Thermal Width Broadening of the 0++ Glueball Spectrum near Tc
broad temperature range 温度
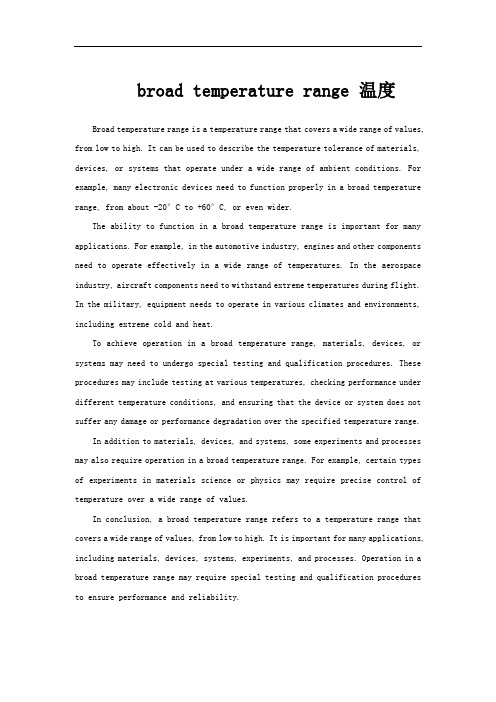
broad temperature range 温度Broad temperature range is a temperature range that covers a wide range of values, from low to high. It can be used to describe the temperature tolerance of materials, devices, or systems that operate under a wide range of ambient conditions. For example, many electronic devices need to function properly in a broad temperature range, from about -20°C to +60°C, or even wider.The ability to function in a broad temperature range is important for many applications. For example, in the automotive industry, engines and other components need to operate effectively in a wide range of temperatures. In the aerospace industry, aircraft components need to withstand extreme temperatures during flight. In the military, equipment needs to operate in various climates and environments, including extreme cold and heat.To achieve operation in a broad temperature range, materials, devices, or systems may need to undergo special testing and qualification procedures. These procedures may include testing at various temperatures, checking performance under different temperature conditions, and ensuring that the device or system does not suffer any damage or performance degradation over the specified temperature range.In addition to materials, devices, and systems, some experiments and processes may also require operation in a broad temperature range. For example, certain types of experiments in materials science or physics may require precise control of temperature over a wide range of values.In conclusion, a broad temperature range refers to a temperature range that covers a wide range of values, from low to high. It is important for many applications, including materials, devices, systems, experiments, and processes. Operation in a broad temperature range may require special testing and qualification procedures to ensure performance and reliability.。
辐射强度与波长与温度的关系 英文解释
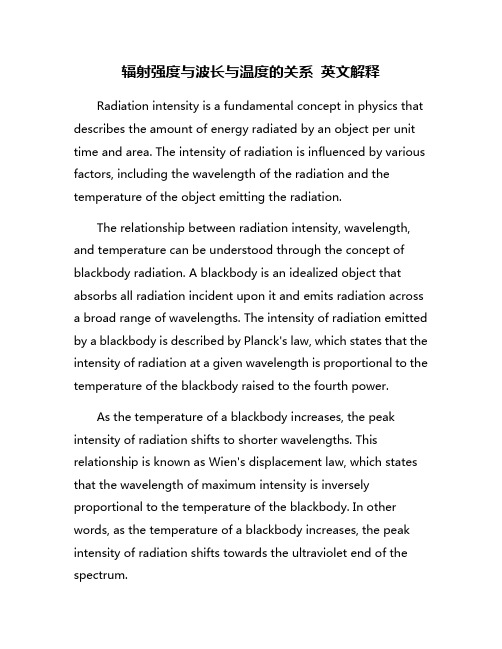
辐射强度与波长与温度的关系英文解释Radiation intensity is a fundamental concept in physics that describes the amount of energy radiated by an object per unit time and area. The intensity of radiation is influenced by various factors, including the wavelength of the radiation and the temperature of the object emitting the radiation.The relationship between radiation intensity, wavelength, and temperature can be understood through the concept of blackbody radiation. A blackbody is an idealized object that absorbs all radiation incident upon it and emits radiation across a broad range of wavelengths. The intensity of radiation emitted by a blackbody is described by Planck's law, which states that the intensity of radiation at a given wavelength is proportional to the temperature of the blackbody raised to the fourth power.As the temperature of a blackbody increases, the peak intensity of radiation shifts to shorter wavelengths. This relationship is known as Wien's displacement law, which states that the wavelength of maximum intensity is inversely proportional to the temperature of the blackbody. In other words, as the temperature of a blackbody increases, the peak intensity of radiation shifts towards the ultraviolet end of the spectrum.The Stefan-Boltzmann law describes the total power radiated by a blackbody as a function of its temperature. According to this law, the total power radiated by a blackbody is proportional to the fourth power of its temperature. This means that as the temperature of a blackbody increases, the total power radiated by the object also increases exponentially.In addition to temperature, the wavelength of radiation also plays a crucial role in determining the intensity of radiation. The relationship between wavelength and intensity is described by the inverse square law, which states that the intensity of radiation is inversely proportional to the square of the wavelength.In conclusion, the intensity of radiation is influenced by both the temperature of the object emitting the radiation and the wavelength of the radiation. As the temperature of a blackbody increases, the peak intensity of radiation shifts to shorter wavelengths, while the total power radiated by the object increases exponentially. Additionally, the intensity of radiation is inversely proportional to the square of the wavelength. These relationships between radiation intensity, wavelength, and temperature are fundamental principles in the study ofblackbody radiation and play a crucial role in various areas of physics and engineering.。
光纤通信英文版常见中英对照单词表教学内容
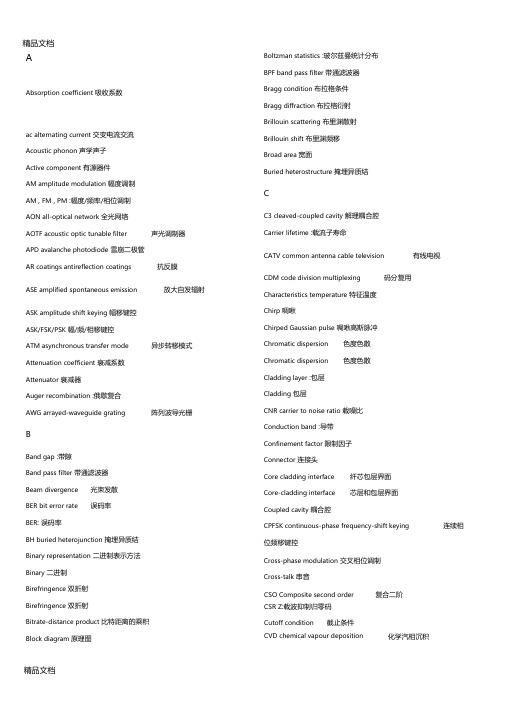
精品文档AAbsorption coefficient 吸收系数ac alternating current 交变电流交流Acoustic phonon 声学声子Active component 有源器件AM amplitude modulation 幅度调制AM , FM , PM :幅度/频率/相位调制AON all-optical network 全光网络AOTF acoustic optic tunable filter 声光调制器APD avalanche photodiode 雪崩二极管AR coatings antireflection coatings 抗反膜ASE amplified spontaneous emission 放大自发辐射ASK amplitude shift keying 幅移键控ASK/FSK/PSK 幅/频/相移键控ATM asynchronous transfer mode 异步转移模式Attenuation coefficient 衰减系数Attenuator 衰减器Auger recombination :俄歇复合AWG arrayed-waveguide grating 阵列波导光栅BBand gap :带隙Band pass filter 带通滤波器Beam divergence 光束发散BER bit error rate 误码率BER: 误码率BH buried heterojunction 掩埋异质结Binary representation 二进制表示方法Binary 二进制Birefringence 双折射Birefringence 双折射Bitrate-distance product 比特距离的乘积Block diagram 原理图Boltzman statistics :玻尔兹曼统计分布BPF band pass filter 带通滤波器Bragg condition 布拉格条件Bragg diffraction 布拉格衍射Brillouin scattering 布里渊散射Brillouin shift 布里渊频移Broad area 宽面Buried heterostructure 掩埋异质结CC3 cleaved-coupled cavity 解理耦合腔Carrier lifetime :载流子寿命CATV common antenna cable television 有线电视CDM code division multiplexing 码分复用Characteristics temperature 特征温度Chirp 啁啾Chirped Gaussian pulse 啁啾高斯脉冲Chromatic dispersion 色度色散Chromatic dispersion 色度色散Cladding layer :包层Cladding 包层CNR carrier to noise ratio 载噪比Conduction band :导带Confinement factor 限制因子Connector 连接头Core cladding interface 纤芯包层界面Core-cladding interface 芯层和包层界面Coupled cavity 耦合腔CPFSK continuous-phase frequency-shift keying 连续相位频移键控Cross-phase modulation 交叉相位调制Cross-talk 串音CSO Composite second order 复合二阶CSR Z:载波抑制归零码Cutoff condition 截止条件CVD chemical vapour deposition 化学汽相沉积CW continuous wave 连续波Cylindrical preform :预制棒DDBR distributed Bragg reflector 分布布拉格反射DBR: distributed Bragg reflector 分布式布拉格反射器精品文档dc direct current 直流DCF dispersion compensating fiber色散补偿光纤Depressed-cladding fiber: DFB distributed feedback DFB: Distributed Feedback Differential gain 微分增益 Differential quantum efficiency Diffusion 扩散Digital hierarchy 数字体系 DIP dual in line package 双列直插 Direct bandgap :直接带隙 Directional coupler定向耦合器Dispersion compensation fiber :色散补偿光纤 Dispersion decreasing fiber:色散渐减光纤Dispersion parameter :色散参数 Dispersion shifted fiber 色散位移光纤Dispersion slope 色散斜率 Dispersion slope :色散斜率 Dispersion-flatten fiber :色散平坦光纤 Dispersion-shifted fiber :色散位移光纤 Double heterojunction 双异质结 Double heterostructure :双异质结Doubly clad :双包层DPSK differential phase-shift keying 差分相移键控Driving circuit 驱动电路 Dry fiber 无水光纤 DSF dispersion shift fiber色散位移光纤DWDM dense wavelength division multiplexing/multiplexer 密集波分复用 / 器DWDM: dense wavelength division multiplexing密集波分 复用E~GEDFA erbium doped fiber amplifier掺铒光纤激光器Edge emitting LED 边发射 LED Edge-emitting 边发射 Effective index 有效折射率 Eigenvalue equation 本征值方程 Elastic scattering 弹性散射 Electron-hole pairs 电子空穴对 Electron-hole recombination 电子空穴复合 Electron-hole recombination :电子空穴复合Electrostriction 电致伸缩效应 Ethernet 以太网 External cavity 外腔External quantum efficiency 外量子效率 Extinction ratio 消光比 Eye diagram 眼图 FBG fiber-bragg grating光纤布拉格光栅FDDI fiber distributed data interface 光纤数据分配接口 FDM frequency division multiplexing 频分复用FDM :频分复用 Fermi level 费米能级 Fermi level :费米能级Fermi-Dirac distribution :费米狄拉克分布 FET field effect transistor场效应管Fiber Manufacturing :光纤制作 Field radius 模场半径Filter 滤波器Flame hydrolysis 火焰裂解FM frequency modulation 频率调制Forward-biased :正向偏置 FP Fabry Perot 法布里 - 珀落 Free spectral range 自由光谱范围 Free - space communication自由空间光通信系统Fresnel transmissivity 菲涅耳透射率 Front end 前端Furnace 熔炉FWHM full width at half maximum 半高全宽Differential-dispersion parameter 凹陷包层光纤 分布反馈 分布式反馈微分量子效率FWHM: 半高全宽FWM four-wave mixing 四波混频Gain coefficient 增益系数Gain coupled 增益耦合Gain-guided semiconductor laser 增益波导半导体激光器Germania 锗GIOF graded index optical fiber 渐变折射率分布Graded-index fiber 渐变折射率光纤Group index 群折射率精品文档 GVD group-velocity dispersion GVD: 群速度色散 H~LHBT heterojunction-bipolar transistor 异质结双极晶体管HDTV high definition television高清晰度电视Heavy doping :重掺杂 Heavy-duty cable 重型光缆Heterodyne 外差 Heterojunction :异质结 HFC hybrid fiber-coaxial混合光纤 / 电缆Higher-order dispersion 高阶色散 Highpass filter 高通滤波器 Homodyne 零差Homojunction :同质结 IC integrated circuit 集成电路 IM/DD intensity modulation with direct detection强度调制直接探测IM/DD: 强度调制 / 直接探测 IMD intermodulation distortion 交互调制失真 Impulse 冲激Impurity 杂质 Index-guided 折射率导引 Indirect bandgap :非直接带隙 Inelastic scattering 非弹性散射 Inhomogeneous 非均匀的Inline amplifier 在线放大器 Intensity noise 强度噪声 Intermodal dispersion : 模间色散 Intermode dispersion 模间色散 Internal quantum efficiency :内量子效率 Intramodal dispersion: 模内色散 Intramode dispersion 模内色散 Intrinsic absorption 本征吸收 ISDN integrated services digital network 综合业务数字网ISI intersymbol interference码间干扰Isotropic 各向同性 Jacket 涂层 Jitter 抖动 Junction :结 Kinetic energy :动能精品文档群速度色散 Lambertian source 朗伯光源LAN local-area network 局域网Large effective-area fiber 大有效面积发光Laser threshold 激光阈值 Laser 激光器 Lateral mode 侧模 Lateral 侧向Lattice constant :晶格常数 Launched power 发射功率 LD laser diode 激光二极管 LD :激光二极管LED light emitting diode 发光二极管LED: 发光二极管 L-I light current 光电关系 Light-duty cable 轻型光缆 Linewidth enhancement factor 线宽加强因子 Linewidth enhancement factor 线宽增强因子Linewidth 线宽Longitudinal mode 纵模 Longitudinal model 纵模 Lowpass filter 低通滤波器 LPE liquid phase epitaxy 液相外延 LPE :液相外延M~NMacrobending 宏弯MAN metropolitan-area network 城域网 Material dispersion 材料色散 Material dispersion:材料色散Maxwell 's equations 麦克斯韦方程组 MBE molecular beam epitaxy 分子束外延 MBE :分子束外延MCVD Modified chemical vapor deposition相沉积MCVD :改进的化学汽相沉积 Meridional rays 子午光线 Microbending 微弯 Mie scattering 米氏散射MOCVD metal-organic chemical vapor deposition机物化学汽相沉积MOCVD :改进的化学汽相沉积 Modal dispersion 模式色散改进的化学汽金属有精品文档Mode index 模式折射率Modulation format 调制格式Modulator 调制器MONET Multiwavelength optical networkMPEG motion-picture entertainment group 小组MQW: 多量子阱MSR mode-suppression ratioMSR: Mode suppression ratioMultimode fiber 多模光纤MZ mach-Zehnder 马赫泽德NA numerical aperture 数值孔径Near infrared 近红外NEP noise-equivalent powerNF noise figure 噪声指数Nonradiative recombinationNonradiative recombinationNormalized frequency 归一化频率NRZ :非归零码NSE nonlinear Schrodinger equationNumerical aperture 数值孔径Nyquist criterion 奈奎斯特准则O P QOC optical carrier 光载波OEIC opto-electronic integrated circuit 光电集成电路OOK on-off keying 开关键控OOK :通断键控OPC optical phase conjugation 光相位共轭Optical mode 光模式Optical phase conjugation 光相位共轭Optical soliton 光孤子Optical switch 光开关Optical transmitter 光发射机Optical transmitter :光发射机OTDM optical time-division multiplexing 光时分复用OVD outside-vapor deposition 轴外汽相沉积OVD :轴外汽相沉积OXC optical cross-connect 光交叉连接Packaging 封装Packet switch 分组交换Parabolic-index fiber 抛物线折射率分布光纤Passive component 无源器件PCM pulse-code modulation 脉冲编码调制PCM :脉冲编码调制PCVD :等离子体化学汽相沉积PDF probability density function 概率密度函数PDM polarization-division multiplexing 偏振复用PDM :脉冲宽度调制Phase-matching condition 相位匹配条件Phase-shifted DFB laser 相移DFB 激光器Photon lifetime 光子寿命PMD 偏振模色散Polarization controller 偏振控制器Polarization mode dispersion :偏振模色散Polarization 偏振PON passive optical network 无源接入网Population inversion :粒子数反转Power amplifier 功率放大器Power-conversion efficiency 功率转换效率PPM :脉冲位置调制Preamplifer 前置放大器PSK phase-shift keying 相移键控Pulse broadening 脉冲展宽Quantization noise 量化噪声Quantum efficiency 量子效率Quantum limit 量子极限Quantum limited 量子极限Quantum noise 量子噪声RRA raman amplifier 喇曼放大器Raman scattering 喇曼散射Rate equation 速率方程Rayleigh scattering 瑞丽散射Rayleigh scattering 瑞利散射精品文档Receiver sensitivity 接收机灵敏度Receiver 接收机Refractive index 折射率Regenerator 再生器Repeater spacing 中继距离Resonant cavity 谐振腔ResponsibilityMPN mode-partition noise 模式分配噪声MQW multiquantum well 多量子阱MSK minimum-shift keying 最小频偏键控模式分配噪声模式抑制比等效噪声功率非辐射复合:非辐射复合NRZ non-return to zero 非归零多波长光网络视频动画专家非线性薛定额方程响应度Responsivity 响应度Ridge waveguide laser 脊波导激光器Ridge waveguide 脊波导RIN relative intensity noise 相对强度噪声RMS root-mean-square 均方根RZ return-to-zero 归零RZ: 归零码SSAGCM separate absorption, grading, charge, and multiplication 吸收渐变电荷倍增区分离APD 的一种SAGM separate absorption and multiplication 吸收渐变倍增区分离APD 的一种SAM separate absorption and multiplication 吸收倍增区分离APD 的一种Sampling theorem 抽样定理SBS 受激布里渊散射SBS stimulated Brillouin scattering 受激布里渊散射SCM subcarrier multiplexing 副载波复用SDH synchronous digital hierarchy 同步数字体系SDH :同步数字体系Self-phase modulation 自相位调制Sellmeier equation :塞米尔方程Sensitivity degradation 灵敏度劣化Sensitivity 灵敏度Shot noise 散粒噪声Shot noise 散粒噪声Single-mode condition 单模条件Sintering :烧结SIOF step index optical fiber 阶跃折射率分布SLA/SOA semiconductor laser/optical amplifier 半导体光放大器SLM single longitudinal mode 单纵模SLM: Single Longitudinal mode 单纵模Slope efficiency 斜率效率SNR signal-to-noise ratio 信噪比Soliton 孤子SONET synchronized optical network 同步光网络SONET :同步光网络Spectral density :光谱密度Spontaneous emission :自发辐射Spontaneous-emission factor 自发辐射因子SRS 受激喇曼散射SRS stimulated Raman scattering 受激喇曼散射Step-index fiber 阶跃折射率光纤Stimulated absorption :受激吸收Stimulated emission :受激发射STM synchronous transport module 同步转移模块STM :同步转移模块Stripe geometry semiconductor laser 条形激光器Stripe geometry 条形STS synchronous transport signal 同步转移信号Submarine transmission system 海底传输系统Substrate: 衬底Superstructure grating 超结构光栅Surface emitting LED 表面发射LED Surface recombination :表面复合Surface-emitting 表面发射TCP/IP transmission control protocol/internet protocol 传输控制协议/ 互联网协议TDM time-division multiplexing 时分复用TDM :时分复用TE transverse electric 横电模Ternary and quaternary compound :三元系和四元系化合物Thermal equilibrium :热平衡Thermal noise 热噪声Thermal noise 热噪声Threshold current 阈值电流Timing jitter 时间抖动TM transverse magnetic 横磁Total internal reflection 全内反射Transceiver module 收发模块精品文档Transmitter 发射机Transverse 横向Transverse mode 横模TW traveling wave 行波U ~ ZVAD vapor-axial epitaxy 轴向汽相沉积VAD :轴向沉积Valence band :价带VCSEL vertical-cavity surface-emitting laser 垂直腔表面发射激光器VCSEL: vertical cavity surface-emitting lasers 垂直腔表面发射激光器VPE vapor-phase epitaxy 汽相沉积VPE :汽相外延VSB vestigial sideband 残留边带Wall-plug efficiency 电光转换效率WAN wide-area network 广域网Waveguide dispersion 波导色散Waveguide dispersion :波导色散Waveguide imperfection 波导不完善WDMA wavelength-division multiple access 波分复用接入系统WGA waveguide-grating router 波导光栅路由器White noise 白噪声XPM cross-phase modulation 交叉相位调制YIG yttrium iron garnet 钇铁石榴石晶体Zero-dispersion wavelength 零色散波长Zero-dispersion wavelength :零色散波长。
TA Instruments Thermal Diffusivity Systems安装要求说明书
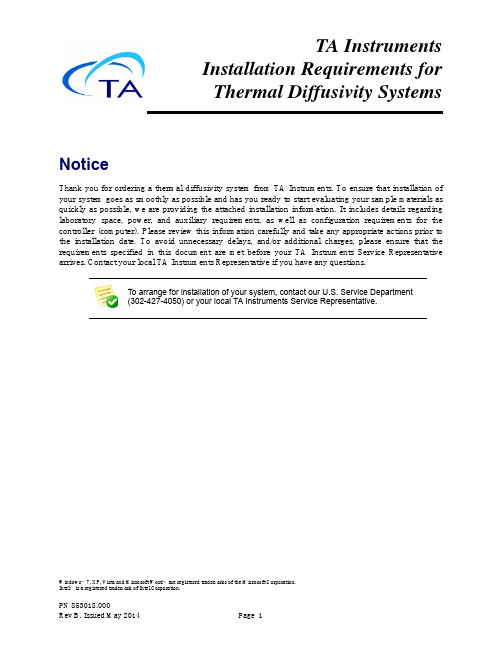
Windows® 7, XP, Vista and Microsoft Word® are registered trademarks of the Microsoft Corporation.Intel® is a registered trademark of Intel Corporation.PN 853018.000TA InstrumentsInstallation Requirements forThermal Diffusivity SystemsNoticeThank you for ordering a thermal diffusivity system from TA Instruments. To ensure that installation of your system goes as smoothly as possible and has you ready to start evaluating your sample materials as quickly as possible, we are providing the attached installation information. It includes details regarding laboratory space, power, and auxiliary requirements, as well as configuration requirements for the controller (computer). Please review this information carefully and take any appropriate actions prior to the installation date. To avoid unnecessary delays, and/or additional charges, please ensure that the requirements specified in this document are met before your TA Instruments Service Representativearrives. Contact your local TA Instruments Representative if you have any questions.To arrange for installation of your system, contact our U.S. Service Department (302-427-4050) or your local TA Instruments Service Representative.Important: TA Instruments Manual Supplement Please refer to the TA Manual Supplement to access the following important information supplemental to this document:•TA Instruments Trademarks•TA Instruments Patents•Other Trademarks•TA Instruments End-User License Agreement•TA Instruments OfficesTable of Contents Notice (1)Important: TA Instruments Manual Supplement (2)Table of Contents (3)Requirements for the Controller (Computer) (4)Hardware Considerations (4)Software Considerations (4)Requirements for the Thermal Diffusivity System Instrument (5)Instrument & Accessory Placement (5)DXF and DLF-1 Pulse Source (5)DLF-2 Pulse Source (6)EM-200 (7)EM-500 (8)EM-900 (9)EM-1200 (10)EM-1600 (11)EM-2800 (12)TA Instruments Offices (13)Requirements for the Controller (Computer)A working thermal diffusivity system consists of one or more measurement instruments (e.g., DLF-1600, DLF-2800, etc.) and a computer configured with appropriate TA Instruments software (this latter combination is subsequently referred to as a controller). The controller is included with the instrument and requires the following:Hardware Considerations•The computer should not be attached to other analytical instruments or any LAN.•The PC requires an unused RS232 serial port and an unused USB for connection to the instrument.•The PC is not permitted to run other programs or any power saving features while a test is running.•Network cards and/or certain network operation frequently interfere with the operation of the instru-ment control programs.•The PC requires a Windows 32-bit operating system.Software Considerations•Peripherals (e.g., printer) must be from the known Windows compatible list. (See Microsoft's web site at /hwtest for the most current list.)•TA Instruments is not responsible for resolving issues associated with connections to your corporate network. [See further information in the next section.]•TA Instruments is not responsible for resolving hardware/software conflicts created by the addition of third party hardware or software to the computer.Requirements for the Thermal Diffusivity System InstrumentA Thermal Diffusivity system consists of an instrument and a computer for instrument control. To obtain installation requirements for the instrument and controller, refer to the appropriate sections of this document.Instrument & Accessory PlacementSelect a location for the instrument with adequate floor space and a rigid laboratory bench that is level.Unless otherwise specified in the instrument requirements, the work space must allow 0.6 m (24 in) in front of the instrument, 0.3 m (12 in) on each side, and 0.3 m (12 in) behind the instrument. The PC must be located within 1.5 m (60 in) of the instrument, and the vacuum pump must be located within 1.2 m (48in) of the Environmental Module.DXF and DLF-1 Pulse Source Dimensions:Depth 102 cm (40 in), Width 61 cm (24 in), Height 46 cm (18 in)Weight:69 kg (155 lbs)Power requirements:Pulse Source supply voltage: 208–240 V AC 1 (rated for 10 A), 50/60 Hz 2, 3PC and peripherals: 208–240 V AC outlet (separate). Surge protection suggested.1.Supply voltages lower than indicated limit may result in a degradation of performance.2.This instrument is supplied with a power cord ~2 meters long. Ensure that the mains assigned do not also supply power to noise generating equipment nearby, such as motors, welders, transformers, etc.3.An independent heavy GROUND wire must be provided through the power hook up. Improper ground-ing may cause severe damage for which the supplier will not accept responsibility. All power strips must be fully grounded and carry the ground through to the sockets into which the computer is plugged.Laboratory conditions:Temperature: 15–35°CRelative Humidity: 5–80% (non-condensing at 15°C)The instrument should be located in a dust-free, vibration-free environment, away from exposure to direct sunlight and direct air drafts. Locate the instrument in a ventilated space (hood, etc.) if noxious gases or vapors are generated during the heating of samples.The PC must be located no more than 150 cm (60 in) from the right side of the instrument (as viewed from the front); Required space surrounding the instrument: 30 cm (12 in.) on all sides of equipmentDLF-2 Pulse Source Dimensions:Depth 25 cm (10 in), Width 30 cm (12 in), Height 114 cm (45 in)Weight:45 kg (100 lbs)Power requirements:Laser supply voltage: 208–240 V AC 1(rated for 5 A), 50/60 Hz 2, 3PC and peripherals: 208–240 V AC outlet (separate). Surge protection suggested.1.Supply voltages lower than indicated limit may result in a degradation of performance.2.This instrument is supplied with a power cord ~2 meters long. Ensure that the mains assigned do not also supply power to noise generating equipment nearby, such as motors, welders, transformers, etc.3.An independent heavy GROUND wire must be provided through the power hook up. Improper ground-ing may cause severe damage for which the supplier will not accept responsibility. All power strips must be fully grounded and carry the ground through to the sockets into which the computer is plugged.Laboratory conditions:Temperature: 15–35°CRelative Humidity: 5–80% (non-condensing at 15°C)The instrument should be located in a dust-free, vibration-free environment, away from exposure to direct sunlight and direct air drafts. Locate the instrument in a ventilated space (hood, etc.) if noxious gases or vapors are generated during the heating of samples.The PC must be located no more than 150 cm (60 in) from the right side of the instrument (as viewed from the front); Required space surrounded the instrument: 30 cm (12 in.) on all sides of equipmentDimensions:Depth 102 cm (40 in), Width 61 cm (24 in), Height 46 cm (18 in)Weight:10 kg (20 lbs)Laboratory requirements:Liquid Nitrogen: Maximum 22 psi (1.5 bar) supply pressure. Pressure exceeding22 psi will damage the instrument.Purge gas1: Nitrogen or argon (supplied by customer); preferred source: gascylinder. Minimum inlet pressure: 0.5 psi (0.03 bar); Maximum inlet pressure: 1psi (0.06 bar). Do NOT pressurize the furnace cavity.1.Improperly regulated, pulsating, or poor quality purge gas may cause irregular or erratic equipment oper-ation. Containment of exhaust is recommended if noxious or poisonous gases are released by samplewhen heated. Venting inert gases into small rooms may reduce the oxygen content of the air and become hazardous to personnel.Dimensions:Depth 102 cm (40 in), Width 61 cm (24 in), Height 77 cm (30 in)Weight:34 kg (75 lbs)Additional power requirements:Vacuum: 208–240 V AC outlet (rated for 10 A) (separate). Laboratory requirements:Liquid Nitrogen: 1 L/day in a small hand-held dewar flask, manually poured into the LN 2 receptacle.Purge gas 1: Nitrogen or argon (supplied by customer); preferred source: gas cylinder. Purge gas inlet must be filtered and dry. Minimum inlet pressure: 40 psi; Maximum inlet pressure 50 psi. Do NOT pressurize the furnace cavity.1.Improperly regulated, pulsating, or poor quality purge gas may cause irregular or erratic equipment oper-ation. Containment of exhaust is recommended if noxious or poisonous gases are released by sample when heated. Venting inert gases into small rooms may reduce the oxygen content of the air and become hazardous to personnel.Cooling water and drain:Minimum inlet pressure to the instrument: 40 psi 2; Maximum inlet pressure 80psi 3Nominal flow rate: 1–2 L/min. Varies with inlet pressurePermissible water temperature 15°C to 30°C, optimum 20°C 4, 5Air temperature/relative humidity: non-condensing at 15°C.The instrument is supplied with two hoses to connect to the Coolant Inlet and Outlet ports on the back of the furnace. The other end of each hose has a 1/4” male fitting. The coolant source must be connected to the 1/4” male fittings of each hose. The customer is required to make all hose connections.If plant-wide recirculation is used, a minimum inlet/outlet differential pressure of 50 psi is required.2.Operating close to or below minimum pressure will result in erratic operation.3.A chiller/circulator may be supplied. Consult the chiller/circulator manual for specifications concerning appropriate fluid types. The instrument is supplied with a hose to be connected between the instrument and the chiller/circulator. The customer is required to make all hose connections.4.Excessively cold water will result in “sweating” and corrosion of cooled metal surfaces. Warm water may not allow starting a test from below 25°C.5.Wall mounted supply shutoff, open drain, and city water are required if no chiller/circulator was ordered.Dimensions:Depth 102 cm (40 in), Width 61 cm (24 in), Height 77 cm (30 in)Weight:31 kg (75 lbs)Additional power requirements:Vacuum: 208–240 V AC outlet (rated for 10 A) (separate). Laboratory requirements:Liquid Nitrogen: 1 L/day in a small hand-held dewar flask, manually poured into the LN 2 receptacle.Purge gas 1: Nitrogen or argon (supplied by customer); preferred source: gas cylinder. Purge gas inlet must be filtered and dry. Minimum inlet pressure: 40 psi; Maximum inlet pressure 50 psi. Do NOT pressurize the furnace cavity.1.Improperly regulated, pulsating, or poor quality purge gas may cause irregular or erratic equipment oper-ation. Containment of exhaust is recommended if noxious or poisonous gases are released by sample when heated. Venting inert gases into small rooms may reduce the oxygen content of the air and become hazardous to personnel.Cooling water and drain:Minimum inlet pressure to the instrument: 40 psi 2; Maximum inlet pressure 80psi 3Nominal flow rate: 1–2 L/min. Varies with inlet pressurePermissible water temperature 15°C to 30°C, optimum 20°C 4, 5Air temperature/relative humidity: non-condensing at 15°C.The instrument is supplied with two hoses to connect to the Coolant Inlet and Outlet ports on the back of the furnace. The other end of each hose has a 1/4” male fitting. The coolant source must be connected to the 1/4” male fittings of each hose. The customer is required to make all hose connections.If plant-wide recirculation is used, a minimum inlet/outlet differential pressure of 50 psi is required.2.Operating close to or below minimum pressure will result in erratic operation.3.A chiller/circulator may be supplied. Consult the chiller/circulator manual for specifications concerning appropriate fluid types. The instrument is supplied with a hose to be connected between the instrument and the chiller/circulator. The customer is required to make all hose connections.4.Excessively cold water will result in “sweating” and corrosion of cooled metal surfaces. Warm water may not allow starting a test from below 25°C.5.Wall mounted supply shutoff, open drain, and city water required if no chiller/circulator was ordered.Dimensions:Depth 102 cm (40 in), Width 61 cm (24 in), Height 77 cm (30 in)Weight:34 kg (75 lbs)Laboratory requirements:Liquid Nitrogen cooling (static): 1 L/day; use a small hand held dewar flask and manually pour into the LN 2 receptacle.Purge gas 1: Nitrogen or argon (supplied by the customer); preferred source: gas cylinder. Purge gas inlet must be filtered and dry. Minimum inlet pressure: 40 psi; Maximum inlet pressure 50 psi. Do NOT pressurize the furnace cavity.The instrument is supplied with hose barbs to be connected to rubber or Tygon tubing, minimum inside tubing diameter of 3.175 mm (or equivalent), minimum pressure rating of 100 psi (7 bar). The customer is required to make all hose connections.1.Improperly regulated, pulsating, or poor quality purge gas may cause irregular or erratic instrument operation. Containment of exhaust is recommended if noxious or poisonous gases are released by sam-ple when heated. Venting inert gases into small rooms may reduce the oxygen content of the air andbecome hazardous to personnel.Cooling water and drain:Minimum inlet pressure to the instrument: 40 psi 2; Maximum inlet pressure 80psi 3Nominal flow rate: 1–2 L/min. Varies with inlet pressurePermissible water temperature 15°C to 30°C, optimum 20°C 4, 5Air temperature/relative humidity: non-condensing at 15°C.The instrument is supplied with two hoses to connect to the Coolant Inlet and Outlet ports on the back of the furnace. The other end of each hose has a 1/4” male fitting. The coolant source must be connected to the 1/4” male fittings of each hose. The customer is required to make all hose connections.If plant-wide recirculation is used, a minimum inlet/outlet differential pressure of 50 psi is required.2.Operating close to or below minimum pressure will result in erratic operation.3.A chiller/circulator may be supplied. Consult the chiller/circulator manual for specifications concerning appropriate fluid types. The instrument is supplied with a hose to be connected between the instrument and the chiller/circulator. The customer is required to make all hose connections.4.Excessively cold water will result in “sweating” and corrosion of cooled metal surfaces. Warm water may not allow starting a test from below 25°C.5.Wall mounted supply shutoff, open drain, and city water required if no chiller/circulator was ordered.Dimensions:Depth 72 cm (28 in), Width 107 cm (42 in), Height 153 cm (60 in)Weight:137 kg (300 lbs)Additional Power requirements:Main furnace supply voltage: 208–240 V AC outlet (rated for 25A), 50/60 Hz Pump: 220–240 V AC outlet (rated for 15A) (separate)Laboratory requirements:Liquid Nitrogen cooling (static): 1 L/day; use a small hand held dewar flask and manually pour into the LN 2 receptacle.Purge gas 1: Dry nitrogen or dry argon (supplied by the customer); preferred source: gas cylinder. Purge gas inlet must be filtered and dry. Minimum inlet pressure: 40 psi; Maximum inlet pressure 50 psi. Do NOT pressurize the furnace cavity.The instrument is supplied with hose barbs to be connected to rubber or Tygon tubing, minimum inside tubing diameter of 3.175 mm (or equivalent), minimum pressure rating of 100 psi (7 bar). The customer is required to make all hose connections.1.Improperly regulated, pulsating, or poor quality purge gas may cause irregular or erratic instrument operation. Containment of exhaust is recommended if noxious or poisonous gases are released by sam-ple when heated. Venting inert gases into small rooms may reduce the oxygen content of the air andbecome hazardous to personnel.Cooling water and drain:Minimum inlet pressure: 40 psi 2, 3; Maximum inlet pressure: 80 psiNominal flow rate: 1–2 L/min (varies with inlet pressure)Minimum inlet/outlet differential pressure (if plant-wide recirculation is used): 50 psiWater temperature: 15°C–30°C 4, optimum 20°CAir temperature/relative humidity: non-condensing at 15°CThe instrument is supplied with two hoses to connect to the Coolant Inlet and Outlet ports on the back of the furnace. The other end of each hose has a 1/4” male fitting. The coolant source must be connected to the 1/4” male fittings of each hose. The customer is required to make all hose connections.2.Operating close to or below minimum pressure will result in erratic operation3.Wall mounted supply shutoff, open drain, and city water required; 2 meters feed and drain hose supplied that may be used in place of hose barbs, if applicable.4.Excessively cold water may result in “sweating” and possible corrosion of cooled metal surfaces. Warm water may not permit beginning a test at 25°C or lower.Dimensions:Depth 183 cm (60 in), Width 76 cm (30 in), Height 153 cm (60 in)Weight:160 kg (350 lbs)Additional Power requirements:Main Furnace power supply voltage: 208-240 V AC (rated for 100A), 50/60 HzV oltage taps may be changed to 208V or 240V , if necessaryPump: 220–240 V AC outlet (rated for 15A) (separate)Laboratory requirements:Liquid Nitrogen: 1 L/day in a small hand-held dewar flask, manually poured into the LN 2 receptacle.Purge gas 1, 2: Argon is required (supplied by customer); preferred source: gas cylinder. Two stage regulator with second stage: 40–50 psi maximum rating required. Maximum delivery pressure: 45 psi. Operation in air or moisture bearing atmosphere is not permitted.Hose (supplied by customer): Rubber/Tygon tubing, minimum inside tubing diameter of 6 mm (or equivalent), minimum pressure rating of 100 psi (7 bar). The customer is required to make all hose connections.1.The instrument has provision for the collection of the effluent purge gas, which may be collected and piped away at the customer's option. Containment of the exhaust is recommended if noxious or poison-ous gases are released by the specimen when heated.2.Improperly regulated, pulsating, or poor quality purge gas may cause irregular or erratic equipment oper-ation. Containment of exhaust is recommended if noxious or poisonous gases are released by sample when heated. Venting inert gases into small rooms may reduce the oxygen content of the air and become hazardous to personnel.Cooling water and drain:Minimum inlet pressure to the instrument: 40 psi; Maximum inlet pressure 80 psiNominal flow rate: 4–5 L/min. Varies with inlet pressurePermissible water temperature 15°C to 30°C, optimum 20°C.Air temperature/relative humidity: non-condensing at 15°C.Vacuum (supplied by customer; required)Mechanical vacuum pump: Minimum 100 lpm, 10-3 torr (port blanked off)TA Instruments OfficesFor information on our latest products, contact information, and more, see our web site at: TA Instruments — Waters LLCCorporate Headquarters159 Lukens DriveNew Castle, DE 19720USATelephone: 302-427-4000Fax: 302-427-4001Email:**********************。
热影响区宽度的英文
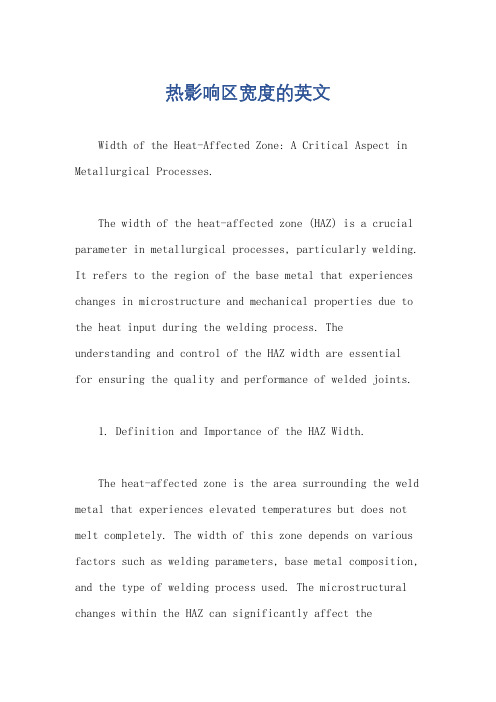
热影响区宽度的英文Width of the Heat-Affected Zone: A Critical Aspect in Metallurgical Processes.The width of the heat-affected zone (HAZ) is a crucial parameter in metallurgical processes, particularly welding. It refers to the region of the base metal that experiences changes in microstructure and mechanical properties due to the heat input during the welding process. The understanding and control of the HAZ width are essentialfor ensuring the quality and performance of welded joints.1. Definition and Importance of the HAZ Width.The heat-affected zone is the area surrounding the weld metal that experiences elevated temperatures but does not melt completely. The width of this zone depends on various factors such as welding parameters, base metal composition, and the type of welding process used. The microstructural changes within the HAZ can significantly affect themechanical properties of the welded joint, including its strength, toughness, and corrosion resistance.2. Factors Influencing the HAZ Width.Welding Parameters: The welding current, voltage, and welding speed significantly affect the heat input and, consequently, the width of the HAZ. Higher heat input results in a wider HAZ.Base Metal Composition: The chemical composition of the base metal determines its thermal conductivity, heat capacity, and phase transformation temperatures. These properties influence how the metal responds to the heat input and, therefore, the width of the HAZ.Welding Process: Different welding processes, such as gas metal arc welding (GMAW), gas tungsten arc welding (GTAW), and submerged arc welding (SAW), have different heat distributions and, thus, affect the HAZ width.3. Impact of the HAZ Width on Welded Joint Properties.Mechanical Properties: The microstructural changes within the HAZ can lead to variations in hardness, ductility, and strength. If the HAZ width is too wide, it can result in a decrease in joint strength and an increase in the risk of cracks.Corrosion Resistance: Changes in the microstructure of the HAZ can affect the corrosion resistance of the welded joint. Wider HAZs may exhibit increased susceptibility to corrosion.Fatigue Resistance: The HAZ width can influence the fatigue life of welded structures. Wider HAZs may introduce stress concentrations and reduce fatigue resistance.4. Controlling the HAZ Width.Selection of Welding Parameters: Careful selection of welding parameters can help limit the heat input and, thus, the width of the HAZ. Adjusting the welding current, voltage, and speed according to the base metal and desiredjoint properties is crucial.Preheat and Postweld Heat Treatment: The application of preheat and postweld heat treatment can control the cooling rate and minimize the risk of harmful microstructural changes within the HAZ.Use of Filler Materials: The selection of appropriate filler materials can influence the microstructural development within the HAZ and, thus, the width.5. Conclusion.The width of the heat-affected zone is a critical aspect in welding and other metallurgical processes. Understanding and controlling this parameter is essential for ensuring the quality and performance of welded joints. By considering factors such as welding parameters, base metal composition, and welding process, it is possible to optimize the HAZ width and achieve optimal welded joint properties.。
Thermal_Forming
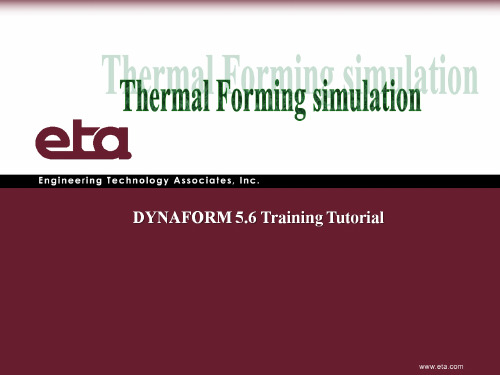
Figure 22
13
IV. Define Blank Structural Material
6. The stress/strain curve at 0 degree is imported. The result is shown in Figure 23. 7. Then Click Ok to accept the result. The first curve definition is displayed with valid. See Figure 24.
Figure 16 Figure 17
10
IV. Define Blank Structural Material
9. Repeat the above procedures (step 4-step 8) – Select LCPR-Poisson.cur as Poisson’s Ratio; – Select LCALPHA-Expansion.cur as Thermal Exp (ALPHA); – Select LCC-C.cur as the Viscous Para.C; – Select LCC-P.cur as the Viscous Para.P. – The result is shown in Figure 18.
Figure 4 Figure 6 Figure 5
4
III. Define Element Formulation
1. Click the button below Property (Figure 7) to define the element formulation as 16.Fully Integrated (Figure 8).
The interstellar medium in the edge-on galaxy NGC 5907
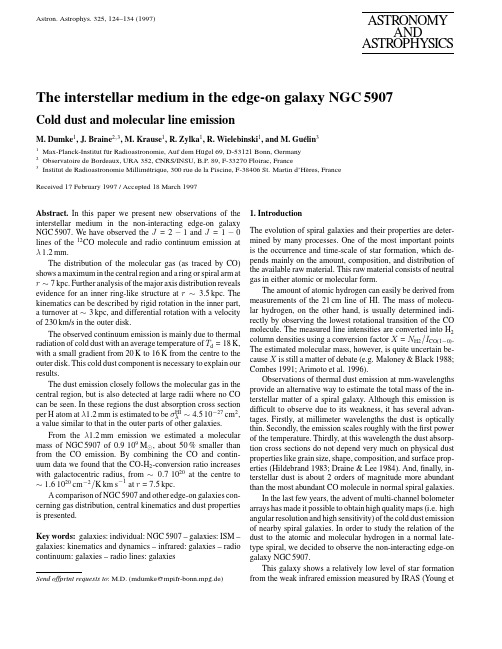
Astron.Astrophys.325,124–134(1997)ASTRONOMYANDASTROPHYSICSThe interstellar medium in the edge-on galaxy NGC5907Cold dust and molecular line emissionM.Dumke1,J.Braine2,3,M.Krause1,R.Zylka1,R.Wielebinski1,and M.Gu´e lin31Max-Planck-Institut f¨u r Radioastronomie,Auf dem H¨u gel69,D-53121Bonn,Germany2Observatoire de Bordeaux,URA352,CNRS/INSU,B.P.89,F-33270Floirac,France3Institut de Radioastronomie Millim´e trique,300rue de la Piscine,F-38406St.Martin d’H`e res,FranceReceived17February1997/Accepted18March1997Abstract.In this paper we present new observations of the interstellar medium in the non-interacting edge-on galaxyNGC5907.We have observed the J=2−1and J=1−0lines of the12CO molecule and radio continuum emission atλ1.2mm.The distribution of the molecular gas(as traced by CO)shows a maximum in the central region and a ring or spiral arm atr∼7kpc.Further analysis of the major axis distribution reveals evidence for an inner ring-like structure at r∼3.5kpc.Thekinematics can be described by rigid rotation in the inner part,a turnover at∼3kpc,and differential rotation with a velocityof230km/s in the outer disk.The observed continuum emission is mainly due to thermalradiation of cold dust with an average temperature of T d=18K,with a small gradient from20K to16K from the centre to theouter disk.This cold dust component is necessary to explain ourresults.The dust emission closely follows the molecular gas in thecentral region,but is also detected at large radii where no COcan be seen.In these regions the dust absorption cross sectionper H atom atλ1.2mm is estimated to beσHIλ∼4.510−27cm2, a value similar to that in the outer parts of other galaxies.From theλ1.2mm emission we estimated a molecularmass of NGC5907of0.9109M ,about50%smaller thanfrom the CO emission.By combining the CO and contin-uum data we found that the CO-H2-conversion ratio increaseswith galactocentric radius,from∼0.71020at the centre to ∼1.61020cm−2/K km s−1at r=7.5kpc.A comparison of NGC5907and other edge-on galaxies con-cerning gas distribution,central kinematics and dust propertiesis presented.Key words:galaxies:individual:NGC5907–galaxies:ISM–galaxies:kinematics and dynamics–infrared:galaxies–radio continuum:galaxies–radio lines:galaxiesSend offprint requests to:M.D.(mdumke@mpifr-bonn.mpg.de)1.IntroductionThe evolution of spiral galaxies and their properties are deter-mined by many processes.One of the most important pointsis the occurrence and time-scale of star formation,which de-pends mainly on the amount,composition,and distribution ofthe available raw material.This raw material consists of neutralgas in either atomic or molecular form.The amount of atomic hydrogen can easily be derived frommeasurements of the21cm line of HI.The mass of molecu-lar hydrogen,on the other hand,is usually determined indi-rectly by observing the lowest rotational transition of the COmolecule.The measured line intensities are converted into H2column densities using a conversion factor X=N H2/I CO(1−0). The estimated molecular mass,however,is quite uncertain be-cause X is still a matter of debate(e.g.Maloney&Black1988;Combes1991;Arimoto et al.1996).Observations of thermal dust emission at mm-wavelengthsprovide an alternative way to estimate the total mass of the in-terstellar matter of a spiral galaxy.Although this emission isdifficult to observe due to its weakness,it has several advan-tages.Firstly,at millimeter wavelengths the dust is opticallythin.Secondly,the emission scales roughly with thefirst powerof the temperature.Thirdly,at this wavelength the dust absorp-tion cross sections do not depend very much on physical dustproperties like grain size,shape,composition,and surface prop-erties(Hildebrand1983;Draine&Lee1984).And,finally,in-terstellar dust is about2orders of magnitude more abundantthan the most abundant CO molecule in normal spiral galaxies.In the last few years,the advent of multi-channel bolometerarrays has made it possible to obtain high quality maps(i.e.highangular resolution and high sensitivity)of the cold dust emissionof nearby spiral galaxies.In order to study the relation of thedust to the atomic and molecular hydrogen in a normal late-type spiral,we decided to observe the non-interacting edge-ongalaxy NGC5907.This galaxy shows a relatively low level of star formationfrom the weak infrared emission measured by IRAS(Young etM.Dumke et al.:The interstellar medium in the edge-on galaxy NGC5907125al.1989).Therefore it is–together with NGC4565observedby Neininger et al.(1996)–some kind of counterpart to themore active galaxies already observed in the radio continuumat mm-wavelengths:NGC891(Gu´e lin et al.1993),NGC3079(Braine et al.1997),NGC3627(Sievers et al.1994),NGC4631(Braine et al.1995),and M51(Gu´e lin et al.1995).NGC5907was observed in the HI-line twenty years ago andwas thefirst non-interacting galaxy where a galactic warp couldbe detected(Gu´e lin et al.1974;Sancisi1976).Former CO mea-surements(Sofue1994;Garc´ıa-Burillo&Gu´e lin1995)haveshown that this galaxy is relatively weak in CO.Although itsnearly edge-on orientation of i∼86◦.5does not allow us to ob-serve the emission of individual star forming regions or spiralarms in the disk,this inclination increases the column densityalong the line of sight to a significant and easier measurablevalue.Therefore NGC5907is a good candidate to study the ra-dial distribution of the dust and its correlation with the molecularand atomic hydrogen distributions.This will be done especiallyin comparison with the other non-interacting edge-on galaxiesmentioned above,namely NGC891and NGC4565.The latterone shows an even lower star formation activity than NGC5907and is also CO-weak,whereas NGC891contains a considerableamount of molecular gas and shows a relatively high level ofstar formation.These two galaxies are classified as Sb and Sbc,respectively,in contrast to the Sc-galaxy NGC5907.Furthermore edge-on galaxies are suitable to investigate thethickness of the gas and dust layer.Since NGC5907is lackingstrong star formation,one does not expect to detect a thick diskor halo in this galaxy because star formation and the existenceand structure of a gaseous halo seem to be directly connected(e.g.Dahlem et al.1995).In this paper we present our molecular line and bolometerobservations of NGC5907.The following section describes theobservations and the data reduction.Sect.3presents the resultswe obtained from the CO observations.In Sect.4we discussthe thermal dust emission.We estimate dust temperatures andabsorption cross sections,and compare the distribution of thecold dust with that of the atomic and molecular gas.From this weget some hints on how the CO-H2-conversion factor may vary ingalactic disks.In Sect.5the results for NGC5907are comparedwith those obtained for other edge-on galaxies,and thefinalsection gives a summary of our results and some concludingremarks.Table1lists some basic parameters of NGC5907which willbe used throughout this paper.2.Observations and data reductionThe observations presented here were all made with the30mtelescope of the Institut de Radio Astronomie Millim´e trique(IRAM),located on Pico Veleta(Spain).2.1.Molecular line observationsThe observations of the12CO(1−0)and the12CO(2−1)lineswere done in May1995and July1996.We used one3mm(two Table1.Some basic parameters of NGC5907Type Sc(de Vaucouleurs et al.1991)λ1.2mm centre:(this work)R.A.[1950]15h14m35s.7Dec.[1950]56◦30 37 .0Dynamical centre:(Garc´ıa-Burillo et al.1997)R.A.[1950]15h14m35s.5Dec.[1950]56◦30 43 .3v hel677km/s(Garc´ıa-Burillo et al.1997) Distance11Mpc(Sasaki1987)(1 corresponds to53pc)Pos.Angle155◦(Barnaby&Thronson1992)Incl.86.5◦(Garc´ıa-Burillo et al.1997)during the second observing period)and two1mm SIS receivers available at the30m telescope simultaneously.The receivers were tuned for image sideband rejections≥10dB(≥30dB at115GHz).The system temperatures were300-400K at 115GHz and500-700K at230GHz(in the T∗A scale).In the following we use main-beam line brightness temperatures T mb. These are converted from the antenna temperatures,corrected for atmospheric attenuation and rear spillover,T∗A,through T mb=T∗A/ηmb.The beam efficienciesηmb=B eff/F eff are0.73 and0.45for115and230GHz,respectively.The beamwidth was measured on Mars to be21 for the12CO(1−0)line and 11 for the12CO(2−1)line.The backends used consisted of two512×1MHz channelfilter banks,connected to one3mm and one1mm receiver,and an autocorrelator unit,connected to the other1mm receiver(and the other3mm receiver in July 1996).The observations were centered on the major axis of the galaxy.Adopting the central position from the bolometer ob-servations(given in Table1),we observed several points out toa projected radius of240 with12 spacings near the centre and24 spacings further out.Additionally we observed a few points above and below the major axis at distances of12 and24 from the plane.The observations were made by wobbling the subre-flector at a rate of0.5Hz between the source and a reference position located between±2 and±4 in azimuth(depending on the observing position and the orientation of the source).Some scans at larger radii were observed in the position-switching mode with on-and off-position located symmetrically around the center.Cold load calibrations were made every4-8minutes.During the CO observations we checked the pointing ac-curacy in two different ways.Firstly,we made pointing scans towards1641+399and1418+546every1-2hours.Sec-ondly,we measured(every∼2hours)small cuts perpendic-ular to the major axis at the center,consisting of three points at z=0 ,+12 ,and−12 ,and checked their symmetry,since the central point is expected to be strongest and the intensity of both off-axis points to be roughly equal.From these cuts and the pointing corrections made after each pointing scan we conclude that the mean pointing uncertainty is∼1 .5.The data reduction was done in a standard manner using the GILDAS software package.126M.Dumke et al.:The interstellar medium in the edge-on galaxy NGC59072.2.Bolometer observationsTheλ1.2mm observations were carried out in March1995withthe19-channel bolometer array developed at the Max-Planck-Institut f¨u r Radioastronomie,Bonn.The19channels are lo-cated in the centre and on the sides of two concentric regu-lar hexagons,with a spacing between two adjacent channels(beams)of20 .The central frequency and bandwidth of thebolometer are estimated to be245GHz and70GHz respec-tively(Gu´e lin et al.1995).For calibration purposes we haveobserved maps of Mars and Uranus during the bolometer obser-vations.These maps yielded a conversion factor from observedcounts to mJy/beam area of0.32mJy(beam area)−1count−1.The beamwidth at this frequency is∼10 .7.The continuum maps of NGC5907were observed in the Az-El coordinate system,with a scanning speed of4 /s in Azimuthwith data-acquisition every2 ,and a subscan separation of4in elevation.During the observations,the subreflector was wob-bled at2Hz in azimuth,with a beam throw of1 .The startingpoint of each subscan was shifted a few arcseconds in azimuthwith respect to the preceding one,which leads to a skewed shapeof each single coverage in the Az-El space,with two edges ofthe maps parallel to the major axis of the galaxy.This as wellas the use of different map sizes(between330 ×100 and 250 ×180 )was done in order to ensure that each subscan covers the galaxy and at least90 of blank sky on either side.We observed a total offifteen single maps of NGC5907,fivecentered on the optical centre(Barnaby&Thronson1992),theothers shifted164 along the major axis to the northwest andsoutheast,respectively.Since the optical centre and the centre oftheλ1.2mm emission(as found by our observations)differ bya few arcseconds,all offsets throughout this paper are relativeto the latter position which is given in Table1.During the bolometer observing session the pointing accu-racy was checked every1-2hours on1418+546.The pointingcorrections were always smaller than3 .The atmosphere wasrelatively stable and the sky opacity was∼0.2most of the time(always smaller than0.3).NGC5907was observed at relativelyhigh elevations(55◦-70◦)what reduces possible calibration er-rors,which are typically of the order15%.The data reduction was done with the MOPS software.Asecond order baseline wasfitted to each individual scan in az-imuth direction.Thefinal restoration was done applying the“mask-and-shift”restoring method,as outlined in the“PocketCookbook”(Zylka1996).3.Molecular gas in NGC59073.1.Observational results and kinematicsThe spectra along the major axis of the galaxy obtained duringthe molecular line observations are shown in Fig.1.The max-imum peak temperatures of∼0.33K and∼0.36K for theCO(1−0)and the(2−1)line respectively are reached near thecenter.CO is detected up to radii of more than200 (∼11kpc).A striking feature of many of the spectra(r≤80 )is thatthe observed lines contain at least two components,and thatthe Fig.1.Maps of the observed12CO(1−0)(left)and12CO(2−1)(right) major axis spectra of NGC5907,smoothed to a velocity resolution of 10.4km/s.The scale of the spectra is indicated by the small box at the bottom.Offsets are along the major axis,north is negativecentral spectrum shows a clear asymmetry.A natural explana-tion for the latter is that the location of the dynamical centre of the galaxy is not at x=0 (the centre of theλ1.2mm emission), but shifted by a few arcseconds to the northwest.This idea is also supported by the small asymmetry visible in the position-velocity diagrams(Fig.2).Garc´ıa-Burillo et al.(1997),who observed the central region of NGC5907using the Plateau de Bure interferometer,found the dynamical centre of the galaxy atα1950=15h14m35.s5,δ1950=56◦30 43. 3.This corresponds to(x,z)=(−6. 4,+1. 2)in our coordinates.They also found a small offset between the dynamical centre of the galaxy and theM.Dumke et al.:The interstellar medium in the edge-on galaxy NGC 5907127Fig. 2.Position-velocity diagram of the 12CO(1−0)(left)and the 12CO(2−1)(right)observations parallel to the major axis at z =0.Contour levels are -0.04(dashed),0.04,0.08,0.12,...,0.4K for both tran-sitions.The velocity resolution is 20km/s.The rms noise is variable along the major axis with a typical value of about 30mK for both transitions.The thick lines indicate the rotation curve as described in the text-200-100100200radius [arcsec]0204060d e p r o j e c t e d C O i n t e n s i t y [a .u .]-200-100100200x offset [arcsec]10203040I C O [K k m /s]Fig.3.a Observed (dashed line and filled black circles)and modelled (solid line)12CO(1−0)intensity distribution along the major axis of NGC 5907.The modelled distribution is obtained by a least-squares-fit and results from the line-of-sight-integrated radial distribution shown in b .b Adopted radial CO profile which leads to the major axis distribution as shown in aposition of the maximum CO intensity,which is in agreement with our results.The kinematics of NGC 5907can basically be described by rigid rotation up to a radius of ∼55 (which corresponds to ∼3kpc),followed by differential rotation with a rotational ve-locity of 230km /s.These values are relative to the systemic ve-locity of 677km /s and the dynamical centre given in Tab.1.The rotation curve follows from this work and the HI data (Caser-tano 1983)and is plotted as a thick line on the position-velocity diagrams (Fig.2).There are,however,a few deviations from this simple behaviour.A second line component is visible in the spectra near the central region (at |x |<30 ).Here the rigid (“normal”)rotation of the inner disk is accompanied by a high-velocity wing,with a much larger velocity gradient.This component is visible in the individual spectra as well as in the p -v -diagrams,and leads to a total line width of about 350km/s atthe assumed central position.It most probably results from non-circular motions due to a bar,as already suggested by Garc´ıa-Burillo &Gu´e lin (1995)and Garc´ıa-Burillo et al.(1997).3.2.Radial gas distributionThe observed major axis distribution of the CO intensity is the sum of the radial distribution and a projection effect (by which the emission at several radii is projected onto a certain position on the major axis).In order to deproject this distribution,we as-sumed a radial model function,consisting of a central Gaussian peak and two Gaussian rings,and fitted the resulting major axis distribution to the data.The existence of two ring-like struc-tures is suggested by two intensity maxima (at x ∼±60 and x ∼±120 )on either side of the centre in the p -v -diagrams.128M.Dumke et al.:The interstellar medium in the edge-on galaxy NGC5907Fig.4.Contour map of the continuum emission of NGC 5907at 245GHz,overlaid onto an optical image extracted from the Digitized Sky Survey.The beam size of 15 is indicated by the filled white circle in the lower right corner.The rms noise depends on the location in the map;on the galaxy it is about 1.5mJy/beam area,contour levels are 4,8,...,24mJy/beam area.The thin black lines indicate the λ1.2mm centre and the major axis of the galaxy as given in Table 1Additionally this distribution with two rings fits the data better than a distribution with just one ring (at r ∼120 ).The result can be seen in Fig.3.The best fit was obtained for ring radii of r 1=3.7kpc and r 2=7.0kpc and widths (FWHM)of Θpeak =4.4kpc,Θring1=1.4kpc,and Θring2=2.3kpc for the central peak and the inner and outer ring respectively.However,the outer “ring”may in fact be spiral arms seen more or less tangentially.4.Cold dust in NGC 59074.1.Observational resultsA contour map of the λ1.2mm continuum emission,overlaid onto an optical image extracted from the Digitized Sky Survey,is shown in Fig.4.This λ1.2mm-map is already smoothed to a beamsize of 15 to improve the signal-to-noise ratio.The emission is concentrated along a narrow ridge which follows closely the dusty optical disk,but is less extended,per-haps because of the sensitivity limit of our data.Although the emission is enhanced near the centre,there is no evidence for a nuclear point source.Several local maxima are visible along the major axis,but with some difference between the northern and the southern half.Whereas in the north there are three separatepeaks at projected radii of about 1 ,2 ,and 3.5,they seem to be somehow smeared out in the southern half,except the one atx ∼3 .5.In Fig.5we show the λ1.2mm continuum map,smoothed to a resolution of 21 HPBW,together with an HI total intensity map as received from Sancisi (m.),and the positions observed in the CO lines.NGC 5907is a really exemplary galaxy for the existence of galactic warps in neutral hydrogen (Sancisi 1976).It is,moreover,the first “normal”galaxy where a warp in the outer disk was observed.But in contrast to NGC 4565,another normal edge-on galaxy recently observed at λ1.2mm (Neininger et al.1996),no indication for a warp of the ther-mal dust emission can be seen (although the northernmost peak seems to be slightly shifted westwards with respect to the major axis).This may of course be due to the decreasing sensitivity at the outer edges of our dust map,which we reach at radii of ∼300 ,where the HI-warp is only marginally detected.4.2.ISM distributions along the major axisFig.6shows the distribution of the λ1.2mm continuum emis-sion along the major axis,together with the line intensities of the 12CO(1−0)and the HI emission.The spatial resolution of all three data sets is 21 ,as given by the 12CO(1−0)data.The continuum emission shows (more clearly in this plot than in the maps)the existence of two bright maxima at the end of the emission ridge (x ∼±200 )and of two less pronounced ones at x ∼±120 ,even if the southeastern one seems to be smeared out.Besides these similarities between the northern and the southern half the distribution is slightly asymmetric on smaller scales.The emission is detected up to radii of ∼250 in the south and even further,up to ∼280 ,in the northern half (with a significance of 2σ).Since there is λ1.2mm continuum emission beyond the edge of the CO disk,dust associated with the atomic component makes a significant contribution to the 1.2mm flux.The distribution of the CO line-intensities (and therefore the column densities of the molecular gas)shows also a maximum in the central region and decreases with increasing distance from the centre.Two further maxima are apparent at x ∼±120 .These features may be due to molecular rings and/or spiral arms in the inner part of the disk.The HI distribution shows a different behaviour.It has a minimum near the centre,increases at x ≤80 ,stays then roughly constant with several local peaks up to x ∼±200 ,and drops again further outwards.Hence this component is much more extended than the molecular gas in this galaxy.If we compare the dust emission with both gas phases,we find that it correlates with the molecular gas in the inner part ofM.Dumke et al.:The interstellar medium in the edge-on galaxy NGC5907129Fig.5.Contour maps of theλ1.2mm con-tinuum emission(left)and the HI line emis-sion(middle),as well as positions observed in the CO lines(right),all at the same scale and aligned in declination.The spatial reso-lution of the two maps is21 .Contour lev-els are3,7,12,18,25,33mJy/beam area for theλ1.2mm map and8,15,25,40,55, 701020atoms cm−2for the HI mapthe disk.At large radii,on the other hand,where CO is no longer detected,it seems to follow the HI emission.This result con-firms qualitatively that for NGC4565of Neininger et al.(1996). At smaller scales,wefind the two outer peaks in the dust emis-sion at x∼±200 corresponding to local maxima in the HI distribution,although there is a small displacement,especially on the southeastern side.From both CO peaks at x∼±120 only the northwestern one has a clear counterpart in the dust distribution,whereas in the southeastern half the dust emission shows just a small enhancement at this radius.4.3.Disk thicknessGarc´ıa-Burillo et al.(1997)estimated from their Plateau de Bure observations of the central region of NGC5907an inclina-tion of86.5◦and a thickness of the molecular disk of≤3 .In or-der to check if this is in agreement with our observations,wefit-ted the observed z-distribution(the averaged spectra are shown in Fig.7)with a Gaussian profiing the beamwidths given in Sect.2.1we determined a deconvolved thickness(FWHM) of the CO emission ridge of(13±5) ,somewhat thicker than in NGC4565(Neininger et al.1996).Additional off-axis ob-servations at x=60 have shown that this apparent thickness is nearly constant along the major axis.In order to estimate the extent of the atomic gas and the thermal dust emission perpen-dicular to the plane,we performed cuts along the minor axis of both maps.These lead to a mean beam deconvolved FWHM of the emission of(47±4) for the HI and(16±4) for the λ1.2mm emission.Since the galaxy is not perfectly seen edge-on,but under an inclination of i=86.5◦,a particular fraction of the off-axis emission is just projected from large radii to large z.We modelled this emission,using the radial CO profile obtained in Sect.3.2and a similar model,consisting of three rings,for the HI emission.The best agreement between model and data is found for a disk with a thickness(FWHM)of8 (which corresponds to∼400pc)for the CO and of28 (∼1.5kpc)for the HI.Both values seem to be unexpectedly large for a non-interacting spiral with only moderate star forming activity.We should note,however,that it is difficult to account for a warp in this simple modelling,and the results are very sen-sitive to the exact values of the inclination and the telescope beamwidth.Therefore,due to the large uncertainties,a thin molecular disk cannot be ruled out.4.4.Dust properties4.4.1.Non-dust contributions to the observedfluxUsing a ring integration method,we have determined the total flux density atλ1.2mm and found S1.2mm=605±55mJy.This value,however,cannot be attributed to thermal dust radiation alone.The broad band emission measured with the bolome-ter at245GHz rather consists of several components:thermal dust emission,free-free radiation,synchrotron radiation,and the CO(2−1)and some weaker lines.Since we are mainly in-terested in thefirst,we have to determine the contributions due to the other processes and to subtract them.The contribution of the12CO(2−1)line to the surface brightness measured with the bolometer can be calculated throughF line=2kν3c−3∆νbolΩbeam I CO(2−1)≈0.058I CO(2−1)(1) (with I CO(2−1)=lineT mb(12CO(2−1))dv in Kkms−1)for a bolometer bandwidth of70GHz and a beamwidth of11 for the continuum observations.With an assumed contribution of other isotopes and lines from other molecules of about10%of 12CO we estimate a totalflux density due to line contributions of S line=52±4mJy.The contribution of the continuum emission due to thermal and relativistic electrons is more difficult to determine since the130M.Dumke et al.:The interstellar medium in the edge-on galaxy NGC 5907300200100-100-200-300x offset [arcsec]010203040I C O [K k m /s ]SENW403020100I 1.2mm [mJy/b.a.]HI1.2 mmCO(1-0)Fig.6.The distribution of the 12CO(1−0)(thick dashed line,left scale)and the λ1.2mm continuum (thick solid line,right scale)along the major axis of NGC 5907.The HI distribution (thin solid line,arbi-trary units)is also shown.The spatial resolution in all three curves is 21radio continuum flux density at ν=1−10GHz originates partly in a double background source in the southern half of the galaxy (Hummel et al.1984;Dumke et al.1995).Furthermore the spectral behaviour of this background source is unknown,and the fraction of the galaxies’thermal emission is difficult to estimate.We used a total flux density of 47±5mJy at a frequency of 10.55GHz as derived by Dumke et al.(1995),a thermal fraction of 30%at this frequency and a nonthermal spectral index of −0.85which are typical for spiral galaxies (Niklas et al.1997)to calculate a value of S sync+ff =13±4mJy at 245GHz,which is about 2%of the total flux density.Besides these integrated values,we had to estimate the non-dust contributions along the major axis.The fraction of the CO lines,i.e.the line-to-continuum ratio,was calculated for each position from the CO(2−1)line following Eq.1and subtracted.Again we assumed that a fraction of 10%of the 12CO(2−1)-line stems from other lines in the bolometer band.For the free-free and synchrotron emission,we subtracted a fraction of 2%at each position,in accordance with the value estimated above.4.4.2.Dust temperaturesAfter subtracting the contributions of molecular lines and of synchrotron and free-free radiation,we determined a total flux density at 245GHz due to thermal dust emission ofS dust =540±60mJy .Including published IRAS flux densities (Young et al.1989)our observations allow to estimate color temperatures for the dust.We fitted a two-component modified Planck function to the data,using the points from 25µm to 1.2mm and under the assumption of a dust spectral index of 2(e.g.Chini et al.1986).The observed spectrum and the fitted curves (as well as their sum)are shown in Fig.8.The estimated temperatures for the two components to which we refer as cold and warm dust are 18K and 54K respectively.This result shows thatcoldFig.7.Averaged 12CO(1−0)and (2−1)spectra of the cuts through the centre.The portion of the spectra shown in each box ranges from v hel =300km /s to v hel =1000km /s and from T mb =−0.1K to T mb =0.4Kdust is necessary to explain the thermal continuum emission at λ1.2mm.The warmer dust alone which can be detected in the far-infrared by IRAS is not sufficient to account for the strong mm-emission and to explain our data.Although NGC 5907is a relatively inactive galaxy which does not show any signs of remarkable star forming activity (e.g.Dumke et al.1995),the dust emission is slightly enhanced at smaller galactocentric radii,and the dust may be somewhat warmer in this region.The FIR emission of NGC 5907was mapped by Wainscoat et al.(1987),using the IRAS CPC in-strument,at λλ50and 100µm with a resolution of 75 and 89 respectively.These maps were used to obtain spectra at different positions along the major axis of NGC 5907.We found that the temperature of the cold dust is somewhat higher in the central region (∼20K)than the value we got from the integrated flux densities,and drops to ∼16K at the outer disk.A similar de-crease is also found for other normal disk galaxies like NGC 891(Gu´e lin et al.1993),NGC 4565(Neininger et al.1996),or our Milky Way (Cox &Mezger 1989).。
atom-ZXXX
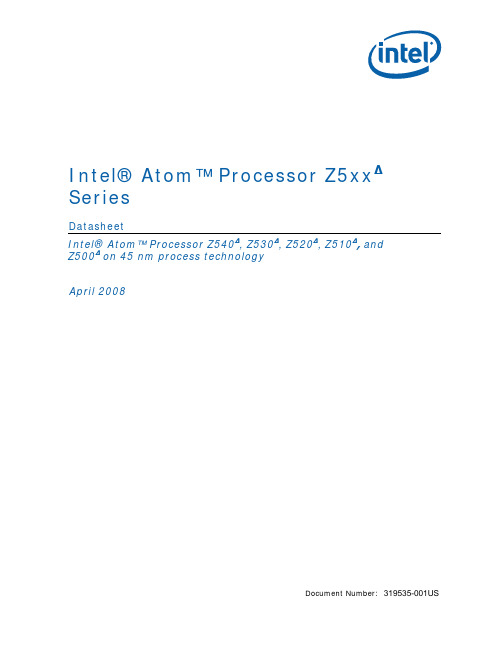
Intel® Atom™ Processor Z5xx∆SeriesDatasheetIntel® Atom™ Processor Z540∆, Z530∆, Z520∆, Z510∆, andZ500∆on 45 nm process technologyApril 2008Document Number: 319535-001USINFORMATION IN THIS DOCUMENT IS PROVIDED IN CONNECTION WITH INTEL® PRODUCTS. NO LICENSE, EXPRESS ORIMPLIED, BY ESTOPPEL OR OTHERWISE, TO ANY INTELLECTUAL PROPERTY RIGHTS IS GRANTED BY THIS DOCUMENT. EXCEPTAS PROVIDED IN INTEL'S TERMS AND CONDITIONS OF SALE FOR SUCH PRODUCTS, INTEL ASSUMES NO LIABILITYWHATSOEVER, AND INTEL DISCLAIMS ANY EXPRESS OR IMPLIED WARRANTY, RELATING TO SALE AND/OR USE OF INTELPRODUCTS INCLUDING LIABILITY OR WARRANTIES RELATING TO FITNESS FOR A PARTICULAR PURPOSE, MERCHANTABILITY,OR INFRINGEMENT OF ANY PATENT, COPYRIGHT OR OTHER INTELLECTUAL PROPERTY RIGHT. Intel products are not intendedfor use in medical, life saving, or life sustaining applications.Intel may make changes to specifications and product descriptions at any time, without notice.Designers must not rely on the absence or characteristics of any features or instructions marked “reserved” or “undefined.” Intelreserves these for future definition and shall have no responsibility whatsoever for conflicts or incompatibilities arising fromfuture changes to them.The Intel® Atom™ processor Z5xx∆ series may contain design defects or errors known as errata which may cause the product todeviate from published specifications. Current characterized errata are available on request.∆Intel processor numbers are not a measure of performance. Processor numbers differentiate features within each processorfamily, not across different processor families. Over time processor numbers will increment based on changes in clock, speed,cache, FSB, or other features, and increments are not intended to represent proportional or quantitative increases in anyparticular feature. Current roadmap processor number progression is not necessarily representative of future roadmaps. See/products/processor_number for details.Intel® Virtualization Technology requires a computer system with an enabled Intel® processor, BIOS, virtual machine monitor(VMM) and, for some uses, certain platform software enabled for it. Functionality, performance or other benefits will varydepending on hardware and software configurations and may require a BIOS update. Software applications may not becompatible with all operating systems. Please check with your application vendor.Hyper-Threading Technology (HT Technology) requires a computer system with a processor supporting Hyper-ThreadingTechnology and HT Technology enabled chipset, BIOS and operating system. Performance will vary depending on the specifichardware and software you see. See /technology/ hypertheading/ for more information including details onwhich processor supports HT Technology.Intel, Intel Atom, Intel SpeedStep, Intel Core 2 Duo, and the Intel logo are trademarks of Intel Corporation in the U. S. andother countries.*Other names and brands may be claimed as the property of others.Copyright © 2008 Intel Corporation. All rights reserved.2 DatasheetContents1Introduction (7)1.1Major Features (7)1.2Terminology (8)1.3References (10)2Low Power Features (11)2.1Clock Control and Low-power States (11)2.1.1Package/Core Low-Power State Descriptions (13)2.1.1.1Normal State (C0, C1) (13)2.1.1.1.1C1/AutoHalt Powerdown State (13)2.1.1.1.2C1/MWAIT Powerdown State (14)2.1.1.2C2 State (14)2.1.1.2.1Stop-Grant State (14)2.1.1.2.2Stop-Grant Snoop State (15)2.1.1.3C4 State (15)2.1.1.3.1Sleep State (15)2.1.1.3.2Deep Sleep State (16)2.1.1.3.3Deeper Sleep State (16)2.1.1.3.4Intel® Atom™ Processor Z5xx Series C5 (17)2.1.1.4C6 State (17)2.1.1.4.1Intel® Deep Power-Down Technology State(Package C6 State) (18)2.2Dynamic Cache Sizing (20)2.3Enhanced Intel SpeedStep® Technology (21)2.4Enhanced Low-Power States (22)2.5FSB Low Power Enhancements (23)2.5.1Split V TT (23)2.5.2CMOS Front Side Bus (23)3Electrical Specifications (25)3.1FSB, GTLREF, and CMREF (25)3.2Power and Ground Pins (25)3.3Decoupling Guidelines (26)3.3.1V CC Decoupling (26)3.3.2FSB AGTL+ Decoupling (26)3.4FSB Clock (BCLK[1:0]) and Processor Clocking (26)3.5Voltage Identification and Power Sequencing (26)3.6Catastrophic Thermal Protection (29)3.7Reserved and Unused Pins (29)3.8FSB Frequency Select Signals (BSEL[2:0]) (29)3.9FSB Signal Groups (30)3.10CMOS Asynchronous Signals (31)3.11Test Access Port (TAP) Connection (31)3.11.1Maximum Ratings (31)3.12Processor DC Specifications (32)Datasheet 33.13AGTL+ FSB Specifications (41)4Package Mechanical Specifications and Pin Information (43)4.1Package Mechanical Specifications (43)4.1.1Processor Package Weight (43)4.2Processor Pinout Assignment (45)4.3Signal Description (52)5Thermal Specifications and Design Considerations (61)5.1Thermal Specifications (64)5.1.1Thermal Diode (64)5.1.2Intel® Thermal Monitor (66)5.1.3Digital Thermal Sensor (68)5.1.4Out of Specification Detection (69)5.1.5PROCHOT# Signal Pin (69)4 DatasheetFiguresFigure 1. Thread Low-Power States (12)Figure 2. Package Low-Power States (12)Figure 3. Deep Power-Down Technology Entry Sequence (18)Figure 4. Deep Power-Down Technology Exit Sequence (18)Figure 5. Exit Latency Table (19)Figure 6. Active V CC and I CC Loadline (36)Figure 7. Deeper Sleep V CC and I CC Loadline (37)Figure 8. Package Mechanical Drawing (44)Figure 9. Pinout Diagram (Top View, Left Side) (45)Figure 10. Pinout Diagram (Top View, Right Side) (46)TablesTable 1. References (10)Table 2. Coordination of Thread Low-Power States at the Package Level/Core Level..13Table 3. Voltage Identification Definition (27)Table 4. BSEL[2:0] Encoding for BCLK Frequency (29)Table 5. FSB Pin Groups (30)Table 6. Processor Absolute Maximum Ratings (32)Table 7. Voltage and Current Specifications for Intel® Atom™ Processor Z540,Z530, Z520, and Z510 (33)Table 8. Voltage and Current Specifications for Intel® Atom™ Processor Z500 (35)Table 9. FSB Differential BCLK Specifications (38)Table 10. AGTL+/CMOS Signal Group DC Specifications (39)Table 11. Legacy CMOS Signal Group DC Specifications (40)Table 12. Open Drain Signal Group DC Specifications (40)Table 13. Pinout Arranged By Signal Name (47)Table 14. Signal Description (52)Table 15. Power Specifications for Intel® Atom™ Processor Z540, Z530, Z520,and Z510 (62)Table 16. Power Specifications for Intel® Atom™ Processor Z500 (63)Table 17. Thermal Diode Interface (65)Table 18. Thermal Diode Parameters using Transistor Model (65)Datasheet 5Revision HistoryRevision Number Description RevisionDate-001 •Initial release April 2008§6 DatasheetIntroductionDatasheet 71 IntroductionThe Intel® Atom™ processor Z5xx series is built on a new 45-nanometer Hi-k low power micro-architecture and 45 nm process technology — the first generation of low-power IA-32 micro-architecture specially designed for the new class of Mobile Internet Devices (MIDs). In the Intel® Centrino® Atom™ processor technology platform, the Intel® Atom™ processor Z5xx series supports the Intel® System Controller Hub (Intel SCH), a single-chip component design for low-power. This document contains electrical, mechanical and thermal specifications for the following processors: • Intel® Atom™ processor Z540, Z530, Z520, Z510, and Z500Note: In this document, Intel® Atom™ processor Z5xx series refers to the Intel® Atom™processor Z540, Z530, Z520, Z510, and Z500 Note: In this document, the Intel® Atom™ processor Z5xx series is referred to as“processor”. The Intel System Controller Hub (Intel SCH) is referred to as “Intel SCH”.1.1 Major FeaturesThe following list provides some processor key features:• New single-core processor for mobile devices offering enhanced performance • On die, primary 32-kB instructions cache and 24-kB write-back data cache • 100-MHz and 133-MHz Source-Synchronous front side bus (FSB) ⎯ 100 MHz: Intel® Atom™ processor Z500⎯ 133 MHz: Intel® Atom™ processor Z540, Z530, Z520, and Z510 • Supports Hyper-Threading Technology 2-threads • On die 512-kB, 8-way L2 cache • Support for IA 32-bit architecture• Intel® Virtualization Technology (Intel® VT)• Intel® Streaming SIMD Extensions 2 and 3 (Intel® SSE2 and Intel® SSE3) and Supplemental Streaming SIMD Extensions 3 (SSSE3) support • Supports new CMOS FSB signaling for reduced power • Micro-FCBGA8 packaging technologies• Thermal management support using TM1 and TM2 • FSB Lane Reversal for flexible routing • Supports C0/C1(e)/C2(e)/C4(e)• Intel® Deep Power-Down Technology (C6) • L2 Dynamic Cache Sizing• New Split-V TT support for lowest processor power state• Advanced power management features including Enhanced Intel SpeedStep® Technology• Execute Disable Bit support for enhanced securityIntroduction8 Datasheet1.2 TerminologyTerm Definition#A “#” symbol after a signal name refers to an active low signal, indicating a signal is in the active state when driven to a low level. For example,when RESET# is low, a reset has been requested. Conversely, when NMI is high, a non-maskable interrupt has occurred. In the case of signals where the name does not imply an active state but describes part of a binarysequence (such as address or data), the “#” symbol implies that the signal is inverted. For example, D[3:0] = “HLHL” refers to a hex ‘A’, and D[3:0]# = “LHLH” also refers to a hex “A” (H= High logic level, L= Low logic level).Front Side Bus (FSB) Refers to the interface between the processor and system core logic (also known as the Intel SCH component).AGTL+Advanced Gunning Transceiver Logic. Used to refer to Assisted GTL+ signaling technology on some Intel processors.CMOS Complementary metal-Oxide semiconductor.Storage ConditionsRefers to a non-operational state. The processor may be installed in aplatform, in a tray, or loose. Processors may be sealed in packaging or exposed to free air. Under these conditions, processor landings should not be connected to any supply voltages, have any I/Os biased, or receive any clocks. Upon exposure to “free air” (i.e., unsealed packaging or a device removed from packaging material) the processor must be handled in accordance with moisture sensitivity labeling (MSL) as indicated on the packaging material.Enhanced Intel SpeedStep® Technology Technology that provides power management capabilities to low power devices.Processor Core Processor core die with integrated L1 and L2 cache. All AC timing and signal integrity specifications are at the pads of the processor core. Intel®Virtualization Technology (Intel® VT) Processor virtualization which when used in conjunction with VirtualMachine Monitor software enables multiple, robust independent software environments inside a single platform. TDP Thermal Design PowerV CCThe processor core power supplyVR Voltage Regulator V SSThe processor groundIntroductionDatasheet 9Term DefinitionV CC HFM V CC at Highest Frequency Mode (HFM). V CC LFM V CC at Lowest Frequency Mode (LFM). V CC ,BOOT Default V CC Voltage for Initial Power Up. V CCP AGTL+ Termination Voltage. V CCPC6 AGTL+ Termination Voltage. V CCA PLL Supply voltage.V CCDPPWDN V CC at Deep Power-Down Technology (C6). V CCDPRSLP V CC at Deeper Sleep (C4). V CCF Fuse Power Supply.I CCDESI CCDES for Intel® Atom™ processor Z5xx series Recommended DesignTarget power delivery (Estimated).I CC I CC for Intel® Atom™ processors Z5xx series is the number that can be use as a reflection on battery life estimates. I AH, I CC Auto-Halt I SGNT I CC Stop-Grant. I DSLP I CC Deep Sleep.dI CC /dt V CC Power Supply Current Slew Rate at Processor Package Pin (Estimated). I CCA I CC for V CCA Supply. P AH Auto Halt Power. P SGNT Stop Grant Power. P DPRSLP Deeper Sleep Power.P DC6 Deep Power-Down Technology (C6). T JJunction Temperature.Introduction10 Datasheet1.3 ReferencesMaterial and concepts available in the following documents may be beneficial when reading this document.Table 1. ReferencesDocumentDocumentNumber/Location Intel® System Controller Hub (Intel® SCH) Datasheet 319537 Intel® Atom™ Processor Z5xx Series Specification Update 319538 Intel® 64 and IA-32 Architectures Software Developer's ManualsVolume 1: Basic ArchitectureVolume 2A: Instruction Set Reference, A-M Volume 2B: Instruction Set Reference, N-Z Volume 3A: System Programming GuideVolume 3B: System Programming Guide/pr oducts/processor/manual s/index.htmAP-485, Intel® Processor Identification and CPUID Instruction Application Note/de sign/processor/applnots/241618.htm§2Low Power Features2.1Clock Control and Low-power StatesThe processor supports low power states at the thread level and the core/packagelevel. Thread states (TCx) loosely correspond to ACPI processor power states (Cx). Athread may independently enter the TC1/AutoHALT, TC1/MWAIT, TC2, TC4 or TC6 lowpower states, but this does not always cause a power state transition. Only when boththreads request a low-power state (TCx) greater than the current processor state willa transition occur. The central power management logic ensures the entire processorenters the new common processor power state. For processor power states higherthan C1, this would be done by initiating a P_LVLx (P_LVL2 and P_LVL3) I/O read tothe chipset by both threads. Package states are states that require externalintervention and typically map back to processor power states. Package states forprocessor include Normal (C0, C1), Stop Grant and Stop Grant Snoop (C2), DeeperSleep (C4), and Deep Power-Down Technology (C6).The processor implements two software interfaces for requesting low power states:MWAIT instruction extensions with sub-state hints and P_LVLx reads to the ACPIP_BLK register block mapped in the processor’s I/O address space. The P_LVLx I/Oreads are converted to equivalent MWAIT C-state requests inside the processor and donot directly result in I/O reads on the processor FSB. The monitor address does notneed to be setup before using the P_LVLx I/O read interface. The sub-state hints usedfor each P_LVLx read can be configured in a software programmable MSR by BIOS. Ifa thread encounters a chipset break event while STPCLK# is asserted, then it assertsthe PBE# output signal. Assertion of PBE# when STPCLK# is asserted indicates tosystem logic that individual threads should return to the C0 state and the processorshould return to the Normal state.Figure 1 shows the thread low-power states. Figure 2 shows the package low-powerstates. Table 2 provides a mapping of thread low-power states to package low powerstates.Figure 1. Thread Low-Power StatesFigure 2. Package Low-Power StatesTable 2. Coordination of Thread Low-Power States at the Package Level/Core LevelNOTE:1 AutoHALT or MWAIT/C1.NOTE:To enter a package/core state, both threads must share a common low power state. If the threads are not in a common low power state, the package state will resolve to thehighest common power C state.2.1.1Package/Core Low-Power State DescriptionsThe following state descriptions assume that both threads are in a common low powerstate. For cases when only one thread is in a low power state no change in powerstate will occur.2.1.1.1Normal State (C0, C1)This is the normal operating state for the processor. The processor remains in theNormal state when the processor/core is in the C0, C1/AutoHALT, or C1/MWAIT state.C0 is the active execution state.2.1.1.1.1C1/AutoHalt Powerdown StateC1/AutoHALT is a low-power state entered when one thread executes the HALTinstruction while the other is in the TC1 or greater thread state. The processor willtransition to the C0 state upon occurrence of SMI#, INIT#, LINT[1:0] (NMI, INTR), orFSB interrupt messages. RESET# will cause the processor to immediately initializeitself.A System Management Interrupt (SMI) handler will return execution to either Normalstate or the AutoHALT Powerdown state. See the Intel® 64 and IA-32 ArchitecturesSoftware Developer's Manuals, Volume 3A/3B: System Programmer's Guide for moreinformation.The system can generate a STPCLK# while the processor is in the AutoHALTPowerdown state. When the system de-asserts the STPCLK# interrupt, the processorwill return to the HALT state.While in AutoHALT Powerdown state, the processor will process bus snoops. Theprocessor will enter an internal snoopable sub-state (not shown in Figure 1) to processthe snoop and then return to the AutoHALT Powerdown state.2.1.1.1.2C1/MWAIT Powerdown StateC1/MWAIT is a low-power state entered when one thread executes the MWAIT(C1)instruction while the other thread is in the TC1 or greater thread state. Processorbehavior in the MWAIT state is identical to the AutoHALT state except that Monitorevents can cause the processor to return to the C0 state. See the Intel® 64 and IA-32Architectures Software Developer's Manuals, Volume 2A: Instruction Set Reference, A-M and Volume 2B: Instruction Set Reference, N-Z, for more information.2.1.1.2C2 StateIndividual threads of the dual-threaded processor can enter the TC2 state by initiatinga P_LVL2 I/O read to the P_BLK or an MWAIT(C2) instruction. Once both threads haveC2 as a common state, the processor will transition to the C2 state; however, theprocessor will not issue a Stop-Grant Acknowledge special bus cycle unless theSTPCLK# pin is also asserted by the chipset.While in the C2 state, the processor will process bus snoops. The processor will entera snoopable sub-state described the following section (and shown in Figure 1), toprocess the snoop and then return to the C2 state.2.1.1.2.1Stop-Grant StateWhen the STPCLK# pin is asserted, each thread of the processors enters the Stop-Grant state within 1384 bus clocks after the response phase of the processor-issuedStop-Grant Acknowledge special bus cycle. When the STPCLK# pin is de-asserted, thecore returns to its previous low-power state.Since the AGTL+ signal pins receive power from the FSB, these pins should not bedriven (allowing the level to return to V CCP) for minimum power drawn by thetermination resistors in this state. In addition, all other input pins on the FSB shouldbe driven to the inactive state.RESET# causes the processor to immediately initialize itself, but the processor willstay in Stop-Grant state. When RESET# is asserted by the system, the STPCLK#,SLP#, DPSLP#, and DPRSTP# pins must be de-asserted prior to RESET# de-assertion.When re-entering the Stop-Grant state from the Sleep state, STPCLK# should be de-asserted after the de-assertion of SLP#.While in Stop-Grant state, the processor will service snoops and latch interruptsdelivered on the FSB. The processor will latch SMI#, INIT#, and LINT[1:0] interruptsand will service only one of each upon return to the Normal state.The PBE# signal may be driven when the processor is in Stop-Grant state. PBE# willbe asserted if there is any pending interrupt or Monitor event latched within theprocessor. Pending interrupts that are blocked by the EFLAGS.IF bit being clear willstill cause assertion of PBE#. Assertion of PBE# indicates to system logic that theentire processor should return to the Normal state.A transition to the Stop-Grant Snoop state occurs when the processor detects a snoopon the FSB (see Section 2.1.1.2.2). A transition to the Sleep state (seeSection 2.1.1.3.1) occurs with the assertion of the SLP# signal.2.1.1.2.2Stop-Grant Snoop StateThe processor responds to snoop or interrupt transactions on the FSB while in Stop-Grant state by entering the Stop-Grant Snoop state. The processor will stay in thisstate until the snoop on the FSB has been serviced (whether by the processor oranother agent on the FSB) or the interrupt has been latched. The processor returns tothe Stop-Grant state once the snoop has been serviced or the interrupt has beenlatched.2.1.1.3C4 StateIndividual threads of the processor can enter the C4 state by initiating a P_LVL4 I/Oread to the P_BLK or an MWAIT(C4) instruction. Attempts to request C3 will alsocovert to C4 requests. If both processor threads are in C4, the central powermanagement logic will request that the entire processor enter the Deeper Sleeppackage low-power state using the sequence through the Sleep and Deep Sleep statesall described in the following sections.To enable the package level Intel Enhanced Deeper Sleep state, Dynamic Cache Sizingand Intel Enhanced Deeper Sleep state fields must be configured in thePMG_CST_CONFIG_CONTROL MSR. Refer to Section 2.1.1.3.3 for further details onIntel Enhanced Deeper Sleep state.2.1.1.3.1Sleep StateThe Sleep state is a low-power state in which the processor maintains its context,maintains the phase-locked loop (PLL), and stops all internal clocks. The Sleep state isentered through assertion of the SLP# signal while in the Stop-Grant state and is onlya transition state for Intel Atom™ processor Z5xx series. The SLP# pin should only beasserted when the processor is in the Stop-Grant state. SLP# assertion while theprocessor is not in the Stop-Grant state is out of specification and may result inunapproved operation.In the Sleep state, the processor is incapable of responding to snoop transactions orlatching interrupt signals. No transitions or assertions of signals (with the exception ofSLP#, DPSLP#, or RESET#) are allowed on the FSB while the processor is in Sleepstate. Snoop events that occur while in Sleep state or during a transition into or out ofSleep state will cause unpredictable behavior. Any transition on an input signal beforethe processor has returned to the Stop-Grant state will result in unpredictablebehavior.If RESET# is driven active while the processor is in the Sleep state, and held active asspecified in the RESET# pin specification, then the processor will reset itself, ignoringthe transition through Stop-Grant state. If RESET# is driven active while the processoris in the Sleep state, the SLP# and STPCLK# signals should be de-assertedimmediately after RESET# is asserted to ensure the processor correctly executes theReset sequence.While in the Sleep state, the processor is capable of entering an even lower powerstate, the Deep Sleep state, by asserting the DPSLP# pin (see Section 2.1.1.3.2).While the processor is in the Sleep state, the SLP# pin must be de-asserted if anotherasynchronous FSB event needs to occur.2.1.1.3.2Deep Sleep StateThe Deep Sleep state is entered through assertion of the DPSLP# pin while in theSleep state and is also only a transition state for the Intel® Atom™ processor Z5xxseries. BCLK may be stopped during the Deep Sleep state for additional platform levelpower savings. As an example, BCLK stop/restart timings on appropriate chipset-based platforms with the CK540 clock chip are as follows:•Deep Sleep entry: the system clock chip may stop/tristate BCLK within 2 BCLKsof DPSLP# assertion. It is permissible to leave BCLK running during Deep Sleep.•Deep Sleep exit: the system clock chip must start toggling BCLK within 10 BCLKperiods within DPSLP# de-assertion.To re-enter the Sleep state, the DPSLP# pin must be de-asserted. BCLK can be re-started after DPSLP# de-assertion as described above. A period of 15 microseconds(to allow for PLL stabilization) must occur before the processor can be considered tobe in the Sleep state. Once in the Sleep state, the SLP# pin must be de-asserted tore-enter the Stop-Grant state.While in Deep Sleep state, the processor is incapable of responding to snooptransactions or latching interrupt signals. No transitions of signals are allowed on theFSB while the processor is in Deep Sleep state. When the processor is in Deep Sleepstate, it will not respond to interrupts or snoop transactions. Any transition on aninput signal before the processor has returned to Stop-Grant state will result inunpredictable behavior.2.1.1.3.3Deeper Sleep StateThe Deeper Sleep state is similar to the Deep Sleep state, but further reduces corevoltage levels. One of the potential lower core voltage levels is achieved by enteringthe base Deeper Sleep state. The Deeper Sleep state is entered through assertion ofthe DPRSTP# pin while in the Deep Sleep state. The following lower core voltage levelis achieved by entering the Intel Enhanced Deeper Sleep state which is a sub-state ofDeeper Sleep state. Intel Enhanced Deeper Sleep state is entered through assertion ofthe DPRSTP# pin while in the Deep Sleep only when the L2 cache has been completelyshut down. Refer to Section 2.1.1.3.4 for further details on reducing the L2 cache andentering Intel Enhanced Deeper Sleep state.In response to entering Deeper Sleep, the processor drives the VID codecorresponding to the Deeper Sleep core voltage on the VID[6:0] pins.Exit from Deeper Sleep or Intel Enhanced Deeper Sleep state is initiated by DPRSTP#de-assertion when the core requests a package state other than C4 or the corerequests a processor performance state other than the lowest operating point.2.1.1.3.4Intel® Atom™ Processor Z5xx Series C5As mentioned previously in this document, each C state has latency and transitorypower costs associated with entering/exiting idle states. When the processor isinterrupted, it must awake to service some request(s). If these requests occur at ahigh frequency, it is possible that more power will be consumed entering/exiting thestates than will be saved. To alleviate this concern, the Intel Atom™ processor Z5xxseries implements a new state called ‘Intel Atom™ processor Z5xx series C5’. TheIntel Atom™ processor Z5xx series C5 is not exposed to software. The only way toenter the C5 state is using a hardware promotion of C4 (with the cache ways shrunkto zero).When the processor is in C4, the chipset assumes the processor has data in its cache.Often, the processor has fully flushed its cache. To avoid waking up the processor toservice snoops when there is no data in its caches, the processor will automaticallypromote C4 requests to C5 (when the cache is flushed). The chipset treats C5 as anon-snoopable state. Therefore, all snoops will be completed from the I/O DMAmasters without waking up the processor.While similar, the Intel Atom™ processor Z5xx series C5 differs from the Core 2 DuoT5000/T7000 C5 implementation. In the Intel Atom™ processor Z5xx series C5, theV CC will not be powered below the retention of caches voltage; there is no need toinitialize the processor’s caches on a C5 exit, and C5 is not architecturally enumeratedto software. This state is the same as the Intel Atom™ processor Z5xx series’s C5. 2.1.1.4C6 StateC6 is a new low power state being introduced on the Intel Atom™ processor Z5xxseries. C6 behavior is the same as Intel Enhanced Deeper Sleep with the addition ofan on-die SRAM. This memory saves the processor state allowing the processor tolower its main core voltage closer to 0 V. It is important to note that V CC cannot belower while only 1 thread is in C6 state.The processor threads can enter the C6 state by initiating a P_LVL6 I/O read to theP_BLK or an MWAIT(C6) instruction. To enter C6, the processor’s caches must beflushed. The primary method to enter C6 used by newer operating systems (thatsupport MWAIT) will be through the MWAIT instruction.When the thread enters C6, it saves the processor state that is relevant to theprocessor context in an on-die SRAM that resides on a separate power plane V CCP (I/Opower supply). This allows the core V CC to be lowered to any arbitrary voltageincluding 0 V. The microcode performs the save and restore of the processor state onentry and exit from C6 respectively.To improve the amount of power reduction possible in the Deep Power-DownTechnology state, a split V TT is implemented. See Section2.5.1 for additionalinformation.。
3 Transition-Edge Sensors
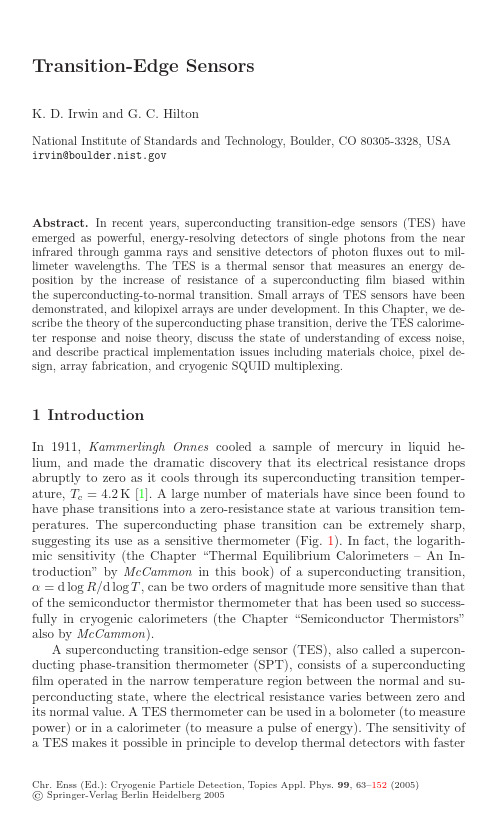
Transition-Edge SensorsK.D.Irwin and G.C.HiltonNational Institute of Standards and Technology,Boulder,CO80305-3328,USA irvin@Abstract.In recent years,superconducting transition-edge sensors(TES)have emerged as powerful,energy-resolving detectors of single photons from the near infrared through gamma rays and sensitive detectors of photonfluxes out to mil-limeter wavelengths.The TES is a thermal sensor that measures an energy de-position by the increase of resistance of a superconductingfilm biased within the superconducting-to-normal transition.Small arrays of TES sensors have been demonstrated,and kilopixel arrays are under development.In this Chapter,we de-scribe the theory of the superconducting phase transition,derive the TES calorime-ter response and noise theory,discuss the state of understanding of excess noise, and describe practical implementation issues including materials choice,pixel de-sign,array fabrication,and cryogenic SQUID multiplexing.1IntroductionIn1911,Kammerlingh Onnes cooled a sample of mercury in liquid he-lium,and made the dramatic discovery that its electrical resistance drops abruptly to zero as it cools through its superconducting transition temper-ature,T c=4.2K[1].A large number of materials have since been found to have phase transitions into a zero-resistance state at various transition tem-peratures.The superconducting phase transition can be extremely sharp, suggesting its use as a sensitive thermometer(Fig.1).In fact,the logarith-mic sensitivity(the Chapter“Thermal Equilibrium Calorimeters–An In-troduction”by McCammon in this book)of a superconducting transition,α=d log R/d log T,can be two orders of magnitude more sensitive than that of the semiconductor thermistor thermometer that has been used so success-fully in cryogenic calorimeters(the Chapter“Semiconductor Thermistors”also by McCammon).A superconducting transition-edge sensor(TES),also called a supercon-ducting phase-transition thermometer(SPT),consists of a superconducting film operated in the narrow temperature region between the normal and su-perconducting state,where the electrical resistance varies between zero and its normal value.A TES thermometer can be used in a bolometer(to measure power)or in a calorimeter(to measure a pulse of energy).The sensitivity of a TES makes it possible in principle to develop thermal detectors with faster Chr.Enss(Ed.):Cryogenic Particle Detection,Topics Appl.Phys.99,63–152(2005)©Springer-Verlag Berlin Heidelberg200564K.Irwin and G.HiltonFig.1.The transition of a superconductingfilm(a Mo/Cu proximity bilayer)from the normal to the superconducting state near96mK.The sharp phase transition suggests its use as a sensitive thermometerresponse,larger heat capacity,and smaller detectable energy input than ther-mal detectors made using conventional semiconductor thermistors.However, the sharp transition leads to a greater tendency for instability and lower saturation energy,so that careful design is required.In1941,Andrews et al.applied a current to afine tantalum wire operating in its superconducting transition region at3.2K and measured the change in resistance caused by an infrared signal[2].This was thefirst demonstra-tion of a TES bolometer.In1949,the same researcher applied a current to a niobium nitride strip within its superconducting transition at15K and measured the voltage pulses when it was bombarded by alpha particles[3]–thefirst reported demonstration of a TES calorimeter.This work followed on earlier suggestions by Andrews himself in1938[4]and Goetz in1939[5].During thefirst half century after their invention,TES detectors were seldom used in practical applications.One of the principal barriers to their adoption was the difficulty of matching their noise to FET amplifiers(the TES normal resistance is typically a few ohms or less).In order to noise match,the TES was sometimes read out using a cross-correlation circuit to cancel noise[6],ac biased in conjunction with a step-up transformer[7],or fabricated in long meander lines with high normal resistance[8,9].In recent years,this problem has been largely eliminated by the use of superconduct-ing quantum interference device(SQUID)current amplifiers[10],which are easily impedance-matched to low-resistance TES detectors[11,12].In addi-tion to their many other advantages,SQUID amplifiers make it possible to multiplex the readout of TES detectors(Sect.4.2),so that large arrays of detectors can be instrumented with a manageable number of wires to room rge arrays of TES detectors are now being deployed for a number of different applications.Transition-Edge Sensors65 Another barrier to the practical use of TES detectors has been that it is difficult to operate them within the extremely narrow superconducting tran-sition region.When they are current-biased,Joule heating of the TES by the current can lead to thermal runaway,and smallfluctuations in bath tem-perature significantly degrade performance.Furthermore,variations in the transition temperature between multiple devices in an array of TES detec-tors can make it impossible to bias them all at the same bath temperature. As will be explained in Sect.2.5,when the TES is instead voltage-biased and read out with a current amplifier,the devices can easily be stably biased and they self-regulate in temperature within the transition with much less sensitivity tofluctuations in the bath temperature[13].The introduction of voltage-biased operation with SQUID current readout has led to an explosive growth in the development of TES detectors in the past decade.The potential of TES detectors is now being realized.TES detectors are being developed for measurements across the electromagnetic spectrum from millimeter[14,15,16]through gamma rays[17,18]as well as with weakly interacting particles[19]and biomolecules[20,21,22].They have contributed to the study of dark matter and supersymmetry[23,24],the chemical compo-sition of materials[25],and the newfield of quantum information[26].They have extended the usefulness of the single-photon calorimeter all the way to the near infrared[27],with possible extension to the far infrared.They are being used in thefirst multiplexed submillimeter,millimeter-wave,and X-ray detectors for spectroscopy and astronomical imaging[15,16,28,29,30].2Superconducting Transition-Edge Sensor TheoryWe now describe the theory of a superconducting transition-edge sensor.We describe the physics of the superconducting transition(Sect.2.1),summa-rize the equations for TES small-signal theory(Sect.2.2),and analyze the bias circuit for a TES and its electrical and thermal response(Sect.2.3),the conditions for the stability of a TES(Sect.2.4),the consequences of neg-ative electrothermal feedback(Sect.2.5),thermodynamic noise(Sect.2.6), unexplained noise(Sect.2.7),and the effects of operation outside of the small-signal limit(Sect.2.8).Particular implementations of both TES single pixels and arrays,including performance results,will be described in Sect.3and Sect.4.2.1The Superconducting TransitionIn this work,we discuss sensors based on traditional“low-T c”superconduc-tors(often those with transition temperatures below1K).Other classes of su-perconductors,including the cuprates such as yttrium-barium-copper-oxide, are also used in thermal detectors.Transition-edge sensors based on these66K.Irwin and G.Hilton“high-T c ”materials have much lower sensitivity and much higher saturation levels than those that are discussed here.In low-T c materials,the phenomenon of superconductivity has been fairly well understood since the 1950s,when detailed microscopic and macroscopic theories were developed.Superconductivity in low-T c materials occurs when two electrons are bound together in “Cooper”pairs,acting as one particle.The energy binding Cooper pairs prevents them from scattering,allowing them to flow without resistance.Bardeen,Cooper,and Schrieffer first ex-plained the formation of Cooper pairs in 1957in the landmark microscopic BCS theory [31].The energy binding the two electrons in a Cooper pair is due to inter-actions with positive ions in the lattice mediated by phonons (quantized latticevibrations).When a negatively charged electron flows in a supercon-ductor,positive ions in the lattice are drawn towards it,creating a cloud of positive charge.A second electron is attracted to this cloud.The energy binding the two electrons is referred to as the “superconducting energy gap”of the material.In the BCS theory,the size of the Cooper pair wave function is determined by the temperature-dependent coherence length ξ(T ),which has the zero-temperature value ξ0≡ξ(0)≈0.18v F /(k B T c ).Here v F is the Fermi velocity of the material,k B is the Boltzmann constant,and T c is the superconducting transition temperature.At temperatures above the transi-tion temperature,thermal energies of order k B T spontaneously break Cooper pairs and superconductivity vanishes.In a BCS superconductor,the transi-tion temperature T c is related to the superconducting energy gap E gap of the material by E gap =≈3.5k B T c .In addition to perfect dc conductivity below T c ,a second hallmark of superconductivity is the Meissner effect:the free energy of the is minimized when an external magnetic field is excluded from the interior of a superconducting sample.An applied mag-netic field is exponentially screened by an induced Cooper-pair supercurrent with an effective temperature-dependent penetration depth,λeff(T ).The ap-proximate zero-temperature value of the penetration depth is the London penetration depth,λL (0).Near the transition temperature,the physics of a superconductor is well described by the macroscopic Ginzburg–Landau theory [32],which was de-rived by a Taylor expansion of a phenomenological order parameter Ψ.Ψwas later shown to be proportional to the density of superconducting pairs [33].One result of the Ginzburg–Landau theory is that the characteristics of a superconductor with penetrating magnetic flux (such as a superconductor on its transition)are strongly dependent on its dimensionless Ginzburg–Landau parameter,κ≡λeff(T )/ξ(T ).If κ<1/√2,the superconductor is of Type I,and the free energy is minimized when magnetic flux that has penetrated the material clumps together.If κ>1/√2,the is of Type II,and magnetic flux that has penetrated the material separates into individual flux quanta that repel each other.The flux quantum is 本页已使用福昕阅读器进行编辑。
pl光谱锋的位置偏移
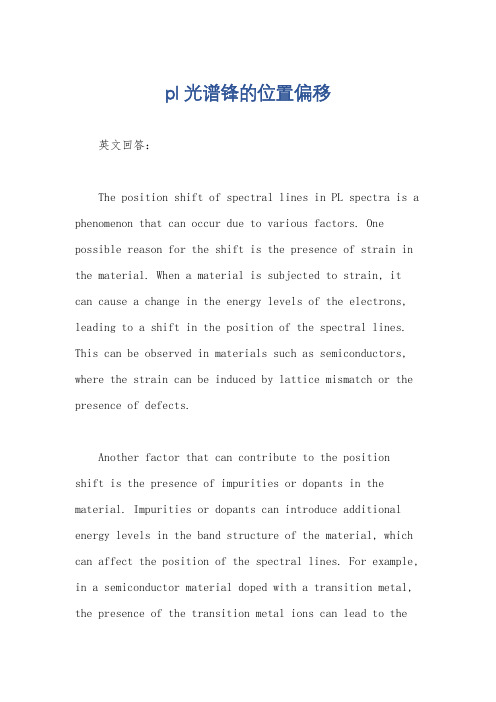
pl光谱锋的位置偏移英文回答:The position shift of spectral lines in PL spectra is a phenomenon that can occur due to various factors. One possible reason for the shift is the presence of strain in the material. When a material is subjected to strain, it can cause a change in the energy levels of the electrons, leading to a shift in the position of the spectral lines. This can be observed in materials such as semiconductors, where the strain can be induced by lattice mismatch or the presence of defects.Another factor that can contribute to the positionshift is the presence of impurities or dopants in the material. Impurities or dopants can introduce additional energy levels in the band structure of the material, which can affect the position of the spectral lines. For example, in a semiconductor material doped with a transition metal, the presence of the transition metal ions can lead to theformation of localized energy levels, resulting in a shiftin the position of the spectral lines.In addition to strain and impurities, the positionshift of spectral lines in PL spectra can also beinfluenced by temperature. As the temperature increases,the thermal energy of the system also increases, leading to a broadening of the spectral lines. This broadening can result in a shift in the position of the spectral lines, as the peak of the line may no longer be at the same energy level as before. This effect is commonly observed in materials such as quantum dots, where the position of the emission peak can change with temperature.中文回答:PL光谱中光谱线位置的偏移是由多种因素引起的现象。
光材料学英文文献(1)
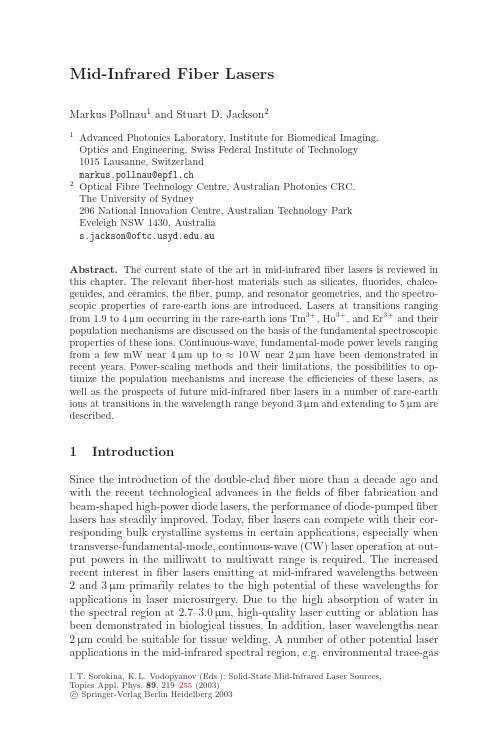
Mid-Infrared Fiber LasersMarkus Pollnau1and Stuart D.Jackson21Advanced Photonics Laboratory,Institute for Biomedical Imaging,Optics and Engineering,Swiss Federal Institute of Technology1015Lausanne,Switzerlandmarkus.pollnau@epfl.ch2Optical Fibre Technology Centre,Australian Photonics CRC.The University of Sydney206National Innovation Centre,Australian Technology ParkEveleigh NSW1430,Australias.jackson@.auAbstract.The current state of the art in mid-infraredfiber lasers is reviewed in this chapter.The relevantfiber-host materials such as silicates,fluorides,chalco-genides,and ceramics,thefiber,pump,and resonator geometries,and the spectro-scopic properties of rare-earth ions are sers at transitions ranging from1.9to4µm occurring in the rare-earth ions Tm3+,Ho3+,and Er3+and their population mechanisms are discussed on the basis of the fundamental spectroscopic properties of these ions.Continuous-wave,fundamental-mode power levels ranging from a few mW near4µm up to≈10W near2µm have been demonstrated in recent years.Power-scaling methods and their limitations,the possibilities to op-timize the population mechanisms and increase the efficiencies of these lasers,as well as the prospects of future mid-infraredfiber lasers in a number of rare-earth ions at transitions in the wavelength range beyond3µm and extending to5µm are described.1IntroductionSince the introduction of the double-cladfiber more than a decade ago and with the recent technological advances in thefields offiber fabrication and beam-shaped high-power diode lasers,the performance of diode-pumpedfiber lasers has steadily improved.Today,fiber lasers can compete with their cor-responding bulk crystalline systems in certain applications,especially when transverse-fundamental-mode,continuous-wave(CW)laser operation at out-put powers in the milliwatt to multiwatt range is required.The increased recent interest infiber lasers emitting at mid-infrared wavelengths between 2and3µm primarily relates to the high potential of these wavelengths for applications in laser microsurgery.Due to the high absorption of water in the spectral region at2.7–3.0µm,high-quality laser cutting or ablation has been demonstrated in biological tissues.In addition,laser wavelengths near 2µm could be suitable for tissue welding.A number of other potential laser applications in the mid-infrared spectral region,e.g.environmental trace-gas I.T.Sorokina,K.L.Vodopyanov(Eds.):Solid-State Mid-Infrared Laser Sources,Topics Appl.Phys.89,219–255(2003)c Springer-Verlag Berlin Heidelberg2003220Markus Pollnau and Stuart D.Jacksondetection,are currently becoming increasingly important.In all these appli-cations fiber lasers may find their niches.The high development costs of fabricating fibers with sufficiently low losses in the mid-infrared spectral region has impeded the necessary research efforts in the field of mid-infrared fiber lasers.The currently available fiber materials that are suitable as host materials for specific rare-earth-doped fiber lasers in the spectral region 2–5µm will be introduced in Sect.2.More than any other idea,the invention of the double-clad fiber geometry has accelerated the output-power scaling and hence the success of fiber lasers.The various aspects of the fiber,pump,and resonator geometries will be de-scribed in Sect.3.A significant number of spectroscopic investigations has led to a better understanding of the population mechanisms of rare-earth-doped laser systems.The fundamental spectroscopic properties of rare-earth ions in solid-state host materials will be reviewed in Sect.4.Equipped with this general information,the performance of the most important mid-infrared fiber laser transitions in the wavelength range 2–3µm can be understood in detail.Sect.5will be devoted to the Tm 3+fiber lasers at 1.9and 2.3µm,whereas the Ho 3+fiber lasers at 2.1and 2.9µm will be discussed in Sect.6.An impressive example of the variety of population mechanisms and operational regimes in a single system is the Er 3+2.7µm fiber laser transition that will be investigated in Sect.7.At wavelengths beyond 3µm,it becomes increasingly difficult to find suitable host materials for actively doped laser systems.This statement holds true for glass fibers in the same way as for crystalline materials.The prospects of future mid-infrared fiber lasers in this wavelength range will be discussed in Sect.8.Besides general introductions to the different topics of lasers [1,2]that include many aspects relevant also to mid-infrared fiber lasers,a comprehen-sive introduction to the field of rare-earth-doped fiber lasers can be found in [3].2Fiber MaterialsThe choice of the fiber material involves a number of considerations:the maximum phonon energy,the environmental durability,the draw ability,the rare-earth solubility,and the purity of the starting materials.The maximum phonon energy of the glass sets the overall infrared transparency range of the fiber and the multiphonon relaxation rates which influence the quantum efficiency.The multiphonon relaxation rates for the common fiber glasses as a function of the energy gap between energy levels are shown in Fig.1.The optical transparency range relates to both the size of the band gap and also the infrared absorption cut-off,hence to the vibrational frequency νof the anion–cation bonds of the glass.For an ordered structure,ν=(1/2π) k/M ,(1)Mid-Infrared Fiber Lasers221101010M u l t i p h o n o n R e l a x a t i o n R a t e (s -1)Energy Gap (cm )Fig.1.Calculated and measured multiphonon relaxation rates as a function of the energy gap between energy levels for glasses with different maximum phonon energies.(Data taken from [4,5])where M =m 1m 2/(m 1+m 2)is the reduced mass for two bodies m 1,m 2vibrating with an elastic restoring force k .While for disordered structures like glass,this is not an accurate expression,nevertheless,it does highlight the important contributions to the glass transparency.The relative cation–anion bond strength is intimated by the field strength Z /r 2,where Z is the valence state of the cation or anion and r is the ionic radius.Generally,glasses composed of large anions and cations with low field strengths display high transparency in the mid-infrared spectral region.The important physical properties of the popular glasses used for optical fibers are shown in Table 1.Table 1.Properties of popular fiber materialsFibermaterialMax.phonon energy (cm −1)Infrared transparency (µm)Propagation losses (λat minimum)(dB/km)Thermal conductivity (W/K m)Silica1100[4]<2.50.2(1.55µm) 1.38[6]ZBLAN550[7]<6.00.05(2.55µm)0.7–0.8[8]GLS 425[5]<8.00.5(3.50µm)0.43–0.5[9]2.1SilicatesThis glass is perhaps the most important material used for optical fiber pro-duction [3,10],however,the maximum phonon energy is high (≈1100cm −1)and has so far limited the emission wavelength of mid-infrared fiber lasers us-ing this material to ≈2.2µm [11].Silica is robust and involves the very effec-222Markus Pollnau and Stuart D.Jacksontive modified chemical vapor deposition(MCVD)technique forfiber fabrica-tion.Reducing the OH−content in the glass,which has two main absorption peaks in the range1.3–2.0µm[12],improves the near-to-mid-infrared utility. Rare-earth ions such as Nd3+and Er3+which have highfield strengths have low solubility in silicate glass which can lead to clustering and micro-scale phase separation.2.2FluoridesThe use offluoride glasses,especially the heavy-metalfluorides[13,14],as host materials for mid-infraredfiber lasers has found wide acceptance.The most common form of heavy-metalfluoride glass is thefluorozirconate(ZrF4) composition and the most widespreadfluoridefiber material is ZBLAN[15], a mixture of53mol.%ZrF4,20mol.%BaF2,4mol.%LaF3,3mol.%AlF3, and20mol.%NaF.Since it can be readily drawn into single-mode optical fiber[16]it is particularly important to mid-infraredfiber lasers[17].The large atomic weight of the zirconium atom combined with relatively weak bonding provides a maximum phonon energy for ZBLAN of≈550cm−1and allows for high infrared transparency up to≈6µm.Multiphonon relaxation, however,becomes significant for transitions at wavelengths longer than≈3µpared to silica,ZBLAN has a lower damage threshold and a lower level of inhomogeneous spectral-line broadening(Sect.4.1)because the rare-earth ion is placed in sites of a less perturbed network.The crystal-field strength is also comparatively weaker[18].An overview of the spectroscopic properties of rare-earth ions doped into ZBLAN has been given in[7].2.3ChalcogenidesChalcogenides are composed of the chalcogen elements S,Se and Te[19,20,21]. They are environmentally durable,have a low toxicity and have reasonably large glass forming regions.When the rare-earth ions are doped into these glasses[22],the radiative transition probabilities and,therefore,the absorp-tion and emission cross-sections are high as a result of the high refractive in-dex(≈2.6)of the glass and the high degree of covalency of the rare-earth ion with the surrounding medium.Maximum phonon energies of300–450cm−1 produce low rates of multiphonon relaxation,see Fig.1,and therefore high quantum efficiencies.The low thermal conductivity,see Table1,is however an important factor to be considered in the design of chalcogenide-based lasers. Of the large number of rare-earth chalcogenides studied for luminescent emis-sion,the most important glasses are the sulfide glasses GaLaS(GLS)[23]and GeGaS[24]because of the reasonably high rare-earth solubility.2.4CeramicsStudies into the use of ceramics as host materials for the rare earths have recently made a lot of progress[25].These ceramics are composed of nano-Mid-Infrared Fiber Lasers223 crystallites of materials such as Y3Al5O12(YAG)and can be produced ina simple and cost-efficient process at relatively low temperatures.This allows the fabrication of materials with very high melting points[26]that are difficultto grow by other techniques such as the Czochralski method[27].This class ofmaterials is also available infiber geometry[28].Ceramicfibers combine the characteristics of crystalline materials such as high absorption and emissioncross-sections,large thermal conductivity,and even the possibility of doping with transition-metal ions[28]with the convenience of guiding the pump andsignal light in afiber.Currently,the losses of thesefibers are comparativelyhigh,but further improvement can be expected.3Fiber,Pump,and Resonator GeometriesThe light oscillating in afiber-laser resonator can be either free running or deliberately modulated depending on whether CW or pulsed output,re-spectively,is desired.Consequently,a large number of techniques for pulsedoperation including Q-switching and mode locking offiber lasers have been explored.These techniques have been investigated intensively for the commonlaser transitions at1µm in Nd3+and Yb3+and at1.5µm in Er3+,and are usually described in combination with these lasers.The smallfiber size limitsthe peak power through the damage-threshold intensity(propagating powerper core area)and,hence,crystalline lasers in bulk geometries or optical parametric processes are often preferred when high-energy short pulses areneeded.This argument accounts especially for mid-infrared ZBLAN-basedfiber lasers,because thesefibers possess a lower damage threshold compared to silicafibers.The description of mid-infraredfiber lasers is,therefore,con-fined to CW operation and specific techniques for pulsed operation offiber lasers are not discussed in this chapter.In an analogous way to the optical excitation of bulk gain media,dopedopticalfibers can be either end pumped(core pumped)or side pumped (cladding pumped).The former method is less scalable since it relies onthe use of expensive high-beam-quality pump sources because core areas areusually<100µm2.On the other hand,the larger cladding area(>104µm2) allows for high-power diode-array pumping[29,30,31,32,33].The obvious sim-plicity of the core-pumping method negates further explanation and we will concentrate on the cladding-pumping technique:one of the most important developments infiber-laser technology.3.1Fiber Designs for Cladding PumpingIn the design offibers for cladding pumping,the core of thefiber is gener-ally made to guide a single-transverse LP01mode.The shape of the mul-timode pump cladding,see Fig.2,however,remains somewhatflexible and can be shaped with a number of considerations in mind.The pump cladding,224Markus Pollnau and Stuart D.Jackson (a)(b)(d)CorePump cladding Outer cladding Jacket Fig.2.Principal double-clad fiber geometries which include (a )circular shaped pump cladding with axially positioned core,(b )circular shaped pump cladding with off-axially positioned core,(c )rectangular shaped pump cladding and (d )D-shaped pump claddingwhich in turn is surrounded by a low-refractive-index transparent polymer or glass,provides a high numerical aperture (NA)of 0.3–0.55for the pump cladding.There are three main double-clad-fiber layouts:circular,circular with offset core,and rectangular as shown schematically in Fig.2.Maxi-mum pump-light absorption sees the core near the outer edge of the circular pump cladding [34]because a portion of the launched light is skew to the fiber axis and produces an inner caustic and never crosses the central re-gion of the pump cladding.Scrambling these skew rays by bending [35]or by using a graded and slightly elliptical pump cladding [36]increases the pump-absorption efficiency as does spatially varying refractive-index fluctuations in inhomogeneous pump claddings [37].Inner caustics can be avoided by rectilinearly shaping the pump cladding [38]which has the ancillary advantage of matching the shape of diode-array output.The overall absorption coefficient of the fiber is reduced by the ratio of the core area to the area of the pump cladding [34].The propagation losses for the rectangular-shaped pump cladding are higher and the effective numerical aperture lower as compared to the circular shape [39];however,in certain cases higher dopant concentrations can provide shorter fiber lengths that also lead to reduced nonlinear effects.A D-shaped or trun-cated circular pump cladding [40],see Fig.2d,is also effective while be-ing easier to make than rectangular preforms.The circular-multimode pump cladding may also have the gain medium distributed in a ring around the edge of the pump cladding either discretely or continuously in multi-core [41]and M-profile [42]arrangements,respectively.The effective absorption coef-ficient is now further increased while maintaining high-beam-quality output.A large-mode-area core [43]can also increase the effective absorption coeffi-cient of the fiber.Recently,double-clad pump schemes have been demonstrated also with holey fibers [44].These structures offer the additional advantage of single-mode guiding over a broad spectral range [45].Mid-Infrared Fiber Lasers 2253.2Fiber-Laser ResonatorsTypical free-running fiber-laser resonators are shown schematically in Fig.3.In the simplest resonator,see Fig.3a,the pump light passes through a dichroic mirror that is highly reflective for the oscillating laser light.Fresnel reflection at the cleaved output end facet of the fiber can provide sufficient feedback for laser oscillation;however,with an output-coupler mirror –and pump retro-reflector –placed at the output end of the fiber the optical efficiency can be maximized.In an alternative arrangement,the pump light can be launched into the output end of the fiber,see Fig.3b.A dichroic mirror oriented at 45◦to the fiber axis extracts the laser output and a broadband highly reflecting mirror is placed at the rear fiber end.To scale the output power,each end of the fiber can be pumped,see Fig.3c.Periodic V-grooves [46]or prism coupling [47]along the fiber to distribute the pump access allow one to further scale the output power and are useful for pumping fiber ring resonators.Spectrally combining the output from a number of separate fiber lasers is also a promising power-scaling technique [48,49,50].The highest reported fiber-laser output powers of 110W in a singly Yb 3+-doped fiber [51]and 150W in a Nd 3+,Yb 3+-codoped fiber [52]have been obtained using arrangements as shown schematically in Fig.3c.Bragg gratings can substitute the fiber-butted mirror if spectrally well-defined output is required.PumpPump Pump Pump Output Output OutputMM M MMFiberFiberFiber(a)(b)(c)Fig.3.Schematic diagram of resonators used for free-running fiber lasers with (a )a single-end co-propagating pump,(b )a single-end counter-propagating pump and (c )dual end pumps.M represents the mirror226Markus Pollnau and Stuart D.Jackson3.3Thermal IssuesAs higher pump powers become available from laser-diode systems,it is gen-erally recognized that thermal and thermo-optical issues set limitations to the power scalability of end-pumped bulk-laser systems.Owing to the unfavor-able temperature dependence of thermal and thermo-optical parameters[53], the large heat load in the crystal leads,firstly,to a significant temperature increase in the rod,secondly,to strong thermal lensing with pronounced spherical aberrations,and ultimately,to rod fracture in a high-average-power end-pumped system.Due to its geometry,thefiber provides potentially high pump-and signal-beam intensities without the drawbacks of significant thermal and thermo-optical effects.Its large surface-area-to-volume ratio means that the heat generated from multiphonon relaxation in the core is dissipated effectively by radiation and convection from the outer surface of thefiber.This is es-pecially true for single-clad,core-pumped single-modefibers where this ratio is highest[54].Double-cladfibers have a relatively smaller surface-area-to-volume ratio and thermal issues need to be taken into account[6,55,56]. Thermal management will be required when very high output powers are desired.In particular,for high-power mid-infrared operation,thermal man-agement may be very important because of the decreased quantum efficiency and the consequently higher amount of heat dissipation.4Spectroscopic and Laser Propertiesof Rare-Earth IonsThe structure of a glass is less well defined as compared to a crystalline mate-rial.The local variation of the chemical environment of active ions in a glass has a number of consequences.Most important,the active ions may undergo chemical reactions during the fabrication process and be incorporated in the host in several oxidation states with different spectroscopic properties.Oxi-dation states other than the desired one may act as impurities that introduce undesired optical effects such as parasitic pump absorption,the reabsorption of oscillating laser light,the lifetime quenching of the laser ion,and the trap-ping of the excitation energy.A stable oxidation state of the optically active ion is thus highly desirable.The necessity of a stable oxidation state excludes a number of transition-metal ions from the list of suitable dopants in glass environments.This is one of the possible reasons why examples of transition-metal-ion-doped lasers in glass hosts are rare.On the other hand,most of the rare-earth ions prefer to stabilize in the trivalent oxidation state and are, therefore,suitable candidates as glass andfiber dopants.This chapter will, therefore,concentrate on the rare-earth ions as active dopants offiber lasers.Mid-Infrared Fiber Lasers227 4.1Spectra of Rare-Earth Ions in GlassesThe optical transitions of lanthanide(rare-earth)ions in the visible and in-frared spectral region occur within the4f subshell.This subshell is shielded by the outer5s and5p subshells and the influence of the host material isrelatively small compared to,e.g.,the3d transitions in transition-metal ions.The electronic structure of trivalent rare-earth ions derives from the perturba-tion of the4f energy level in the central-field approximation by the noncen-trosymmetric electron–electron interaction,the spin–orbit interaction,andthe crystal-field splitting(Stark effect);see the example of the energy-level scheme of Er3+in Fig.4.The spin–orbit multiplets are commonly denotedby their2S+1L J terms in Russell–Saunders coupling,although the4f elec-trons of lanthanide ions exhibit intermediate coupling and the total angularmomenta J of the spin–orbit multiplets are linear combinations of the totalorbital angular momenta L and total spins S.Single crystal-field(Stark)tran-sitions between two spin–orbit multiplets cannot be distinguished in glasses at ambient temperature,because inhomogeneous spectral-line broadening oc-curs due to the local variation of the ligand electricfield.Also homogeneous (lifetime)broadening mechanisms are relevant in a number of glasses.This spectral-line broadening makes glasses the preferred hosts when broadband,Fig.4.Energy-level scheme of triva-lent erbium indicating the splitting ofthe4f11configuration in the central-field approximation by the noncen-trosymmetric electron–electron inter-action,the spin–orbit interaction,andthe Stark splitting by the local elec-tricfield of the host material(indi-cated only for selected spin–orbit mul-tiplets)228Markus Pollnau and Stuart D.Jacksoncontinuous tunability of lasers is desired.On the other hand,the spectral-line broadening leads to lower absorption and emission cross-sections for the same transition in glasses compared to single-crystalline hosts.The reducedcross-sections lead to generally higher pump threshold of laser transitions inglasses,a fact that is compensated infiber geometry because a high pump confinement is achieved over the wholefiber length.4.2Intraionic ProcessesGenerally,the probability of an allowed electric-dipole transition is seven or-ders of magnitude larger than that of an allowed magnetic-dipole transition.Since electric-dipole transitions within the4f subshell are parity forbidden,the intensities of radiative transitions in rare-earth ions are weak and the radiative lifetimes of the emitting states are long,typically in the ms range.Mixing of the4f states with higher-lying(typically5d)electronic states of opposite parity at ion sites without inversion symmetry,however,means thatelectric-dipole transitions become partially allowed and are usually the dom-inant transitions between4f electronic states.The oscillator strengths f and integrated absorption and emission cross-sectionsσof these spin–orbit mul-tiplet-to-multiplet transitions can be calculated with the help of the semi-empirical Judd–Ofelt theory[57,58].If the degree of inhomogeneous spectral-line broadening is relatively small and the absorption and emission spectraremain structured,as is the case for ZBLAN,the cross-sectionsσ(λ)at in-dividual wavelengths that are relevant to pump absorption and stimulatedemission of narrow laser lines must be determined experimentally.Besides ground-state absorption(GSA),excited-state absorption(ESA) of pump photons,see Fig.5a,can play a significant role infiber lasers,specif-ically in the case of high-intensity core pumping.An experimental examplewill be given later in Sect.7.1.Since the absorption increases exponentially with the absorption coefficientα(λP)=Nσ(λP),ESA becomes relevant forthe population dynamics of a laser when(a)the ESA and GSA cross-sectionsσ(λP)are comparable at the pump wavelengthλP and(b)the population density N of the excited state in which the second pump-absorption steporiginates becomes a significant fraction of the density of ions in the ground state,i.e.,a large degree of ground-state bleaching must be present for ESA to play a significant role.A radiative transition from an excited state i to a lower-lying state j is characterized by the radiative rate constant A ij.If the decay occurs to sev-eral lower-lying states,the overall radiative rate constant A i is the sum of all individual rate constants.The branching ratio of each radiative transition is defined asβij=A ij/A i.Radiative decay of excited states is in competition with nonradiative decay by interaction with vibrations of the host material, called multiphonon relaxation.The rate constant of a multiphonon relaxation process decreases exponentially with the energy gap to the next lower-lying state and with the order of the process,i.e.,the number of phonons required(a)Ion (b)12Donor Ion Acceptor IonAcceptor Ion (c)Sensitizing Ion ALaser Ion B(d)1Laser Ion AQuenching Ion B (e)Donor Ion Acceptor Ion(f)1Donor Ion Acceptor Ion Fig.5.Intra-and interionic processes infiber lasers:(a)excited-state absorption (ESA);(b)energy migration;(c)sensitization and(d)quenching of a laser ion by an ion of a different type;(e)cross-relaxation and(f)energy-transfer upconversionto bridge the energy gap[59,60].This fact is illustrated in Fig.1for differ-ent glasses.The rate constant of multiphonon relaxation increases with host temperature.The measurable luminescence lifetimeτi of an excited state i is the inverse of the sum of the overall radiative rate constant A i and the rate constant of multiphonon relaxation,W i.The radiative quantum efficiency is defined asη=A i/(A i+W i).The influence of multiphonon relaxations is stronger in oxides as com-pared tofluorides because of the smaller atomic mass m2of the anion and the larger elastic restoring force k,see(1),due to stronger covalent bonds in oxides[3],both resulting in larger maximum phonon energies in oxides.A brief example:The luminescence lifetime of the4I11/2upper laser level of the erbium3µm laser(Sect.7)is partly quenched by multiphonon relaxation. Typically,nonradiative decay becomes dominant iffive or less phonons are required to bridge the energy gap.With an energy gap between the4I11/2and the next lower lying4I13/2levels of≈3400–3500cm−1,radiative decay pre-vails for phonon energies below≈600cm−1,roughly the maximum phonon energy of ZBLAN,see Table1.Fluorides are,therefore,preferred over oxides as host materials for most of the mid-infrared laser transitions.Like absorption,the strength of a stimulated-emission process is char-acterized by the emission cross-sectionσ(λL)of the laser transition.From a simple analysis,for one resonator round-trip of oscillating laser photons, the productτσ(λL)withτthe luminescence lifetime of the upper laser level, is identified as a“figure of merit”for a possible laser transition.The larger this product,the lower is the expected pump threshold of the laser transition. This“figure of merit”,however,does not take into account the numerous par-asitic effects that can occur in the population dynamics of a laser system,such as pump ESA,reabsorption of laser photons,and energy-transfer processes. It is often these parasitic processes that lead to surprising performance char-acteristics–as likely in the negative as in the positive sense–and make the interpretation of rare-earth-doped solid-state lasers challenging.Examples will be discussed in Sects.5–7.4.3Interionic ProcessesIn addition to intraionic excitation and decay mechanisms,radiative en-ergy transfer due to reabsorption of emitted photons by other active ions in the sample and nonradiative energy-transfer processes due to multipole–multipole or exchange interactions between neighboring active ions can occur. Radiative energy transfer leads to an increase in the luminescence lifetime. Among the nonradiative energy-transfer processes,most common is the elec-tric dipole–dipole interaction,which can occur as a direct[61]or phonon-assisted[62]energy transfer.A direct energy transfer requires spectral reso-nance between the involved emission and absorption transitions whereas an indirect transfer can also be nonresonant,i.e.,an existing energy gap between the emission and absorption transitions involved in the transfer is bridged by。
On the origin and excitation of the extended nebula surrounding NGC 1275
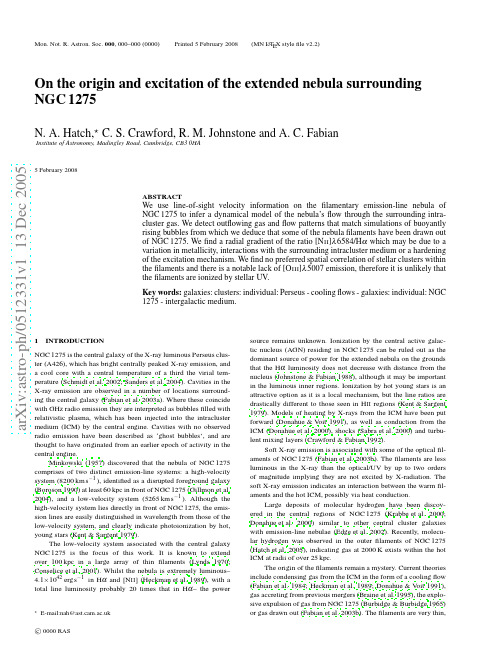
a r X i v :a s t r o -p h /0512331v 1 13 D e c 2005Mon.Not.R.Astron.Soc.000,000–000(0000)Printed 5February 2008(MN L A T E X style file v2.2)On the origin and excitation of the extended nebula surroundingNGC 1275N.A.Hatch,⋆C.S.Crawford,R.M.Johnstone and A.C.FabianInstitute of Astronomy,Madingley Road,Cambridge,CB30HA5February 2008ABSTRACTWe use line-of-sight velocity information on the filamentary emission-line nebula of NGC 1275to infer a dynamical model of the nebula’s flow through the surrounding intra-cluster gas.We detect outflowing gas and flow patterns that match simulations of buoyantly rising bubbles from which we deduce that some of the nebula filaments have been drawn out of NGC 1275.We find a radial gradient of the ratio [N II ]λ6584/H αwhich may be due to a variation in metallicity,interactions with the surrounding intracluster medium or a hardening of the excitation mechanism.We find no preferred spatial correlation of stellar clusters within the filaments and there is a notable lack of [O III ]λ5007emission,therefore it is unlikely that the filaments are ionized by stellar UV .Key words:galaxies:clusters:individual:Perseus -cooling flows -galaxies:individual:NGC 1275-intergalactic medium.1INTRODUCTIONNGC 1275is the central galaxy of the X-ray luminous Perseus clus-ter (A426),which has bright centrally peaked X-ray emission,and a cool core with a central temperature of a third the virial tem-perature (Schmidt et al.2002;Sanders et al.2004).Cavities in the X-ray emission are observed in a number of locations surround-ing the central galaxy (Fabian et al.2003a).Where these coincide with GHz radio emission they are interpreted as bubbles filled with relativistic plasma,which has been injected into the intracluster medium (ICM)by the central engine.Cavities with no observed radio emission have been described as ‘ghost bubbles’,and are thought to have originated from an earlier epoch of activity in the central engine.Minkowski (1957)discovered that the nebula of NGC 1275comprises of two distinct emission-line systems:a high-velocity system (8200kms −1),identified as a disrupted foreground galaxy (Boroson 1990)at least 60kpc in front of NGC 1275(Gillmon et al.2004),and a low-velocity system (5265kms −1).Although the high-velocity system lies directly in front of NGC 1275,the emis-sion lines are easily distinguished in wavelength from those of the low-velocity system,and clearly indicate photoionization by hot,young stars (Kent &Sargent 1979).The low-velocity system associated with the central galaxy NGC 1275is the focus of this work.It is known to extend over 100kpc in a large array of thin filaments (Lynds 1970;Conselice et al.2001).Whilst the nebula is extremely luminous–4.1×1042ergs −1in H αand [N II ](Heckman et al.1989),with a total line luminosity probably 20times that in H α–the power⋆E-mail:nah@source remains unknown.Ionization by the central active galac-tic nucleus (AGN)residing in NGC 1275can be ruled out as the dominant source of power for the extended nebula on the grounds that the H αluminosity does not decrease with distance from the nucleus (Johnstone &Fabian 1988),although it may be important in the luminous inner regions.Ionization by hot young stars is an attractive option as it is a local mechanism,but the line ratios are drastically different to those seen in H II regions (Kent &Sargent 1979).Models of heating by X-rays from the ICM have been put forward (Donahue &V oit 1991),as well as conduction from the ICM (Donahue et al.2000),shocks (Sabra et al.2000)and turbu-lent mixing layers (Crawford &Fabian 1992).Soft X-ray emission is associated with some of the optical fil-aments of NGC 1275(Fabian et al.2003b).The filaments are less luminous in the X-ray than the optical/UV by up to two orders of magnitude implying they are not excited by X-radiation.The soft X-ray emission indicates an interaction between the warm fil-aments and the hot ICM,possibly via heat conduction.Large deposits of molecular hydrogen have been discov-ered in the central regions of NGC 1275(Krabbe et al.2000;Donahue et al.2000)similar to other central cluster galaxies with emission-line nebulae (Edge et al.2002).Recently,molecu-lar hydrogen was observed in the outer filaments of NGC 1275(Hatch et al.2005),indicating gas at 2000K exists within the hot ICM at radii of over 25kpc.The origin of the filaments remain a mystery.Current theories include condensing gas from the ICM in the form of a cooling flow (Fabian et al.1984;Heckman et al.1989;Donahue &V oit 1991),gas accreting from previous mergers (Braine et al.1995),the explo-sive expulsion of gas from NGC 1275(Burbidge &Burbidge 1965)or gas drawn out (Fabian et al.2003b).The filaments are very thin,c0000RAS2N.A.Hatch,C.S.Crawford,A.C.Fabian&R.M.Johnstone long and the majority are radial.Submerged within the ICM,theyenable us to constrain the level of turbulence in the ICM and arguefor a laminarflow.As the ICM moves it may drag the warm opti-cally emitting gas,thus thefilaments can act as streamlines tracing theflow direction(Fabian et al.2003b).None of these problems are exclusive to NGC1275as ex-tended emission-line nebulae are commonly found surrounding other massive galaxies in the centre of X-ray bright‘cool cores’, where the X-ray emission is centrally peaked(Crawford et al. 1999).This work presents new spectroscopic data that explore the kinematic and line-emission properties of the nebula that surrounds NGC1275.After analysing and interpreting the kinematics we put forward a dynamical model of the nebula and discuss the origin of thefilaments.The redshift of NGC1275is0.0176,which using H0=70kms−1Mpc−1,gives1kpc≃2.7arcsec.2OBSERV ATIONS AND DATA REDUCTIONThe data were obtained on the nights of2004Sept23and2004 Oct06using the GMOS North instrument on the Gemini North telescope on Mauna Kea,Hawaii.The sky on both nights was pho-tometric and the seeing was less than0.8arcsec.Six slit positions were chosen using the map of Conselice et al.(2001;see Fig1), selected to feature particular structures of interest.Two bright stars were aligned on each slit so its exact position was known.The slit width was0.5arcsec,filter R831+G590was used and the exposure time for each slit was900seconds(3×300s);all exposures were binned2×2before readout.This setup allows us to explore the observed wavelength range4850–6945˚A.The spectroscopic stan-dards BD+28d4211and G1912B2were observed in order toflux-calibrate the data.Aflat was taken with the GCAL instrument after every slit position observed.CuAr arcs and bias frames were taken with the GCAL instrument on the nights of the2004Sept23and 2004Oct05.A summary of all science exposures taken is given in Table1.The data were reduced using the IRAF Gemini package(ver-sion1.7).The bias frames were combined,flatfields were nor-malised and mosaiced together.All science and standard star frames were reduced by bias subtraction,mosaicing the individ-ual chips together,flatfielding and interpolating across the detector gaps.The arcs were calibrated and checked manually against a line list before the wavelength solution was transferred to the data.The science observations wereflux calibrated using the standard star G1912B2(BD+28d4211was not used as it was observed35◦from the parallactic angle and showed signs of strong atmospheric dif-ferential refraction),andfinally sky-subtracted.The data were de-reddened for Galactic extinction using E(B–V)=0.315.The spec-tra were extracted,converted to ASCII format and analysis was performed using QDP(Tennant1990).The red section covering [O I]λ6300to[S II]λ6731wasfitted separately to a blue section covering the lines of Hβto[N I]λ5199.The He Iλ5876wasfit-ted separately.The lines were assumed to be Gaussian in profile and share the same redshift and velocity width.[N II]λ6548was assumed to have a third of the intensity of the[N II]λ6584line.Acquisition images were taken at all slit positions through the r G0303filter.These images were reduced by bias subtraction,flatfielding,then normalised and combined using the IRAF IM-AGES package to form an R-band image with a scale of0.1454 arcsec/pixel.The image was used to accurately align the slits with the Hαimage from Conselice et al.(2001).Slit1Spectral R831+G590900 Slit1Image rG0303100 Slit2Spectral R831+G590900 Slit3Spectral R831+G590900 Slit3Image rG030360 Slit4Spectral R831+G590900 Slit4Image rG0303300 Slit5Spectral R831+G590900 Slit5Image rG030380 Slit6Spectral R831+G590900 Slit6Image rG0303300On the origin and excitation of the extended nebula surrounding NGC12753Figure1.Position of the six longslit observations on the Hαnebula surrounding NGC1275.The background image is taken from the data of Conselice et al. (2001)within the low velocity system.The spatial connection and similar-ity in colour to the structures within the high-velocity system sug-gest that many of these blue star clusters may be associated with the disrupted foreground galaxy.The high-resolution HST image shows the Hαfilaments themselves to be highly smooth(Figure4) and continuous,consisting of several strands which are individually unresolved on scales of0.25arcsec(corresponding to90parsecs).Excluding the central region where continuum from the galaxy is prominent,our spectra show signs of continuum only at9re-gions.Two of these regions exhibit no line emission so the con-tinuum may have originated from stellar clusters which typically do not have Hαemission(Holtzman et al.1992).The other7re-gions exhibited line emission with very similar line intensity ratios to regions without continuum and only one exhibited[O III]λ5007 emission(see Figure16in section7.3.1).The Hαluminosity from these continuum regions is fairly typical.Although the continuum regions are distinct in the R-band acquisition image as stellar clus-ters,the HST images show that these bright knots are ubiquitous throughout the region stretching beyond the emission-line nebula. These are likely to be chance associations of alignment.It does not appear that the stellar clusters are formed or located within thefil-aments.5KINEMATICS OF THE NEBULAThe morphology of thefilaments suggest they act as streamlines tracing the gasflow in the ICM.Therefore the Doppler shifts of thefilaments may reveal the velocityfield in the core of the clus-ter,near NGC1275.The forces that could drive theflow offil-aments are gravity(from NGC1275),which would draw thefil-aments inwards,or the outward pull following a buoyantly ris-ing radio/ghost bubble as proposed by Bohringer et al.(1995);c 0000RAS,MNRAS000,000–0004N.A.Hatch,C.S.Crawford,A.C.Fabian&R.M.Johnstonebined colour image of the North and West environment of NGC1275as seen through the HST F450W(shown as blue),F702W(green)and F814W(red)broad-bandfilters.The F702Wfilter encompasses Hα+[N II]line emission at the redshift of NGC1275.The image is120arcsec by73arcsec in size.The Northwest and Northernfilaments including the‘horseshoe’loop are visible.A1-arcminute-long chain of blue stellar clusters runs from the top left(above the cluster galaxy)toward the bottom right where it meets the Western edge of the infalling galaxy(high-velocity system)near the bright star.The white arrow points to a region where the detected Hαemission is redshifted by5538km s−1placing it in the low-velocity system.Figure3.A zoom to the Northwest of the previousfigures,showing detail of the loops in the region of the‘horseshoe’filament.The three colour images have each been unsharp-masked to remove the light from the underlying central galaxy.The image is66by38arcsec in size.c 0000RAS,MNRAS000,000–000On the origin and excitation of the extended nebula surrounding NGC12755Figure4.A detailed image of the Hα‘horseshoe’filament as seen through the HST F702W broad-bandfilter,showing thefine structure and smooth-ness of the individual strands.The image is24arcsec on a side,and has been unsharp-masked(i.e.it has had a highly smoothed version of itself subtracted)to remove the underlying gradient due to the light from the cen-tral galaxy continuum.Churazov et al.(2001);Reynolds et al.(2005).A three dimensional flow pattern can differentiate between galactic outflow and inflow models,and thus constrain the origin of thefilaments.The ve-locities are determined from binning the spatial dimension of the longslit spectra in bins of4pixels(0.58arcsecond).All veloci-ties presented are heliocentric and the line-of-sight zero point is defined as the velocity of NGC1275,assumed to be5265kms−1 (Ferruit et al.1997).All distances referred to are projected dis-tances.5.1NorthernfilamentThe Northernfilament is the dominant long(∼60kpc),thin (<1kpc)structure stretching radially North-South,situated North of NGC1275;slit6was positioned along thisfilament.The veloc-ity structure of the Hαand[N II]lines is shown in Figure5.The filament appears extremely radial for the majority of its length sug-gesting that the dominant direction offlow is also radial.As this filament is the only structure detected so far out from the galaxy it is extremely unlikely to be in projection with anotherfilament,and therefore we assume it to be a single structure.It is improbable that we should be viewing an intrinsically-curvedfilament as a linear one,so we assume that it is intrinsically straight.Thefilament has a kinematic North-South divide:the North displaying a velocity blueshift by up to−180km s−1,whereas the South is erratically redshifted.The Northern half(above37kpc) is clumpy on scales of up to5kpc in length.Each clump exhibits smooth velocity gradients,although there are velocity discontinu-ities between the clumps.It is possible that some of the velocity could be due to thefilament twisting as it falls(note the helical na-ture of the lower parts of thefilament,Fig.5).The Southern part of thisfilament is split into two vertical segments:slit6covers only the western dimmer segment,whilst slits1and2slice across both. The Western segment is very thin and redshifted.The Eastern seg-ment appears thicker and clumpier,and slit2shows that the emis-sion is blueshifted.Slit1shows that the very bottom of the Eastern segment is redshifted.The kinematic North-south divide indicates the lower part of thefilament is moving in the opposite direction to the upper part of thefilament:thefilament is either being stretched or is collaps-ing,depending on whether it is orientated toward or away from the observer.Half of thisfilament must be falling into the galaxy,and the other half must beflowing away from the galaxy.Thus we can immediately rule out a model in which thefilaments are smoothly falling onto the galaxy below.The Doppler shifts alone do not en-able us to determine which end of thefilament is inflowing or out-flowing since we then need to know the inclination.However,as part of thefilament must beflowing away from the galaxy,there must be a mechanism for drawing gas away from the galaxy.We note that the Southern end of the three radial Northern filaments coincides with a shock front seen in the X-ray images (Fabian et al.2003a).This front is due to the formation of the inner Northern bubble around the radio source which is a cyclical pro-cess taking place every107yr or so(as indicated by the presence of the outer ghost bubbles).If an expanding shock front destroys the emission-linefilaments,then the lower part of the Northernfila-ments must previously have been at a larger radial distance in order to have survived the shock emitted from the Northwest ghost bub-ble when it was forming.Therefore the lower half of the Northern filament is probably moving inward,whilst the upper segment is moving outward,i.e.thefilament is likely to be stretching.There is a depression in the thermal pressure just above the Northernfilament(Fabian et al.2005),that could be a remnant of a ghost bubble that has buoyantly risen from the central region. We can now interpret thefilament in the context of the rising bubble models of Bohringer et al.(1995);Churazov et al.(2001); Fabian et al.(2003b);Reynolds et al.(2005).The radialfilament morphology traces the primary direction offlow therefore it acts as a streamline.Part of thefilament isflowing away from the galaxy due to the uplift caused by the ghost bubble’s buoyant rise through the ICM,whilst the other half has been overcome by the galaxy’s gravity and is nowflowing back.The pull from the bubbles com-petes with gravity.For a totalfilament length of25kpc and a range in velocity of 400km s−1the dispersion time is6×107years;if thefilament is at a small angle from the plane of the sky(as is likely due to its large projected length)the velocity range may be much larger,reducing the dispersion time.5.2Northwestfilaments and‘horseshoe’featureTo the Northwest of the galaxy lies an array of radialfilaments, one of which extends30kpc from the nucleus and ends in a curved loop that Conselice et al.(2001)refer to as the‘horseshoe’(detail in Fig.4).The loop is positioned underneath a ghost bubble visible as a prominent depression in the X-ray image(Fabian et al.2003a).The morphology of thesefilaments has previously been noted to resemble theflow underneath an air bubble rising in water (Fabian et al.2003b).Figure6details the line-of-sight velocities of thesefilaments.The long radialfilament(Western part of the ‘horseshoe’loop)begins with a redshifted line-of-sight velocity of95kms−1which remains fairly constant for5kpc until the line emission shifts Southwest beyond the slit for3kpc,to reappear at ac 0000RAS,MNRAS000,000–0006N.A.Hatch,C.S.Crawford,A.C.Fabian &R.M.JohnstoneFigure 5.Line-of-sight velocities of the Northern filaments.Purple-blue indicate blueshifted emission,yellow-red indicate redshifted emission,whilst green has zero velocity relative to the central galaxy.Velocities from slit 1(bottom)and slit 2(top)which cut across the Northern filament are displayed in the image but not shown in graph.Background images are from the data of Conselice et al.(2001).distance of 18kpc from the galaxy with a velocity of 60kms −1.The difference of 35kms −1between the two sides of this gap cannot be unambiguously attributed to a change in speed as a small change in orientation to the plane of our line of sight could also produce the observed velocity deviations.From 18kpc upward,this filament divides into two velocity structures before curving into the loop which starts at 22kpc (see right panel of Fig.6).These velocity structures have smooth gra-dients with no small scale random deviations in excess of the error (1-10km s −1depending on the line strength).The low velocities,morphological structure of the filaments,and the spherical cap ap-pearance of the ghost bubble,indicate the filament may be close to being in the plane of the sky.The curved part of the ‘horseshoe’starts at a projected dis-tance of 22kpc from the nucleus where the velocity increases rapidly to 200kms −1,and remains steady over 4kpc.The top of the loop has the highest velocity,peaking at 300kms −1,then slow-ing down to 200kms −1as the loop turns over.On the short side of the loop the emission is still redshifted but the velocity dies to 60kms −1in under 1kpc and remains steady for the rest of the loop.Above the loop is gas with a blueshifted line-of-sight velocity of –230kms −1,a jump in velocity space of more than 480kms −1over a projected distance of 3kpc.Further above the central axis of the bubble slit 5cuts across some dim emission which is also blueshifted.The gas in the loop and above the loop surrounds theghost bubble suggesting the gas above the loop is part of the same structure as that in the loop and is not just a projection effect.The flow pattern qualitatively matches the simulations of Reynolds et al.(2005)of a bubble rising through a viscous ICM:gas above the bubble flows in the opposite direction to the gas be-low the bubble,and the largest velocities occur near the central axis and close to the bubble.If the flow of cool gas starts from the galaxy,to stream up the long straight side,around the curve and down the short straight side,we would expect the material in the short straight side to be flowing in the opposite direction to the long straight side,similar to that in an eddy,with blueshifted emission underneath the bubble.Indeed the emission from the short straight side is blueshifted relative to the rest of the loop:at a height of 25kpc from the nucleus,one side of the loop has a velocity of 200kms −1whereas the other side is at 60kms −1.The agreement of the velocities,morphology and the clear ghost bubble visible in the X-ray images of the Perseus clus-ter (Fabian et al.2003a),suggest the most likely dynamical model is one in which the loop and radial filament is flow-ing out of the galaxy,with the filament slightly orientated away from us.As the bubble has risen (possibly with velocities of 700kms −1Fabian et al.2003b),it has dragged up cool material from the galaxy producing the filamentary structure we observe.c0000RAS,MNRAS 000,000–000On the origin and excitation of the extended nebula surrounding NGC 12757-200-1000100200300Line-of-sight velocity (km/s)101520253035P r o j e c t e d d i s t a n c e f r o m g a l a x y c e n t r e (k p c )050100150200250300Figure 6.Left:Line-of-sight velocities along the ‘horseshoe’loop.Positive velocities are red,blue indicates negative velocities relative to galaxy.Right:Left panel shows velocities on the short straight of the loop covered by slit 3(triangles)and along the top of the loop,covered by slit 2(stars).Right panel shows data from the long straight on the right-hand side covered by slit 4(crosses)and data from slit 5that crossed through the loop (squares).Only data above dashed line is presented in image.Background image is from the data of (Conselice et al.2001).Figure 7.Line-of-sight velocities of the tangential filament running along the Northeast of NGC 1275.Purple-blue indicate blueshifted emission,yellow-red is redshifted emission and green has zero velocity relative to the central galaxy.Data from slit 5is presented in the graph with crosses.Slit 3also covered some nearby regions which have been marked by the square symbols.Luminous radial filaments extending from the galaxy to the tangential filament appear at the bottom right of the image.Background image is from the data of Conselice et al.(2001).c0000RAS,MNRAS 000,000–0008N.A.Hatch,C.S.Crawford,A.C.Fabian &R.M.Johnstone5.3Tangential filamentFig.7shows the line-of-sight velocities of the filament that appears to run tangentially along the North of the galaxy.Slit 3also covered line emitting regions situated between the tangential filament and the galaxy whose velocities have been added into Figure 7as square symbols.The velocity structure along 14.5kpc varies without large ve-locity jumps so the Eastern section of the filament appears to be a coherent structure.The emission is blueshifted to a similar velocity along ∼10kpc of its length then smoothly decreases in speed at the most Eastern end.Small scale deviation from the large scale trends are seen in excess of the error (∼1–10km s −1depending on the line intensity),suggesting some small scale random variations in velocity.Beyond 14.5kpc the emission first jumps by –80kms −1in velocity then jumps again by almost +400kms −1.The Western section of this filament is not coherent in velocity space,and the morphology suggests that slit 5is slicing across radial filaments that extend to the Northwest,in the same direction as the ‘horse-shoe’feature.The Eastern section is a puzzling structure since it is tangential,unlike the majority of the filaments.Interpretation of this region is complicated by the presence of the Northern radio lobe and complex X-ray emission.6FILAMENT LINE-WIDTHS AND VELOCITIES The FWHM (full width at half maximum)of the instrumental profile is 85km s −1(2.85pixels)at H αdetermined from nearby sky lines.Most of the material has FWHM line-widths of 50-160kms −1after correcting for the instrumental broadening (Fig.8),much greater than the thermal width of hydrogen gas at 10,000K (∼20kms −1).If the filaments represent an inflow of gas as predicted by early models of cooling flows (Fabian et al.1984)we would expect an anti-correlation between line-widths and radial distance from the nucleus and extremely large (∼500–1000kms −1)central line-widths (Heckman et al.1989).We observe a few points within 10kpc that have large (>200kms −1)line-widths.It is within 10kpc of the galactic centre that the density of the line-emitting filaments increases greatly and there are many regions where the spectra display double peaked lines indicating that the line-of-sight crosses at least two clumps of line-emitting material which have different kinematics.It is not necessary that these clumps be physi-cally close,therefore they do not imply small-scale velocity devia-tions along a single filament as observed in slit 5.Examples of such regions are shown in Fig.9,and were either resolved into two sets of lines or removed from the dataset if the result was ambiguous.However,it is likely that some of these central regions would have clumps with similar line-of-sight velocities and result in spectra with a single wide peak.Most regions with large line-widths also have a large H αsurface brightness (Fig.8),therefore it is probable that the spectra from these inner regions are caused by filaments overlapping in the same line-of-sight with slightly different veloc-ities.Beyond the inner 10kpc,the line-widths are uniformly 2–8times the thermal width of gas at 10,000K.Some radially extend-ing filaments exhibit similar line-widths along their whole length (see Fig.14in section 7).Therefore the line-widths provide no ev-idence to suggest the nebula flows into the galaxy.No line-of-sight velocity greater than 350kms −1was de-tected.This work primarily probes the outer filaments,which are likely to have small angles from the plane of the sky due to their large projected distance from the galaxy,and therefore are not ex-pected to have large line-of-sight velocities.Cigan et al.(2004)0102030405060Projected radial distance from galaxy centre (kpc)50100150200250300L i n e w i d t h (k m s -1)0102030405060H α (10-15 erg s -1cm -2arcsec -2)Figure 8.Left :Radial projection of emission line widths in kms −1based on the H αand [N II ]lines.Right :Line width verses H αsurface brightness.Most points with large line-widths (>200km s −1)are within the central few kpc and have large H αsurface brightness suggesting they may be overlap-ping filaments.Figure 9.Examples of central clumps which show double-peaked line emis-sion.These regions are all centrally located.HV denotes emission from the high-velocity system which lies infront of NGC 1275.who probe the central regions as well as the outer filaments find no velocities greater than 450kms −1.In section 5.2we argue that the ‘horseshoe’feature and the Northwest filaments covered by slit 4are very close to being in the plane of the sky.Therefore the observed line-of-sight velocity of 200kms −1,at the top of the ‘horseshoe’loop could transform to a velocity much greater than 700kms −1(expected if tilted by a conservative 75◦from the line-of-sight).This is far beyond what is observed in the rest of the dataset.It is possible that the two inner radio lobes have pushed or destroyed the filaments pointing toward our line-of-sight.7SPECTRAL FEATURESOur data probe the outer nebula that extends beyond 10kpc in de-tail for the first time.All intensity line ratios and line intensities are determined from binning the spatial dimension of the longslit spec-tra in bins of 6pixels (0.87arcsecond).A typical spectrum is shown in Fig.11and line intensity ratios are summarised in Table 2.Allc0000RAS,MNRAS 000,000–000。
迪伦弹性加热器指南说明书
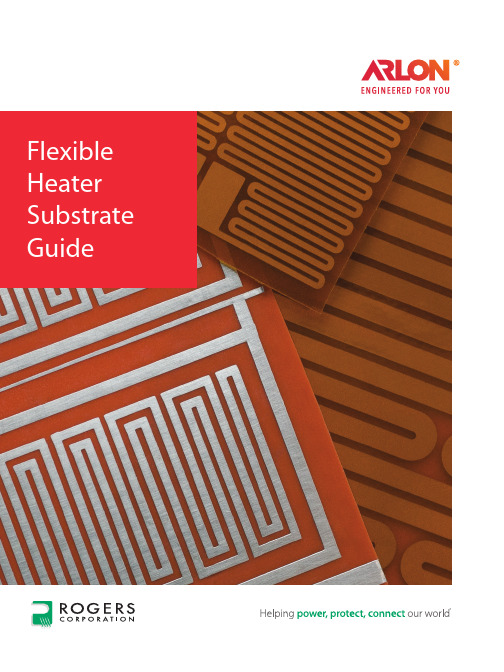
FlexibleHeaterSubstrateGuide®LightweightThin design minimizes the material neededGood Thermal Control Rapid heating and distribution of thermal energy evenly across the surfaceHighly Conformable Bendable and fl exible; can conform to complex geometriesDurableResistant to ozone, moisture, and many common environmental chemicalsCustomizableAvailable in various thicknesses and several colorsQuality Engineering Precisely engineered to customer specifi cationsThermally Stable Thermally stable from -50ºC to 232ºC (-58ºF to 450ºF)Flame ResistantHorizontal burn rated at UL 94 HB. V-0 options availableHigh Dielectric Strength Both silicone and polyimide have dielectric strengths exceeding 500 V/milBondableCompatible with silicone pressure sensitive adhesive; can be bonded to wire or metal foil elementsEconomical Cost-eff ective solutionsReliableRogers’ fl exible heater reputation is unmatched in the industryARLON® Flexible Heater DielectricsStandard Silicone DielectricThe use of thin, fl exible siliconerubber/fi berglass fabric composite materials is the standard for high temperature heaters. Rogers manufactures a full line of silicone rubber/fi berglass fabric composites with UL-recognized relative thermal index (RTI) ratings up to 220ºC (428ºF), fl ammability ratings for horizontal burn (HB), and the highest resistance to vertical burn (UL94 V-0) for electrical insulation of fl exible heaters. These materials are available with pre-bonded resistive foils, such as Inconel 600 and stainless steel. This eliminates the initial bonding step which realizes reduced processing costs. Rogers’ silicone rubber/fi berglass fabric composites operate reliably, and off er long service life in both wire-woundand etched foil heater applications.Figure 1. Wire-wound fl ex heater with silicone substrate.ARLON Heater SubstratesRogers off ers two lines of substrates for fl exible heaters: silicone rubber and polyimide.raPId Polyimide DielectricARLON raPId polyimide substrates are comprised of a revolutionary new construction that incorporates the benefi ts of polyimide heater dielectrics with the fl exibility and usability of a silicone adhesive system. The raPId substrates process in less time and at lower temperatures than competitive acrylic or FEP adhesive systems with zero circuit swimming. The unique silicone adhesive system enables the manufacture of etched-foil and wire-wound fl exible heaters with improved thermal stability and low outgassing performance. Flexible heaters manufactured with raPId substrates have a continous operating temperature of 220ºC (428ºF). They can be bonded to wire or metal foilelements at 121ºC (250ºF). In the event of an over-temperature situation, either due to a hot spot or a runaway heater, ARLON raPId substrates will not melt like FEP fluoropolymer.Figure 2. Etched-foil (top) and wire-wound (bottom)fl ex heaters with polyimide substrates.ARLON Heater SubstratesTypical Material Properties Silicone vs. PolyimideThis section outlines the advantages of each substrate option—silicone and polyimide—and outlines thespecific design options for each.Silicone vs. PolyimideProduct Naming ConventionFlexible Heater Product ExamplesFlexible Heater Product ExamplesThe information contained in this Design Guide is intended to assist you in designing with Rogers’ Elastomeric Material Solutions. It is not intended to and does not create any warranties, express or implied, including any warranty of merchantability or fitness for a particular purpose or that the results shown in this Design Guide will be achieved by a user for a particularpurpose. The user should determine the suitability of Rogers’ ARLON products for each application. The Rogers logo, Helping power, protect, connect our world, ARLON and the ARLON logo are trademarks of Rogers Corporation or one of its subsidiaries. Kapton is a trademark of the Dupont Company. © 2018 Rogers Corporation. All rights reserved. 1118-2.5 • Publication #202-178Rogers CorporationElastomeric Material Solutions1100 Governor Lea Road, Bear, DE 19701 USA Phone: (302) 834-2100Fax: (302) /arlon ************************®。
GT-MHRPROJECT

GT-MHR PROJECTA. KIRYUSHIN, N. KODOCHIGOVOKB Mechanical Engineering15, Burnakovsky proezd, 603074 Nizhny Novgorod,Russian FederationABSTRACTThe paper presents new generation reactor - modular helium reactor with gas turbine(GT-MHR), which satisfies the requirements of the developing nuclear power.The paper describes the reactor plant and peculiarities of GT-MHR technical concept, suchas high efficiency (about 48%) of electric power generation, increased safety of the plant,etc.The technologies, which are the innovative essence of GT-MHR project, are:(1)fuel in the form of fuel particles with multi-layer ceramic coatings which confinefission products at high temperatures (of about 1600°);(2)large gas turbines;(3)single-shaft vertical turbomachine design;(4)electromagnetic bearings;(5)high-effective compact heat exchangers.Main technical and economical indices of GT-MHR project are presented.1. IntroductionOne of new generation power plants meeting the requirements of developing wide-scale atomic energy is gas turbine modular helium reactor plant (GT-MHR).In 1997 GT-MHR Conceptual Design which has been reviewed several times was developed within the framework of 4-lateral Agreement between Minatom of Russia General Atomics, Framatome, Fuji Electric. International Project review was held in France (June, 1999) with participation of independent experts of Russia, USA, Japan and European Community. Review results showed that there are no insurmountable difficulties for the Project implementation. Technical and financial risk may be reduced with international cooperation.Main goals of the GT-MHR Project development are as follows:(1)creation of the plant meeting the requirements imposed on innovative technologies of the XXI-st century as for safety, competitiveness and minimization of radiation and thermal impact to environment;(2)commissioning of the first GT-MHR module not later than 2010, minimizing R&D by use of accumulated world experience on the HTGR technology;(3)creation of base for further commercial application of this technology to generate power and heat for domestic and industrial needs, including hydrogen fabrication;(4)use of the first and next modules for excessive weapon-grade plutonium burnup.GT-MHR Project is included into “Strategy for atomic power development in Russia for the first half of the XXI century”. Strategy stages Stipulate: “Participation in the international project on the GT-MHR NPP development and construction” till 2010 and “Pilot operation of the GT-MHR prototype module and fuel fabrication for it (within the framework of the international Project)”till 2030.The GT-MHR Project is included into the Russian Federal purpose-oriented Program “Power effective economy” for 2002-2005 and 2010.Survey of the GT-MHR fuel cycle showed good capabilities of the reactor to burn weapon-grade plutonium, therefore, the GT-MHR Project was included into activities program within the framework of appropriate agreement on research and technical cooperation between Russia and USA dated July 24, 1998.International cooperation of enterprises in Russia, USA, France, Japan (OKBM, RRC “KI”, VNIPIET, SCC, NPO “Luch”, General Atomics, Oak Ridge National Laboratory, Framatome ANP, Fuji Electric, etc.) takes place to implement the GT-MHR Project. Hundreds of experts accumulating knowledge and experience on HTGR are working on the Project.2. Main activity stages on the GT-MHR prototype module project•Preliminary Design– 2001•Final Design– 2005•Commissioning of fuel fabrication for prototype NPP– 2008•Commissioning of prototype NPP– 2010Prototype NPP Project includes: development of nuclear power plant with the GT-MHR reactor plant, stationary fuel fabrication for 250 kg per year.Main activity directions for the Project are turbomachine, recuperator and fuel fabrication, including reactor tests.3. GT-MHR technical conceptPrototype NPP with the GT-MHR RP includes one power module with the GT-MHR reactor module of 600 MW thermal capacity. GT-MHR reactor module consists of two integrated units: modular high-temperature reactor and power conversion system with direct gas-turbine cycle (Figure. 1).The reactor includes core and metalworks, which supportit and form the coolant circulation path.The power conversion system implementing closed gas-turbine cycle is completely arranged in PCS vessel. Theturbomachine consists of generator, gas turbine, and twocompressor sections mounted in a single-shaft structurecompletely suspended on electromagnets. PCS includesthree compact heat exchangers: high efficiencyrecuperator, water-cooled precooler and intercooler.The reactor unit and associated primary systems arearranged in the underground building (Figure 2).The schematic diagram of GT-MHR plant is given inFigure 3.1 – generator;2 – recuperator module;3 – turbocompressor;4 – intercooler module;5 – precooler module;6 –reactivity control system assembly;7 – core; 8 – vessel system;9 – reactor shutdown cooling systemFig.1 GT-MHR reactor moduleFig. 2 GT-MHR module1 – reactor,2 – turbine,3 – recuperator,4 – precooler,5 – low-pressure compressor,6 – intercooler,7 – high-pressure compressor,8 – generator,9 – generator cooler, 10 – bypass valve of the TM control and protection system, 11 – heat exchanger of the power conversion unit (PCU) cooling water system (CWS), 12 – PCU CWS pump, 13 – recirculation water supply system pump, 14 – cooling tower,15 – reactor shutdown cooling system (SCS) unit heat exchanger, 16 – SCS unit gas circulator,17 - SCS unit gas circulator isolation valve, 18 - SCS CWS heat exchanger, 19 – SCS CWS pump,20 – reliable recirculation water supply system pump, 21 – reactor shutdown cooling system surface cooler, 22 – air ducts, 23 – heat exchanger with heat pipes, 24 – primary circuit purification system,25 – helium transportation and storage system.Fig. 3 Principle diagram of the GT-MHR NPP system equipment Refueling RCCS ReactorSCS PCUPCU CWSMain indices of the GT-MHR prototype plant are presented below.Description Indices Plant capacity:- thermal, MW - electric, MW 600 285Power conversion cycle Brayton, with gas turbine in the primarycircuitPower conversion system efficiency∼ 48 %Core type Annular of prismatic FAFuel type Microspheres of multi-layer ceramiccoatingPower density 6.5 MW/m3Average fuel burn up640 MW⋅day/kgFuel life duration750 days – for plutonium fuel Operation duration between refueling250 daysRefueling ratio3Design service life of basic equipment60 yearsTotal construction cost355 US M $Electric power prime cost 1.62 cent/kWHigh level of the GT-MHR safety is stipulated by structural features and physical properties of the core, as well as technical solutions adopted in the project:(1)use of fuel in the form of small particles (of 200 µm diameter) with multi-layer pyrocarbon and silicon carbide coating, which are capable of effective fission products confinement at high temperatures (of up to 1600°С) and fuel burnup;(2)structural features of the core and reactor (annular core geometry, low specific power). These design features and high heat accumulating capacity of the core ensure reactor shutdown cooling under emergency conditions owing to passive heat removal from the reactor vessel by irradiation, thermal conductivity and convection at the fuel and core temperature maintaining within allowable limits for safe operation (<1600°), including loss of coolant;(3)negative feedback between the core temperature and reactor power (negative reactivity temperature coefficient);(4)use of graphite as the core structural material including control rods that excludes the core damages.The GT-MHR fuel cycle concept allows obtaining deep burnup of fission material and disposing spent fuel without additional processing.Nuclear materials non-proliferation mode at use of weapons-grade plutonium as fuel is ensured for the entire fuel cycle beginning from fresh fuel fabrication. It is related to the fact that at present there is no processing technology of fuel with ceramic coatings, it is needed to perform fuel-scaled processing due to low fuel concentration inside the graphite block (< 0.1 %), and it is also related to the fact that about 30% of Pu-240 is contained in plutonium being unloaded.Flexible fuel cycle with possible use of various fuel types (U, Pu, U-Pu, U-Th) can be implemented as applied to the GT-MHR reactor within the boundaries of the same core design ensuring stability of its main characteristics and fulfilling safety regulatory document requirements.Considerable reduction of thermal and radiation impact to the environment per generated power unit compared to other reactor plant types, is ensured as a consequence of high efficiency and effective radioactive products confinement by safety barriers and by multi-layer ceramic coating of fuel particles.NPP with the GT-MHR RP allows power and heat co-generation due high value of low coolant temperature in gas-turbine cycle (∼ 130 O C) without any changes in power conversion unit. This double function is of unique importance in the climatic conditions of Russia. It is proven by the data on annual natural gas consumption for power and heat generation which amounts to about 135 and about 220 billion m3, respectively. In this case reactor thermal power is completely used, that considerably improves NPP economic indices.4. ConclusionImplementation of program for creation of NPP with the GT-MHR, involving international intellectual, process and financial resources allows:(1)broadening opportunities of weapons-grade plutonium disposition program in Russia, fulfilling requirement of agreement on nuclear material non-proliferation;(2)creating the base for further commercial application of this plant type for both power generation with high efficiency and power and heat co-generation with complete use of reactor thermal power; (3)using experience on development and operation of NPP with the GT-MHR for further application of this high-temperature heat generation technology for various processes, especially for production of hydrogen and synthetic liquid fuel.。
冷原子光谱法 英语

冷原子光谱法英语Okay, here's a piece of writing on cold atom spectroscopy in an informal, conversational, and varied English style:Hey, you know what's fascinating? Cold atom spectroscopy! It's this crazy technique where you chill atoms down to near absolute zero and study their light emissions. It's like you're looking at the universe in a whole new way.Just imagine, you've got these tiny particles, frozen in place almost, and they're still putting out this beautiful light. It's kind of like looking at a fireworks display in a snow globe. The colors and patterns are incredible.The thing about cold atoms is that they're so slow-moving, it's easier to measure their properties. You can get really precise data on things like energy levels andtransitions. It's like having a super-high-resolution microscope for the quantum world.So, why do we bother with all this? Well, it turns out that cold atom spectroscopy has tons of applications. From building better sensors to understanding the fundamental laws of nature, it's a powerful tool. It's like having a key that unlocks secrets of the universe.And the coolest part? It's just so darn cool! I mean, chilling atoms to near absolute zero? That's crazy science fiction stuff, right?。
中子辐照半绝缘SiC单晶的光学性质

王鹏飞等:中子辐照半绝缘SiC单晶的光学性质・ 353 ・第41卷第3期DOI:10.7521/j.issn.0454–5648.2013.03.13 中子辐照半绝缘SiC单晶的光学性质王鹏飞,阮永丰,侯贝贝,陈敬(天津大学理学院,天津 300072)摘要:利用荧光光谱、紫外–可见–近红外透射光谱和Raman光谱对经1.67×1020 n/cm2中子辐照的半绝缘SiC的光学性质进行了研究。
结果表明:中子未辐照的和辐照后退火温度低于1000℃的样品未出现任何发射峰;1200℃退火的样品在510、540和575nm处出现了3个绿色发射峰,其中,510和540nm处的发射峰在经过1600℃退火后依然存在。
中子辐照导致SiC的截止波长由393nm增大到1726nm;随退火温度提高,截止波长逐渐减小,并在1600℃退火后完全回复。
Raman光谱显示:辐照诱发了许多新的振动模,如187、278、435和538cm–1处的Si—Si键振动模,600、655和709cm–1处的Si—C键振动模和1419cm–1处C—C键振动模。
辐照缺陷引起声子限制效应,表现为FTO2/6和FLO0/6Raman特征峰的不对称性展宽和红移。
在低于1000℃退火阶段,主要表现为不对称性展宽和红移逐渐减弱;在高于1000℃退火阶段,主要表现为辐照产生的新Raman峰逐渐消失。
关键词:碳化硅;中子辐照;光学性质中图分类号:O77+4 文献标志码:A 文章编号:0454–5648(2013)03–0353–06网络出版时间:网络出版地址:Optical Properties of Neutron-irradiated Semi-insulating SiC Single CrystalsWANG Pengfei,RUAN Yongfeng,HOU Beibei,CHEN Jing(School of Science, Tianjin University, Tianjin 300072)Abstract: The optical properties of semi-insulating SiC neutron irradiated up to 1.67×1020 n/cm2 were analyzed by photolumines-cence (PL) spectroscopy, UV–Vis–Nir transmission spectroscopy and Raman spectroscopy, respectively. The samples neutron irradi-ated and post-irradiation annealed below 1000℃had no PL peaks. However, the 510, 540 and 575nm peaks appeared after thermal treatment at 1200℃. The 510 and 540nm peaks could maintain after thermal treatment at 1600℃. The neutron irradiation led to the increase of threshold wavelength from 393nm to 1726nm. The threshold wavelength decreased with increasing annealing tempera-ture, and recovered after thermal treatment at 1600℃. Several additional vibration modes appeared after irradiation. The peaks at 187, 278, 435 and 538cm–1 were related to Si–Si vibration, the peaks at 600, 655, and 709cm–1 originated from the Si–C vibration, and the peak at 1419cm–1 arised from the C–C vibration. The phonon confinement effect was also observed for FTO2/6 and FLO0/6 peaks, showing the asymmetric broadening and red shift. The thermal evolution of Raman spectra with annealing temperature was that the asymmetric broadening and red shift was diminished during annealing at <1000℃, but the irradiation-produced additional Raman peaks were removed during annealing at >1000℃.Key words: silicon carbide; neutron irradiation; optical propertySiC是一种重要的半导体材料,具有带隙宽、热稳定性好、临界击穿电压高等优良特性,可用于制造高温、高频、大功率电子器件[1]。
新晋院士马军简介
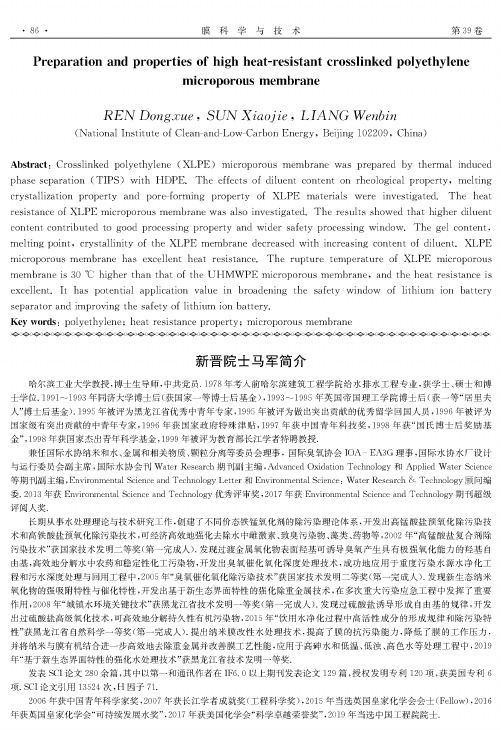
-86-膜科学与技术第39卷Preparation and properties of high heat-resistant crosslinked polyethylenemicroporous membraneREN Dongxue,SUN Xiaojie,LIANG Wenbin(National Institute of Clean-and-Low-Carbon Energy,Beijing102209,China)Abstract:Crosslinked polyethylene(XLPE)microporous membrane was prepared by thermal induced phase separation(TIPS)with HDPE.The effects of diluent content on rheological property,melting crystallization property and pore-forming property of XLPE materials were investigated.The heat resistance of XLPE microporous membrane was also investigated.The results showed that higher diluent content contributed to good processing property and wider safety processing window.The gel content, melting point,crystallinity of the XLPE membrane decreased with increasing content of diluent.XLPE microporous membrane has excellent heat resistance.The rupture temperature of XLPE microporous membraneis30C higherthanthatoftheUHMWPE microporousmembrane,andtheheatresistanceis excellent.It has potential application value in broadening the safety window of lithium ion battery separator and improving the safety of lithium ion battery.Key words:polyethylene;heat resistance property;microporous membrane新晋院士马军简介哈尔滨工业大学教授,博士生导师,中共党员.1978年考入前哈尔滨建筑工程学院给水排水工程专业,获学士、硕士和博士学位.1991〜1993年同济大学博士后(获国家一等博士后基金),1993〜1995年英国帝国理工学院博士后(获一等“居里夫人”博士后基金).1995年被评为黑龙江省优秀中青年专家,1995年被评为做出突出贡献的优秀留学回国人员,1996年被评为国家级有突出贡献的中青年专家,1996年获国家政府特殊津贴,1997年获中国青年科技奖,1998年获“国氏博士后奖励基金”,1998年获国家杰出青年科学基金,1999年被评为教育部长江学者特聘教授.兼任国际水协纳米和水、金属和相关物质、颗粒分离等委员会理事,国际臭氧协会IOA-EA3G理事,国际水协水厂设计与运行委员会副主席,国际水协会刊Water Research期刊副主编,Advanced Oxidation Technology和Applied Water Science 等期刊畐!J主编,Environmentll Science and Technology Letter和Environmental Science:Water Research&Technology顾问编委.2013年获Environmental Science and Technology优秀评审奖,2017年获Environmental Science and Technology期刊超级 评阅人奖.长期从事水处理理论与技术研究工作,创建了不同价态铁猛氧化剂的除污染理论体系,开发出高猛酸盐预氧化除污染技术和高铁酸盐预氧化除污染技术,可经济高效地强化去除水中雌激素、致臭污染物、藻类、药物等,2002年“高猛酸盐复合剂除污染技术”获国家技术发明二等奖(第一完成人)•发现过渡金属氧化物表面径基可诱导臭氧产生具有极强氧化能力的径基自由基,高效地分解水中农药和稳定性化工污染物,开发出臭氧催化氧化深度处理技术,成功地应用于重度污染水源水净化工程和污水深度处理与回用工程中,2005年“臭氧催化氧化除污染技术”获国家技术发明二等奖(第一完成人).发现新生态纳米氧化物的强吸附特性与催化特性,开发出基于新生态界面特性的强化除重金属技术,在多次重大污染应急工程中发挥了重要作用,2008年“城镇水环境关键技术”获黑龙江省技术发明一等奖(第一完成人).发现过硫酸盐诱导形成自由基的规律,开发出过硫酸盐高级氧化技术,可高效地分解持久性有机污染物,2015年“饮用水净化过程中高活性成分的形成规律和除污染特性”获黑龙江省自然科学一等奖(第一完成人)•提出纳米膜改性水处理技术,提高了膜的抗污染能力,降低了膜的工作压力,并将纳米与膜有机结合进一步高效地去除重金属并改善膜工艺性能,应用于高H水和低温、低浊、高色水等处理工程中,2019年“基于新生态界面特性的强化水处理技术”获黑龙江省技术发明一等奖.发表SCI论文280余篇,其中以第一和通讯作者在IF6.0以上期刊发表论文129篇,授权发明专利120项,获美国专利6项.SCI论文引用13524次,H因子71.2006年获中国青年科学家奖,2007年获长江学者成就奖(工程科学奖),2015年当选英国皇家化学会会士(Fellow),2016年获英国皇家化学会“可持续发展水奖”,2017年获美国化学会“科学卓越荣誉奖”,2019年当选中国工程院院士.。
Solar Carbon Monoxide, Thermal Profiling, and the Abundances of C, O, and their Isotopes
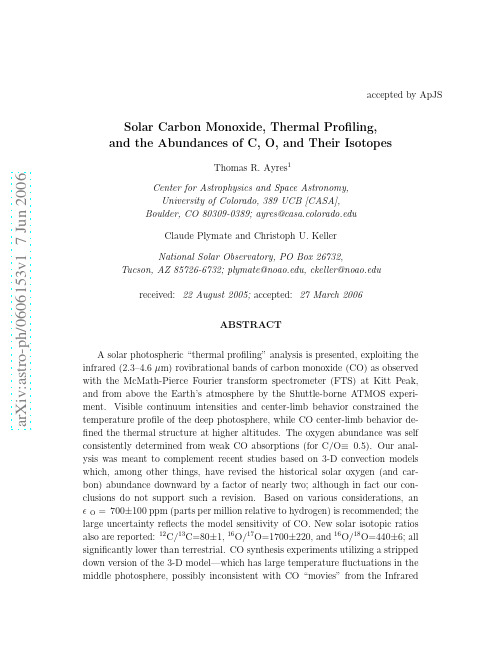
a r X i v :a s t r o -p h /0606153v 1 7 J u n 2006accepted by ApJSSolar Carbon Monoxide,Thermal Profiling,and the Abundances of C,O,and Their IsotopesThomas R.Ayres 1Center for Astrophysics and Space Astronomy,University of Colorado,389UCB [CASA],Boulder,CO 80309-0389;ayres@ Claude Plymate and Christoph U.Keller National Solar Observatory,PO Box 26732,Tucson,AZ 85726-6732;plymate@,ckeller@ received:22August 2005;accepted:27March 2006ABSTRACT A solar photospheric “thermal profiling”analysis is presented,exploiting the infrared (2.3–4.6µm)rovibrational bands of carbon monoxide (CO)as observed with the McMath-Pierce Fourier transform spectrometer (FTS)at Kitt Peak,and from above the Earth’s atmosphere by the Shuttle-borne ATMOS experi-ment.Visible continuum intensities and center-limb behavior constrained the temperature profile of the deep photosphere,while CO center-limb behavior de-fined the thermal structure at higher altitudes.The oxygen abundance was self consistently determined from weak CO absorptions (for C/O ≡0.5).Our anal-ysis was meant to complement recent studies based on 3-D convection models which,among other things,have revised the historical solar oxygen (and car-bon)abundance downward by a factor of nearly two;although in fact our con-clusions do not support such a revision.Based on various considerations,an ǫO =700±100ppm (parts per million relative to hydrogen)is recommended;the large uncertainty reflects the model sensitivity of CO.New solar isotopic ratios also are reported:12C/13C=80±1,16O/17O=1700±220,and 16O/18O=440±6;all significantly lower than terrestrial.CO synthesis experiments utilizing a stripped down version of the 3-D model—which has large temperature fluctuations in the middle photosphere,possibly inconsistent with CO “movies”from the InfraredImaging Spectrometer(IRIS),and a steeper mean temperature gradient thanmatches visible continuum center-limb measurements—point to a lower oxygenabundance(∼500ppm)and isotopic ratios closer to terrestrial.A low oxygenabundance from CO—and other molecules like OH—thus hinges on the realityof the theoretically predicted mid-photospheric convective properties.Subject headings:Sun:photosphere—Sun:infrared—Sun:abundances1.IntroductionFor nearly three decades,the“solar neutrino problem”haunted Solar Physics.The ap-parent dearth of the elusive subnuclear byproducts of proton–proton cycle fusion presented severe challenges for theoretical descriptions of interior conditions in the Sun;models that heretofore had been regarded as ironclad and unassailable(Bahcall,Basu,&Pinsonneault 2003,and references to previous work therein).The neutrino problem thankfully was re-solved in recent years,ironically from the particle-physics rather than astrophysics side(e.g., Bahcall,Krastev,&Smirnov1999),and solar(and stellar)interior models once again seemed secure.A new challenge,however,has confronted the even more refined contemporary genera-tion of solar interior models crafted for—and to a large extent by—helioseismology;what one might call the“solar oxygen crisis.”Sophisticated analyses,employing time dependent3-D simulations of convection driven solar surface velocityfields and thermal inhomogeneities, have pointed to a surprisingly low oxygen abundance,based on an impressive collection of tracers including forbidden and weak permitted O I lines in the visible spectrum,and hydroxyl(OH)rovibrational and pure rotational bands in the3–10µm thermal infrared (Asplund et al.2004,and references to previous work therein).The new low O abundance(ǫO=460±50parts per million[ppm]relative to hydrogen versus the most recent previously recommended value650±100ppm[Grevesse&Sauval 1998,hereafter GS98]:see Figure1)plays an important role in interior models of the Sun because oxygen ranks third after hydrogen and helium by number in a solar chemical mixture, and contributes very substantially to the interior radiative opacity.Furthermore,the next most abundant species—C,N,and Ne—are very difficult to measure(as is O itself)in thevisible spectrum owing to lack of suitable absorption lines,and changes in their abundances often follow closely any alterations toǫO.In other words,abundance ratios such as C/O (∼0.5[Allende Prieto,Lambert,&Asplund2002])or Ne/O(derived from solar energetic particles[e.g.,Meyer1985]or high resolution spectra of Ne and O ions in the solar transition zone[Warren2005])often are regarded as better determined than the absolute abundances themselves.Thus,a revision inǫO could have a domino-like effect on the overall solar chemical composition and heavy element mass fraction Z,one of the crucial parameters governing the interior structure of a star and its evolution.The revised low oxygen abundance is said to resolve outstanding issues in interstellar medium and young stellar population studies(Asplund et al.2004),which previously had pegged the Sun as an“oxygen-rich”dwarf;at odds with its middle-aged status in a galaxy undergoing steady metal enrichment owing to successive generations of stellar birth and death(Timmes,Woosley,&Weaver1995).At the same time,the solar oxygen crisis has provoked the helioseismology community by casting doubt on the previous spectacularly good agreement between simulated interior sound speed profiles,and the depth of the convection zone,with values deduced from detailed,high precision measurements of surface p−mode oscillations from the ground and space(Bahcall et al.2005).Ironically enough,the new oxygen crisis also is having an impact on understanding solar neutrinofluxes(Bahcall& Serenelli2005).There appears to be no easy way out of the dilemma from the helioseismology side: the upward revisions in interior opacities needed to accommodate the new lowǫO are larger than permitted by uncertainties in the best contemporary theoretical and laboratory atomic physics data(Antia&Basu2005).On the low O side,the latest generation of time depen-dent3-D solar convection models have become quite sophisticated,and are said to allow the precise matching of intensity profiles of weak velocity-sensitive photospheric lines without the ad hoc specification of extraneous dynamical parameters(such as the micro-or macro-turbulent velocityfields familiar from classical1-D abundance modeling[e.g.,Gray1976]). This is an extremely important advance in the ab initio modeling—and understanding—of the structure of the solar photosphere,and by extension of all late-type stars dominated by strong convective heatfluxes in their surface layers.Furthermore,the analyses by Asplund and collaborators of the fundamental atomic physics of the various oxygen abundance diagnostics,of blending issues,and of departures from Local Thermodynamic Equilibrium(LTE)in the permitted O I lines are persuasive. In fact,the three major facets of the low O problem—3-D model,atomic physics(including non-LTE effects),and blending—play roughly equal,positively reinforcing roles in explaining the nearly0.3dex(factor of∼2)decrease of the revised oxygen abundance relative to thevalue recommended little more than a decade ago(e.g.,850±70ppm[Grevesse&Anders 1991,hereafter GA91]).Thus the solar low O problem truly is vexing.The purpose of the present paper is to add,in our opinion,two key perspectives:(1) the importance of accurately“calibrating”the reference photospheric model,be it3-D or 1-D,against,say,absolute visible continuum intensities and center-limb behavior;and(2)a complementary oxygen abundance(and thermal profile)diagnostic overlooked in the recent work,namely the rovibrational bands of solar carbon monoxide with its fundamental(∆v= 1)near4.6µm andfirst overtone(∆v=2)near2.3µm.The former issue—properly scaling the reference model in temperature—was discussed specifically in a pioneering comprehensive study of the solar carbon,nitrogen,and oxygen abundances by Lambert(1978,hereafter L78),and more generally by Ayres(1977,hereafter A77;1978a).The solar COfirst overtone spectrum,and its significance for the carbon abundance(and thus forǫO if C/O is known) was described by Tsuji(1977)and Ayres(1978b),and an extension to the fundamental bands by Ayres&Testerman(1981,hereafter AT81).To preview our conclusions,the solar CO analysis does not support the recently proposed substantial lowering of the oxygen(and carbon)abundances,but instead favors a higher value for oxygen(and carbon),closer to the recent recommendations of GS98,and the earlier work of L78and GA91.In addition,we derive new accurate abundance ratios for13C, 17O,and18O.The stable isotopes of C and O are signatures of galactic chemical evolution, and should increase over time as the galaxy becomes progressively enriched in the nuclear detritus of successive generations of star formation.The oxygen isotopes,in particular,can trace fractionation processes in the solar nebula associated with the establishment(or not)of dust-gas equilibrium.It is commonly lamented in the solar system literature,however,that solar astronomy has failed to provide definitive ratios for either16O/17O or16O/18O(Wiens et al.2004).This was one of the major goals,in fact,of NASA’s ill-fated Genesis Discovery mission(Burnett et al.2003).Nevertheless,we demonstrate that it is straightforward to derive accurate oxygen(and carbon)isotopic abundance ratios from CO lines,and further that the per mil(parts per thousand)differences—e.g.,δ18O—are an order of magnitude,or more,larger than anticipated by theoretical models of the gas-dust chemistry in primitive solar system material.2.ObservationsThe CO rovibrational spectrum is a valuable tracer for the mid-photospheric thermal profile,and the oxygen abundance,for several reasons.First,the weak lines of the∆v=1,2 bands form deep in the warmer layers of the photosphere where the CO concentration isunsaturated,in the sense that n CO∼ǫCǫO.If one knows the C/O ratio to better accuracy thanǫC itself,then the CO column density becomes quadratically sensitive toǫO.Second,the CO-rich zone nevertheless is shallow enough to lie above the layers where the strongest convective overshooting occurs,in fact where the thermalfluctuation pattern is thought to experience a reversal(e.g.,Uitenbroek2000a),and measured temperature inhomogeneities are mild(∆T rms∼40K:e.g.,Ayres&Brault1990,hereafter AB90).Third,the∆v=1,2bands contain literally thousands of individual transitions covering a wide range of gf-values and lower level excitation energies,compared with the mere handful of useful O I lines in the visible(L78).Also,potential blends with atomic absorption features are rare in the infrared,particularly in the4.6µm interval,contrasted to the heavy atomic line blanketing in the visible.(A key issue in the current low O debate is the strength of a weak Ni I blend in the important[O I]0.630µm feature.)Fourth,the CO∆v=1bands closely trace the photospheric thermal profile:the strongest lines respond preferentially to the conditions in the higher layers where they be-come optically thick,whereas the weaker lines reflect conditions at lower altitudes,closer to the infrared continuum formation horizon.Observations of the center-limb behavior of representative CO lines can serve the same“thermal profiling”purpose,in fact redundantly.A subsidiary issue involves the thermal structure of the very high layers,above the nominal base of the warm chromosphere,where the anomalous limb darkening of the strongest CO fundamental lines(Noyes&Hall1972a,AT81)and their off-limb emissions(Solanki,Liv-ingston,&Ayres1994)reveal cold gas at altitudes where—in traditional1-D models like the FAL C of Fontenla,Avrett,&Loeser(1993,hereafter FAL93)—temperatures should be too hot(T∼7000K)for molecules to survive.The origin and spatial scope of this counterin-tuitive“COmosphere”is controversial(Ayres2002,and references to previous work therein; hereafter A02),but—as we describe below(§3.3.4)—any high altitude cool material has only a minor influence on deriving an oxygen abundance from the weak∆v=1,2lines.Fifth,the CO rovibrational bands form very close to Local Thermodynamic Equilib-rium(LTE),owing to the large atomic hydrogen inelastic collision rates that quench the pure rotation and rotation-vibration transitions compared with their slow radiative decays (see Ayres&Wiedemann1989,and references to previous work therein,hereafter AW89). LTE formation applies,as well,to the background continuum in the infrared longward of 1.64µm,where the opacity sources thankfully are simple and well understood:mainly free-free(f–f)transitions of the negative hydrogen ion,H−,with a very small contribution from hydrogen bound-free(b–f)and f–f(Vernazza,Avrett,&Loeser1976).The CO gas-phase chemistry also is very close to“instantaneous chemical equilibrium”(ICE)in the middle photosphere,even in the face of time dependent molecular formation and destruction,andadvection processes,as demonstrated in recent detailed2-D hydrodynamical simulations by Wedemeyer-B¨o hm et al.(2005),and earlier in1-D time dependent models by Asensio Ramos et al.(2003).Sixth,the CO molecular parameters including the all important dissociation energy (D0=11.108eV:Morton&Noreau1994,hereafter MN94),line positions and transition strengths(Goorvitch1994,hereafter G94)are thought to be accurately known.Owing to the importance of CO as a terrestrial pollutant,these values have been vetted thoroughly, at least for the lowest vJ transitions that are prevalent in a cold planetary atmosphere like Earth’s.Thefinal practical advantage of the CO IR bands is the availability of very high quality measurements from the ground and space.The large Fourier transform spectrometer(FTS) on the National Solar Observatory’s McMath-Pierce telescope at Kitt Peak(Brault1978), for example,can record the fundamental region at resolutions of R≡ω/∆ω∼2×105,or more,(ωis the frequency measured in wavenumbers[cm−1]),fully resolving the narrow solar CO lines,and with signal-to-noise(S/N)in excess of several thousand.The McMath-Pierce also is home to the Infrared Imaging Spectrometer(IRIS),a grating-based instrument with lower resolving power(∼6×104),but a long slit stigmatic imaging capability,and ten times higher time resolution than the FTS can muster(Uitenbroek,Noyes,&Rabin1994, hereafter UNR94;Ayres&Rabin1996,hereafter AR96).Furthermore,the Shuttle-borne ATMOS experiment(also an FTS)obtained excellent solar disk center reference spectra in the thermal infrared during its severalflights(Farmer1994),achieving2×105resolution and S/N>1000in the best quality scans provided to the community.Despite the many positive attributes of CO as an abundance diagnostic,a specific downside—shared by molecules in general—is the strong sensitivity of the dissociative equi-librium to temperature.For this reason it is imperative to accurately characterize the thermal conditions in the mid-photosphere where the abundance sensitive weak CO lines arise.The following sections summarize the several types of observational material used in the subsequent thermal profiling—and oxygen abundance—analysis;including besides2–6µm CO,calibrated intensity highpoints in the0.4–0.7µm visible continuum region,and continuum center-limb behavior at visible and infrared wavelengths.2.1.ATMOS/ATLAS-3The Shuttle-borne ATMOS instrument was designed to study trace molecular species in the Earth’s atmosphere backlighted by the rising or setting Sun as viewed from low-Earthorbit.The baseline solar reference spectra,obtained from zenith pointings,were mainly free of terrestrial contamination,and consequently are extremely valuable for a study like the present one.The best results were obtained from thefinal ATMOSflight in1994November as part of the ATLAS-3payload(Abrams et al.1999).For the particular scan ranges used here,isolated by one of three passbandfilters,the instrument accepted a1mrad-diameter (200′′)circular region at disk center,which corresponds toµ=1for all intents and purposes (µ≡cosθ,whereθis the heliocentric angle:0◦at disk center,90◦at the extreme limb).Scans were downloaded from the ATMOS public archive and calibrated according to the specifications in the associated documentation.For the COfirst overtone interval,3200–4800cm−1,only scan#4had suitable coverage.For the CO fundamental region,1600–2400 cm−1,scans#3and#9provided overlapping coverage.Each was independently normal-ized to a continuum level by sequentiallyfitting low order polynomials to intensity high-points in partially overlapping5cm−1intervals:the ATMOS scans are dominated by the ubiquitous—but mainly isolated—absorption features of the CO bands,and generally there are smooth intervals between the lines where the pristine continuum—free of solar or tel-luric absorptions—shines through.The two separate continuum-normalized scans then were cross-correlated to determine any global frequency offsets.The derived∼0.5pixel shift was applied to scan#9;then the two datasets were combined by interleaving the intensity points,rather than interpolating and coadding which would have involved a smoothing of at least one of the scans,perhaps compromising the intrinsic high resolution of the ATMOS spectra.Thus,the tracings presented here for the CO fundamental region consist of two en-tirely independent datasets:any deviations represent mainly differences in the assigned local continuum levels or scan-specific distortions,and therefore are a fair measure of systematic intensity uncertainties beyond the purely photometric noise component.Figure2illustrates selected intervals of the fundamental andfirst overtone CO bands from the ATMOS spectra.Figure3illustrates selected transitions from the1–0R-branch (∆v≡v upper−v lower=+1,∆J≡J upper−J lower=+1;P-branch transitions have∆J=−1)of the CO fundamental from ATMOS,together with line profiles calculated with an“optimum”1-D thermal profile described later(§3.3).2.2.McMath-Pierce FTSThe large(1m path difference)FTS on the McMath-Pierce telescope achieves superb spectral resolution and excellent signal-to-noise in the thermal infrared,with broad fre-quency coverage and negligible scattered light.The McMath-Pierce telescope itself,with its 1.58m primary mirror and all reflecting unobscured design,not only can observe beyondthe∼2.5µm cutoffof typical window materials used in evacuated solar telescopes,but also delivers sub-arcsecond diffraction limited performance(∼0.′′8at5µm)during periods of good seeing.These capabilities are crucial for center-limb work in the CO bands,where core depths of a wide range of narrow∆v=1lines must be measured accurately at eachµvalue,and one wants to record as close as a few arcseconds from the limb in order to obtain the best slant-angle advantage for observing the highest atmospheric layers.Errors of only a few percent in line depths,due to under resolving line profiles or the presence of scattered light, can translate to an overestimate of hundreds of degrees in core brightness temperatures(an indirect diagnostic of kinetic temperatures nearτ∼1:AT81).The main disadvantages of the FTS are that it can record only a single spatial point at a time(defined by an entrance aperture which can have an arbitrary shape and size within certain limits),and it requires several minutes to accumulate a full interferogram at highest resolution and S/N.By its nature,the FTS also benefits from a highly stable source region;a particular concern,for example,in observations very close to the edge of the disk,where seeingfluctuations or wind shake could transiently alter the intensityfield.For that reason, the highest quality previous FTS work on the anomalous limb darkening of the CO bands had been done atµ 0.2(AT81,AB90),corresponding to8.1mm or19′′inside the IR limb. (The plate scale of the McMath-Pierce is2.37′′mm−1.)2.2.1.Fast Tip/Tilt Image StabilizerRecently the FTS was outfitted with a novel fast tip/tilt image stabilization system, developed by C.Keller and C.Plymate of NSO;a major improvement for limb observations. The system mounts on the front plate of the FTS,and delivers a stabilized image to the entrance port of the interferometer.The transfer optics of the stabilizer pick offa portion of the solar image,collimate it onto a piezioelectrically actuated tip/tilt mirror,and refocus the corrected image onto a beamsplitter.The98%reflected light is directed to the FTS entrance aperture,while the2%transmitted image is sensed by a CCD camera,which can operate in a variety of tracking modes—limb,quad cell,or granular correlation—to complete the feedback loop.The CCD accepts a∼100˚A passband centered in the red at∼7000˚A. The system operates at500Hz,fast enough to compensate for seeingfluctuations and image shake.2.2.2.2002April Observing RunThe observations described here were acquired during a5-day run in2002April(13–17),mainly on the14th.A98cm full path difference of the FTS was utilized,and the interferograms were accumulated in double-sided mode.The theoretical resolving power was R∼2×105in the2145cm−1(4.66µm)atmospheric“window”where most CO∆v=1mea-surements are made.Each interferogram required nearly four minutes to record,including fly-back.Twin L–N2-cooled InSb detectors(“A”and“B”)were sampled at2500Hz.(The two outputs view opposite sides of the FTS recombining optic and thus are out of phase with one another.The interferogram signal is the difference of the two,which has the advantage of canceling noise[such as60Hz]common to both detectors.)A broad-bandfilter limited the spectral coverage to1800–2900cm−1(3.4–5.6µm),to suppress out-of-band photometricnoise.On the14th,we took advantage of superb image quality and lack of(wind-induced) image shake to work close to the limb,exploiting the new image stabilizer.We chose a 10mm×0.25mm rectangular entrance aperture(24′′×0.′′6;the radius of the solar image was 957′′).The width of the slit was comparable to the diffraction limit of the telescope,andsmall enough to impose a negligibleδµ/µon observations very close to the limb.The slit was as long as could be accommodated in good focus with the FTS,but short enough that δµ/µdue to curvature of the limb was negligible.We zeroed the coordinate frame of the image stabilizer,in limb tracking mode,at the half power point of the A+B central fringe signal(representing source intensity)as it rolled offacross the edge of the disk.Owing to the passband limitingfilter,we were measuring the true infrared limb,which can be displaced a few arcseconds from the visible limb by atmospheric refraction.We then backed the limb away from the aperture a set number of steps on the image stabilization system(the plate scale at the CCD was0.′′14pix−1),to position a specificµ-value over the entrance slot.We frequently checked the null position at the IR limb to ensure that no drifts had occurred.During that morning,we obtained 21separate useful observations at a range ofµ-values:fromµ=0.169(5.83mm inside the limb)down to as close asµ=0.076(1.17mm inside the limb).We concluded with a pair of measurements at disk center(µ=1).Since the image stabilizer had to be mounted in afixed orientation on the vertical circular“table”of the FTS,we were not able to actively compensate for the diurnal rotation of the solar image.Accordingly,our observations effectively covered an arc at the limb as the solar disk rotated over thefixed slit position;providing,in fact,a broad,unbiased spatial average at the limb.A full account of the FTS program will be provided in a future paper,including details of the data reduction,scan averaging,and compensation for atmospheric absorption.Figure4 compares a disk center tracing from the McMath-Pierce FTS with the ATMOS/ATLAS-3 spectrum described previously.Center-limb lineshapes of selected2145cm−1CO transitions will be presented later(§3.3.3).2.3.McMath-Pierce IRISThe Infrared Imaging Spectrograph(IRIS),commissioned in1993,utilizes the Main (“vertical”)spectrograph of the McMath-Pierce,a256×256Amber Engineering InSb camera cooled by solid nitrogen,and the control system of the Near Infrared Magnetograph(NIM: Rabin1994).We made use of two general types of IRIS observations in the present study:(1)short exposure single slit measurements of the CO translimb(emission)spectrum;and(2)rapid cadence sequences of raster scans to record2-D time dependent behavior of the CO bands at disk center.2.3.1.Translimb CO MeasurementsTranslimb CO measurements are a diagnostic for the coolest parts of the high altitude “COmosphere,”especially the height of the temperature minimum(“T min”).The strong saturated low excitation CO features,in particular,can have significant contributions from this cold(T<4000K)region,thereby affecting the determination of the temperature stratification and potentially also the oxygen abundance.The present study utilized sequences of translimb image bursts obtained1996May9, representative of the quiet Sun near sunspot minimum,as described fully by A02.Although not stated explicitly in that study in these terms,the single component thermal profile that best matches the off-limb CO emissions has a T min at a column mass density m∼2.5×10−3gr cm−2,compared with the m∼5×10−2of the FAL C reference model(FAL93). Unfortunately,the off-limb CO emissions—as recorded by the relatively low dispersion IRIS instrument—are not useful for quantifying T min itself.However,the new FTS extreme limb scans can be used for that purpose,as described later(§3.3.4).(To preview our results,we obtain T min∼3500K,as compared with the4400K of the FAL C model.)2.3.2.Disk-Center CO TimeseriesThe second set of IRIS data comprised high spatial resolution,rapid cadence surfacetemperature maps recorded during a coordinated multiwavelength campaign in1999May.The program,which included simultaneous UV imaging spectroscopy from SoHO SUMERand narrow band UV imaging from TRACE,has been described previously in a qualitativeway by Ayres(2000).Here,we provide additional details concerning the IRIS part of theprogram.The objective on the IRIS side was to measure,with high time resolution,temperatureand velocityfluctuations at several levels of the outer photosphere,ultimately to comparewith the response of the overlying chromosphere imaged by SUMER and TRACE.In thepresent work,however,the amplitude of thermalfluctuations in the mid-and high photo-sphere play a direct and crucial role in backing out realistic abundances from temporallyand spatially averaged solar CO measurements.The IRIS spectra described here were obtained on the morning of1999May14.Thevisible seeing was good at the start of observations(08:30MST),but deteriorated later inthe morning.The infrared seeing usually is much better than in the visible,and the effectiveresolution often is limited by telescope diffraction rather than the atmosphere.We operated the vertical spectrograph in single pass,recording a free spectral range of2.3cm−1centered at2143cm−1;with a“minifying”lens in the transfer optics to achieve a spatial coverage of97′′along the slit.The slit was oriented N/S heliocentric,with a width of160µm(0.′′4).In mapping mode,the slit was stepped sequentially in the E/W direction, 8steps per raster with a step spacing of1.′′9;thus covering a swath13′′×97′′at Sun center. The exposure time at each slit position was300ms,and with stepping and data writingoverheads,a full area scan required45s to complete(well sampling the photospheric p-modeoscillations timescale[5minutes]and that associated with higher frequency chromosphericmodes[3minutes]).We accumulated40rasters per observation:320spectral frames in30minutes.In each individual spectrogram,the main parameters of interest were central depthsand frequency shifts of the CO lines,and the relative variation of continuum intensity,asrecorded along the1-D slit direction.(Reduction and measurement techniques are describedby AR96).The depth of a strong saturated CO line is tied closely to the kinetic temperatureof the atmospheric layer in which the line core becomes optically thick.Similarly,the Dopplershift records the line-of-sight component of the velocityfield where the feature forms.Finally,the relative continuum intensity responds to temperature contrasts in the layers where the IRbackground continuum becomes optically thick.In general,the line FWHM also would be a。
- 1、下载文档前请自行甄别文档内容的完整性,平台不提供额外的编辑、内容补充、找答案等附加服务。
- 2、"仅部分预览"的文档,不可在线预览部分如存在完整性等问题,可反馈申请退款(可完整预览的文档不适用该条件!)。
- 3、如文档侵犯您的权益,请联系客服反馈,我们会尽快为您处理(人工客服工作时间:9:00-18:30)。
a r X i v :h e p -l a t /0304010v 1 16 A p r 20031Thermal Width Broadening of the 0++Glueball Spectrum near T cNoriyoshi Ishii 1,Hideo Suganuma 2,and Hideo Matsufuru 31RadiationLaboratory,RIKEN (The Institute of Physical and Chemical Research),2–1Hirosawa,Wako,Saitama 351–0198,Japan 2Faculty of Science,Tokyo Institute of Technology,2–12–1Ohkayama,Meguro,Tokyo 152–8552,Japan 3Yukawa Institute for Theoretical Physics,Kyoto University,Kitashirakawa-Oiwake,Sakyo,Kyoto 606–8502,Japan We study the 0++glueball correlator constructed with SU(3)anisotropic quenched lattice QCD at various temperature taking into account the possible existence of the thermal width in the ground-state peak.For this purpose,we adopt the Breit-Wigner ansatz,analysing the lattice data obtained with 5,500–9,900gauge configurations at each T .The results indicate the significant thermal width broadening as Γ(T c )∼300MeV with a reduction in the peak center as ∆ω0(T c )∼100MeV in the vicinity of the critical temperature T c .§1.Introduction At finite temperature/density,QCD changes its vacuum structure such as the reduction of the string tension,the partial chiral restoration,etc,even below the critical temperature T c of the QCD phase transition.These changes are expected to affect the hadrons leading to the changes in the various hadronic properties,since hadrons are composite particles consisting of quarks and gluons.The hadronic pole-mass shifts are thus considered to serve as the important precritical phenomena of the QCD phase transition near the critical temperature T c ,and have been exten-sively studied by using various QCD-motivated low-energy effective theories.1),2),3),4)These studies suggest the pole-mass reductions of charmoniums,light q ¯q mesons and the glueball near the critical temperature.As for the lattice QCD,it has been more popular to study the spatial correla-tions (the screening mass)than the temporal correlations (the pole-mass)at finite temperature.This is because the temporal extension of the lattice is subject to 1/T .Accordingly,number of the temporal lattice data decreases at finite temperature,which used to be the main obstacle for the lattice QCD to measure the hadronic pole-mass.Recently,by using the anisotropic lattice,which has a finer lattice spac-ing in the temporal direction than in the spatial direction,the accurate measurement of the pole-mass with the lattice QCD has become possible at finite temperature.5),6)Quenched-level Monte Carlo calculations reveal the profound results that the pole-masses of the q ¯q mesons are almost unchanged from their zero-temperature values in the confinement phase,6),7)while the pole-mass of the 0++glueball shows the 300MeV reduction near the critical temperature.8)Although these thermal effects are weaker than anticipated by the effective model studies,these tendencies are consis-tent with the recent quenched-level lattice studies on the screening mass at finitetypeset using P T P T E X.cls Ver.0.892N.Ishii,H.Suganuma and H.Matsufuru temperature.9),10)In theseanalysis,thebound-state peaks in the spectral function are assumed to be narrow enough.However,at finite temperature,each bound-state acquires the thermal width through the interaction with the heat bath.The ther-mal width is expected to grow up with temperature,which may lead to a possible collapse of the narrow-peak assumption in some cases.In this paper,we first dis-cuss what is the expected consequence of the thermal width broadening.Then,we propose the Breit-Wigner ansatz for the fit-function for the temporal correlator at finite temperature.We finally show the results of the Breit-Wigner analysis of the 0++glueball correlator at finite temperature.11)§2.The Breit-Wigner AnsatzWe begin by considering the temporal correlator G (τ)≡Z (β)−1Tr e −βH φ(τ)φ(0)and its spectral representation asG (τ)= ∞−∞dω2sinh(βω/2)ρ(ω),(2.1)where H denotes the QCD Hamiltonian,Z (β)≡Tr(e −βH )the partition func-tion,φ(τ)is the zero-momentum projected glueball operator 8)represented in the imaginary-time Heisenberg picture as φ(τ)≡e τH φ(0)e −τH .Here,ρ(ω)denotes the spectral functionρ(ω)≡ m,n| n |φ|m |22sinh(βω/2).(2.4)Thermal Width Broadening of the0++Glueball Spectrum near T c3 Here,2sinh(βω/2)in the denominator works as a biased factor,which enhances the smallerωregion while suppressing the largerωregion.Consequently,the pole-mass m(T),which is approximated with the peak position of W(ω),is smaller than the peak centerω0of the spectral functionρ(ω),i.e.,the observed hadron“mass”.11) What is the appropriate functional form of thefit-function?Tofind this,we consider the retarded Green function G R(ω).At T=0,bound-state poles of G R(ω)are located on the realω-axis.At T>0,bound-state poles are moving into the complex ω-plane with increasing temperature.Suppose that a bound-state pole is located at ω=ω0−iΓasG R(ω)=AπIm 1πǫ2πcosh(ω(β/2−τ))N c 1N cγG i,j≤3ReTr(1−P i4(s)),(3.1)where Pµν(s)∈SU(3)denotes the plaquette operator in theµ-ν-plane.The lattice parameter and the bare anisotropic parameter arefixed asβlat≡2N c/g2=6.25and4N.Ishii,H.Suganuma and H.MatsufuruγG=3.2552,respectively,so as to reproduce renormalized anisotropyξ≡a s/a t= 4.5),6)These parameter set reproduces a−1s=2.341(16)GeV and a−1t=9.365(66) GeV,where the scale unit is introduced from the on-axis data of the static inter-√quark potential with the string tensionThermal Width Broadening of the0++Glueball Spectrum near T c5 ate one.Above T c,however,the particle spectrum is expected to change drastically, and a proper assumption on the spectral function becomes less trivial.In this re-spect,it is interesting to apply the recently developed maximum entropy method (MEM),13),14)which requires no assumption on the shape of the spectral function.Comparing our results8),11)with the related lattice QCD results,6),7)the quenched lattice QCD indicates that no significant thermal effects on the pole-mass have been so far observed in the q¯q sector,6),7)whereas a significant thermal effects can be ob-served in the glueball sector.These behaviors are consistent with the thermal effects on the screening mass.9),10)To discuss the experimental observability,it is important to estimate the effects of the dynamical quarks.One of the most significant contributions of the light dynamical quarks in unquenched QCD is the“string breaking”phenomenon,i.e.,the light dynamical quark screens the coloredflux and the longflux tubes are expected to be broken into pieces.The string breaking thus affects the long distance behavior of the inter-particle interactions,which could lead to a possible changes in the structures of hadrons in quenched QCD.In the case of the0++glueball,since its size is known to be rather compact as R≃0.4fm,8),11)such changes of the glueball structure is expected to be less significant.As for the mixing effect with the0++q¯q mesons in unquenched QCD,a recent unquenched lattice QCD study shows that there is no quark mass dependence on the glueball mass,which suggests that the mixing is actually rather small.However,it is in principle desirable to estimate the size of this mixing explicitly,which is left for the future studies.AcknowledgmentsThe lattice QCD Monte Carlo calculations have been performed partly on NEC-SX5at Osaka University and partly on HITACHI SR8000at KEK.References1)T.Hatsuda,and T.Kunihiro,Phys.Rev.Lett.55(1985),158.2)T.Hashimoto,K.Hirose,T.Kinki,and O.Miyamura,Phys.Rev.Lett.57(1986),2123.3)T.Hatsuda,Y.Koike,and S.-H.Lee,Phys.Rev.D47(1993),1225.4)H.Ichie,H.Suganuma,and H.Toki,Phys.Rev.D52(1995),2944.5)T.R.Klassen,Nucl.Phys.B533(1998),557.6)QCD-Taro Collaboration,P.de Forcrand,Phys.Rev.D63(2001),054501.7)T.Umeda,R.Katayama,O.Miyamura,and H.Matsufuru,Int.J.Mod.Phys.A16(2001),2215.8)N.Ishii,H.Suganuma,and H.Matsufuru,Phys.Rev.D66(2002),014507.9) ermann,and P.Schmidt,Eur.Phys.J.C20(2001),541.10)S.Datta,and S.Gupta,hep-lat/0208001.11)N.Ishii,H.Suganuma,and H.Matsufuru,Phys.Rev.D66(2002),094506.12) C.J.Morningstar,and M.Peardon,Phys.Rev.D66(1999),034509.and referencestherein.13)M.Asakawa,T.Hatsuda,and Y.Nakahara,Prog.Part.Nucl.Phys.46(2001),459.14)M.Asakawa,T.Hatsuda,and Y.Nakahara,hep-lat/0208059.15) A.Hart,and M.Teper,Phys.Rev.D65(2002),034502.。