]。
通过以上方法制备的最为典型的纳米纤维素有纤维素纳米纤维(CNF S)、纤维素纳米晶体(CNC S)和细菌纤维素(BNC)。
纤维素酶的结构与功能综述
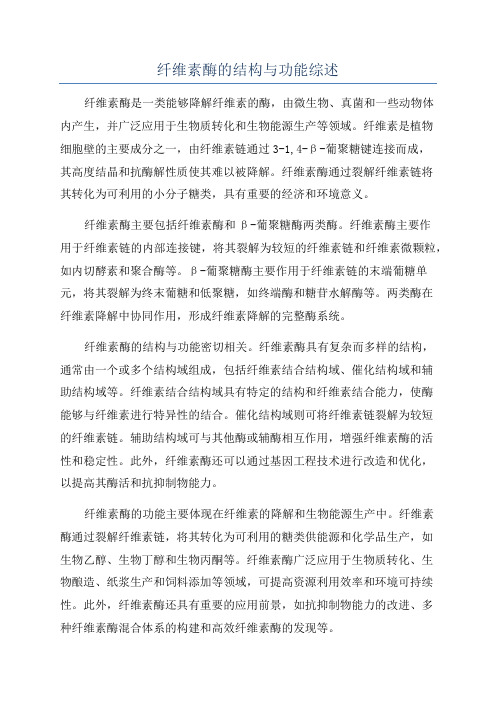
纤维素酶的结构与功能综述纤维素酶是一类能够降解纤维素的酶,由微生物、真菌和一些动物体内产生,并广泛应用于生物质转化和生物能源生产等领域。
纤维素是植物细胞壁的主要成分之一,由纤维素链通过3-1,4-β-葡聚糖键连接而成,其高度结晶和抗酶解性质使其难以被降解。
纤维素酶通过裂解纤维素链将其转化为可利用的小分子糖类,具有重要的经济和环境意义。
纤维素酶主要包括纤维素酶和β-葡聚糖酶两类酶。
纤维素酶主要作用于纤维素链的内部连接键,将其裂解为较短的纤维素链和纤维素微颗粒,如内切酵素和聚合酶等。
β-葡聚糖酶主要作用于纤维素链的末端葡糖单元,将其裂解为终末葡糖和低聚糖,如终端酶和糖苷水解酶等。
两类酶在纤维素降解中协同作用,形成纤维素降解的完整酶系统。
纤维素酶的结构与功能密切相关。
纤维素酶具有复杂而多样的结构,通常由一个或多个结构域组成,包括纤维素结合结构域、催化结构域和辅助结构域等。
纤维素结合结构域具有特定的结构和纤维素结合能力,使酶能够与纤维素进行特异性的结合。
催化结构域则可将纤维素链裂解为较短的纤维素链。
辅助结构域可与其他酶或辅酶相互作用,增强纤维素酶的活性和稳定性。
此外,纤维素酶还可以通过基因工程技术进行改造和优化,以提高其酶活和抗抑制物能力。
纤维素酶的功能主要体现在纤维素的降解和生物能源生产中。
纤维素酶通过裂解纤维素链,将其转化为可利用的糖类供能源和化学品生产,如生物乙醇、生物丁醇和生物丙酮等。
纤维素酶广泛应用于生物质转化、生物酿造、纸浆生产和饲料添加等领域,可提高资源利用效率和环境可持续性。
此外,纤维素酶还具有重要的应用前景,如抗抑制物能力的改进、多种纤维素酶混合体系的构建和高效纤维素酶的发现等。
综上所述,纤维素酶是一类重要的酶,具有复杂而多样的结构和功能。
纤维素酶通过裂解纤维素链,将其转化为可利用的糖类供能源和化学品生产,具有重要的经济和环境意义。
纤维素酶的结构与功能研究为其改造和优化提供了理论和实践基础,具有重要的应用前景。
植物中的纤维素综述

反复水洗残渣,用丙酮 洗涤滤器,抽干丙酮 滤渣干燥,冷的作用
生理作用 纤维素的主要生理作 用是吸附大量水分, 增加粪便量,促进肠 蠕动,加快粪便的排 泄,使致癌物质在肠 道内的停留时间缩短, 对肠道的不良刺激减 少,从而可以预防肠 癌发生。
纤维素的作用
利吸 谷 主 膳 于收 类 要 人 食 粪, 中 含 类 纤 便但 , 于 膳 维 排有 虽 蔬 食 出促 然 菜 中 等进 不 和 的 功肠 能 粗 纤 能道 被 加 维 。蠕 消 工 素 动化的 ,
粗纤维的测定——酸性洗涤剂发
原理
季铵盐是一种表面活性剂,在0.50mol/L H2SO4溶液样中能有效地使动物饲料、植 物样品中的蛋白质、多糖、核酸等组分水 解、湿润、乳化、分散,而纤维素和木质 素则很少变化。
回 流 装 置
主要仪器
回流装置、250mL玻璃 三角瓶上附橡皮塞及冷 凝玻璃管、1号玻璃虑 (40~50mL)、 或古氏坩埚、真空泵抽滤 装置
纤维素的性质
1.溶解性 常温下,纤维素既不溶 于水,又不溶于一般的有机溶剂 ,因此, 在常温下,它是比较稳定的,这是因为纤 维素分子之间存在氢键。 2.纤维素水解 在一定条件下,纤维 素与水发生反应。
纤维素的性质
3.纤维素氧化 纤 维素与氧化剂发生化 学反应,生成一系列 与原来纤维素结构不 同的物质,这样的反 应过程,成为纤维素 氧化。 4.柔顺性 纤维 素柔顺性很差,是刚 性的
纤维素的制法
纤维素的实验室制法是先用水、有机溶 剂处理植物原料,再用氯、亚氯酸盐、二 氧化氯、过乙酸去除其中所含的木素,得 到纤维素和半纤维素,然后采用各种方法 除去半纤维素 ,制得纯纤维素。 工业制法是用亚硫酸盐溶液或碱溶液蒸煮 植物原料,除去木素,然后经过漂白进一 步除去残留木素,所得漂白浆可用于造纸。
细菌纤维素的研究和应用新进展

细菌纤维素的研究和应用新进展纤维素是地球上最丰富的生物聚合物,主要分布于植物如树木、棉花等中,它是形成植物细胞壁的主要成分,也是形成许多真菌、藻类细胞壁的主要成分。
随着人们对纤维素类产品需求的增加,人们获取纤维素的方法正不断地改进和更新。
近年,发现一些细菌也能产生纤维素,其结构、理化特性和生化特性等皆与植物纤维素有较大的差异,与植物纤维相比,细菌纤维素(Bacterial Cellulose,BC)是由超微纤维组成的超微纤维网。
不仅是地球上除植物纤维素之外的另一类由细菌合成的天然惰性材料,而且是自1989 年Yamanaka 等[1]发现BC具有独特的功能后,以微生物作为载体,在分子水平上有高纯度、高结晶度、绿色环保的BC成为世界上公认的性能优异的新型生物学材料。
本文就BC的结构、性质、研究历史以及在生物医学材料上的应用综述如下。
1细菌纤维素的结构与特性1.1细菌纤维素的结构特点:BC是由葡萄糖分子以β-1,4糖苷键聚合而成的一种具有多孔性结构及一定纳米级孔径分布的高分子材料[2]。
早在1940 年,人们就用电镜观察到BC由独特的束状纤维组成,这种束状纤维的宽度大约为100 nm,厚度为3~8 nm,每一束由许多微纤维组成,而微纤维又与其晶状结构相关。
术醋杆菌(A.xylinum)是合成BC最强的细菌之一[3],BC的生物合成可分为聚合、分泌、组装、结晶四大过程,这四大过程是高度耦合的,并和细胞膜上的特定位点密切相关。
1.2 細菌纤维素有许多独特的性质:①强的持水性和透气性:BC是一种水不溶性的惰性支持物,有很多“孔道”,有良好的透气、透水性能。
依据合成条件的不同,它能吸收60~700倍于其干重的水份[2],未经干燥的BC的强持水性能(waterretentionvalues,wRv)值高达1000%以上,冷冻干燥后的持水能力仍超过600%。
经100℃干燥后的BC在水中的再溶胀能力与棉短绒相当,即有非凡的持水性,并具有高湿强度[4];②高化学纯度和高结晶度:BC是一种“纯纤维素”,以100%纤维素的形式存在,不含半纤维素、木质素、果胶和其他细胞壁成分,结构单一,提纯过程简单;③较高的生物适应性和生物可降解性:Helenius等[5]开展了BC植入小鼠皮下组织的生物适应性研究及Klenm等[6]用BC微管材料取代老鼠颈动脉的研究都表明BC与老鼠身体没有任何排斥反应。
纤维素酶经典综述
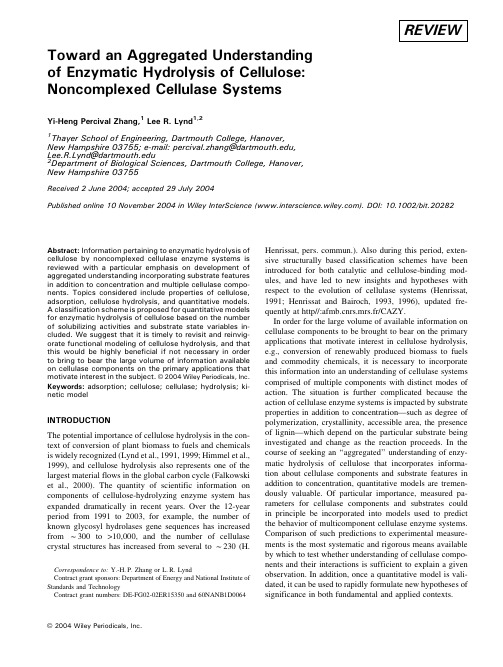
Toward an Aggregated Understanding of Enzymatic Hydrolysis of Cellulose:Noncomplexed Cellulase SystemsYi-Heng Percival Zhang,1Lee R.Lynd 1,21Thayer School of Engineering,Dartmouth College,Hanover,New Hampshire 03755;e-mail:percival.zhang @,Lee.R.Lynd @ 2Department of Biological Sciences,Dartmouth College,Hanover,New Hampshire 03755Received 2June 2004;accepted 29July 2004Published online 10November 2004in Wiley InterScience ().DOI:10.1002/bit.20282Abstract:Information pertaining to enzymatic hydrolysis of cellulose by noncomplexed cellulase enzyme systems is reviewed with a particular emphasis on development of aggregated understanding incorporating substrate features in addition to concentration and multiple cellulase compo-nents.Topics considered include properties of cellulose,adsorption,cellulose hydrolysis,and quantitative models.A classification scheme is proposed for quantitative models for enzymatic hydrolysis of cellulose based on the number of solubilizing activities and substrate state variables in-cluded.We suggest that it is timely to revisit and reinvig-orate functional modeling of cellulose hydrolysis,and that this would be highly beneficial if not necessary in order to bring to bear the large volume of information available on cellulase components on the primary applications that motivate interest in the subject.B 2004Wiley Periodicals,Inc.Keywords:adsorption;cellulose;cellulase;hydrolysis;ki-netic modelINTRODUCTIONThe potential importance of cellulose hydrolysis in the con-text of conversion of plant biomass to fuels and chemicals is widely recognized (Lynd et al.,1991,1999;Himmel et al.,1999),and cellulose hydrolysis also represents one of the largest material flows in the global carbon cycle (Falkowski et al.,2000).The quantity of scientific information on components of cellulose-hydrolyzing enzyme system has expanded dramatically in recent years.Over the 12-year period from 1991to 2003,for example,the number of known glycosyl hydrolases gene sequences has increased from f 300to >10,000,and the number of cellulase crystal structures has increased from several to f 230(H.Henrissat,mun.).Also during this period,exten-sive structurally based classification schemes have been introduced for both catalytic and cellulose-binding mod-ules,and have led to new insights and hypotheses with respect to the evolution of cellulase systems (Henrissat,1991;Henrissat and Bairoch,1993,1996),updated fre-quently at http//:rs.mrs.fr/CAZY.In order for the large volume of available information on cellulase components to be brought to bear on the primary applications that motivate interest in cellulose hydrolysis,e.g.,conversion of renewably produced biomass to fuels and commodity chemicals,it is necessary to incorporate this information into an understanding of cellulase systems comprised of multiple components with distinct modes of action.The situation is further complicated because the action of cellulase enzyme systems is impacted by substrate properties in addition to concentration—such as degree of polymerization,crystallinity,accessible area,the presence of lignin—which depend on the particular substrate being investigated and change as the reaction proceeds.In the course of seeking an ‘‘aggregated’’understanding of enzy-matic hydrolysis of cellulose that incorporates informa-tion about cellulase components and substrate features in addition to concentration,quantitative models are tremen-dously valuable.Of particular importance,measured pa-rameters for cellulase components and substrates could in principle be incorporated into models used to predict the behavior of multicomponent cellulase enzyme parison of such predictions to experimental measure-ments is the most systematic and rigorous means available by which to test whether understanding of cellulase compo-nents and their interactions is sufficient to explain a given observation.In addition,once a quantitative model is vali-dated,it can be used to rapidly formulate new hypotheses of significance in both fundamental and applied contexts.B 2004Wiley Periodicals,Inc.Correspondence to:Y.-H.P.Zhang or L.R.LyndContract grant sponsors:Department of Energy and National Institute of Standards and TechnologyContract grant numbers:DE-FG02-02ER15350and 60NANB1D0064REVIEWThis article reviews available information on enzymatic hydrolysis by noncomplexed cellulase systems;that is,sys-tems based on components that act discretely rather than as stable complexes(Lamed et al.,1983;Tomme et al., 1995a).A considerable portion of this review is spent on the properties of cellulose in light of the central role such properties play in mechanistically based quantitative models of cellulose hydrolysis.In particular,the following section considers crystallinity,degree of polymerization, accessibility,preparation and properties of model sub-strates,and pretreated lignocellulosic materials.The section Cellulase Adsorption is devoted to adsorption leading to the formation of cellulose–cellulase complexes,including ad-sorption models,reversibility,and enzyme mobility,as well as inferred accessibility of cellulose from cellulase adsorp-tion.Thereafter,mechanistic understanding of cellulose hydrolysis by noncomplexed systems is addressed in Cellulose Hydrolysis,with attention given to concep-tual understanding of cellulose hydrolysis,features of the widely studied Trichoderma reesei cellulase system,docu-mentation and understanding of synergism among cellu-lase components,and a summary of current mechanistic understanding.The section Quantitative Models presents a classification scheme and summarizes features of mod-els reported in the literature.The final section offers con-cluding perspectives and outlines outstanding challenges associated with understanding and modeling noncomplexed cellulase systems.Since our primary focus is on the function of cellulases rather than their structure,we use the older, functionally defined nomenclature rather than the newer nomenclature based on amino-acid sequence and molecu-lar structure.CELLULOSEAll cellulose is produced biosynthetically.While cellulose production by photosynthetic higher plants and algae is thought to be by far the most important in terms of global carbon flows,cellulose production by nonphotosynthetic organisms(certain bacteria,marine invertebrates,fungi, slime molds and amoebae)has also been documented (Coughlan,1985;Jarvis,2003;Lynd et al.,2002;Tomme et al.,1995a).Cellulose is a linear condensation polymer consisting of D-anhydroglucopyranose joined together by h-1,4-glycosidic bonds.Anhydrocellobiose is the repeating unit of cellulose,since adjacent anhydroglucose mole-cules are rotated180j with respect to their neighbors (Fig.1a).This rotation causes cellulose to be highly sym-metrical,since each side of the chain has an equal number of hydroxyl groups.Coupling of adjacent cellulose mol-ecules by hydrogen bonds and van der Waal’s forces re-sults in a parallel alignment and a crystalline structure. The extensive hydrogen bonds of interchain(2per anhy-droglucopyranose)and intrachain(2f3per anhydrogluco-pyranose)produces straight,stable supramolecular fibers of great tensile strength(Gardner and Blackwell,1974a,b;Krassig,1993;Nevell and Zeronian,1985).In contrast, starch contains amylose and amylopectin connected by a-1,4and to some extent a-1,6glucosidic bonds,forming a tightly coiled helical structure maintained by interchain hydrogen bonds(Buleon et al.,1998;Calvert,1997).Na-tive cellulose,referred to as cellulose I,has two distinct crystallite forms,I a,which is dominant in bacterial and algal cellulose,and I h,which is dominant in higher plants (Atalla and Vanderhart,1984).Native cellulose(cellulose I) can be converted to other crystalline forms(II–IV)by var-ious treatments(Klein and Snodgrass,1993;Krassig,1993; O’Sullivan,1997).Cellulose exist as sheets of glucopyranose rings lying in a plane with successive sheets stacked on top of each other to form a three-dimensional particle.Because of this ar-rangement,the surface of a cellulose particle has distinct ‘‘faces’’that interact with the aqueous environment and cellulase enzymes.The six carbons in the glucopyranose ring and internal h-glucosidic bonds lie in the ab plane or ‘‘110’’face,whereas the ac plane or11¯0face consists of the edges of rings(see Fig.1b).Additional faces present reducing and nonreducing ends,respectively.The repeating unit of the110face is the cellobiose lattice,which mea-sures1.04nm along the axis of the cellulose molecule and 0.54nm in the perpendicular direction.About100cellu-lose glucans are aggregated into elementary fibrils with a crystalline width of4–5nm(O’Sullivan,1997),and bunches of elementary fibrils are embedded in a matrix of hemicellulose with a thickness of7–30nm.The lignifica-tion process occurs late in the process of synthesizing nat-ural fibers,so lignin is located primarily on the exterior of microfibrils where it covalently bonds to hemicellulose (Fig.1c;Klein and Snodgrass,1993).The relationship between structural features of cellu-lose and rates of enzymatic hydrolysis has been the subject of extensive study and several reviews(Converse,1993; Cowling and Kirk,1976;Lynd et al.,2002;Mansfield et al., 1999;McMillian,1994),but is still incompletely under-stood.Structural features of cellulose commonly considered as rate-impacting factors include crystallinity index,degree of polymerization,and accessible area.Crystallinity Index(CrI)Crystallinity has often been thought of as providing an indication of substrate reactivity,and is prominently fea-tured in the model of Wood(1975)as well as other models. The crystallinity of dried cellulose samples can be quan-titatively measured from the wide-range X-ray diffraction pattern(Krassig,1993).In the case of cellulose-I,the crys-tallinity index(CrI)is calculated using the formula:CrI¼1Àh am=h cr¼1Àh am=ðh totÀh amÞð1Þbased on the ratio of the height of crystalline cellulose in the002reflection at2u=22.5j(h cr)to the height of amorphous cellulose(h am),and h tot=h cr+h am.Cotton798BIOTECHNOLOGY AND BIOENGINEERING,VOL.88,NO.7,DECEMBER30,2004(Hoshino et al.,1997;Lee et al.,1982;Sinitsyn et al.,1991), bacterial cellulose from Aacetobacter xylinum(Boisset et al.,1999;Gilkes et al.,1992;Valjamae et al.,1999),and cellulose from the alga Valonia ventricosa(Boisset et al.,1999;Fierobe et al.,2002)provide examples of highly crystalline cellulose,while phosphoric acid swollen cellu-lose and ball-milled cellulose are regarded as amorphous cellulose(Hoshino et al.,1997;Lee et al.,1982;Ooshima Figure1.a:Structure of cellulose featuring repeating h1,4-linked anhydrocellobiose units.b:Cellulose I crystal.The axes of the repeating unit (cellobiose)are:a=0.817nm,b=1.04nm,and c=0.786nm.The faces of the glucopyranose rings are parallel to the ab plane(110face)of the crystal (Mosier et al.,1999).c:Organization of lignocellulose origanization into elementary fibrils and microfibrils(Klein and Snodgrass,1993).ZHANG AND LYND:NONCOMPLEXED CELLULASE SYSTEMS799et al.,1983).Common model substrates derived from bleached commercial wood pulps,such as Avicel(Wood and Bhat,1988;Wood,1988),filter paper(Henrissat et al., 1985),and Solka Floc(Bertrain and Dale,1985;Fan et al., 1980;Lee et al.,1982;Sinitsyn et al.,1991)are regarded as a blend of amorphous and crystalline forms(Gilkes et al., 1991).Typical values of CrI for various model cellulosic substrates are presented in Table I.The CrI value of cel-lulose increases after a period of water swelling due to re-crystallization(Fan et al.,1980;Lee et al.,1983;Fengel and Wegener,1984),and the variations in drying condition prior to measurement of CrI may cause differences between substrates arising from the method of substrate preparation rather than properties of the substrate per se(Lenze et al., 1990;Weimer et al.,1995).The presence of residual cells and proteins can also result in artifacts in the CrI assay (Converse,1993).Cellulose hydrolysis rates mediated by fungal cellulases are typically3–30times faster for amorphous cellulose as compared to high crystalline cellulose(Lynd et al.,2002; Table III).This observation led investigators in the1980s to postulate a model for cellulose structure consisting of amorphous and crystalline fractions(Fan et al.,1980,1981; Lee et al.,1983).If this hypothesis were correct,it would be expected that crystallinity should increase over the course of cellulose hydrolysis as a result of preferential reaction of amorphous cellulose(Betrabet and Paralikar, 1977;Ooshima et al.,1983).However,several studies have found that crystallinity does not increase during enzymatic hydrolysis(Lenze et al.,1990;Ohmine et al.,1983;Puls and Wood,1991;Schurz et al.,1985;Sinitsyn et al.,1989).Con-sidering both the uncertainty of methodologies for mea-suring CrI as well as conflicting results on the change of CrI during hydrolysis,it is difficult to conclude at this time that CrI is a key determinant of the rate of enzymatic hy-drolysis(Lynd et al.,2002;Mansfield et al.,1999). Future studies aimed at developing and applying im-proved methods would be useful to more definitively re-solve the role of CrI in impacting hydrolysis.In interpreting crystallinity data,and indeed data for all cellulose physical properties,care must be taken to distinguish correlation from cause and effect.For example,several treatments that decrease crystallinity also increase surface area,and it has been suggested that the increased hydrolysis rates observed with substrates arising from such treatments may be due to increasing adsorptive capacity rather than substrate reac-tivity(Caulfield and Moore,1974;Howell and Stuck,1975; Lee and Fan,1982).Comparing the hydrolysis rates on various sources of model cellulosic substrates,Fierobe et al. (2002)concluded that accessibility of cellulose is a more important factor than crystallinity index in determining the hydrolysis rate.Degree of PolymerizationThe degree of polymerization(DP)of cellulosic substrates determines the relative abundance of terminal and interior h-glucosidic bonds,and of substrates for exo-acting and endo-acting enzymes,respectively.DP may be defined in terms of the number average DP(DP N),weight average DP (DP W),or DP inferred from viscosity(DP V):DP N¼M nMW glu¼P N i M i P N i=MW gluð2ÞDP W¼M WMW glu¼P N i M2i P N i=MW gluð3ÞDP V¼M VMW glu¼P N i D P N i=MW gluð4Þwhere N i is the number of moles of a given fraction i having molar mass M i,M N is the number-average molecular weight,M w is the weight-average molecular weight,M V is the viscosity-average molecular weight,MW glu is the molecular weight of anhydroglucose(162g/mol),and D is viscosity.Measurement of DP begins with dissolution of cellulose using a technique that does not alter chain length. Several such methods appear satisfactory,including:1) metal complex solutions such as Cuam solution(Klemm et al.,1998)and cupriethylenediamine(Klemen-Leyer et al., 1992,1994,1996);2)forming cellulose derivatives by reacting with organic solvents(Ng and Zeikus,1980)or inorganic acids such as nitric acid(Whitaker,1957);and3) ionic solutions such as N,N-dimethylacetamide(DMAc)/ LiCl(Striegel,1997).After dissolution,DP N can be mea-sured by membrane or vapor pressure osmometry,cry-oscopy,ebullioscopy,determination of reducing end con-centration,or electron microscopy(Krassig,1993).DP W can be measured based on light scattering,sedimentation equilibrium,and X-ray small angle scattering,and DP V is measured based on viscosity.The viscosity of dissolved cellulose or cellulose derivatives has been found to equal:D¼K m M aþ1ið5ÞTable I.Summary of some physical properties of model cellulosicsubstrates.Substrate1CrI2SSA2(m2/g)DP N2F RE(%)Avicel0.5–0.6203000.33BC0.76–0.9520020000.05PASC0–0.04240100 1.0Cotton0.81–0.95na.1000–30000.1–0.033Filter Paper–0.45na.7500.13Wood pulp0.5–0.761–55500–15000.06–0.21BC,bacterial cellulose;PASC,phosphoric acid swollen cellulose;CrIdenotes crystallinity index;SSA denotes specific surface area by BET;DP N denotes the number-average degree of polymerization;F RE denotesthe fraction of reducing ends.2References in text.800BIOTECHNOLOGY AND BIOENGINEERING,VOL.88,NO.7,DECEMBER30,2004in which K m =constant,with the value of a for cellulose and cellulose derivatives in most cases ranging from 0.75to 1(Krassig,1993).Therefore,DP V can be written as:DP V ¼PN i M 1:75À2i P N i=MW glu ð6ÞSince cellulose is polydisperse,DP W z DP V >DP N .The DP N values are adequate in dealing with cellulose hydrol-ysis,and DP W and DP V frequently show a good correlation to polymer properties (Klemm et al.,1998;Krassig,1993).The distribution of DPs among a population of cellulose molecules can be measured by size exclusion chromatog-raphy (Yau et al.,1979).The reciprocal of DP corresponds to the fraction of reducing ends relative to all glucan units present (F NR ,unitless).Cellulose solubility decreases drastically with increasing DP due to intermolecular hydrogen bonds.Cellodextrins with DP from 2–6are soluble in water (Klemm et al.,1998;Miller,1963;Pereira et al.,1988),while cellodextrins from 7–13or longer are somewhat soluble in hot water (Zhang and Lynd,2003;Schmid et al.,1988).A glucan of DP =30already represents the polymer ‘‘cellulose’’in its structure and properties (Klemm et al.,1998).The DP of cellulosic substrates varies greatly,from <100to >15,000,depending on substrate origin and preparation,as shown in Figure 2.The DP of wood after pulping is reduced to 500–1,500(Bertrain and Dale,1985;Klein and Snodgrass,1993;Lee et al.,1982;Swatloski et al.,2002).After partial acid hydrolysis,the DP of Avicel is further decreased to 130–800(Hoshino et al.,1997;Ng and Zeikus,1980;Ross-Murphy,1985;Steiner et al.,1988;Wood,1985),depending on hydrolysis conditions (Dong et al.,1998)and the DP of the original substrate (Wood,1988).Similarly,the DP of natural cotton can be as high as 15,000,but is reduced to 1,000–3,000or less in the preparation of cotton linters involving treatment to accomplish dewaxing and whitening (Kleman-Leyer et al.,1992,1996;Okazaki and Moo-Young,1978;Ryu and Lee,1982),and filter paper made from cotton pulp has a DP of 500–1,000or higher (Nisizawa,1973;Kongruang et al.,2004).Bacterial cel-lulose (BC)has an average DP of 2,000–3,000(Hestrin,1963;Fierobe et al.,2002;Valjamae et al.,1999),while bacterial microcrystalline cellulose (BMCC)prepared by treatment of BC with acids ranges from 130–1,300,de-pending on hydrolysis conditions (Valjamae et al.,1999).The DP of phosphoric-acid swollen cellulose (PASC)ranges from 30to more than 1,000(Fan et al.,1980;Krassig,1985;Petre et al.,1981;Wood and McCrae,1972),depending on the DP of the starting substrate (Wood,1988;Hoshino et al.,1997),as well as the phosphoric acid incubation time and temperature (Krassig,1993).The change in DP over the course of hydrolysis for cellulosic substrates is determined by the relative propor-tion of exo-and endo-acting activities and cellulose proper-ties.Exoglucanases act on chain ends,and thus decrease DP only incrementally (Kleman-Leyer et al.,1992,1996;Srisodsuk et al.,1998).Endoglucanases act on interior portions of the chain and thus rapidly decrease DP (Kleman-Leyer et al.,1992,1994;Selby,1961;Srisodsuk et al.,1998;Whitaker,1957;Wood and McCrae,1978).Exoglucanase has been found to have a marked preference for substrates with lower DP (Wood,1975),as would be expected given the greater availability of chain ends with decreasing DP.It is well known that endoglucanase activity leads to an increase in chain ends without resulting in appreciable solubilization (Irwin et al.,1993;Kruus et al.,1995;Re-verbel-Leroy et al.,1997).We know of no indication in the literature that the rate of chain end creation by endogluca-nase is impacted by substrate DP.AccessibilityCellulase enzymes must bind to the surface of substrate particles before hydrolysis of insoluble cellulose can take place.The 3D structure of such particles (including micro-structure)in combination with the size and shape of the cellulase enzyme(s)under consideration determine whether h -glucosidic bonds are or are not accessible to enzymatic attack.Cellulosic particles have both external and internal surfaces.In general,the internal surface area of cellulose is 1–2orders higher than the external surface area (Chang et al.,1981),but this is not always the case,for example,in the case of bacterial cellulose.The internal surface area can be measured by small angle X-ray scattering (SAXS),mer-cury porosimetry,water vapor sorption,and size exclusion (Grethlein,1985;Neuman and Walker,1992;Stone etal.,Figure 2.Typical DP values of cellulose and soluble cellodextrins.NC,natural cotton;NW,natural wood;P,pulp;CT,cotton linter;FP,filter paper.ZHANG AND LYND:NONCOMPLEXED CELLULASE SYSTEMS 8011969).The internal surface area of porous cellulose particles depends on the capillary structure and includes intrapar-ticulate pores(1–10nm)as well as interparticulate voids (>5A m)(Marshall and Sixsmith,1974).Grethlein(1985) found linear correlations between the initial hydrolysis rate of pretreated biomass and the pore size accessible to a molecule with a diameter of51A˚,similar to the size of T.reesei cellulase components.But the surface exposed to dextran cannot distinguish the specific active cellulose sur-face area at which enzymatic hydrolysis occurs from the surface area which is not a site for enzymatic attack(Chanzy et al.,1984;Gilkes et al.,1992;Lehtio et al.,2003),re-sulting in potential overestimation of effective cellulase-accessible area.Techniques for measuring internal surface generally do not estimate external area(Converse,1993). External surface area is closely related to shape and par-ticle size,and can be estimated by microscopic observation (Gilkes et al.,1992;Henrissat et al.,1988;Reinikainen et al., 1995b;Weimer et al.,1990;White and Brown,1981).For example,the external surface area of BMCC is f115m2/g (Gilkes et al.,1992)whereas that of Avicel is f0.3m2/g (Weimer et al.,1990).Increasing cellulase adsorption and cellulose reactivity with decreasing particle size has been reported(Kim et al.,1992;Mandels et al.,1971).However, this may be due to causes other than increased external area, perhaps decreasing mass transfer resistance,since external surface is thought to be a small fraction of overall surface area for most substrates.The gross cellulose accessibility is generally measured by the sorption of nitrogen,argon or water vapor,dimensional change or weight gain by swelling in water or organic liquids,and exchange of H to D atoms with D2H.The most widely used procedure for specific surface area(SSA)is the Brunauer-Emmett-Teller(BET)method using nitrogen adsorption.Due to variations in the experimental condi-tions such as adsorption time,vacuum time and vacuum pressure(Marshall and Sixsmith,1974),sample prepara-tion(Grethlein,1985;Lee et al.,1983),and sample origin and features(Marshall and Sixsmith,1974;Weimer et al., 1990),a wide range of gross area values have been reported in the literature even for the same substrate.The specific area of Avicel PH102increases from5.4m2/g surface area to18m2/g after a long time of water swelling,because the capillary structure of air-dried cellulose from the water-swollen state collapses,resulting in drastic changes in phys-ical parameters(Grethlein,1985;Lee et al.,1983).To keep substrate capillary structure as it exists in the hydrated state,it is recommended that SSA be measured using solvent-dried samples(Grethlein,1985;Lee et al.,1983). The typical SSA of BMCC,Avicel,and wet pulp are f200m2/g BMCC(Bothwell et al.,1997),1.8–22m2/g Avicel(Fan et al.,1980;Lee et al.,1983;Marshall and Sixsmith,1974),and55–61m2/g pulp(Fan et al.,1980; Kyriacou et al.,1988).The specific surface area of PASC from Solka Floc increases from19.5to239m2/g when phosphoric acid concentration increases from75%to85% (Lee et al.,1982).Because a nitrogen molecule is much smaller than cellulase,it has access to pores and cavities on the fiber surface that cellulase cannot enter.Therefore, there is limited basis to infer that SSA measured using the BET method is a key determinant of enzymatic hydrolysis rate(Mansfield et al.,1999).Preparation and Properties of Model Substrates Wood pulp is made from wood using several steps,in-cluding shredding,delignification,bleaching,and washing (Klemm et al.,1998).For example,Solka Floc is made from SO2-bleached spruce pulp by ball milling(Ghose, 1969).Avicel,also called hydrocellulose and microcrystal-line cellulose,is prepared from cellulosic fibers(wood pulp) by partial acid hydrolysis and then spray drying of the washed pulp slurry,but microcystalline cellulose(Avicel) still contains a substantial amount(f30–50%)of amor-phous cellulose(Krassig,1993).Bacterial cellulose(BC) is prepared from the pellicle produced by Acetobacter xylinum(ATCC23769)(Hestrin,1963)or from Nata de Coco(Daiwa Fine Produces,Singapore;Boisset et al., 2000).Bacterial microcrystalline cellulose(BMCC)is pre-pared from BC by partial acid hydrolysis to remove amor-phous cellulose(Valjamae et al.,1999).Cotton cellulose is made from natural cotton after removing impurities such as wax,pectin,and colored matter(Corbett,1963). Whatman No.1filter paper is made from cotton pulp (Dong et al.,1998).Homogenous amorphous cellulose can be made from various pure cellulose powders,e.g.,Avicel, cotton linters,by swelling treatments such as phosphoric acid,alkali,DMSO,DMAc/LiCl.Phosphoric acid swol-len cellulose(PASC)is most commonly made by swelling cellulose powder using concentrated phosphoric acid,re-sulting in decreased crystallinity(Wood,1988).Typical values for CrI,DP,gross surface area values(SSA by BET), and fraction of reducing ends(F NR,reciprocal of DP)for model cellulosic substrates are presented in Table I. Characteristics of Pretreated LignocelluloseNatural cellulose molecules occur in elementary fibrils closely associated with hemicellulose and other structural polysaccharides as well as lignin(Fig.1c).Such ligno-cellulose typically contains cellulose(35–50wt.%),hemi-cellulose(20–35wt.%),and lignin(5–30wt.%)(Chang et al.,1981;Klein and Snodgrass,1993;Lynd et al.,2002; Mansfield et al.,1999).A detailed consideration of en-zymatic hydrolysis of native lignocellulose may be found elsewhere(Hatfield et al.,1999).Since enzymatic hydro-lysis of native lignocellulose usually results in solubiliza-tion of V20%of the originally present glucan,some form of pretreatment to increase amenability to enzymatic hy-drolysis is included in most process concepts for biological conversion of lignocellulose.Pretreatment,under appro-priate conditions,retains nearly all of the cellulose pres-ent in the original material and allows close to theoretical yields upon enzymatic hydrolysis.Proposed pretreatment802BIOTECHNOLOGY AND BIOENGINEERING,VOL.88,NO.7,DECEMBER30,2004processes include dilute acid,steam explosion at high solid concentration,‘‘hydrothermal’’process,‘‘organosolv’’pro-cesses involving organic acid solvents in an aqueous phase, ammonia fiber explosion(AFEX),strong alkali process (Lynd et al.,2002),as well as mechanical treatments such as hammer and ball milling(Millett et al.,1976;Sun and Cheng,2002).Comparative features of these processes as well as consideration of substrate factors impacting the hy-drolysis rate are reviewed elsewhere(Chang et al.,1981; Converse,1993;Cowling and Kirk,1976;Dale,1985;Hsu, 1996;Ladisch et al.,1983;Mansfield et al.,1999;McMillian 1994;Lynd,1996;Sun and Cheng,2002;Weil et al.,1994; Wood and Saddler,1988).Hydrolysis of lignocellulosic biomass is more compli-cated than that of pure cellulose due to the presence of nonglucan components such as lignin and hemicellulose. Lignin removal and/or redistribution are thought to have a significant effect on observed rates of enzymatic hydrolysis (Chernoglazov et al.,1988;Converse,1993;Lynd et al., 2002).Lignin has been implicated as a competitive cel-lulase adsorbent which reduces the amount of cellulase available to catalyze cellulose hydrolysis(Bernardez et al., 1993;Ooshima et al.,1990;Sutcliffe and Saddler,1986). In addition,it has been suggested that residual lignin blocks the progress of cellulase down the cellulose chain(Eriksson et al.,2002;Mansfield et al.,1999).The measured crystallinity index of lignocellulose is impacted by the presence of lignin and hemicellulose.Thus, care must be taken in comparing CrI values for lignocellu-losic substrates to values for cellulosic substrates,and also in comparing the CrI of lignocellulosic substrates before and after pretreatment.Reported CrI values for pretreated materials are generally in the range of0.4–0.7(Chang and Holtzapple,2000;Gharpuray et al.,1983;Koullas et al., 1992;Sinitsyn et al.,1989,1991).Pretreatment by either dilute-acid or steam explosion under conditions that are quite effective in enhancing hydrolysis has been found to increase the composite CrI of lignocellulose(Deschamps et al.,1996;Kim et al.,2003;Knappert et al.,1980; Meunier-Goddik et al.,1999).Consistent with this,a negative correlation between hydrolysis rate and CrI has been shown in experiments that involved chemical pre-treatments followed by ball milling(Chang and Holtzapple, 2000;Gharpuray et al.,1983;Knappert et al.,1980;Koullas et al.,1992;Sinitsyn et al.,1989,1991),and also ex-periments that examined various pretreatment conditions (Chang and Holtzapple,2000).In contrast to the trend ob-served for other pretreatment processes,AFEX pretreat-ment has been reported to result in a decrease in CrI (Gollapalli et al.,2002).Several investigators have impli-cated accessible surface area as an important factor in determining the effectiveness of pretreatment(Gharpuray et al.,1983;Grethlein,1985;Grethlein and Converse,1991; Sinitsyn et al.,1991).A significant difficulty in interpreting the effects of pretreatment at a mechanistic level is that exposure of substrates to conditions that cause one poten-tial determinant of reactivity to change usually bring about changes in other such potential determinants.For example, Sinitsyn et al.(1991)found a strong negative correlation between CrI and accessible surface area accompanying several pretreatment processes.We suspect that the impact of increased surface area accompanying pretreatment may in many cases be more important than changes in CrI,al-though further work will be needed to establish this point and the relative significance of these and other factors may well be different for different processes.DP values of lignocellulosic substrates such as ba-gasse,wheat straw,and Eucalyptus regnans pretreated using steam explosion,supercritical CO2,alkali,and ozone mostly fall in the range of600–1,100,although values as high as3,000have been recorded for Pinus radiata chips (Puri,1984;Sinitsyn et al.,1991).During dilute acid-catalyzed cellulose hydrolysis,the DP of cellulosic ma-terials decreases rapidly initially and achieves a nearly constant value thereafter called the level-off DP(LODP) (Klemm et al.,1998;Krassig,1993;Wood,1988).LODP values in the range of100–300have been measured,de-pending on the substrate and conditions such as temperature and acid concentration(Krassig,1993;Wood,1988).This LODP value may limit the rates of hydrolysis that can oc-cur with dilute acid pretreated lignocellulose,although this has not been investigated experimentally.Different con-clusions about the importance of DP in determining hydrolysis rates of pretreated cellulosic biomass have been drawn,with Sinitsyn et al.(1991)concluding that DP is relatively unimportant,but Puri(1984)concluding that it is quite important.CELLULASE ADSORPTIONAdsorptionCellulase adsorption is rapid compared to the time re-quired for hydrolysis,with many studies finding that ad-sorption reaches steady-state within half an hour(Lynd et al.,2002).The most common description of cellulase adsorption is the Langmuir isotherm(Eq.[7]),derived as-suming that adsorption can be described by a single ad-sorption equilibrium constant and a specified adsorption capacity.The Langmuir isotherm may be represented as:E a¼W max K P E f1þK P E fð7Þin which E a is adsorbed cellulase(mg or A mol cellulase/L), W max is the maximum cellulase adsorption=A max*S(mg or A mol cellulase/L),A max is the maximum cellulase adsorp-tion per g cellulose(mg or A mol cellulase/g cellulose),S is cellulose concentration(g cellulose/L),E f is free cellulase (mg or A mol cellulase/L),and K P is the dissociation constant (K P¼E a f)in terms of L/g cellulose.The distribution coef-ficient or partition coefficient,R,is defined as:R¼K P W maxð8ÞZHANG AND LYND:NONCOMPLEXED CELLULASE SYSTEMS803。
木质纤维素预处理方法研究进展综述
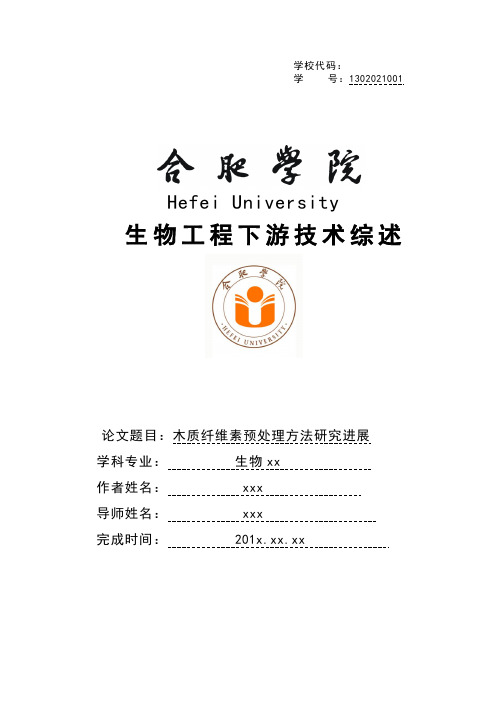
综上所述,利用二氧化钛和光催化处理技术,可减少木质素在氧化过程中的碳损耗,较好地保留了木质素中的苯环结构,是一种有效降解木质素的新方法。
5.3电晕放电法
芬兰的E.Koivula等人[11]为了有效地回收天然木质素,用不同的预处理方法,比较了木材水解产物中的杂质对后续过滤材料的影响。实验发现,利用脉冲电晕放电的氧化方法处理后,可提升过滤膜的过滤效率,对于过滤膜来讲,其流速提高了6倍。其他的前处理方法,也会影响过滤体系对木质素的过滤效果,比如添加活性炭吸附剂以及调节体系pH值也可提高过滤效率。该研究表明,针对木材水解产物中的各种化学结构,综合利用如电晕放电等前处理手段,可获得较高的木质素回收率。
木质纤维素材料经过预处理后,纤维素水解效率的差异取决于预处理方式的选择、所处理物料的特性、纤维素酶用量及酶系的组成。
预处理强度对纤维素的水解效率有着显著的影响,为了获得较好的纤维素水解效率,需要适当提高处理强度,但是强度的提高会增加能量的消耗,同时也会降低糖的回收率,因此需要在纤维素的水解效率与能量消耗及糖回收率之间找到平衡点[6]。
5.2光催化法
处理木质素的手段并不局限于传统的诸如热水处理、酸处理和碱处理等方法。将光化学技术应用于木质素,是绿色化学在化学工艺进步方面的体现。因为光化学所使用的绝大部分能量可用绿色能源来替代,因此该技术结合木质素这种传统工业废料的回收利用,可进一步对环境保护产生深V手段预处理水稻秸秆,并用酶把秸秆中的纤维素转化成乙醇,再用测定酶降解后所获得的葡萄糖数量表征木质素降解的程度。该方法可以避免传统降解过程中不可回避的高温高压以及其他强烈腐蚀条件,是一种高效、绿色环保的秸秆预处理方式,为绿色化学下的木质素处理,探索了一条值得研究的路线。
纤维素溶解现状研究
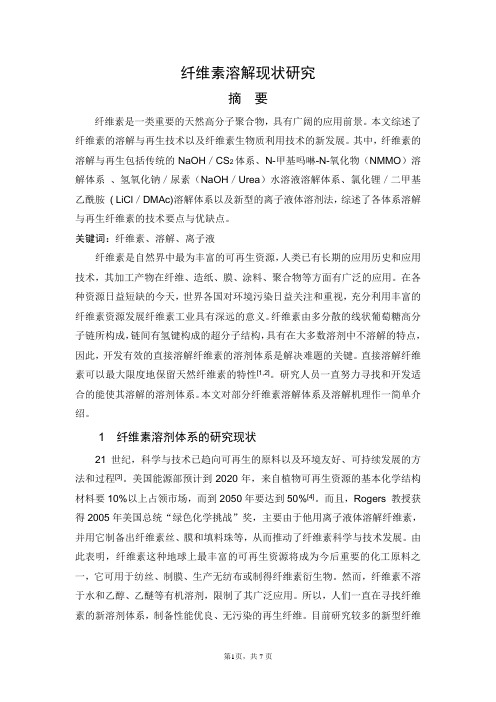
纤维素溶解现状研究摘要纤维素是一类重要的天然高分子聚合物,具有广阔的应用前景。
本文综述了纤维素的溶解与再生技术以及纤维素生物质利用技术的新发展。
其中,纤维素的溶解与再生包括传统的NaOH/CS2体系、N-甲基吗啉-N-氧化物(NMMO)溶解体系、氢氧化钠/尿素(NaOH/Urea)水溶液溶解体系、氯化锂/二甲基乙酰胺( LiCl/DMAc)溶解体系以及新型的离子液体溶剂法,综述了各体系溶解与再生纤维素的技术要点与优缺点。
关键词:纤维素、溶解、离子液纤维素是自然界中最为丰富的可再生资源,人类已有长期的应用历史和应用技术,其加工产物在纤维、造纸、膜、涂料、聚合物等方面有广泛的应用。
在各种资源日益短缺的今天,世界各国对环境污染日益关注和重视,充分利用丰富的纤维素资源发展纤维素工业具有深远的意义。
纤维素由多分散的线状葡萄糖高分子链所构成,链间有氢键构成的超分子结构,具有在大多数溶剂中不溶解的特点,因此,开发有效的直接溶解纤维素的溶剂体系是解决难题的关键。
直接溶解纤维素可以最大限度地保留天然纤维素的特性[1,2]。
研究人员一直努力寻找和开发适合的能使其溶解的溶剂体系。
本文对部分纤维素溶解体系及溶解机理作一简单介绍。
1 纤维素溶剂体系的研究现状21世纪,科学与技术已趋向可再生的原料以及环境友好、可持续发展的方法和过程[3]。
美国能源部预计到2020年,来自植物可再生资源的基本化学结构材料要10%以上占领市场,而到2050年要达到50%[4]。
而且,Rogers 教授获得2005年美国总统“绿色化学挑战”奖,主要由于他用离子液体溶解纤维素,并用它制备出纤维素丝、膜和填料珠等,从而推动了纤维素科学与技术发展。
由此表明,纤维素这种地球上最丰富的可再生资源将成为今后重要的化工原料之一,它可用于纺丝、制膜、生产无纺布或制得纤维素衍生物。
然而,纤维素不溶于水和乙醇、乙醚等有机溶剂,限制了其广泛应用。
所以,人们一直在寻找纤维素的新溶剂体系,制备性能优良、无污染的再生纤维。
纤维素改性研究进展
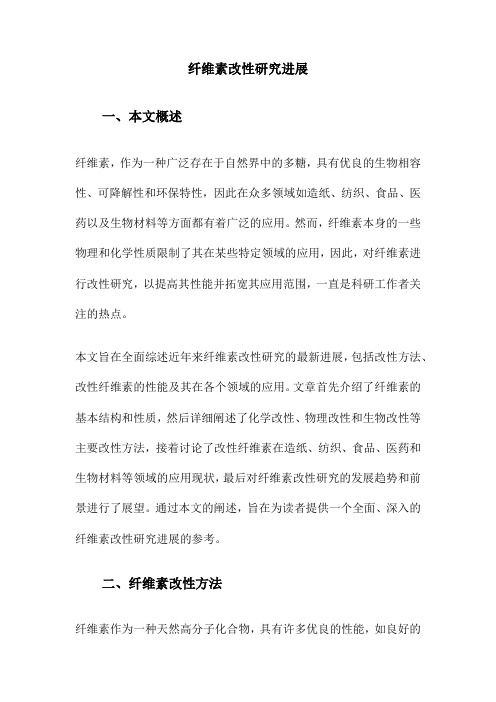
纤维素改性研究进展一、本文概述纤维素,作为一种广泛存在于自然界中的多糖,具有优良的生物相容性、可降解性和环保特性,因此在众多领域如造纸、纺织、食品、医药以及生物材料等方面都有着广泛的应用。
然而,纤维素本身的一些物理和化学性质限制了其在某些特定领域的应用,因此,对纤维素进行改性研究,以提高其性能并拓宽其应用范围,一直是科研工作者关注的热点。
本文旨在全面综述近年来纤维素改性研究的最新进展,包括改性方法、改性纤维素的性能及其在各个领域的应用。
文章首先介绍了纤维素的基本结构和性质,然后详细阐述了化学改性、物理改性和生物改性等主要改性方法,接着讨论了改性纤维素在造纸、纺织、食品、医药和生物材料等领域的应用现状,最后对纤维素改性研究的发展趋势和前景进行了展望。
通过本文的阐述,旨在为读者提供一个全面、深入的纤维素改性研究进展的参考。
二、纤维素改性方法纤维素作为一种天然高分子化合物,具有许多优良的性能,如良好的生物相容性、可降解性和环境友好性等。
然而,其固有的物理和化学性质,如亲水性、结晶性和热稳定性,限制了其在某些领域的应用。
因此,通过改性方法提高纤维素的性能,拓宽其应用范围,一直是科研领域的热点课题。
物理改性是一种简单而有效的改变纤维素性能的方法。
通过热处理、机械处理或高能辐射等手段,可以改变纤维素的结晶结构、形貌和分子链排列,从而改善其物理性能。
例如,热处理可以使纤维素分子链发生重排,提高其结晶度和热稳定性;而高能辐射则可以引发纤维素分子链的断裂和交联,形成新的功能基团。
化学改性是另一种广泛应用的纤维素改性方法。
通过引入化学试剂,如酸、碱、氧化剂或还原剂等,可以改变纤维素的化学结构和性质。
例如,酸处理可以使纤维素发生水解反应,生成低分子量的纤维素衍生物;而碱处理则可以破坏纤维素的结晶结构,增加其反应活性。
通过与有机化合物反应,还可以在纤维素分子链上引入特定的功能基团,如羟基、羧基、氨基等,从而赋予其新的性能。
纤维素的综述
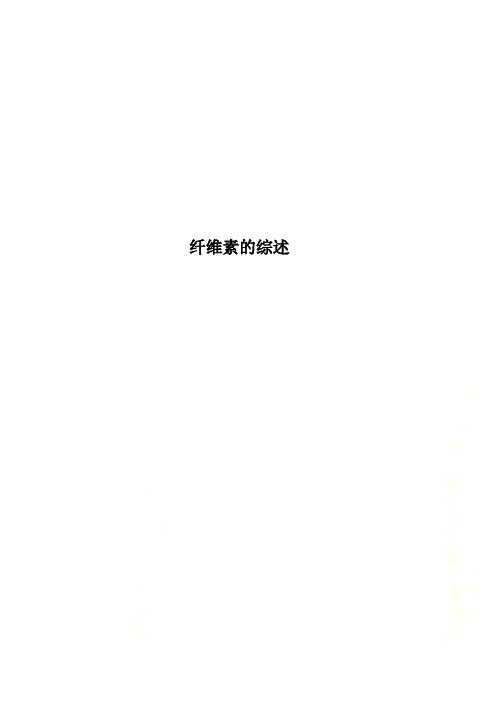
纤维素的综述纤维素091060049 杨俊昇摘要:纤维素是棉花、木材、亚麻、草类等高等植物细胞壁的主要成分,纤维素在纺织工业,造纸工业,木材工业等领域有着多种重要的用途,自然界中的植物原料是年复一年地不断生长和更新着,可以这样认为,纤维素在自然界中是一种最丰富的可再生的有机资源。
关键词:纤维素、应用、造纸、资源纤维素(cellulose)是由葡萄糖组成的大分子多糖。
不溶于水及一般有机溶剂。
是植物细胞壁的主要成分。
纤维素是世界上最丰富的天然有机物,占植物界碳含量的50%以上。
棉花的纤维素含量接近100%,为天然的最纯纤维素来源。
一般木材中,纤维素占40~50%,还有10~30%的半纤维素和20~30%的木质素。
34(OH)2溶液和铜乙二胺[NH2CH2CH2NH2]Cu(OH)2溶液等。
水可使纤维素发生有限溶胀,某些酸、碱和盐的水溶液可渗入纤维结晶区,产生无限溶胀,使纤维素溶解。
纤维素加热到约150℃时不发生显著变化,超过这温度会由于脱水而逐渐焦化。
纤维素与较浓的无机酸起水解作用生成葡萄糖等,与较浓的苛性碱溶液作用生成碱纤维素,与强氧化剂作用生成氧化纤维素。
纤维素柔顺性很差,是刚性的,因为它分子有极性,分子链之间相互作用力很强;纤维素中的六元吡喃环结构致使内旋转困难;其分子内和分子间都能形成氢键特别是分子内氢键致使糖苷键不能旋转从而使其刚性大大增加。
纤维素的实验室制法是先用水、有机溶剂处理植物原料,再用氯、亚氯酸盐、二氧化氯、过乙酸去除其中所含的木素,得到纤维素和半纤维素,然后采用各种方法除去半纤维素,制得纯纤维素。
工业制法是用亚硫酸盐溶液或碱溶液蒸煮植物原料,除去木素,然后经过漂白进一步除去残留木素,所得漂白浆可用于造纸。
纤维素是地球上最古老、最丰富的天然高分子,是取之不尽用之不竭的,人类最宝贵的天然可再生资源。
纤维素化学与工业始于160多年前,是高分子化学诞生及发展时期的主要研究对象,纤维素及其衍生物的研究成果为高分子物理及化学学科的创立、发展和丰富作出了重大贡献。
纤维素 木质素 甲壳素
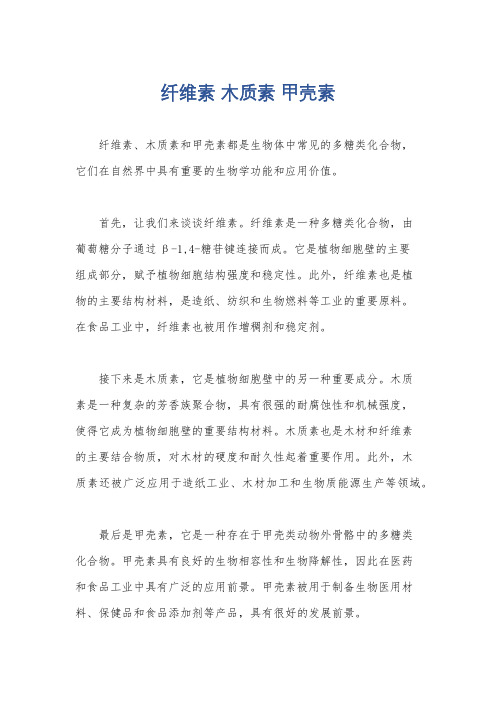
纤维素木质素甲壳素
纤维素、木质素和甲壳素都是生物体中常见的多糖类化合物,
它们在自然界中具有重要的生物学功能和应用价值。
首先,让我们来谈谈纤维素。
纤维素是一种多糖类化合物,由
葡萄糖分子通过β-1,4-糖苷键连接而成。
它是植物细胞壁的主要
组成部分,赋予植物细胞结构强度和稳定性。
此外,纤维素也是植
物的主要结构材料,是造纸、纺织和生物燃料等工业的重要原料。
在食品工业中,纤维素也被用作增稠剂和稳定剂。
接下来是木质素,它是植物细胞壁中的另一种重要成分。
木质
素是一种复杂的芳香族聚合物,具有很强的耐腐蚀性和机械强度,
使得它成为植物细胞壁的重要结构材料。
木质素也是木材和纤维素
的主要结合物质,对木材的硬度和耐久性起着重要作用。
此外,木
质素还被广泛应用于造纸工业、木材加工和生物质能源生产等领域。
最后是甲壳素,它是一种存在于甲壳类动物外骨骼中的多糖类
化合物。
甲壳素具有良好的生物相容性和生物降解性,因此在医药
和食品工业中具有广泛的应用前景。
甲壳素被用于制备生物医用材料、保健品和食品添加剂等产品,具有很好的发展前景。
总的来说,纤维素、木质素和甲壳素作为重要的多糖类化合物,在植物细胞壁构建、工业生产和生物医药领域都具有重要的应用价值,对于人类社会的发展和生活水平的提高起着重要的作用。
- 1、下载文档前请自行甄别文档内容的完整性,平台不提供额外的编辑、内容补充、找答案等附加服务。
- 2、"仅部分预览"的文档,不可在线预览部分如存在完整性等问题,可反馈申请退款(可完整预览的文档不适用该条件!)。
- 3、如文档侵犯您的权益,请联系客服反馈,我们会尽快为您处理(人工客服工作时间:9:00-18:30)。
纤维素综述
简述:
微晶纤维素是天然纤维素的水解产物,外观呈白色或类白色,是一种晶形粉末产品,不溶于水,性质稳定,与主药不发生化学反应。
作为填充剂,适量用于处方中,可以使制得的颗粒较松散,均匀细小,结合性能好,同时,由于它吸水后能使片子迅速膨胀而崩解,因此,它又是一种良好的崩解剂。
近年来,作为一种新型辅料,微晶纤维素在片剂生产制造中的应用越来越广泛。
在近些年的新产品开发研制,原有产品处方的改进及不合格中间产品的再处理等方面,均在不同程度上使用了这一新型辅料,并收到了良好的效果。
用于新处方设计中由于微晶纤维素具有良好的结合性与崩解性,因此在开发研制新产品时,它是一种优先选用的辅料,微晶纤维的作用要强于其它类似辅料如淀粉、糊精等。
这在处方设计时,我们优先选用了微晶纤维素,经过数次处方调整与试验,当微晶纤维素在片中的含量达到0.025g/片时,生产出的片子结合很好,具有足够的硬度。
制法:
微晶纤维素可用稀无机酸溶液将α-纤维素控制水解制得,α-纤维素可从含纤维素植物的纤维浆制得。
水解后的纤维素经过滤、提纯、水浆喷雾干燥形成干的。
粒径分布广泛的多孔颗粒。
应用:
解决因工艺带来的问题有些产品的工艺是在迁就产品达到某些
指标情况下制定的,一旦工艺变化就会带来诸多问题。
例如新速效感冒片,在原处方基础上,为了保证其溶出度符合药典规定,在工艺上不得已将颗粒制得比正常的软一些,打片时打片机的压力也要尽量小一些,使得打出的片子硬度低。
在进行薄膜包装时,常常会造成很多碎片,使得成片率降低,为了提高成片率就必须提高片子的硬度。
要提高硬度,又不影响溶出度,就要改变原处方,这时微晶纤维素又是一个良好的优选辅料。
生产中,压力提高后,我们用一定量的微晶纤素取代了原处方中相应量的某些其它辅料,当其用量达到。
片时,收到了良好的效果。
片子的硬度由原来的提高至,同时其溶出度也很好,完全符合质量要求。
用于不合格中间体的再处理有时制出的片子鼓盖掉盖,裂片或崩解超限。
原因在于软材制得不实、颗粒不完整、颗粒太软、干燥程度不够、淀粉糊浓度过大或温度过高,都可以造成上述问题。
此外,有些经再处理才合格的原料,如乙酞螺旋霉素,其某些物理性质会发生变化,这也可以导致上述问题的产生。
处理方法中间体再处理时,其处理方法因产品的不同及成因不同也有所区别,微晶纤维素的用量也不一样。
乙酞螺旋霉素将制好的不合格的中间体颗粒过目筛,细粉按的比例加人微晶纤维素,混匀后加人适量的温淀粉糊制成软材,混实后再制粒,干燥整粒后再加人适量润滑剂,混匀,再投人到开始筛出的颗粒中大混、打片。
如为原料原因时,则在投料时直接用一定量的微晶纤维素取代相应量的淀粉投料。
每万片投料量用微晶纤维素。
交沙霉素、维生素、峡喃坦陡在实际生产中,交沙霉素、维生素容易出现软材制得不实,颗粒发散、结合不好的现象,峡喃坦睫易出现颗粒过硬、崩解
不好的现象,致使中间体不合格。
处理时根据不同的产品按不同的比例,同时还要考虑中间体不合格的程度,一般比例在范围内,适当加人微晶纤维素,实施补救,一般均可收到满意的结果。
具体操作为先从不合格中间体颗粒中用目或目筛选出适量细粉,将该粉与过目筛、给定量的微晶纤维素混合均匀,将该混合物重新投人到不合格中间体颗粒中,大混,打片。
由于微晶纤维素分子之间存在氢键,受压时氢键缔合,故具有高度的可压性,常被用作于猫合剂;压制的片剂遇到液体后,水分迅速进入含有微晶纤维素的片剂内部,氢键即刻断裂,所以可作为崩解剂"因此,它是片剂生产中广泛使用的一种辅料,能够提高片剂的硬度"例如.在制备利福平药片中可用MCC与淀粉(6.25:l质量比)和各种原料混合均匀后直接压片,产品在lmin内崩散成雾状.而且在有效期内含t不变,并能很好地提高药物稳定性"又如,由于加人微晶纤维素,醋酸泼尼松与醋酸黄连素(盐酸小劈碱)片剂的溶出度提高到80%以上"用微晶纤维素做辅料压片时不需经过传统的造粒过程,例如在制备咳必清药片中由于加人了MCC,解决了咳必清湿法造粒压片易吸潮而出现的严重猫冲现象,并且崩解迅速"微晶纤维素也可用作药品的缓释剂"缓释过程是由活性物质进人载体的多孔结构.活性物质被分子间氢键包含,干燥后活性物质被固定"活性物质释放时由于水在聚合物载体的毛细管系统内扩散引起润胀,载体经基和被固定的活性物质之间的化合键被破坏,活性物质缓慢地释放出来"微晶纤维素粉末在水中能形成稳定的分散体系,将其与药物配合可制成奶油状或悬浮状的药液,同时
还可用作胶囊剂"微晶纤维素在水中经强力搅拌生成凝胶,也可用于制造*状和悬浮液类型医药制剂"美国学者使用流化床凝集作用,以微晶纤维素及预糊化淀粉为填充剂与崩解剂,直接压成具优良生理有效性的扑热息痛片"英国专利指出,微晶纤维素与不溶性药物混合,可直接挤压出逐步释放药效的球形药丸"据相关文献报导,利用微晶纤维素吸附氯化甲基蔷薇苯胺水溶液来制造油膏,可防止褪色和抗菌性。
结论:
在片剂生产制造中,对改善片子的结合性、崩解性或溶出性,微晶纤维素是一种优选的辅助原料,性能优于同类的传统辅料,其应用面必将越来越大,用量亦将越来越高。