Ergosterol and microbial biomass in the rhizosphere of grassland
一种用于治疗脑卒中的螺环化合物

一种用于治疗脑卒中的螺环化合物摘要:目的:研究一种用于治疗脑卒中的螺环化合物。
方法:观察该螺环化合物对存活鼠24小时后行为变化,脑梗塞体积及脑组织含水量的影响。
结果:该发明化合物可以显著改善脑缺血再灌注小鼠因缺血再灌注引起的神经损伤,能够明显降低其脑梗塞百分比和减轻其脑组织的水肿程度,降低其含水量。
结论:该发明化合物对于脑卒中特别是缺血性脑卒中具有优异的治疗作用。
关键词:脑卒中;螺环化合物;治疗脑血管病是导致人类死亡的三大疾病之一,其高死亡率、高致残率给社会和家庭带来了沉重的负担。
脑卒中是其中最严重的病症之一,其主要分为出血性脑卒中和缺血性脑卒中两类。
缺血性脑卒中占全部脑卒中发病率的80%左右,并且其发病率随年龄的增长而增高。
缺血性脑卒中的发生主要是由于脑栓塞及局部血栓的形成,这可能是由动脉粥样硬化引起的血管狭窄、血栓栓塞,或者是来源于心脏的栓子随血流至脑部引起脑栓塞;而各种原因造成的血管损伤、血管炎症也是重要原因之一。
缺血性脑卒中对患者健康危害极大,往往造成不可逆的脑部损伤。
因大脑动脉血流量和氧供应的中断,显著损害脑部生理活性,从而导致了一系列复杂的病理生理过程,包括脑组织能量代谢紊乱、兴奋性氨基酸毒性、自由基损伤、炎症反应、细胞凋亡等。
脑缺血一定时间恢复血液供应后,其功能不但未能恢复,反而出现了更加严重的脑机能障碍,即脑缺血再灌注损伤。
缺血再灌注损伤病生理过程是一个多环节、多因素、多途径损伤的酶促级联反应,涉及脑细胞能量代谢紊乱、兴奋性氨基酸毒性、细胞内钙超载、氧化应激损伤及神经细胞凋亡等。
目前,对缺血性脑卒中治疗的策略主要包括两方面,一是恢复脑再灌,改善脑血供(即溶栓);二是阻断损伤级联反应,防止神经元损伤(即脑保护)。
但是,目前还没有一种药物能够十分有效地治疗缺血性脑卒中。
因此,开发更多的可治疗脑卒中特别是缺血性脑卒中的药物显得尤为迫切且具有重大意义。
1材料与方法1.1材料1.1.1 一种式(I)化合物一种式(I)化合物、其药学上可接受的盐、水合物、溶剂合物、光学异构体或前药:其中:R1、R2、R3、R4各自独立地选自氢、C1-6烷基、C1-6烷氧基、卤代C1-6烷基、羟基、氰基、硝基、氨基、C1-6烷基氨基、二(C1-6烷基)氨基或C6-14芳基,其中所述C6-14芳基任选被1-3个选自C1-4烷基、C1-4烷氧基、卤代C1-4烷基的基团所取代;R5选自氢、羟基、C1-6烷氧基、卤代C1-6烷氧基或C1-4烷氧基C1-6烷氧基;R6选自氢、C1-6烷基、C3-12环烷基、COR7或COOR8,R7、R8各自独立地选自氢、C1-6烷基、C3-8环烷基、C6-10芳基或5-10元杂环烷基,其中,所述C1-6烷基、C3-8环烷基、C6-10芳基、5-10元杂环烷基任选被1-3个选自卤素、氰基、硝基、C1-4烷基、C1-4烷氧基、卤代C1-4烷基的基团所取代。
真菌和细菌生物量在土壤团聚体中的分布和耕作响应
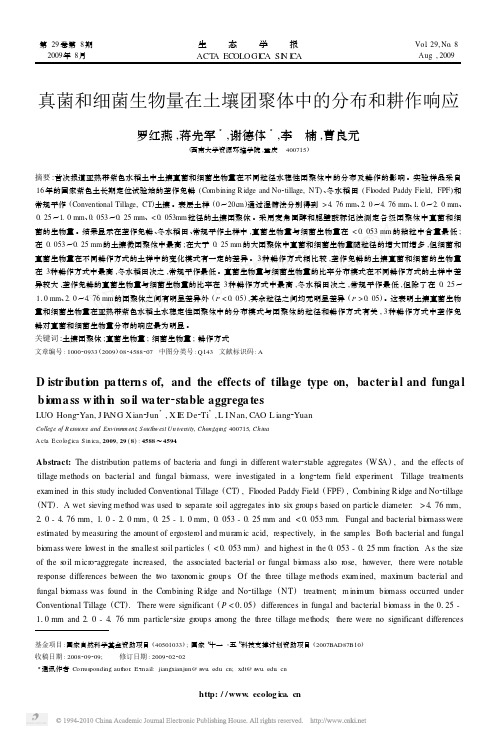
第29卷第8期2009年8月生态学报ACT A ECOLOGI CA SI N I CA Vol .29,No .8Aug .,2009基金项目:国家自然科学基金资助项目(40501033);国家“十一・五”科技支撑计划资助项目(2007BAD87B10)收稿日期:2008209209; 修订日期:20092022023通讯作者Corres ponding author .E 2mail:jiangxianjun@s wu .edu .cn;xdt@s wu .edu .cn真菌和细菌生物量在土壤团聚体中的分布和耕作响应罗红燕,蒋先军3,谢德体3,李 楠,曹良元(西南大学资源环境学院,重庆 400715)摘要:首次报道亚热带紫色水稻土中土壤真菌和细菌生物量在不同粒径水稳性团聚体中的分布及耕作的影响。
实验样品采自16年的国家紫色土长期定位试验站的垄作免耕(Combining R idge and No 2tillage,NT )、冬水稻田(Fl ooded Paddy Field,FPF )和常规平作(Conventi onal Tillage,CT )土壤。
表层土样(0~20c m )通过湿筛法分别得到>4.76mm 、2.0~4.76mm 、1.0~2.0mm 、0.25~1.0mm 、0.053~0.25mm 、<0.053mm 粒径的土壤团聚体。
采用麦角固醇和胞壁酸标记法测定各级团聚体中真菌和细菌的生物量。
结果显示在垄作免耕、冬水稻田、常规平作土样中,真菌生物量与细菌生物量在<0.053mm 的粘粒中含量最低;在0.053~0.25mm 的土壤微团聚体中最高;在大于0.25mm 的大团聚体中真菌和细菌生物量随粒径的增大而增多,但细菌和真菌生物量在不同耕作方式的土样中的变化模式有一定的差异。
3种耕作方式相比较,垄作免耕的土壤真菌和细菌的生物量在3种耕作方式中最高,冬水稻田次之,常规平作最低。
Soil chemical properties and microbial biomass
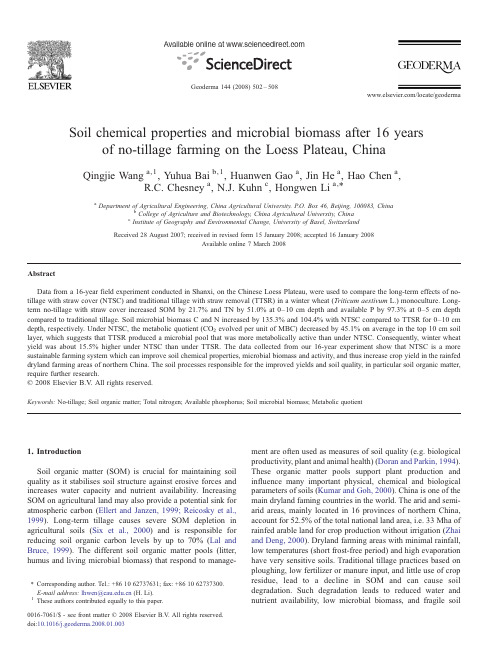
Soil chemical properties and microbial biomass after 16yearsof no-tillage farming on the Loess Plateau,ChinaQingjie Wang a,1,Yuhua Bai b,1,Huanwen Gao a ,Jin He a ,Hao Chen a ,R.C.Chesney a ,N.J.Kuhn c ,Hongwen Li a,⁎aDepartment of Agricultural Engineering,China Agricultural University.P .O.Box 46,Beijing,100083,ChinabCollege of Agriculture and Biotechnology,China Agricultural University,China cInstitute of Geography and Environmental Change,University of Basel,SwitzerlandReceived 28August 2007;received in revised form 15January 2008;accepted 16January 2008Available online 7March 2008AbstractData from a 16-year field experiment conducted in Shanxi,on the Chinese Loess Plateau,were used to compare the long-term effects of no-tillage with straw cover (NTSC)and traditional tillage with straw removal (TTSR)in a winter wheat (Triticum aestivum L.)monoculture.Long-term no-tillage with straw cover increased SOM by 21.7%and TN by 51.0%at 0–10cm depth and available P by 97.3%at 0–5cm depth compared to traditional tillage.Soil microbial biomass C and N increased by 135.3%and 104.4%with NTSC compared to TTSR for 0–10cm depth,respectively.Under NTSC,the metabolic quotient (CO 2evolved per unit of MBC)decreased by 45.1%on average in the top 10cm soil layer,which suggests that TTSR produced a microbial pool that was more metabolically active than under NTSC.Consequently,winter wheat yield was about 15.5%higher under NTSC than under TTSR.The data collected from our 16-year experiment show that NTSC is a more sustainable farming system which can improve soil chemical properties,microbial biomass and activity,and thus increase crop yield in the rainfed dryland farming areas of northern China.The soil processes responsible for the improved yields and soil quality,in particular soil organic matter,require further research.©2008Elsevier B.V .All rights reserved.Keywords:No-tillage;Soil organic matter;Total nitrogen;Available phosphorus;Soil microbial biomass;Metabolic quotient1.IntroductionSoil organic matter (SOM)is crucial for maintaining soil quality as it stabilises soil structure against erosive forces and increases water capacity and nutrient availability.Increasing SOM on agricultural land may also provide a potential sink for atmospheric carbon (Ellert and Janzen,1999;Reicosky et al.,1999).Long-term tillage causes severe SOM depletion in agricultural soils (Six et al.,2000)and is responsible for reducing soil organic carbon levels by up to 70%(Lal and Bruce,1999).The different soil organic matter pools (litter,humus and living microbial biomass)that respond to manage-ment are often used as measures of soil quality (e.g.biological productivity,plant and animal health)(Doran and Parkin,1994).These organic matter pools support plant production and influence many important physical,chemical and biological parameters of soils (Kumar and Goh,2000).China is one of the main dryland faming countries in the world.The arid and semi-arid areas,mainly located in 16provinces of northern China,account for 52.5%of the total national land area,i.e.33Mha of rainfed arable land for crop production without irrigation (Zhai and Deng,2000).Dryland farming areas with minimal rainfall,low temperatures (short frost-free period)and high evaporation have very sensitive soils.Traditional tillage practices based on ploughing,low fertilizer or manure input,and little use of crop residue,lead to a decline in SOM and can cause soil degradation.Such degradation leads to reduced water and nutrient availability,low microbial biomass,and fragile soilAvailable online at Geoderma 144(2008)502–508/locate/geoderma⁎Corresponding author.Tel.:+861062737631;fax:+861062737300.E-mail address:lhwen@ (H.Li).1These authors contributed equally to this paper.0016-7061/$-see front matter ©2008Elsevier B.V .All rights reserved.doi:10.1016/j.geoderma.2008.01.003physical structure.Consequently,yields become unstable and tend to decline and fertilizer,water,energy and labor are not used efficiently.Traditional tillage in northern China has already resulted in widespread soil degradation(Gao et al., 1999;Liu,2004).No-tillage practices featuring residue cover and less soil disturbance have been shown to reduce runoff by 52.5%and reduce erosion by80.2%compared to traditional tillage(Wang,2000).Liang et al.(2007)demonstrated that no-tillage significantly increased the concentration of soil organic C in5–20cm soil layer by5.6–5.9%on the clay loam soils after 3years in the humid northeastern China.In the more arid northern China,Li et al.(2006)conducted a4years no-tillage experiment and showed that active C and total organic C down to10cm depth were up to5%higher in no-tillage than traditional tillage systems.The application of no-tillage was also associated with increased yields and water use efficiency. Such improvement is largely due to improved soil quality. Experiments conducted by Liu(2004)in the village of Dingxing in the Hebei province showed that conservation tillage systems can increase organic matter,nitrogen,phosphorus,and potassium in the topsoil layer.Additionally,if crop residue is left in the field,this eliminates straw-burning and the labor requirements for removing straws from the fields.The studies mentioned above have shown that conservation tillage can improve soil physical properties,reduce wind and water erosion and increase soil fertility in northern China(e.g. Li et al.,2005;Luo et al.,2005;Zhou et al.,2007).However, comparatively little information is available on the changes in soil organic matter and nutrients,in particular microbial biomass for different tillage systems.A better understanding of the long-term effects of no-tillage and straw management practices on SOM,nutrients,microbial biomass and activity is also necessary for the further development of conservation tillage in dryland farming areas in China.Since1992,the Australian Centre for International Agricultural Research (ACIAR)and the Chinese Ministry of Agriculture have conducted experimental research on conservation tillage in the Loess Plateau of Shanxi province,northern China.The objective of this research is to identify the long-term effects of no-tillage with full straw cover to traditional tillage with full straw removal on soil chemical properties and microbial biomass on a rainfed dryland farming system on the Chinese Loess Plateau.2.Materials and methods2.1.Site descriptionThe study was conducted at the site of a long-term experiment(1992–2007)located in the village of Chenghuang near the city of Linfen(38°6N,113°E,456m a.s.l.)on the Loess Plateau in the south-central Shanxi province.Linfen lies in a semi-arid and warm temperate zone and has a continental climate.The mean annual temperature in the region is10.7°C and precipitation is about555mm(Fig.1),but highly variable between years.About65%of the annual precipitation occurs as rainfall during the summer season(June–September).The frost-free season lasts180days.A single crop system of winter wheat is common,with crops sown in September and harvested in June.In the experimental plots,the soil type is a Chromic Cambisol(sand23.1%,silt43.3%,clay33.6%,pH8.1) according to the FAO/UNESCO soil classification.In the top 30cm layer,soil bulk density was1.3Mg m−3,and total porosity was about40%.The field capacity and wilting point were21%and4%by weight,respectively.2.2.Experimental designAt the beginning of the experiment in1992,the entire field was ploughed to a depth of40cm to mix soil thoroughly and ensure uniform soil conditions in each experimental plot.The experiment was designed as a randomized block with three replications.Each plot was9m wide and78m long.The two tillage systems,traditional tillage with straw removal(TTSR) and no-tillage with straw cover(NTSC),were applied to the experimental plots from1992to2007.The TTSR system included spreading of fertilizer,ploughing to15cm depth and tillage for seedbed preparation,planting between September 20th and30th,herbicide(2,4-D butylate)and insecticide(40% dimethoate)spraying in April,and manual harvesting between June1st and10th.While the majority of straw was removed,a small amount of standing stubble of8to15cm in height(0.7t ha−1)remained after the winter wheat was harvested.A fallow period followed harvest until mid-September when the soil was ploughed to15cm depth again.The NTSC system was applied as follows:no-tillage planting and fertilizing between Septem-ber20th and30th,herbicide(2,4-D butylate)and insecticide (40%dimethoate)spraying in April,and harvesting between June1st and10th by mechanized harvester.Standing stubble of 15to25cm in height was retained with all wheat straw left as mulch cover(3.8t ha−1)Properties of the winter wheat residue were showed in Table1.A fallow period followed harvest until mid-September,with chemical weed control applied when necessary.During the experimental period of1992–2007,for each crop cycle,2,4-D butylate and40%dimethoate were applied at the rate of0.9and0.3kg(a.i.)ha−1using a knapsack sprayer with a flat fan nozzle.The winter wheat varietywas Fig.1.Distribution of mean monthly rainfall at the experimental site from June 2002to May2007.Error bars represent standard deviation.503Q.Wang et al./Geoderma144(2008)502–508Linfen225with a seeding rate of225kg/ha.The CO(NH2)2,(NH4)2HPO4and KCl(K2O content:60%)fertilizers were ap-plied to provide150kg N/ha,140kg P2O5/ha and62kg K2O/ha.Winter wheat yields were determined by manual harvesting,threshing,and air-drying grain from three1m2areas sampled atrandom in each plot.2.3.Soil sampling and analysisSoil cores(54mm diameter)were collected from the plots ofthe two tillage systems in May2007.In each plot,one soilsample formed by3subsamples was taken at0–5,5–10,10–20and20–30cm depths.In order to minimize compression and toobtain a representative sample of the soil,the cores wereremoved by inserting a trowel into the soil at the lower level ofeach sampling depth.The soil samples were stored in cleanplastic containers for chemical analysis.Each soil sample wasfirst passed through an8mm sieve by gently breaking the soilclods,pebbles and stable clods larger than8mm werediscarded.Before the analyses,soil samples were air-dried for24h in the laboratory.Soil organic matter(SOM)was determined by wet oxidation(Black,1965)and the percentage of organic carbon wascalculated by applying the Van Bemmelen factor of1.73(Piper,1950).Soil total nitrogen(STN)was determined using theKjeldahl digestion method.Plant available phosphorus(P)wasextracted with0.5M NaHCO3(Hedley and Stewar,1982).Allthe measurements were replicated three times.Microbial Biomass Carbon(MBC)was determined by afumigation–extraction method(Vance et al.,1987).Fumigatedand non-fumigated soils were extracted with0.5M K2SO4for30min(1:5soil:extractant ratio),filtered and the aliquot wasanalyzed for organic C by acid-dichromate oxidation.Theadditional oxidisable C obtained from the fumigated soilsrepresents the microbial C flush and was converted to MBCusing the relationship:microbial C=C flush/0.35.Microbialbiomass nitrogen(MBN)was measured following the methoddescribed by Ross(1992).The K2SO4extracts from fumigatedand non-fumigated soil samples were digested in aqueousK2S2O8(0.165M)for30min at121°C.The resultant NO3−–N and NH4+–N were measured by an auto analyser.MBN was estimated using the relationship:Microbial N=N flush/0.45(Jenkinson,1988).All the measurements were replicated threetimes.Respiration was measured as CO2evolution according toAlef(1995).Soil samples were placed in300ml glasscontainers closed with rubber stoppers,moistened at60%ofthe maximum water holding capacity and incubated for7daysat25°C.Glass vials holding10ml of0.5N NaOH to trap the evolved CO2were placed in the above containers.On day7 after the incubation,the glass vials were removed and the CO2 trapped in NaOH was determined by titration.The metabolic quotient(q CO2)was obtained by dividing the basal respiration by the microbial biomass C.The basal respiration measurement was replicated three times.2.4.Statistical analysisMean values were calculated for each of the variables,and ANOVA was used to assess the effects of no-tillage with straw cover and traditional tillage with straw removal on the measured soil proprieties.When ANOV A indicated a significant F-value, multiple comparisons of annual mean values were performed by the least significant difference method.In all analyses,a probability of error smaller5%(P b0.05)was considered statistically significant.The SPSS analytical software package (13.0)was used for all the statistical analyses.3.Results and discussion3.1.Soil organic matterThe mean SOM in0–30cm soil layer for TTSR was slightly higher(2.3%)than for NTSC.These differences were not significant at the beginning of the experiment in1992,but pronounced treatment effects on SOM could be observed after long-term different tillage management in2007(Table2).SOM to30cm depth for NTSC in2007was0.3g kg−1higher than that in1992,while TTSR decreased by0.2g kg−1in2007 compared to1992.Consequently,SOM to30cm depth in NTSC was1.5%higher than in TTSR after16years.In the surface soil layer(0–10cm),the average SOM was even more strongly increased for NTSC(33.6%in0to5cm and9.8%in5 to10cm)than TTSR.However,the SOM content declined in the10to20cm and20to30cm layer(2.9and2.7g kg−1)on NTSC.The significant improvement of SOM in the NTSC treatment was probably due to increased carbon input from residue Table2Treatment effects on soil organic matter(SOM),total nitrogen(TN)and available phosphorus(P)for0–5,5–10,10–20and20–30cm depthsSoildepth(cm)Treatment SOM(g kg−1)TN(g kg−1)Available P(mg kg−1)199220071992200719922007 0–5TTSR14.2a14.6a0.73a0.70a23.0a22.4a NTSC13.9a19.5b0.65a 1.29b23.2a44.3b 5–10TTSR14.5a15.3a0.65a0.66a25.2a31.9a NTSC14.7a16.8b0.64a0.77a24.8a25.6b 10–20TTSR14.7a13.8a0.74a0.66a21.8a22.9a NTSC13.9a11.1b0.70a0.67a20.8a10.5b 20–30TTSR10.2a9.2a0.57a0.35a15.2a7.6a NTSC9.7a 6.3b0.59a0.40a14.8a 6.4a Values within a column followed by the same letters are not significantly different(P b0.05).The data in1992were tested at the beginning of experiment (after the ploughing to40cm depth,but before the planting in September).Table1Some characteristics of the winter wheat residue retained in the experimentalplotsN(g/kg)C(g/kg)Ca(g/kg)Lignin(g/kg)Cellulose(g/kg)5.2272 2.1202340The residue samples were air-dried before testing.504Q.Wang et al./Geoderma144(2008)502–508retention(Brevik et al.,2002).For the TTSR,when soils are ploughed,some standing wheat stubble is moved into deeper soil layers(10–30cm),resulting in higher SOM content below 10cm depth than under NTSC.The higher organic matter in the topsoil layer achieved by NTSC shows that results from other arid regions can be applied in China.In Texas,Zibilske et al. (2002)recorded that no-tillage resulted in soil organic matter increase of up to58%in the top4cm of soil for no-till treatment.In the4–8cm layer,organic matter was15%greater than on the plow-till control.Roldan et al.(2005)recorded that no-tillage resulted in soil organic matter increase of up to33% in the0–5cm layer in Mexico.Similarly,Koch and Stockfisch (2006)also reported that conservation tillage concentrated soil organic matter and carbon in the top soil layer(0–10cm)in Germany.3.2.Soil total nitrogenSoil total Nitrogen showed the same trend than SOM in relation to tillage treatments(Table2).Compared to1992, overall STN(0–30cm)improved by21.3%on NTSC while it decreased by11.9%on TTSR.In the0–5cm layer,STN under NTSC was increased by81.25%compared to TTSR,while in the5–10cm layer STN was increased by only16.44% compared to TTSR.Below10cm,the STN differences were not significant.The results support earlier studies by Chowdhury et al.(2007)and Embacher et al.(2007),who demonstrated that total nitrogen decreased with increasing soil depth.The higher total N under no-tillage was also consistent with the findings of other researchers(e.g.Rasmussen and Collins,1991;Torbert and Reeves,1995;Thomas et al.,2007).However,in our study the increase(51.47%)of nitrogen in0–10cm depth appears to be higher than in other tests.For example,Thomas et al.(2007) recorded that the total N to10cm depth under no-tillage was only21%higher than for traditional tillage.3.3.Plant available phosphorusTable2shows that the available P in both treatments was very similar in1992,but significant differences developed across the soil profile during the16-year experiment.In2007, the available P under NTSC was97.5%higher than under TTSR in the0–5cm layer,significant at P b0.05.In the5–10 and10–20cm soil layers,the P content was19.75%and 54.06%,respectively,lower under NTSC than under TTSR, both significant at P b0.05.In the20–30cm layer the difference was not significant.Long-term no-tillage manage-ment commonly leads to a stratification of available P in soils (Zibilske et al.,2002).The topsoil accumulation of P in NTSC is attributed to the limited downward movement of particle-bound P in no-tillage soils and the upward movement of nutrients from deeper layers through nutrient uptake by roots (Urioste et al.,2006).A similar result was found in a five-year experiment conducted by Kang et al.(2001)on the Chinese Loess Plateau.However,in their test no-tillage increased soil available P to10cm depth only by5%compared to ploughing in winter wheat production in northern China.3.4.Microbial biomassThe effects of tillage practices on MBC and MBN con-centrations were significant,but less pronounced during the16-year experiment(Fig.2).In the0–5and5–10cm soil layers, MBC and MBN were significantly(P b0.05)higher under NTSC than under TTSR.This pattern was reversed below 10cm depth where differences between treatments were not significant(P b0.05).The results show that the trend of MBC and MBN with depth is similar,but that the tillage method had no significant effect on MBC and MBN below10cm.Higher levels of microbial biomass under NTSC can be explainedby Fig.2.Microbial biomass C(MBC),microbial biomass N(MBN)and MBC/ MBN ratio at0–5,5–10,10–20and20–30cm depth intervals for TTSR and NTSC in2007.Means within each depth followed by the same letter were not significantly different(P b0.05).505Q.Wang et al./Geoderma144(2008)502–508greater availability of substrate to sustain the microbial biomass when crop residues are retained.Furthermore,NTSC improves the soil MBC/MBN ratio in the topsoil,which is reflected by the increased microbial C and N pools compared to TTSR(Powlson et al.,1987).In TTSR on the other hand,the crop residues were removed and resulted in a lower MBC and MBN in0–10cm depth.Similar results were reported by Wright et al.(2005)who found that microbial biomass C was increased by80%and microbial biomass N by65%under no-tillage compared to conventional ploughing in the topsoil(0–10cm).They also found no significant treatment effects below10cm at the end of a10-year experiment.3.5.Soil microbial respiration and metabolic quotientThe value of basal respiration(BR)to a depth of30cm variedfrom3.4to9.7μg CO2–C g−1per day under TTSR and from3.0 to10.9μg CO2–C g−1per day under NTSC(Table3).Long-term tillage had a significant effect on BR,particularly in the0–5 and5–10cm soil depths,where NTSC significantly(P b0.05) improved BR by at least12%compared to TTSR.In the10–30cm depth,there was no significant difference in BR between treatments TTSR and NTSC.Our result was consistent with the findings of Franzluebbers and Arshad(1996)who also observed a tillage effect that varied from4to28μg CO2–C g−1per day under conventional tillage and from4to31μg CO2–C g−1per day under no-tillage in Northwestern Canada.The metabolic quotient(soil respiration per unit of microbial C(q CO2))ranged from66.3to85.1under TTSR and from25.7 to91.4mg CO2–C g−1MBC per day under NTSC(Table3). Averaged across the depths,q CO2in soil under NTSC was 21.8%lower than under TTSR.In0–5and5–10cm depths, q CO2values for NTSC were61.2%and31.6%significantly(P b 0.05)lower than for TTSR,while below10cm depth values for both treatments were similar,indicating that tillage effects on q CO2were pronounced in the topsoil.The inverse relationship between MBC and q CO2was similar to a four-year experiment conducted by Wang et al.(2007),who observed an increase in MBC of93.1%and decrease of18.8%in the q CO2in no-tillage soils as compared with conventional ploughing soils in northern China.These differences may be due to differences of acces-sibility to C substrates by microorganisms,changes in the metabolic rates and changes in microbial community composi-tion(Alvarez et al.,1995).NTSC soils also may have a greater amount of larger aggregates,which protect microorganisms from adverse conditions.This has potentially important implications for the impact of Chinese agriculture on climate, because less C is emitted from the NTSC soils and more can be stored in soil organic matter.3.6.Soil bulk densityThe mean soil bulk density to30cm depth for TTSR and NTSC was about1.30Mg m−3before planting in1992.After 16years of no-tillage,bulk density to30cm depth was just 2.2%lower on NTSC than TTSR(Fig.3).The differences were stratified again,with the most pronounced decline on NTSC between in20and30cm depth(6.0%).The lower bulk density in NTSC can be attributed to the increased soil organic C and biotic activity after long-term no-tillage practice(Karlen et al., 1994).3.7.Winter wheat yieldMean winter wheat yields,as indicated in Fig.4,were15.5% higher for NTSC than for TTSR during2003to2007,and a further significant(P b0.05)improvement of42.3%was achievedTable3Treatment effects on soil basal respiration(BR)and respiratory quotient(q CO2) for0–5,5–10,10–20and20–30cm depthsSoil depth Treatment Basal respiration q CO2(μg CO2–C g−1d−1)(mg CO2–C g−1d−1) 0–5cm TTSR9.7a66.3aNTSC10.9b25.7b5–10cm TTSR8.9a78.8aNTSC10.0b53.9b10–20cm TTSR 4.0a76.8aNTSC 3.6a69.1a20–30cm TTSR 3.4a85.1aNTSC 3.0a91.4aValues within a column followed by the same letters are not significantly different(P b0.05).Fig.3.Mean bulk density for2treatments at0–5,5–10,10–20and20–30cm depths.Samples were taken before harvesting in May,2007.Means within each depth followed by the same letter were not significantly different(P b0.05). Fig.4.Mean winter wheat yield for NTSC and TTSR from2003to2007.Means followed by the same letter were not significantly different(P b0.05).506Q.Wang et al./Geoderma144(2008)502–508in2005.These varied,but significant improvements are con-sistent with the experimental results of He et al.(2007),Ma and Tong(2007)and Su et al.(2007).Their research showed that the winter wheat yields for conservation tillage were9–23%higher than for conventional tillage in arid northern China.This sig-nificant improvement under no-tillage management was attrib-uted to increased soil nutrient and organic matter status.4.ConclusionsNo-tillage with straw cover and conventional tillage with straw removal had different effects on soil physical properties,organic matter content,nutrient concentrations,and microbial biomass and activity during the16-year experiment presented in this study. Continuous long-term conservation tillage practice(no-tillage, straw cover)significantly increased SOM,TN,available P,soil microbial biomass C and N in the topsoil(0to10cm)layer. Furthermore,the results of q CO2show that a greater microbial biomass respiration at a lower rate could be achieved by con-servation tillage,which is attributed to higher nutrient availability for microorganisms in no-tillage with straw cover soils.Below 10cm,the trends were reversed.This is attributed to lower degree of mixing straw below the topsoil under no-tillage treatments. Overall,no-tillage with straw cover also appears to improve nutrient supply.The long-term effect on yields is positive,but appears to be highly variable.This indicates that NTSC might require fertilizing for stabilizing yields.This study demonstrated that conservation tillage offers a significant improvement for the current farming systems in dryland areas of northern China.While generally in agreement with other studies,a range of effects of no-tillage soil management on soil condition and yields differed from those reported in the literature,for example the strong increase in N or the moderate and stratified increase in SOM,which is of particular interest due to its implications for climate change.These differences indicate that further research into the soil processes responsible for the long-term effects of no-tillage soil management is required.A particular emphasis should be given to understanding the influence of time under no-tillage required to achieve equilibrium soil conditions and stable yields.Only by identifying the role of time,the range of data from studies in China and worldwide can be compared comprehensively.AcknowledgementsThis work was financed by the Australian Centre for In-ternational Agricultural Research(ACIAR)and Ministry of Ag-riculture(MOA),China.We are grateful to Mr.Deng Jing and Zhou Wanrong for managing the field experiment.Also thanks to all the postgraduate students working in the Conservation Tillage Research Centre,MOA,who provided their data and assistance. ReferencesAlef,K.,1995.Estimation of soil respiration.In:Alef,K.,Nannipieri,P.(Eds.), Methods in Soil Microbiology and Biochemistry.Academic Press,New York, pp.464–470.Alvarez,R.,Diaz,R.A.,Barbero,N.,Santanatoglia,O.J.,Blotta,L.,1995.Soil organic carbon,microbial biomass and CO2–C production from three tillage systems.Soil Tillage Res.33,17–28.Black,C.A.,1965.Methods of Soil Analysis,Part I and II.Amer Soc.Inc.Pub., Madison,U.S.A.,pp.770–779.Brevik,E.,Fenton,T.,Moran,L.,2002.Effect of soil compaction on organic carbon amounts and distribution,South-central Iowa.Environ.Pollut.116, 137–141.Chowdhury,M.H.,Biswas,S.,Halim,M.A.,Haque,S.M.,Muhammed,N., Koike,M.,parative analysis of some selected macronutrients of soil in orange orchard and degraded forests in Chittagong Hill Tracts, Bangladesh.J.For.Res.18,27–30.Doran,J.W.,Parkin,T.B.,1994.Defining and assessing soil quality.In:Doran, J.W.,Coleman,J.W.,Bezdicek,D.F.(Eds.),Defining Soil Quality for a Sustainable Environment.Soil Sci.Soc.Am.Special Publ.,vol.35.ASA-SSSA,Madison,WI,pp.3–21.Ellert,B.H.,Janzen,H.H.,1999.Short-term influence of tillage no CO2fluxes from a semi-arid soil on the Canadian Prairies.Soil Tillage Res.50,21–32. Embacher,A.,Zsolnay,A.,Gattinger,A.,Munch,J.C.,2007.The dynamics of water extractable organic matter(WEOM)in common arable topsoils:I.Quantity,quality and function over a three year period.Geoderma139,11–22. Franzluebbers,A.J.,Arshad,M.A.,1996.Soil organic carbon pools during early adoption of conservation tillage in Northwestern Canada.Soil Sci.Soc.Am.J.60,1422–1427.Gao,H.W.,Li,H.W.,Chen,J.D.,1999.Research on sustainable mechanized dryland farming.Agric.Res.Arid Areas1,57–62(in Chinese).He,J.,Li,H.W.,Wang,X.Y.,McHugh,A.D.,Li,W.Y.,Gao,H.W.,Kuhn,N.J., 2007.The adoption of annual subsoiling as conservation tillage in dryland maize and wheat cultivation in northern China.Soil Tillage Res.94,493–502. Hedley,M.J.,Stewar,J.B.,1982.Method to measure microbial phosphate in soils.Soil Biol.Biochem.14,377–385.Jenkinson,D.S.,1988.Determination of microbial biomass carbon and nitrogen in soil.In:Wilson,J.R.(Ed.),Advances in Nitrogen Cycling in Agricultural Ecosystems Wallingford.CAB International,pp.368–386.Kang,H.,Zhu,B.A.,Hong,L.H.,Dong,C.J.,2001.The effect of no-tillage with straw cover on soil fertility and wheat yield.Shaanxi Agric.Sci.9, 1–3(in Chinese).Karlen,D.L.,Wollenhaupt,N.C.,Erbach,D.C.,Berry,E.C.,Swan,J.B.,Eash, N.S.,Jordahl,J.L.,1994.Long-term tillage effects on soil quality.Soil Tillage Res.32,313–327.Koch,H.J.,Stockfisch,N.,2006.Loss of soil organic matter upon ploughing under a loess soil after several years of conservation tillage.Soil Tillage Res.86,73–83.Kumar,K.,Goh,K.M.,2000.Biological nitrogen fixation,accumulation of soil nitrogen and nitrogen balance for white clover(Trifolium repens L.)and field pea(Pisum sativum L.)grown for seed.Field Crop Res.68,49–59. Lal,R.,Bruce,J.P.,1999.The potential of world cropland soils to sequester C and mitigate the greenhouse effect.Environ.Sci.Policy2,177–185.Li,H.W.,Gao,H.W.,Feng,X.J.,Wang,X.Y.,Du,B.,2005.Contribution of farmland wind erosion to sand storms in northern China.proceedings of SPIE—the international society for optical engineering,San Diego,CA, United States,pp.1–11.Li,L.,Li,S.J.,Zhang,H.L.,Chen,F.,2006.Study on soil C pool management index of conservation tillage.J.Soil Water Conserv.20,106–109(in Chinese). Liang,A.Z.,Zhang,X.P.,Fang,H.J.,Yang,X.M.,Drury,C.F.,2007.Short-term effects of tillage practices on organic carbon in clay loam soil of Northeast China.Pedosphere17,619–623.Liu,L.J.,2004.Systematic experiments and effect analysis of all year conservation tillage in two crops a year region.Ph.D.Dissertation,China agricultural University,Beijing,China(in Chinese).Luo,Z.Z.,Huang,G.B.,Zhang,G.S.,2005.Effects of conservation tillage on bulk density and water infiltration of surface soil in semi-arid area of west Loess Plateau.Agric.Res.Arid Areas23,7–11(in Chinese).Ma,G.Z.,Tong,H.,2007.The study and analyzing of the affection to the winter wheat by conservation agricultural technology in the dry land farming area.J.Agric.Mech.Res.5,139–142(in Chinese).Piper,C.S.,1950.Soil and plant analysis.Adelaide Univ.Hassel Press Australia3.pp.368.507Q.Wang et al./Geoderma144(2008)502–508。
关于路易斯巴斯德的英语作文

关于路易斯巴斯德的英语作文Louis Pasteur was a French chemist and microbiologist whose work laid the foundation of modern hygiene and germ theory. His contributions to science are immeasurable, and his discoveries have saved countless lives.Pasteur's most famous experiment was the "Swine Fever Experiment," which demonstrated that microorganisms could only come from other microorganisms, not spontaneously generate. This concept, known as "pasteurization," is still used today to preserve food and beverages.His work in immunology led to the development of thefirst vaccines for rabies and anthrax. Pasteur's dedication to research and the pursuit of knowledge has left a lasting impact on the field of medicine.Pasteur was not just a brilliant scientist but also a visionary who understood the practical applications of his findings. His work in pasteurization has made food safer and more accessible, improving the quality of life for people around the world.Despite facing numerous challenges and setbacks,Pasteur's perseverance and innovative spirit have inspired generations of scientists. His legacy continues to influence the way we approach health, disease, and the microscopic world.In conclusion, Louis Pasteur's life and work exemplify the power of scientific inquiry and the importance of applying knowledge for the betterment of society. His legacy serves as a reminder of the potential for scientific discovery to transform the world.。
微生物在药用中的作用300字作文

微生物在药用中的作用300字作文英文回答:Microorganisms play a crucial role in medicine. They have both positive and negative effects on human health. Let's focus on their positive contributions.Firstly, microorganisms are used in the production of antibiotics. Antibiotics are essential in treatingbacterial infections. They work by killing or inhibiting the growth of bacteria. Many antibiotics, such aspenicillin and erythromycin, are derived from microorganisms like fungi and bacteria. These medications have saved countless lives and continue to be a cornerstone of modern medicine.Secondly, microorganisms are involved in the production of vaccines. Vaccines are crucial in preventing the spread of infectious diseases. They contain weakened or killed microorganisms that stimulate the immune system to produceantibodies. These antibodies provide immunity againstfuture infections. Vaccines have eradicated orsignificantly reduced the incidence of diseases like polio, measles, and smallpox.Furthermore, microorganisms are used in the productionof various biologics, such as insulin and growth hormones. These biologics are used to treat conditions like diabetes and growth disorders. Microorganisms are genetically engineered to produce these substances in large quantities, making them more accessible and affordable for patients.In addition to their direct medical applications, microorganisms also play a role in research and development. They are used in studying diseases, testing the efficacy of drugs, and understanding the mechanisms of variousbiological processes. Microorganisms, especially bacteria, are also used as model organisms in genetic studies.In conclusion, microorganisms have a significant impact on medicine. They are essential in the production of antibiotics, vaccines, and other biologics. They alsocontribute to research and development in the field of medicine. Without microorganisms, many medical advancements and treatments would not be possible.中文回答:微生物在药用中扮演着至关重要的角色。
微生物工程生产次级代谢产物的流程
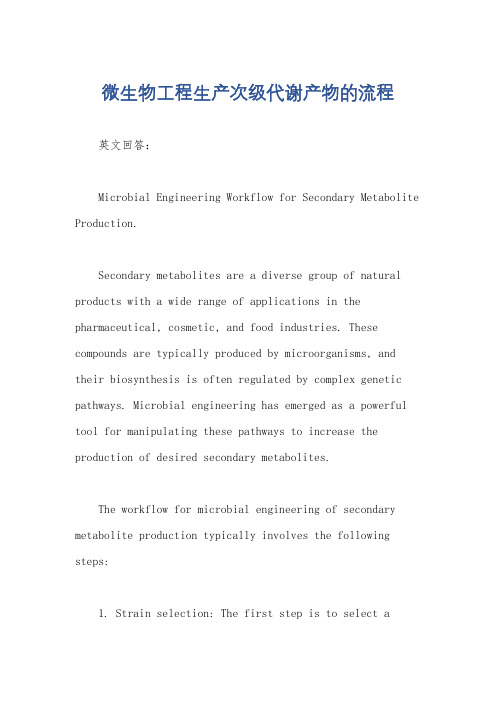
微生物工程生产次级代谢产物的流程英文回答:Microbial Engineering Workflow for Secondary Metabolite Production.Secondary metabolites are a diverse group of natural products with a wide range of applications in the pharmaceutical, cosmetic, and food industries. These compounds are typically produced by microorganisms, and their biosynthesis is often regulated by complex genetic pathways. Microbial engineering has emerged as a powerful tool for manipulating these pathways to increase the production of desired secondary metabolites.The workflow for microbial engineering of secondary metabolite production typically involves the following steps:1. Strain selection: The first step is to select asuitable microbial strain that is capable of producing the desired secondary metabolite. This may involve screening strains from natural sources or using genetically modified strains.2. Pathway elucidation: The next step is to elucidate the biosynthetic pathway for the secondary metabolite. This involves identifying the genes and enzymes involved in the pathway, as well as their regulatory mechanisms.3. Genetic engineering: Once the biosynthetic pathway has been elucidated, genetic engineering techniques can be used to manipulate the pathway to increase the production of the desired secondary metabolite. This may involve overexpressing genes encoding key enzymes, deleting genes that inhibit production, or introducing genes from other organisms that encode desired enzymes.4. Culture optimization: Once the microbial strain has been engineered, the culture conditions can be optimized to maximize secondary metabolite production. This may involve optimizing the growth medium, temperature, pH, and otherfactors.5. Downstream processing: The final step is to extract and purify the secondary metabolite from the culture broth. This may involve a variety of techniques, such asfiltration, chromatography, and crystallization.中文回答:微生物工程生产次级代谢产物的流程。
微生物组学英语
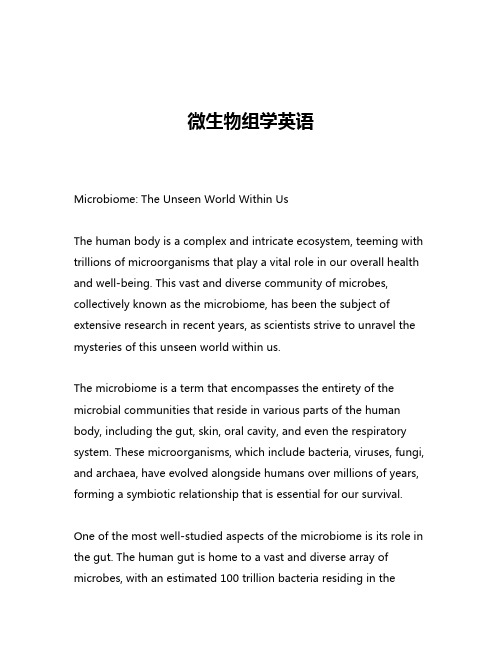
微生物组学英语Microbiome: The Unseen World Within UsThe human body is a complex and intricate ecosystem, teeming with trillions of microorganisms that play a vital role in our overall health and well-being. This vast and diverse community of microbes, collectively known as the microbiome, has been the subject of extensive research in recent years, as scientists strive to unravel the mysteries of this unseen world within us.The microbiome is a term that encompasses the entirety of the microbial communities that reside in various parts of the human body, including the gut, skin, oral cavity, and even the respiratory system. These microorganisms, which include bacteria, viruses, fungi, and archaea, have evolved alongside humans over millions of years, forming a symbiotic relationship that is essential for our survival.One of the most well-studied aspects of the microbiome is its role in the gut. The human gut is home to a vast and diverse array of microbes, with an estimated 100 trillion bacteria residing in thedigestive tract. These gut microbes play a crucial role in digesting and metabolizing the food we consume, extracting essential nutrients and energy that our bodies can then utilize.Beyond their role in digestion, gut microbes also have a profound impact on our immune system. They help to train and regulate the immune cells, ensuring that they are able to effectively fight off harmful pathogens while also maintaining a delicate balance that prevents autoimmune disorders. This intricate relationship between the gut microbiome and the immune system has been the focus of numerous studies, with researchers exploring the potential of probiotics and other microbial-based therapies to treat a wide range of health conditions.The skin microbiome is another area of intense research. The skin is the largest organ in the human body and is home to a diverse array of microbes, including bacteria, fungi, and viruses. These skin-dwelling microbes play a crucial role in maintaining the skin's barrier function, protecting us from harmful environmental factors and pathogens. They also contribute to the skin's overall health, helping to regulate inflammation, prevent the overgrowth of harmful microbes, and even influence the appearance of the skin.The oral microbiome is another important aspect of the human microbiome. The mouth is a complex ecosystem, with a diverse arrayof microbes that play a critical role in maintaining oral health. These microbes help to break down food, regulate pH levels, and prevent the overgrowth of harmful bacteria that can lead to dental problems such as cavities and gum disease.In addition to these well-known aspects of the microbiome, there is growing evidence that the microbial communities in other parts of the body, such as the respiratory system and the urogenital tract, also play important roles in human health and disease.One of the most exciting areas of microbiome research is the potential for microbiome-based therapies to treat a wide range of health conditions. By understanding the composition and function of the microbiome, researchers are exploring ways to manipulate it to improve human health. This includes the use of probiotics, which are live microorganisms that can be consumed to help restore the balance of the microbiome, as well as the development of personalized therapies that target specific microbial imbalances.Another promising area of research is the role of the microbiome in mental health. Emerging evidence suggests that the gut microbiome may play a significant role in the development and maintenance of mental health disorders, such as depression and anxiety. This has led to the concept of the "gut-brain axis," which posits that the bidirectional communication between the gut and the brain can havea profound impact on our emotional and cognitive well-being.As our understanding of the microbiome continues to grow, it is clear that this unseen world within us is a critical component of human health and well-being. By unraveling the complexities of the microbiome, researchers and clinicians are paving the way for new and innovative approaches to disease prevention and treatment. From improving gut health to enhancing mental well-being, the potential of the microbiome is limitless, and the future of personalized, microbiome-based medicine is rapidly taking shape.。
杂乱的纤维素
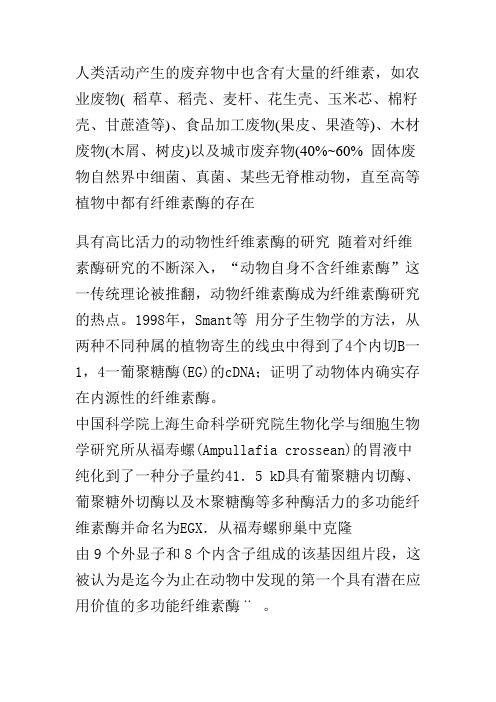
人类活动产生的废弃物中也含有大量的纤维素,如农业废物( 稻草、稻壳、麦杆、花生壳、玉米芯、棉籽壳、甘蔗渣等)、食品加工废物(果皮、果渣等)、木材废物(木屑、树皮)以及城市废弃物(40%~60% 固体废物自然界中细菌、真菌、某些无脊椎动物,直至高等植物中都有纤维素酶的存在
具有高比活力的动物性纤维素酶的研究随着对纤维素酶研究的不断深入,“动物自身不含纤维素酶”这一传统理论被推翻,动物纤维素酶成为纤维素酶研究的热点。
1998年,Smant等用分子生物学的方法,从两种不同种属的植物寄生的线虫中得到了4个内切B一1,4一葡聚糖酶(EG)的cDNA;证明了动物体内确实存在内源性的纤维素酶。
中国科学院上海生命科学研究院生物化学与细胞生物学研究所从福寿螺(Ampullafia crossean)的胃液中纯化到了一种分子量约41.5 kD具有葡聚糖内切酶、葡聚糖外切酶以及木聚糖酶等多种酶活力的多功能纤维素酶并命名为EGX.从福寿螺卵巢中克隆
由9个外显子和8个内含子组成的该基因组片段,这被认为是迄今为止在动物中发现的第一个具有潜在应用价值的多功能纤维素酶¨。
找到适合于工业生产的、高活性的纤维素酶是目前将纤维素物质转化为再生能源的关键点,动物性纤维素酶的研究为我们增添了一个解决问题的方向。
有报道指日本一家实验室从甲虫中得到一种葡聚糖内切酶水解羧甲基纤维素(CMC.Na)的比活力可高达150 IU/mg 。
兽医微生物学英语
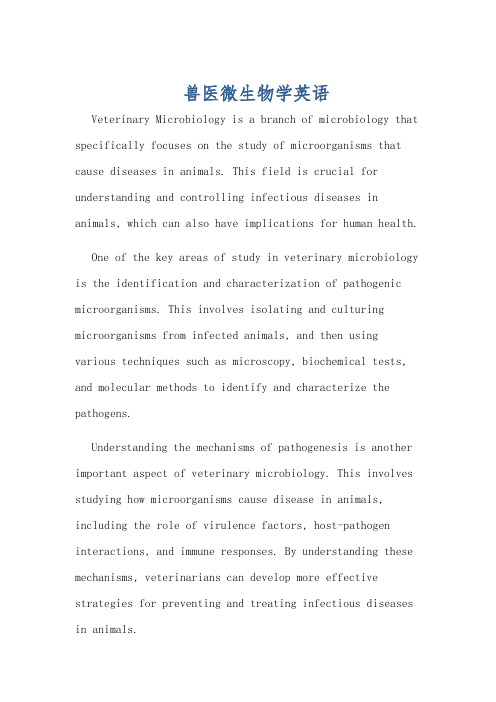
兽医微生物学英语Veterinary Microbiology is a branch of microbiology that specifically focuses on the study of microorganisms that cause diseases in animals. This field is crucial for understanding and controlling infectious diseases in animals, which can also have implications for human health.One of the key areas of study in veterinary microbiology is the identification and characterization of pathogenic microorganisms. This involves isolating and culturing microorganisms from infected animals, and then using various techniques such as microscopy, biochemical tests, and molecular methods to identify and characterize the pathogens.Understanding the mechanisms of pathogenesis is another important aspect of veterinary microbiology. This involves studying how microorganisms cause disease in animals, including the role of virulence factors, host-pathogen interactions, and immune responses. By understanding these mechanisms, veterinarians can develop more effective strategies for preventing and treating infectious diseases in animals.Epidemiology is also a critical component of veterinary microbiology. This involves studying the distribution and spread of infectious diseases in animal populations, aswell as identifying risk factors and potential sources of infection. Epidemiological studies are essential for implementing control measures and preventing outbreaks of infectious diseases in animal populations.In addition to studying infectious diseases, veterinary microbiologists also play a key role in monitoring and controlling zoonotic diseases, which are diseases that can be transmitted from animals to humans. By understanding the epidemiology and transmission dynamics of zoonotic diseases, veterinarians can help prevent the spread of these diseases to humans and protect public health.In summary, veterinary microbiology is a diverse and essential field that encompasses the study of pathogenic microorganisms, mechanisms of pathogenesis, epidemiology, and zoonotic diseases. By advancing our understanding of infectious diseases in animals, veterinary microbiologists contribute to the health and well-being of both animals and humans.兽医微生物学是微生物学的一个分支,专门研究导致动物疾病的微生物。
以色列二穗短柄草谷胱甘肽过氧化物酶活性分析

Y N J n’ X EW nto, E Y W N i — e , H N u. ag , H N a -i ( . o A u , U e— H a , A GXn m i Z O GG i in 。 C E GJ npn a e g x i g 1 C1 .
ro . Alo,sg i c n i ee c r b e v d a n 0 c tp s ot s i f a tdf rn eweeo s r e mo g1 5 e oy e .Ex e tr o ,e c ft eGS P cii n i c p o t a h o h H— x a tv—
由遗传 决定的 , 为模式植物的遗传 差异性对于禾谷 类植物 的遗传和基 因比较分析 等极具价值。 作 关键词 : 式植物 ; 色列; 模 以 二穗短 柄草 ; 胱甘肽过氧化物酶 谷
中 图分 类 号 :9 53 Q 4 .5 文献标识码 : A 文 章 编 号 : 0 —05 (0 1 0 0 9 0 1 8 4 7 2 1 ) 3— 18— 5 0
严 俊 , 薛文韬 何 , 跃 王兴梅 钟桂香 程剑 平 , , ,
395 10 ;
( .贵州大学 生命科学学 院, 1 贵州 贵阳 502 ;. 505 2 海法大学 进化研究所 , 以色列 海法 3 贵 州大 学 农 学 院 , 州 贵 阳 5 02 ) . 贵 5 05
摘
要: 二穗短柄草是一种理想的研 究温带禾谷类植物和牧 草的模 式植物。本文对 来源于以 色列 4个不 同地 区
e c s O h H— x a tvte fro ,se a d la at fB.di a h o r w n te n tr lmai o - n e n t e GS P cii so o t tm n e fp rso i s c y n g e i h au a ci t c n t l c
IVM

一段关于伊维菌素对不同类型寄生虫有效给药剂量和有效血液浓度的文字,并注明相应参考文献。
《Plumb';s兽药手册++第5版》中关于伊维菌素的具体信息见于附加文件夹,伊维菌素对不同种属动物不同寄生虫的剂量简要总结如下:用于犬时,预防犬心丝虫病的剂量为6μg/kg,每月口服1次;用作微丝蚴杀虫剂时,50~200 μg/kg;用作外用杀虫剂,疥螨耳螨为300 μg/kg,重复给药14天,毛囊虫为400~600 μg/(kg·d);用作体内杀虫药时,肺部寄生虫为200 μg/kg(口服),奥氏奥斯勒丝虫为400 μg/kg (皮下注射),Eucoleusboehmi为200 μg/kg(口服),犬类肺刺螨为200 μg/kg(皮下注射)。
用于猫时,预防心丝虫的最小有效剂量为24 μg/kg(口服),杀灭莫名猫圆线虫剂量为400 μg/kg(皮下注射)。
此外,伊维菌素还可以用作治疗马、牛、绵羊、美洲驼的敏感寄生虫药物,一般剂量为200 μg/kg;用作猪敏感寄生虫时,一般剂量为300 μg/kg;用作雪貂预防心丝虫时为20 μg/kg;用于禽时,稍有区别;用作爬行动物、兔、啮齿类、袖珍宠物寄生虫治疗药物时,一般剂量在200~500 μg/kg之间,皮下或口服给药。
百度百科中关于伊维菌素的介绍:1.药效学:适用于盘尾丝虫病和类圆线虫病及钩虫、蛔虫、鞭虫、蛲虫感染。
由于吸虫和绦虫不以GABA为传递递质,并且缺少受谷氨酸控制的Cl通道,故本类药物对其无效。
2.药动学:伊维菌素的药代动力学因畜种、剂型和给药途径不同而有明显差异。
以血浆半衰期为例,给牛、绵羊静脉注射300μg/kg量,t1/2虽然差别不大(分别为2.8天和2.7天),但羊的血浆浓度较低是由于表观分布容积大于牛所致。
伊维菌素在犬体内排泄较快(t1/2=1.6~1.8天)。
猪的半衰期长达4天。
用美国专用的商品制剂给牛皮下注射(200μg/kg),由于从注射局部缓慢吸收,而半衰期延长(t1/2=8天),.48h血药达峰值,据临床驱虫效果观察,药效能维持2周。
嗜热脂肪芽孢杆菌高温中性蛋白酶在枯草芽孢杆菌中的表达_纯化及其酶学性质的研究.kdh
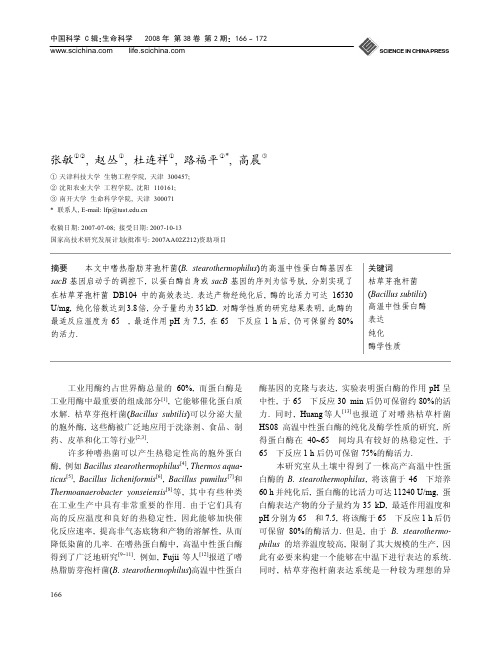
中国科学C辑:生命科学 2008年 第38卷 第2期: 166~172 166 《中国科学》杂志社SCIENCE IN CHINA PRESS嗜热脂肪芽孢杆菌高温中性蛋白酶在枯草芽孢杆菌中的表达、纯化及其酶学性质的研究张敏①②, 赵丛①, 杜连祥①, 路福平①*, 高晨③①天津科技大学生物工程学院, 天津 300457;②沈阳农业大学工程学院, 沈阳 110161;③南开大学生命科学学院, 天津 300071* 联系人, E-mail: lfp@收稿日期: 2007-07-08; 接受日期: 2007-10-13国家高技术研究发展计划(批准号: 2007AA02Z212)资助项目摘要本文中嗜热脂肪芽孢杆菌(B. stearothermophilus)的高温中性蛋白酶基因在sacB基因启动子的调控下, 以蛋白酶自身或sacB基因的序列为信号肽, 分别实现了在枯草芽孢杆菌DB104中的高效表达. 表达产物经纯化后, 酶的比活力可达16530 U/mg, 纯化倍数达到3.8倍, 分子量约为35 kD. 对酶学性质的研究结果表明, 此酶的最适反应温度为65,℃最适作用pH为7.5, 在65℃下反应1 h后, 仍可保留约80%的活力. 关键词枯草芽孢杆菌(Bacillus subtilis) 高温中性蛋白酶表达纯化酶学性质工业用酶约占世界酶总量的60%, 而蛋白酶是工业用酶中最重要的组成部分[1], 它能够催化蛋白质水解. 枯草芽孢杆菌(Bacillus subtilis)可以分泌大量的胞外酶, 这些酶被广泛地应用于洗涤剂、食品、制药、皮革和化工等行业[2,3].许多种嗜热菌可以产生热稳定性高的胞外蛋白酶, 例如Bacillus stearothermophilus[4], Thermos aqua-ticus[5], Bacillus licheniformis[6], Bacillus pumilus[7]和Thermoanaerobacter yonseiensis[8]等, 其中有些种类在工业生产中具有非常重要的作用. 由于它们具有高的反应温度和良好的热稳定性, 因此能够加快催化反应速率, 提高非气态底物和产物的溶解性, 从而降低染菌的几率. 在嗜热蛋白酶中, 高温中性蛋白酶得到了广泛地研究[9∼11]. 例如, Fujii等人[12]报道了嗜热脂肪芽孢杆菌(B. stearothermophilus)高温中性蛋白酶基因的克隆与表达, 实验表明蛋白酶的作用pH呈中性, 于65℃下反应30 min后仍可保留约80%的活力. 同时, Huang等人[13]也报道了对嗜热枯草杆菌HS08高温中性蛋白酶的纯化及酶学性质的研究, 所得蛋白酶在40~65℃间均具有较好的热稳定性, 于65℃下反应1 h后仍可保留75%的酶活力.本研究室从土壤中得到了一株高产高温中性蛋白酶的B. stearothermophilus, 将该菌于46℃下培养60 h并纯化后, 蛋白酶的比活力可达11240 U/mg, 蛋白酶表达产物的分子量约为35 kD, 最适作用温度和pH分别为65℃和7.5, 将该酶于65℃下反应1 h后仍可保留80%的酶活力. 但是, 由于 B. stearothermo-philus的培养温度较高, 限制了其大规模的生产, 因此有必要来构建一个能够在中温下进行表达的系统. 同时, 枯草芽孢杆菌表达系统是一种较为理想的异中国科学 C 辑: 生命科学 2008年 第38卷 第2期167源蛋白表达系统[14], 其优点在于可以高效地表达具有生物学活性的异源蛋白并将其分泌至培养基中[15], 而且枯草芽孢杆菌也具有较高的生物安全性.因此, 本文将高温中性蛋白酶基因克隆入B. sub-tilis , 来构建一个对高温中性蛋白酶基因进行诱导表达的系统, 使该基因处于sacB 基因启动子的调控下, 并分别以自身或sacB 基因的序列作为信号肽. 同时, 对高温中性蛋白酶的纯化及酶学性质也进行了研究.1 材料与方法1.1 菌种、质粒和培养条件B. subtilis DB104是双蛋白酶缺陷型菌株, 作为蛋白酶的表达宿主[16]; 大肠杆菌(E. coli ) JM109是基因操作的克隆宿主[17], 2个菌株均于37℃下LB 培养基中进行培养. 重组大肠杆菌用LB 培养基(含30 µg/mL 氯霉素(Cm))进行培养和筛选, 而重组枯草芽孢杆菌用LB 培养基(含5 µg/mL 红霉素(Em))进行培养和筛选.pBSAT 质粒上含有sacB 基因, 由本研究室构建. 质粒pHP13PS 是由pHP13载体(E. coli -B. subtilis 穿梭载体)构建而来的, 其上含有sacB 基因的启动子和信号肽序列; 质粒pHP13P 也是由pHP13载体构建而来的, 但其仅含有sacB 基因的启动子序列.1.2 DNA 的操作本实验所采用的限制性内切酶、T4 DNA 连接酶和Taq DNA 聚合酶均购自TaKaRa 公司; DNA 片段的分离纯化、酶切反应、琼脂糖凝胶电泳、DNA 连接以及对大肠杆菌的转化等操作均参照分子克隆实验指南[17].1.3 高温中性蛋白酶序列的克隆根据已发表的高温中性蛋白酶基因的全序列, 设计用于扩增高温中性蛋白酶基因(nprT )的一对引物, 此基因包括信号肽、前肽和成熟肽序列. 两个引物序列分别为5′-CCGGTCGACGGGAAAATTGGAAAA- TGAAAAGG-3′(Sal Ⅰ)和5′-CCGAATTCACATCAG- TGGAGGAAAAAATCCCCCA-3′(Eco R Ⅰ). PCR 反应条件: 94℃, 5 min; 94℃, 1 min, 55℃, 1 min, 72℃, 2 min, 35个循环; 72℃, 10 min.根据nprT 基因序列, 设计用于扩增nprT"的2个引物, nprT"基因不包含信号肽序列. 2个引物序列分别为: 5′-CCCCCGGGGCCCAAGTATTTTTACCTTAC- AAT-3′(Sma Ⅰ)和5′-CCGAATTCACATCAGTGGAG- GAAAAAATCCCCCA-3′(Eco R Ⅰ). 扩增的产物利用琼脂糖凝胶进行分离纯化, 并通过DNA 测序进行验证.1.4 诱导型表达载体的构建将用Sal Ⅰ和Eco R Ⅰ双酶切后大小为1725 bp 的nprT 基因片段, 克隆入同样用Sal Ⅰ和Eco R Ⅰ双酶切的pHP13P 载体上, 得到质粒pHP13PN, 该质粒上nprT 基因处于sacB 基因的启动子和自身信号肽序列的调控下(图1(a)); 将用Sma Ⅰ和Eco R Ⅰ双酶切后大小为1651 bp 的nprT"基因片段, 克隆入同样用Sma Ⅰ和Eco R Ⅰ双酶切的pHP13PS 载体上, 得到质粒pHP13PSN, 该质粒上npr T"基因处于sacB 基因的启动子和信号肽序列的调控下(图1(b)).1.5 高温中性蛋白酶基因的诱导表达将质粒pHP13PN 和pHP13PSN 按照Chang 等 人[18]的方法转化入B. subtilis DB104中, 分别得到重组子DB104/pHP13PN 和DB104/pHP13PSN. 再将重组子接入LB 培养基(含5 µg/mL 红霉素(Em)中, 于37℃, 200 r/min 的条件下培养2 h 后, 加入2%的蔗糖溶液诱导高温中性蛋白酶基因的表达, 以未加蔗糖溶液的作为对照; 继续培养50 h, 将培养液于4℃, 14000× g 离心15 min 后收集上清以测定其酶活力. 同时, 用SDS- PAGE 法来检测高温中性蛋白酶基因的表达情况.1.6 高温中性蛋白酶(NprT)的纯化将培养物上清液用饱和度为80%的硫酸铵溶液进行沉淀后, 再加入20 mL 的Tris-HCl 缓冲液 (50 mmol/L, pH 7.5)溶解沉淀, 并于4℃下用同样的缓冲液透析12 h, 透析得到的溶液经DEAE-Sephadex A25柱层析后收集含有NprT 的部分, 再次用80%饱和度的硫酸铵溶液进行沉淀后, 以0.3 mL/min 的速率用DEAE-Sephadex A50柱进行洗脱.1.7 蛋白酶NprT 酶活力的测定蛋白酶活力的测定是将1 mL 1%的酪素溶液(pH 7.5)与1 mL 的酶稀释液混匀, 于65℃下反应10 min张敏等: 嗜热脂肪芽孢杆菌高温中性蛋白酶在枯草芽孢杆菌中的表达、纯化及其酶学性质的研究168图1 质粒的构建图谱(a) 质粒pHP13PN包含由sacB基因启动子和自身信号肽序列调控的nprT基因; (b) 质粒pHP13PSN包含由sacB基因启动子和信号肽序列调控的nprT"基因. Cm和Em分别为E. coli和B. subtilis中的筛选标记后, 加入2 mL 10%的三氯乙酸溶液(TCA), 充分振荡后于14000×g下离心20 min, 取1 mL上清液采用Folin酚法测定酪氨酸的含量[19]. 蛋白酶活力单位定义为: l mL液体酶在65℃和pH 7.5的条件下, l min水解酪素产生l µg酪氨酸为1个酶活力单位.1.8温度和pH对NprT酶活力的影响将溶于Tris-HCl 缓冲液(50 mmol/L, pH 7.5) 的NprT分别于40~75℃下进行反应, 以测定纯化后NprT的最适反应温度. 纯化NprT的最适作用pH于65℃下分别在不同的缓冲体系中进行测定, 3种缓冲液分别为50 mmol/L乙酸钠溶液(pH 4.0~5.5)、50 mmol/L磷酸钠溶液(pH 6.0~7.0)和50 mmol/L Tris- HCl溶液(pH 7.5~9.0). 为测定NprT的热稳定性, 将溶于50 mmol/L Tris-HCl缓冲液(pH 7.5)的纯酶于65℃下作用不同的时间后, 测定其剩余的酶活力.2结果与分析2.1质粒pHP13PSN和pHP13PN的构建通过PCR扩增分别得到nprT和nprT"基因, nprT 基因包括信号肽序列(1~57 bp)、前肽序列(58~708 bp)和成熟肽序列(709~1656 bp)(图2)[20]. 为研究高温中性蛋白酶基因在B. subtilis DB104中的表达, 构建了2个不同的表达载体. 其中, 质粒pHP13PN包含sacB 基因的启动子和nprT基因的信号肽序列(图1(a)), 而质粒pHP13PSN包含nprT"基因以及位于其上游的sacB基因的启动子和信号肽序列(图1(b)). 利用含Em的LB平板来筛选重组子, 每次筛选大约有20~30个转化子, 然后通过酶切和PCR扩增来验证插入基因片段的正确性.为确认质粒pHP13PSN和pHP13PN中sacB基因序列与高温中性蛋白酶基因序列之间的可读框连接正确, 我们分别测定了整个基因序列. 结果表明, 两个连接处的读码框均正确, 并且本实验克隆的高温中性蛋白酶基因序列与已报道的基因序列有99%的同源性(GenBank登录号: M21663).2.2高温中性蛋白酶基因在B. subtilis DB104中的表达将构建的重组质粒pHP13PSN和pHP13PN分别转化B. subtilis DB104后, 向培养液中分别加入蔗糖溶液来诱导高温中性蛋白酶基因的表达, 利用12% SDS-PAGE来进行检测. 电泳结果表明, NprT存在于培养物上清液中, 同时也表明NprT被成功地分泌到胞外(图3).在培养过程中, 定时取样测定上清液中NprT的酶活力(图4). 结果显示, 重组子pHP13PN/DB104培养物上清液中的NprT酶活力达7020 U/mL, 而重组子pHP13PSN/DB104培养物上清液中的NprT酶活力高达13580 U/mL, 表明sacB基因的信号肽序列对于nprT基因的表达效果更显著. 对含有NprT的粗酶液进行纯化(表1), 当纯化倍数为3.8时, 其比活力可达中国科学C辑: 生命科学 2008年第38卷第2期图2 nprT基因的核苷酸和氨基酸序列169张敏等: 嗜热脂肪芽孢杆菌高温中性蛋白酶在枯草芽孢杆菌中的表达、纯化及其酶学性质的研究170图3 高温中性蛋白酶基因在B. subtilis DB104中表达产物的聚丙烯酰胺凝胶电泳分析M: 分子量标记; 1: pHP13/DB104培养物的上清液(对照组); 2: pHP13PN/DB104培养物的上清液; 3: pHP13PSN/DB104培养物的上清液图4 高温中性蛋白酶基因在B. subtilis DB104中表达情况的比较■: 质粒pHP13PN上含有nprT基因和自身的信号肽序列; ▲: 质粒pHP13PSN上含有nprT"基因和sacB基因的信号肽序列; ×: 质粒pHP13上未插入外源基因16530 U/mg, 提高了13.4%, 而Huang等人[13]的研究表明NprT的比活力仅为13172 U/mg, 提高 5.1%. SDS-PAGE分析的结果表明(图5), 有一分子量约为35 kD的特征蛋白带出现, 大小与出发菌株的一致.图5 NprT纯化的聚丙烯酰胺凝胶电泳分析M: 分子量标记; 1: 纯化NprT2.3温度和pH对NprT酶活力的影响将纯化的NprT分别于40~75℃温度下反应, 测定NprT酶活力. 结果表明(图6(a)), 酶的最适反应温度为65℃, 当反应温度超过65℃以后, 酶活力迅速下降; NprT的作用温度范围较宽, 为55~75℃, 并且在40~ 65℃之间, NprT具有较好的热稳定性; 在65℃下反应1 h后仍可保留约80%的酶活力, 因此, NprT可被定义为嗜热酶. 研究不同pH对NprT酶活力的影响, 结果表明(图6(b)), 当pH<5.5时, 酶活力较低, 当pH>5.5时, 酶活力迅速升高; NprT作用的最适pH为7.5, 在pH 6.5~9.0范围内均有较高的酶活力.3讨论由于 B. subtilis向胞外分泌高浓度的蛋白酶[21],中国科学 C 辑: 生命科学 2008年 第38卷 第2期171表1 NprT 的纯化结果步骤 体积/mL 总蛋白/mg 总酶活力/×104U 比活力/U·mg -1回收率/% 纯化倍数 粗酶液 500 525.5 228.6 4350 100 1 (NH 4)2SO 4盐析 300 387.2 195.1 5040 85.3 1.15 DEAE-Sepharose A25 167 74.4 92.6 12450 40.5 2.86 DEAE-Sepharose A5093 18.5 30.6 16530 13.4 3.8图6 温度(a)和pH(b)对高温中性蛋白酶活力的影响以致影响了表达产物的稳定性, 而B. subtilis DB104是双蛋白酶缺陷型菌株, 有助于提高分泌蛋白的稳定性. 同时在枯草杆菌表达系统中使用诱导启动子系统, 可以使外源基因在加入诱导剂之前并不表达, 从而不会影响到宿主菌的生长; 当加入诱导剂后, 就可以使外源基因得到表达, 从而保证了外源蛋白的稳定性和高产量. 蔗糖诱导系统是B. subtilis 两大天然诱导系统之一, 由sacA 和sacB 两个基因组成. 其中, sacB 基因编码果聚糖蔗糖酶, 是一种受蔗糖诱导的胞外酶, 既具有蔗糖诱导表达所需要的诱导区域, 也具有使蛋白有效分泌的信号肽序列.对于中温菌 B. subtilis 的培养比高温菌 B. stearothermophilus 要容易得多, 将B. subtilis 作为高温中性蛋白酶基因的表达宿主会对酶的应用提供一个很有前景的方式. 本文中高温中性蛋白酶基因在 B. subtilis DB104中成功地实现了表达, 酶的比活力较出发菌株有所提高, 可达16530 U/mg. 实验结果表 明, sacB 基因的信号肽序列在引导高温中性蛋白酶进行分泌时所起的作用要优于蛋白酶自身的信号肽序列. 纯化酶的最适作用pH 和反应温度分别为7.5和65, ℃在65℃下反应1 h 后, 仍可保留约80%的活力. 实验结果也表明, 重组酶的性质与出发菌株的相同, 有必要对高温中性蛋白酶的动力学性质进行分析, 以确定蛋白酶的种类.本文首次利用sacB 诱导基因来构建一个对高温中性蛋白酶基因进行高效诱导表达的系统, sacB 基因的表达不仅可以利用蔗糖来诱导, 其表达水平也可以通过一些多效调控基因来得到提高, 例如degQ , degU 和degS 基因[22∼24]. 目前, 本研究室正在构建含有多效调控基因的重组质粒, 这样将会使高温中性蛋白酶基因的诱导表达水平有更大的提高.张敏等: 嗜热脂肪芽孢杆菌高温中性蛋白酶在枯草芽孢杆菌中的表达、纯化及其酶学性质的研究参考文献1 Nascimento W C A, Martins M L L. Production and properties of an extracellular protease from thermophilic Bacillus sp. Braz J Mi-crobiol, 2004, 35: 1—22 Pastor M D, Lorda G S, Balatti A. Protease obtention using Bacillus subtilis 3411 and amaranth seed meal medium at different aera-tion rates. Braz J Microbiol, 2001, 32: 1—83 Ward O P. Proteolytic Enzymes. Oxford: Pergamon Press, 1985. 789—8184 Sookkheo B, Sinchaikul S, Phutrakul S, et al. Purification and characterization of the highly thermostable proteases from Bacillusstearothermophilus TLS33. Protein Expr Purif, 2000, 20: 142—1515 Oledzka G, Dabrowski S, Kur J. High-level expression, secretion, and purification of the thermostable aqualysin I from Thermusaquaticus YT-1 in Pichia pastoris. Protein Expr Purif, 2003, 29: 223—2296 Ferrero M A, Castro G R, Abate C M, et al. Thermostable alkaline protease of Bacillus licheniformis MIR29: isolation, production andcharacterization. Appl Microbiol Biotechnol, 1996, 45: 327—3327 Kumar C G. Purification and characterization of a thermostable alkaline protease from alkalophilic Bacillus pumilus. Lett Appl Micro-biol, 2002, 34: 13—178 Hyenung J J, Byoung C K, Yu R P, et al. A novel subtilisn-like serine protease from Thermoanerobacter yonseiensis KB-1: its cloning,expression, and biochemical properties. Extremophiles, 2002, 6: 233—2439 Helmann J D. Compilation and analysis of Bacillus subtilisσA-dependent promoter sequences: evidence for extended contact betweenRNA polymerase and upstream promoter DNA. Nucleic Acids Res, 1995, 23: 2351—236010 Van den Burg B, Eijsink V G H, Veltman O R, et al. Evolution reversed: engineering an enzyme to resist boiling. Proc Natl Acad SciUSA, 1998, 95: 2056—206011 Rao M B, Tankasale A M, Ghatge M S, et al. Molecular and biotechnological aspects of microbial proteases. Microbiol Mol Biol Rev,1998, 62: 597—63412 Fujii M, Takagi M, Imanaka T, et al. Molecular cloning of a thermostable neutral protease gene from Bacillus stearothermophilus in avector plasmid and its expression in Bacillus stearothermophilus and Bacillus subtilis. J Bacteriol, 1983, 154: 831—83713 Huang G R, Ying T J, Huo P, et al. Purification and characterization of a protease from Thermophilic bacillus strain HS08. Afr J Bio-technol, 2006, 5(24): 2433—243814 Doi R H, Wong S L, Kawamura F. Potential use of Bacillus subtilis for secretion and production of foreign proteins. Trends Biotech-nol, 1986, 4: 232—23515 Goeddle D V. Expression in B. subtilis. Meth Enzymol, 1990, 85: 199—22816 Behnke D, Gerlach D. Cloning and expression in Escherichia coli, Bacillus subtilis, and Streptococcus sanguis of a gene for staphylo-kinase, a bacterial plasminogen activator. Mol Gen Genet, 1987, 210: 528—53417 Sambrook J, Fritsch E F, Maniatis T. Molecular Cloning: A Laboratory Manual. 2 ed. New York: Cold Spring Harbor Laboratory,198918 Chang S, Cohen S N. High-frequency transformation of Bacillus subtilis protoplast by plasmid DNA. Mol Gen Genet, 1979, 168:111—11519 Ledoux M, Lamy F. Determination of proteins and sulfobetaine with the folin-phenol reagent. Anal Biochem, 1986, 157: 28—3120 Kubo M, Imanaka T. Cloning and nucleotide sequence of the highly thermostable neutral protease gene from Bacillus stearothermo-philus. J Gen Microbiol, 1988, 134(7): 1883—189221 Priest F G. Extracellular enzyme synthesis in the genus Bacillus. J Bacteriol, 1977, 41: 711—75322 Ayusawa D, Yoneda Y, Yamane K, et al. Pleiotropic phenomena in autolytic enzyme(s) content, flagellation, and simultaneous hyper-production of extracellular α-amylase and protease in a Bacillus subtilis mutant. Bacteriol, 1975, 124: 459—46923 Jacobs M F. Expression of the subtilisin Carlsberg-encoding gene in Bacillus licheniformis and Bacillus subtilis. Gene, 1995, 152: 69—7424 Pan X F. Effects of degU32(Hy), degQa and degR pleiotropic regulatory genes on the growth and protease fermentation of BacillusSubtilis K i-2-132. Acta Genetica Sinica, 2006, 33: 373—380172。
chapter 2 Microbiology
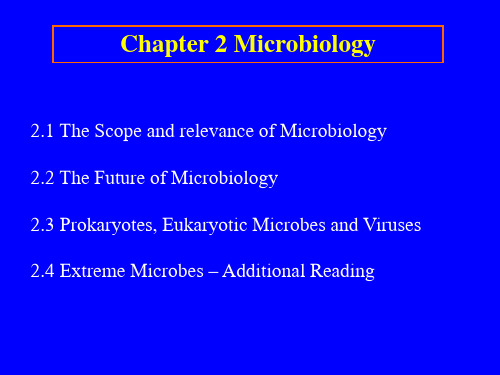
2.1 The Scope and relevance of Microbiology (Continued)
• What are some of the current occupations of professional microbiologists? • One of the most active and important is medical microbiology, which deals with the diseases of humans and animals. Medical microbiologists identify the agent causing an infectious disease and plan measures to eliminate it. Frequently they are involved in tracking down new, unidentified pathogens such as the agent that causes variant creutzfeldt-Jacob disease, the hantavirus, and the virus responsible for AIDS. These microbiologists also study the ways in which microorganisms cause disease. • Public health microbiology is closely related to medical microbiology. Public health microbiologists try to control the spread of communicable diseases. They often monitor community food establishments and water supplies in an attempt to keep them safe and free from infectious disease agents. • Immunology is concerned with how the immune system protects the body from pathogens and the response of infectious agents. It is one of the fastest growing areas in science; for example, techniques for the production and use of monoclonal antibodies have developed extremely rapidly. Immunology also deals with practical health problems such as the nature and treatment of allergies and autoimmune diseases like rheumatoid arthritis.
黄芩汤的抗须癣毛癣菌活性及作用机制研究
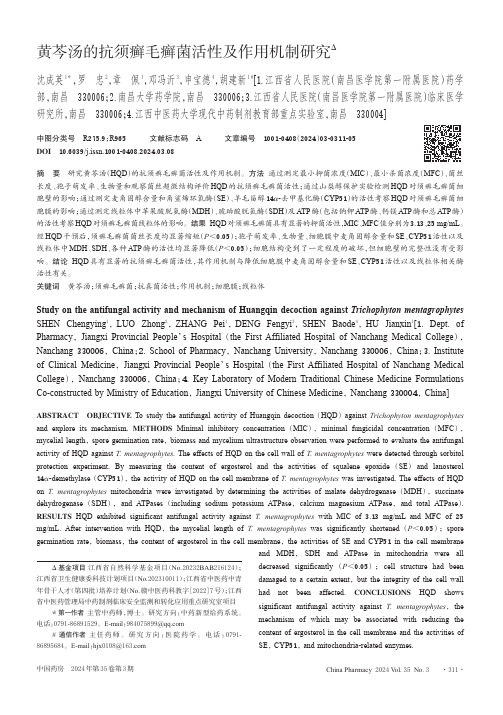
黄芩汤的抗须癣毛癣菌活性及作用机制研究Δ沈成英 1*,罗忠 2,章佩 1,邓冯沂 3,申宝德 4,胡建新 1 #[1.江西省人民医院(南昌医学院第一附属医院)药学部,南昌 330006;2.南昌大学药学院,南昌 330006;3.江西省人民医院(南昌医学院第一附属医院)临床医学研究所,南昌 330006;4.江西中医药大学现代中药制剂教育部重点实验室,南昌 330004]中图分类号 R 275.9;R 965 文献标志码 A 文章编号 1001-0408(2024)03-0311-05DOI 10.6039/j.issn.1001-0408.2024.03.08摘要 研究黄芩汤(HQD )的抗须癣毛癣菌活性及作用机制。
方法 通过测定最小抑菌浓度(MIC )、最小杀菌浓度(MFC )、菌丝长度、孢子萌发率、生物量和观察菌丝超微结构评价HQD 的抗须癣毛癣菌活性;通过山梨醇保护实验检测HQD 对须癣毛癣菌细胞壁的影响;通过测定麦角固醇含量和角鲨烯环氧酶(SE )、羊毛甾醇14α-去甲基化酶(CYP 51)的活性考察HQD 对须癣毛癣菌细胞膜的影响;通过测定线粒体中苹果酸脱氢酶(MDH )、琥珀酸脱氢酶(SDH )及ATP 酶(包括钠钾ATP 酶、钙镁ATP 酶和总ATP 酶)的活性考察HQD 对须癣毛癣菌线粒体的影响。
结果 HQD 对须癣毛癣菌具有显著的抑菌活性,MIC 、MFC 值分别为3.13、25 mg/mL 。
经HQD 干预后,须癣毛癣菌菌丝长度均显著缩短(P <0.05);孢子萌发率、生物量、细胞膜中麦角固醇含量和SE 、CYP 51活性以及线粒体中MDH 、SDH 、各种ATP 酶的活性均显著降低(P <0.05);细胞结构受到了一定程度的破坏,但细胞壁的完整性没有受影响。
结论 HQD 具有显著的抗须癣毛癣菌活性,其作用机制与降低细胞膜中麦角固醇含量和SE 、CYP 51活性以及线粒体相关酶活性有关。
leptospira interrogans ss. icterohaemorrhagiae-感染的
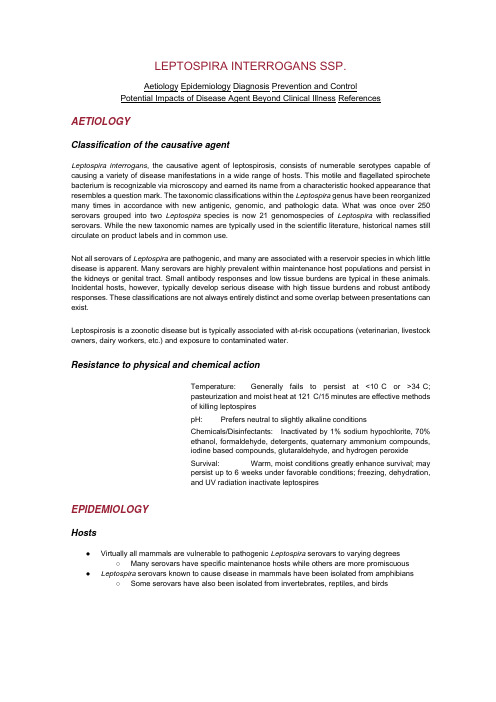
LEPTOSPIRA INTERROGANS SSP.Aetiology Epidemiology Diagnosis Prevention and ControlPotential Impacts of Disease Agent Beyond Clinical Illness References AETIOLOGYClassification of the causative agentLeptospira interrogans, the causative agent of leptospirosis, consists of numerable serotypes capable of causing a variety of disease manifestations in a wide range of hosts. This motile and flagellated spirochete bacterium is recognizable via microscopy and earned its name from a characteristic hooked appearance that resembles a question mark. The taxonomic classifications within the Leptospira genus have been reorganized many times in accordance with new antigenic, genomic, and pathologic data. What was once over 250 serovars grouped into two Leptospira species is now 21 genomospecies of Leptospira with reclassified serovars. While the new taxonomic names are typically used in the scientific literature, historical names still circulate on product labels and in common use.Not all serovars of Leptospira are pathogenic, and many are associated with a reservoir species in which little disease is apparent. Many serovars are highly prevalent within maintenance host populations and persist in the kidneys or genital tract. Small antibody responses and low tissue burdens are typical in these animals. Incidental hosts, however, typically develop serious disease with high tissue burdens and robust antibody responses. These classifications are not always entirely distinct and some overlap between presentations can exist.Leptospirosis is a zoonotic disease but is typically associated with at-risk occupations (veterinarian, livestock owners, dairy workers, etc.) and exposure to contaminated water.Resistance to physical and chemical actionTemperature: Generally fails to persist at <10°C or >34°C;pasteurization and moist heat at 121°C/15 minutes are effective methodsof killing leptospirespH: Prefers neutral to slightly alkaline conditionsChemicals/Disinfectants: Inactivated by 1% sodium hypochlorite, 70%ethanol, formaldehyde, detergents, quaternary ammonium compounds,iodine based compounds, glutaraldehyde, and hydrogen peroxideSurvival: Warm, moist conditions greatly enhance survival; maypersist up to 6 weeks under favorable conditions; freezing, dehydration,and UV radiation inactivate leptospiresEPIDEMIOLOGYHosts●Virtually all mammals are vulnerable to pathogenic Leptospira serovars to varying degrees○Many serovars have specific maintenance hosts while others are more promiscuous●Leptospira serovars known to cause disease in mammals have been isolated from amphibians○Some serovars have also been isolated from invertebrates, reptiles, and birdsProminent host-serovar associations●Armadillos (Dasypus novemcinctus, Euphractus sexcinctus) - Autumnalis, Cynopteri, Hebdomadis,Pomona●Bandicoots (Isoodon macrourus, Perameles spp.) - numerous serovars have been associated withthese species●Brazilian tapir (Tapirus terrestris) - Pomona●Canids (Canis latrans, C. familiaris, C. lupus) - Bratislava, Canicola, Grippotyphosa, Hardjo,Icterohaemorrhagiae, Pomona●Cattle (Bos taurus, Syncerus caffer) - Hardjo and others●Cervids - Bratislava, Canicola, Grippotyphosa, Hardjo, Icterohaemorrhagiae, Pomona●European hedgehog (Erinaceus europaeus)●Felids (Felis silvestris silvestris, F. silvestris catus, Lynx spp.)●Flying foxes (Pteropus spp.) - a multitude of Leptospira serovars have been identified in variousspecies of bat●Foxes (Vulpes lagopus, V. vulpes, Urocyon cinereoargenteus, Lycalopex griseus) - Bratislava,Canicola, Grippotyphosa●Giant anteater (Myrmecophaga tridactyla) - Djasiman●Horses (Equus ferus) - Bratislava●Lagomorphs (Lepus europaeus, L. timidus, Oryctolagus cuniculus) - Grippotyphosa●Marine mammals (Eubalaena australis,Trichechus manatus ) - Australis, Manaua○Sea lions (Zalophus californianus, Z. wollebaeki) and seals (Callorhinus ursinus, Phoca vitulina, Mirounga angustirostris, Arctocephalus forsteri) - Canicola, Hardjo, Pomona ○There have been multiple mass-mortality events attributed to leptospirosis in California sea lions●Marsupials - Australis, Autumnalis, Ballum, Bataviae, Celledoni, Cynopteri, Djasiman,Grippotyphosa, Hardjo, Hebdomadis, Icterohaemorrhagiae, Javanica, Mini, Panama, Pomona, Pyrogenes, Sejroe, Tarassov, Topaz●Mongooses (Herpestes auropunctatus, Mungos mungo, Paracynictis selousi) - Bratislava, Hardjo●Mustelids (Meles meles, Martes fiona, M. martes, Mustela putorius, M. nivalis, M. ermine, Lutra lutra)●Platypus (Ornithorhynchus anatinus) - Autumnalis, Hardjo, Grippotyphosa●Raccoons (Procyon itor) and skunks (Mephitis mephitis) - Bratislava, Canicola, Grippotyphosa,Hardjo, Icterohaemorrhagiae, Pomona●Rodents and insectivores - Arborea, Australis, Ballum, Bindjei, Broomi, Canicola, Celledoni,Gryppotyphosa, Icterohaemorrhagiae, Javanica, Mini, Pomona, Pyrogenes, Sejroe, Tarrasovi, Zanoni○Rats are well-appreciated hosts for serotype Icterohaemorrhagiae●Swine (Sus scrofa and other spp.) - Bratislava, Hardjo, Pomona●Vervet monkey (Cercopithecus aethiops sabaeus) - Australis, Grippotyphosa, Javanica●Multiple snake, turtle, toad, and frog species have also been identified as PCR and/or serologicallypositiveTransmission●Ingestion●Contact with mucous membranes or wet, abraded skin●Some serovars can be transmitted venerally or transplacentallySources●Urine●Contaminated soil and water●Placental fluids●Genital secretions●Milk●BloodOccurrenceMany wildlife species are reservoirs for Leptospira and subsequently maintain host-bacterium interactions that do not negatively impact the animal. Over time, selection pressures on the leptospires many change and drive reservoir species relationships to shift accordingly. Therefore, it is important to assess the epidemiology of leptospirosis on a more local level to understand transmission risks and disease impacts.Leptospira is globally enzootic, but disease is more frequently seen in warm and moist environments. This may be seasonal (temperate zones) or more constant (tropical regions). Rainfall encourages persistence of the organism in the environment. Additionally, some serotypes are much more geographically dispersed and others are found in more limited regions.For more recent, detailed information on the occurrence of this disease worldwide, see the OIE World Animal Health Information System - Wild (WAHIS-Wild) Interface [http://www.oie.int/wahis_2/public/wahidwild.php/Index].DIAGNOSISAfter invasion of mucous membranes or damaged skin, there is a 4-20 day incubation period followed by a 7-10 day period of circulation in the bloodstream. Clinical signs of acute leptospirosis depend on the tissues colonized during this period of bacteraemia, the host species, and infecting serovar. A robust antibody response follows and is associated with a declining bacteraemia. Tissues may recover slowly or not at all depending on the degree of damage. Death is possible in severe cases.Incidental hosts maintain the bacterium in renal tubules for days to weeks and shed the organism in urine. Maintenance hosts, however, maintain the bacterium in the renal tubules, genital tract, and/or eyes and shed it in urine or genital secretions for months to years after infection.Clinical diagnosisLeptospirosis is a highly variable, systemic infection and the presentation depends on the infecting serovar, the host species, and the host’s general health and immune status. Maintenance hosts typically do not develop significant clinical disease. Incidental hosts typically experience severe, acute disease secondary to bacterial toxins and inflammatory responses generated by the immune system. Initially, animals may be febrile and anorectic. They may quickly develop signs of haemorrhage and haemolytic anaemia secondary to endothelial damage such as mucosal petechiation, icterus, haemoglobinuria/haematuria, dehydration, vomiting, and colic. Acute renal injury develops rapidly and is a significant contributor to mortality. Pneumonia, meningitis, uveitis, corneal opacification, photosensitization, myalgia, and pancreatitis are also possible.Reproductive disease is often characterised by abortion/stillbirth, mummified fetuses, infertility, blood in milk, or a cessation of milk production. If not aborted, neonates infected transplacentally are typically weak. Maintenance hosts do not develop reproductive disease acutely like incidental hosts, but instead remain subclinical for weeks to months.Lesions●Renal tubular necrosis and suppurative nephritis○Pale, oedematous parenchyma +/- pitting of the serosal surface and capsular adhesions○Subcapsular haemorrhage○Inflammation initially characterised by neutrophils but becomes lymphoplasmacytic○Mixed inflammatory processes are associated with higher mortality rates●Hepatomegaly +/- necrotizing hepatitis○The liver is often friable and discolored in a lobular pattern●Pulmonary haemorrhage●Petechiae and ecchymoses on mucous membranes and internal organs●Horses may develop uveitis with conjunctivitis, corneal oedema, synechia, or cataractsDifferential diagnoses●Ocular disease○Equine recurrent uveitis○Traumatic uveitis/reflex uveitis○Infectious conjunctivitis●Kidney disease○Toxin exposure (e.g., ethylene glycol)○Infectious nephritis, pyelonephritis, glomerulonephritis○Renal tubular acidosis○Nematodes (Stephanurus dentatus, Dioctophyma renale)●Reproductive failure or compromise○Brucellosis○Bovine viral diarrhea virus (BVDV)○Porcine reproductive and respiratory syndrome (PRRS)○Q-Fever (Coxiella burnetii)○Neospora spp.○Tritrichomonas foetus○Mastitis, metritis●Liver disease, icterus, and haemolytic anaemia○Viral hepatitis○Toxin exposure (e.g., heavy metals, anticoagulant rodenticides)○Rickettsial infection○Clostridium haemolyticum, C. perfringens A○Neonatal isoerythrolysis●Bacterial septicaemiaLaboratory diagnosisSamplesFor isolation of agent●Kidney●Blood●Urine●Other grossly affected tissue such as liverSerological tests●Serum●Whole bloodProceduresIdentification of the agent●Silver-stained histopathology slides allow for direct visualization of the organism in renal tubules●Immunohistochemistry (IHC)●Bacterial culture○Because the organism is low-growing, this may take 12-26 weeks○Best available method to determine infecting serovar●Polymerase chain reaction (PCR)○Widely variable protocols○Does not provide serovar-specific resultsSerological tests●Microscopic agglutination test (MAT)○Uses live, regionally common serovars of Leptospira○Requires diagnostic laboratory to maintain live cultures of serovars○Provides quantitative titre level●Antibody capture enzyme-linked immunosorbent assay (ELISA)○Currently used for domestic canines; detects antibodies to LipL32 protein○Results are qualitative (positive/negative) and may yield false positives in the event of prior vaccination●Immunofluorescence assay (IFA)●There is not yet a consensus on what a diagnostic titre for Leptospira should be, therefore pairedacute and convalescent sera are recommended for testing●Caution should be taken when interpreting serology data; antibody titre does not always correspondwith disease stateFor more detailed information regarding laboratory diagnostic methodologies, please refer to Chapter 3.1.12 Leptospirosis in the latest edition of the OIE Manual of Diagnostic Tests and Vaccines for Terrestrial Animals.PREVENTION AND CONTROLSanitary prophylaxis●Extra precautions should be taken when cleaning areas frequented by potential Leptospira hosts.Wear gowns, shoe covers, and gloves to prevent contamination of personal clothing. Face shields are recommended to protect mucous membranes from aerosols.Medical prophylaxis●There are a variety of Leptospira vaccines available for domestic animals, including livestock○Vaccine intent may vary from prevention of infection to reduction of renal colonization and urine shedding○Read vaccine labels to determine which serovars are targeted, as immunity is believed to be serovar-specificPOTENTIAL IMPACTS OF DISEASE AGENT BEYOND CLINICAL ILLNESS Risks to public health●Leptospirosis is a zoonotic disease. Because clinical signs can be vague and maintenance hostscan be asymptomatic carriers, basic protective measures are suggested for at-risk populations (veterinarians, livestock owners, dairy workers, etc.): protect eyes with safety glasses, wear gloves especially if there are openings in the skin, thoroughly wash hands after interacting with animals of unknown status and before consuming food or water, etc.○Pregnant individuals within at-risk populations are particularly advised to utilise protective measures.●Many domestic animal species, including dogs, horses, and livestock, are susceptible to leptospirosisand could potentially transmit it to humans. Individuals should speak with local veterinarians to determine risk and appropriate prevention strategies, including animal vaccines.Risks to agriculture●If livestock or working animals (horses, dogs) develop clinical disease due to Leptospira, decreasedthrift and reproductive compromise can significantly impact production. Working animals may not beable to do their jobs as efficiently, and livestock may demand increased resources for treatment while producing less.REFERENCES AND OTHER INFORMATION●Atherstone, C., Picozzi, K., & Kalema-Zikusoka, G. (2014). Seroprevalence of Leptospira hardjo incattle and African buffalos in southwestern Uganda. The American Journal of Tropical Medicine and Hygiene, 90(2), 288–290.●Ayral, F., Djelouadji, Z., Raton, V., Zilber, A. L., Gasqui, P., et al. (2016). Hedgehogs and mustelidspecies: major carriers of pathogenic Leptospira, a survey in 28 animal species in France (20122015). PloS One, 11(9), e0162549.●Biscola, N. P., Fornazari, F., Saad, E., Richini-Pereira, V. B., Campagner, M. V., et al. (2011).Serological investigation and PCR in detection of pathogenic leptospires in snakes. Pesquisa Veterinária Brasileira, 31(9), 806-811.●Buhnerkempe, M. G., Pragger, K. C., Strelloff, C. C., Greig, D. J., Laake, J. L., et al. (2017). Detectingsignals of chronic shedding to explain pathogen persistence: Leptospira interrogans in California sea lions. Journal of Animal Ecology, 86(3), 460-472.●Denkinger, J., Guevara, N., Ayala, S., Murillo, J. C., Hirschfeld, M., et al. (2017). Pup mortality andevidence for pathogen exposure in Galapagos sea lions (Zalophus wollebaeki) on San Cristobal Island, Galapagos, Ecuador. Journal of Wildlife Disease, 53(3), 491-498.●Gravekamp, C., Korver, H., Montgomery, J., Everard, C. O. R., Carrington, D., et al. (1991).Leptospires isolated from toads and frogs on the island of Barbados. Zentralblatt für Bakteriologie, 275(3), 403-411.●Jobbins, S. E., Sanderson, S. E., & Alexander, K. A. (2014). Leptospira interrogans at the human-wildlife interface in northern Botswana: a newly identified public health threat. Zoonoses and Public Health, 61, 113-123.●Karesh, W. B., Hart, J. A., Hart, T. B., House, C., Torres, A., et al. (1995). Health evaluation of fivesympatric duiker species (Cephalophus spp). Journal of Zoo and Wildlife Medicine, 26(4), 485-502.●Leighton, F. A. & Kuiken, T. (2001). Leptospirosis. In E. S. Williams and I. K. Barker (Eds.), InfectiousDiseases of Wild Mammals (3rd ed., pp. 498-502). Iowa State Press.●Loffler, G. S., Rago, V., Martinez, M., Uhart, M., Florin-Christensen, M., et al. (2015). Isolation of aseawater tolerant Leptospira spp. from a southern right whale (Eubalaena australis). PLoS One, 10(12), e0144974.●Lunn, K. F. (2018). Overview of leptospirosis. Merck Veterinary Manual.Accessed 2020:https:///generalized-conditions/leptospirosis/overview-of-leptospirosis?query=leptospira●Pedersen, K., Anderson, T. D., Maison, R. M., Wiscomb, G. W., Pipas, M. J., et al. (2018). Leptospiraantibodies detected in wildlife in the USA and the US Virgin Islands. Journal of Wildlife Diseases, 54(3), 450-459.●Spickler, A. R. & Leedom, L. K. R. (2013). Leptospirosis. Accessed 2020:/Factsheets/pdfs/leptospirosis.pdf●The World Organisation for Animal Health (2018). Leptospirosis. Accessed 2020:https://www.oie.int/fileadmin/Home/eng/Health_standards/tahm/3.01.12_LEPTO.pdf●Vieira, A. S., Pinto, P. S, & Lillenbaum, W. (2018). A systematic review of leptospirosis on wildanimals in Latin America. Tropical Animal Health and Production, 50(2), 229-238.●Wildlife Health Australia (2018). Leptospira infection in Australian mammals. Accessed 2020:https://.au/FactSheets.aspx●Wildlife Health Australia (2011). Leptospira infection in Australian seals. Accessed 2020:https://.au/FactSheets.aspx** *。
211188541_基于体外发酵的双孢菇膳食纤维及双孢菇粉对人体肠道菌群的调节作用
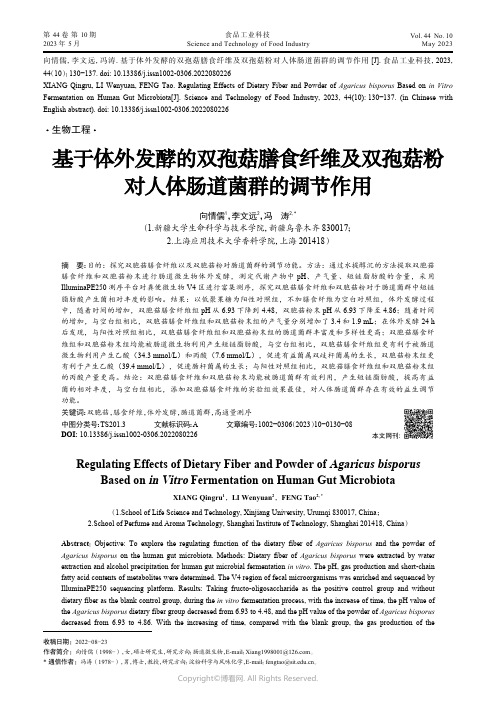
向情儒,李文远,冯涛. 基于体外发酵的双孢菇膳食纤维及双孢菇粉对人体肠道菌群的调节作用[J]. 食品工业科技,2023,44(10):130−137. doi: 10.13386/j.issn1002-0306.2022080226XIANG Qingru, LI Wenyuan, FENG Tao. Regulating Effects of Dietary Fiber and Powder of Agaricus bisporus Based on in Vitro Fermentation on Human Gut Microbiota[J]. Science and Technology of Food Industry, 2023, 44(10): 130−137. (in Chinese with English abstract). doi: 10.13386/j.issn1002-0306.2022080226· 生物工程 ·基于体外发酵的双孢菇膳食纤维及双孢菇粉对人体肠道菌群的调节作用向情儒1,李文远2,冯 涛2,*(1.新疆大学生命科学与技术学院,新疆乌鲁木齐 830017;2.上海应用技术大学香料学院,上海 201418)摘 要:目的:探究双胞菇膳食纤维以及双胞菇粉对肠道菌群的调节功能。
方法:通过水提醇沉的方法提取双胞菇膳食纤维和双胞菇粉末进行肠道微生物体外发酵,测定代谢产物中pH 、产气量、短链脂肪酸的含量,采用IlluminaPE250测序平台对粪便微生物V4区进行富集测序,探究双胞菇膳食纤维和双胞菇粉对于肠道菌群中短链脂肪酸产生菌相对丰度的影响。
结果:以低聚果糖为阳性对照组,不加膳食纤维为空白对照组,体外发酵过程中,随着时间的增加,双胞菇膳食纤维组pH 从6.93下降到4.48,双胞菇粉末pH 从6.93下降至4.86;随着时间的增加,与空白组相比,双胞菇膳食纤维组和双胞菇粉末组的产气量分别增加了3.4和1.9 mL ;在体外发酵24 h 后发现,与阳性对照组相比,双胞菇膳食纤维组和双胞菇粉末组的肠道菌群丰富度和多样性更高;双胞菇膳食纤维组和双胞菇粉末组均能被肠道微生物利用产生短链脂肪酸,与空白组相比,双胞菇膳食纤维组更有利于被肠道微生物利用产生乙酸(34.3 mmol/L )和丙酸(7.6 mmol/L ),促进有益菌属双歧杆菌属的生长,双胞菇粉末组更有利于产生乙酸(39.4 mmol/L ),促进肠杆菌属的生长;与阳性对照组相比,双胞菇膳食纤维组和双胞菇粉末组的丙酸产量更高。
肠道微生物的英语单词

肠道微生物的英语单词The Complex World of Gut Microbiota.The gut microbiota, often referred to as the "microbiome" or the "intestinal flora," refers to the vast community of microorganisms that reside within the human gastrointestinal tract. This intricate ecosystem plays a crucial role in maintaining our overall health and well-being. The gut microbiota is composed of a diverse range of bacteria, fungi, viruses, and other microorganisms that coexist in a delicate balance.The human body is estimated to contain trillions of microbial cells, outnumbering the human cells by a ratio of 10 to 1. The majority of these microbial cells reside in the gastrointestinal tract, particularly in the colon. The gut microbiota performs various vital functions, including digesting food, synthesizing vitamins, and regulating the immune system.Functions of the Gut Microbiota.Digestion and Nutrition: The gut microbiota aids in the breakdown of dietary fiber and other complex carbohydrates, releasing short-chain fatty acids (SCFAs) such as acetate, propionate, and butyrate. These SCFAs serve as a source of energy for the host and have been linked to various health benefits, including improved insulin sensitivity and reduced inflammation.Immune System Regulation: The gut microbiota plays a crucial role in shaping and regulating the immune system. It stimulates the development of immune cells and helps maintain a balanced immune response, protecting against both infectious diseases and autoimmune conditions.Barrier Function: The gut microbiota contributes to maintaining the integrity of the gut barrier, which prevents harmful bacteria and toxins from leaking into the bloodstream. A healthy gut microbiota supports tight junctions between gut cells, ensuring a strong barrier against pathogens.Brain-Gut Axis: The gut microbiota also interacts with the brain through the gut-brain axis, influencing mood, cognition, and behavior. This axis involves a complex communication network between the gastrointestinal tract and the central nervous system, which is believed to play a role in conditions like depression, anxiety, and autism.Importance of Gut Microbiota Balance.Disruptions to the gut microbiota, known as "dysbiosis," can lead to various health issues. Changes in the composition of the microbiota can be triggered by various factors, including diet, antibiotics, stress, and chronic illnesses.Diet: The composition of the gut microbiota is significantly influenced by the diet. A diet rich in fiber and diverse in plant-based foods promotes the growth of beneficial bacteria, while a diet high in processed foods and low in fiber can lead to a decrease in microbial diversity and an increase in harmful bacteria.Antibiotics: The use of antibiotics can have a profound impact on the gut microbiota, killing off both harmful and beneficial bacteria. This can lead to a temporary imbalance in the microbiota, allowing opportunistic pathogens to proliferate.Stress: Chronic stress has been shown to alter the gut microbiota composition, leading to an increase in inflammatory markers and a decrease in beneficial bacteria.Chronic Illnesses: Conditions like inflammatory bowel disease (IBD), irritable bowel syndrome (IBS), and obesity have been linked to alterations in the gut microbiota. These changes can contribute to the development and progression of these diseases.Modulating the Gut Microbiota.Given the crucial role of the gut microbiota in maintaining health, there has been increasing interest in modulating its composition through various strategies.Probiotics: Probiotics are live microorganisms that, when administered in adequate amounts, confer a health benefit on the host. They are commonly found in yogurt, fermented foods, and dietary supplements. Probiotics can help restore balance to the gut microbiota, improving digestive health and immune function.Prebiotics: Prebiotics are dietary fibers that promote the growth and activity of beneficial bacteria in the gut. By providing food for the probiotic bacteria, prebiotics can help support a healthy gut microbiota.Dietary Changes: Incorporating a diet rich in fiber, fruits, vegetables, and whole grains can promote the growth of beneficial bacteria and maintain gut microbiota diversity.Conclusion.The gut microbiota plays a pivotal role in maintaining human health and well-being. Its intricate balance ofmicroorganisms is essential for digestion, immune system regulation, and overall physiological functions. Disruptions to this balance can lead to various health issues, emphasizing the importance of maintaining a healthy gut microbiota through diet, lifestyle choices, and probiotic supplementation. As research in this field continues to evolve, so does our understanding of the crucial role the gut microbiota plays in our lives.。
- 1、下载文档前请自行甄别文档内容的完整性,平台不提供额外的编辑、内容补充、找答案等附加服务。
- 2、"仅部分预览"的文档,不可在线预览部分如存在完整性等问题,可反馈申请退款(可完整预览的文档不适用该条件!)。
- 3、如文档侵犯您的权益,请联系客服反馈,我们会尽快为您处理(人工客服工作时间:9:00-18:30)。
Ergosterol and microbial biomass in the rhizosphere of grasslandsoilsRainer Georg Joergensen*Department of Soil Biology,University of Kassel,Nordbahnhofstr.1a,37213Witzenhausen,GermanyAccepted2October1999AbstractFungal and microbial biomass were determined by ergosterol and fumigation±extraction,respectively,in bulk grassland soil (<2mm),rhizosphere and root material.The aim was to quantify the contribution of these three microbial fractions to the total soil microbial biomass and to soil organic matter.In the bulk soil,the average concentration was3.37m g gÀ1for ergosterol,860m g gÀ1for microbial biomass C,and30.4mg gÀ1for organic C.In the rhizosphere material,the corresponding concentrations exceeded those of the bulk soil by80,80and50%,respectively.The large average ergosterol concentration of 74.2mg gÀ1root revealed a strong fungal colonisation of the root material.About75%of the total ergosterol was found in the bulk soil fraction,11%in the rhizosphere and14%in the root material.In one soil nearly half of total ergosterol was found in the root material.However,the average ergosterol-to-biomass ratio in the root material was less than a third of the bulk soil or the rhizosphere soil,indicating that approximately two-thirds of CHCl3-labile C are presumably root-derived.72000Elsevier Science Ltd.All rights reserved.Keywords:Ergosterol;Fungal biomass;Microbial biomass C;Rhizosphere;Fumigation extraction;CHCl3-labile root material1.IntroductionThe development and use of new methods more than20yr ago for quantifying microbial biomass in soil such as fumigation±incubation(Jenkinson and Powlson,1976)and substrate-induced respiration (Anderson and Domsch,1978)was one of the major advances in soil microbial ecology.The next important step was the development of the fumigation±extraction method,giving the possibility of measuring microbial biomass in the presence of actively decomposing sub-strates(Vance et al.,1987;Ocio and Brookes,1990). However,the fumigation±extraction method su ers from the problem that results are a ected by the pre-sence of intact living roots,leading to overestimations of the actual microbial biomass(Mueller et al.,1992).It was shown repeatedly that organic carbon is ren-dered extractable by CHCl3fumigation(Martin and Foster,1985;Sparling et al.,1985).Consequently,soil samples freshly taken in the®eld need a pre-treatment before the microbial biomass can be measured.Soils must be sieved to remove roots or preincubated to condition the micro¯ora(Jenkinson,1988).However, this pre-treatment is always a point of critical discus-sion,especially if the microbial biomass is measured in ®eld experiments(Joergensen et al.,1994;Ohlfs and Scherer,1996).An unknown amount of microbial biomass in the rhizosphere soil attached to the roots remains on the sieve,i.e.soil material with much higher microbial bio-mass and carbon availability than the bulk soil(Nor-ton et al.,1990;Cheng et al.,1996).For this reason, the risk of underestimating microbial biomass is es-pecially large in densely rooted grassland soils.Never-theless,it is possible to detect at least the fungal part of the microbial biomass by measuring the cell-mem-Soil Biology&Biochemistry32(2000)647±6520038-0717/00/$-see front matter72000Elsevier Science Ltd.All rights reserved.PII:S0038-0717(99)00191-/locate/soilbio*Tel.:+49-5542-981-591;fax:+49-5542-981-596.E-mail address:joerge@wiz.uni-kassel.de(R.G.Joergensen).brane component ergosterol,which is a speci®c bio-marker for fungi.In contrast to the methods men-tioned above,ergosterol is not a ected by the presence of roots and can be easily detected by a variety of methods in solid substrates(West et al.,1987;Padgett and Posey,1993;Stahl and Parkin,1996).For these reasons,ergosterol has been successfully used to di er-entiate between fungal and plant tissue(Seitz et al., 1977;Newell,1992,1996;Nylund and Wallander, 1992;Wallander et al.,1997).However,the conversion of ergosterol data to fungal biomass is hampered by the large variability of the ergosterol concentration in di erent fungal species as reviewed by Djajakirana et al.(1996).The conversion of carbon rendered extracta-ble by CHCl3fumigation is less variable(Joergensen, 1996).Consequently,fumigation±extraction and ergosterol data were combined in the present study in an attempt to quantify the contribution of rhizosphere microor-ganisms to the total soil microbial biomass.For this purpose,soils were sampled at several grassland sites and divided into the sample fractions bulk soil(<2 mm),rhizosphere and root material by combining ordinary sieving of®eld moist soil with the wet-sieving procedure of Mueller et al.(1992).2.Materials and methods2.1.Soil sampling and preparationSoils were sampled on13Mondays from October to January in periods without frost at grassland sites around Go ttingen,south lower Saxony,Germany. Four125-cm3cores were sampled at each site for the determination of bulk density.Samples of between4 and5kg were taken to a depth of10cm using a spade.The samples were transferred to the laboratory and divided into four parts.First of all,each quarter was weighed and then it was sieved(2mm)to remove roots.The sieved fraction of the sample was called the bulk soil.The soil attached to the roots,called rhizo-sphere material,was washed away on a2mm sieve and collected in a bucket.The soil slurry in the bucket was transferred in500-ml polyethylene bottles and cen-trifuged for20min at500g to separate rhizosphere soil and water.Then,subsamples were taken from the wet rhizosphere material for fumigation±extraction and ergosterol measurement.The remaining rhizosphere material was dried at1058C for the determination of dry weight.From the cleaned and thus soil-free root material,also moist subsamples for fumigation±extrac-tion and ergosterol were taken and the remaining root material was dried at1058C for the determination of dry weight and then ashed at7008C for the determi-nation of organic matter.All samples were analysed separately within4d for ergosterol and microbial bio-mass.2.2.AnalysisMicrobial biomass C was measured by fumigation extraction(Vance et al.,1987).Moist bulk soil(20g) was extracted for45min by oscillating shaking at250 rev minÀ1with80ml10mM CaCl2for the fumigated and for the nonfumigated treatment and®ltered through a folded paper®lter(Schleicher&Schuell,595 1/2).Moist rhizosphere material(4g)was extracted for45min by oscillating shaking at250rev minÀ1 with16ml10mM CaCl2for the fumigated and for the nonfumigated treatment and centrifuged at2000g. The organic C concentration in®ltered and centrifuged extracts is not signi®cantly di erent.However,®l-tration is less laborious and centrifugation gives larger amounts of extract which is important using small samples sizes.Moist root material(2g)was extracted for45min by oscillating shaking at250rev minÀ1 with30ml10mM CaCl2for the fumigated and for the nonfumigated treatment and®ltered through a folded paper®lter(Schleicher&Schuell,5951/2).Or-ganic C in the extracts was measured by catalytic oven oxidation at8008C using a Maihak Tocor2automatic analyser.Soil microbial biomass C was estimated from the relationship:biomass C E C a k EC,where E C is(or-ganic C extracted from fumigated soil)minus(organic C extracted from nonfumigated soil)and k EC 0X45 (Joergensen,1995).Ergosterol was measured according to Djajakirana et al.(1996).Moist soil of1g dry weight was extracted with100ml ethanol for30min by oscillating shaking at250rev minÀ1.Ergosterol was measured by reversed-phase HPLC analysis at258C using a column of12.5cm Spherisorb ODS II S5with a mobile phase of97vol%methanol/3vol%water and detection at282nm.Subsamples of dried bulk soil and rhizosphere ma-terial were homogenised in a ball mill.Total C was determined after dry combustion at12008C using an elemental analyser(Carlo Erba).Organic C was measured as total C minus carbonate C which was measured gas-volumetrically after the addition of4M anic C in the root material was from the for-mula:0.5555Âash-free organic matter(Joergensen, 1991).Ash concentration of root material was gravi-metrically determined after combustion of a2g air-dried subsample at7008C for5h.Soil pH was measured in water using a soil-to-water ratio of1-to-2.5.Clay content was determined by sedimentation after pre-treatment with H2O2,Na-citrate and Na2S2O4to remove organic matter and iron oxides (Schlichting et al.,1995).All statistical calculations were performed by StatView(Version5,SAS Insti-tute).The®gures presented in the tables are arithmeticR.G.Joergensen/Soil Biology&Biochemistry32(2000)647±652 648means and are given on an oven-dry basis(1058C,24 h).3.ResultsSoil pH ranged from4.3to7.9,bulk density from 0.99to1.54kg dmÀ3and clay content from8to27% re¯ecting the di erent parent materials(Table1).The sieved bulk soil(<2mm)represented on average 91.9%of the total sample dry weight sum of bulk soil,rhizosphere and root material),the rhizosphere material7.4%and the root material only 0.7%,ranging from0.3to2.1%.The average concen-trations were0.86mg gÀ1for microbial biomass C (Table2),3.37m g gÀ1for ergosterol(Table3)and30.4 mg gÀ1for organic C(Table2)in the bulk soil.There, microbial biomass C showed signi®cant correlations(P <0.01)with the ergosterol r 0X80 and organic C r 0X82).In the rhizosphere material,the correspond-ing concentrations exceeded those of the bulk soil by 80,80and50%,respectively.This means that the bio-mass C-to-organic C ratios were usually larger in the rhizosphere material than in the bulk soil(Table2) and that the ergosterol-to-biomass C ratios were almost identical in these two sample fractions(Table3). In the root material,the average ergosterol concen-tration was74.2mg gÀ1dry weight,but the average ergosterol-to-biomass C ratio was only0.13%,less than a third of that in the bulk soil or in the rhizo-sphere material(Table3).The ergosterol-to-biomass C ratios of bulk soil and rhizosphere material were highly signi®cantly correlated r 0X83,P<0.01)indi-cating a stable relationship between these two mi-crobial indices within a soil.About75%of total ergosterol was found in the bulk soil fraction,11%in the rhizosphere and14%in the root material(Table3). In soil No.7nearly half of the total sum was found in the root material,exceeding the value of the bulk soil.4.DiscussionThe large ergosterol concentrations in the soil-free root material revealed a strong fungal colonisation. Similar concentrations of52and72m g ergosterol gÀ1 dry material were determined in the roots of Zea mays and Trifolium alexandrinum,respectively,infected with the vesicular±arbuscular endomycorrhizal fungus Glo-mus intraradices after80d of growth(Frey et al., 1992).In the bulk soil,the concentrations of ergosterol and microbial biomass C are in the range of those reported for grassland soils,i.e.relatively low in com-parison to that of arable or forest soils(Djajakirana et al.,1996).However,the extremely low ergosterol-to-biomass C ratio in the root material indicates that a signi®cant percentage of CHCl3-labile C is probably root-derived,although the amount of CHCl3-labile C is much lower than those reported by Mueller et al. (1992)who extracted young intact wheat roots.The ergosterol-to-biomass C ratios in all soils were almost identical in soil and rhizosphere material. Assuming that the same is true for root colonising microorganisms,microbial derived CHCl3-labile C in the root fraction can be calculated as follows:D C a A B a2Â100,Table1Soil classi®cation according to the Food and Agricultural Organization(FAO)system,soil properties,dominating grass species,distribution of the sample on the fractions sieved bulk soil(<2mm),rhizosphere material,and root materialNo.Soil pH-H2O Bulk density(kg dmÀ3)Clay(%soil)Soil(%sample b)Rhizosphere(%sample)Root(%sample)Dominating grass species(1)Gleyic Chernozem7.90.9924.496.7 2.70.6Arrhenatherum elatius,Poa trivialis(2)Orthic Luvisol7.8 1.5419.894.4 5.30.3Festuca rubra,P.trivialis(3)Orthic Luvisol7.7 1.3622.793.6 5.90.6P.trivialis,F.pratensis(4)Orthic Luvisol7.7 1.4818.790.88.60.7 A.elatius,P.trivialis(5)Orthic Luvisol 6.5 1.2124.992.47.30.3Holcus lanatus,Phleum pratense(6)Orthic Luvisol7.7 1.2019.086.312.7 1.0Dactylis glomerata,P.trivialis(7)Dystric Luvisol 5.1 1.4616.686.311.7 2.1Agrostis tenuis,F.rubra(8)Dystric Cambisol 5.1 1.319.796.8 2.40.8 A.elatius,P.pratensis(9)Dystric Cambisol 4.3 1.008.294.6 4.80.6Festuca altissima,natus(10)Eutric Cambisol 6.5 1.18 5.790.88.50.7 D.glomerata,A.tenuis(11)Eutric Fluvisol7.4 1.3612.895.1 3.90.9Alopecurus pratensis,P.trivialis(12)Eutric Fluvisol7.8 1.1216.391.77.70.6P.trivialis,D.glomerata(13)Eutric Fluvisol7.2 1.2142.784.814.70.5 A.elatius,Lolium perenneMean 6.8 1.2691.97.40.7LSD a0.10.15 6.7 6.40.5b Total sample weight=sum of bulk soil,rhizosphere and root material.a LSD=least signi®cant di erence.Minimum and maximum values are in bold.R.G.Joergensen/Soil Biology&Biochemistry32(2000)647±652649where A is the ergosterol-to-biomass C ratio in the bulk soil,B the ergosterol-to-biomass C ratio in the rhizosphere material,C the ergosterol concentration in the root material and D the true microbial biomass concentration in the root material.According to this calculation,on average 33%of CHCl 3-labile C was microbially derived and 66%was non-microbial,root-derived organic C (Table 4).The corrected microbial biomass C concentrations in the root material were still very large compared to the grassland soils,ranging from 7.1to 36.7mg g À1,but were similar to those values measured in fresh leaf litter of forest ¯oors (Joergensen and Scheu,1999).This is still less than the fungal biomass concentration of between 13and 26%estimated by Salmanowicsz and Nylund (1988)in bulk root material from Scots pine infected with the ecto-mycorrhizal fungus Laccaria laccata ,converting ergos-terol data to fungal biomass.The basic assumption of my work is that the ergos-terol-to-biomass C ratio is identical throughout the di erent soil compartments.This assumption might be wrong.Root colonising microorganisms may have a lower ergosterol-to-biomass C ratio because bacteria contribute a larger percentage of biomass to rhizo-Table 2Biomass C,organic C and the biomass C-to-organic C ratio in the sample fractions bulk soil (<2mm),rhizosphere material,and root material No.Biomass C (mg g À1)Organic C (mg g À1)Biomass C-to-organic C (%)SoilRhizosphere Root Soil Rhizosphere Soil Rhizosphere Root 1 1.71 2.0363.644.251.1 3.9 3.9 4.72 1.04 1.96109.734.045.8 3.1 4.3 6.63 1.19 1.9549.434.137.1 3.5 5.3 2.340.87 1.1881.826.531.1 3.3 3.3 4.350.58 1.0879.822.234.1 2.6 3.28.260.70 1.38142.530.353.4 2.3 2.612.270.43 1.1963.516.619.5 2.6 6.17.280.51 1.4895.618.427.7 2.8 3.3 3.790.56 1.9540.939.396.8 1.4 2.0 3.4100.440.8138.613.727.1 3.2 3.0 2.6110.79 1.6218.735.043.7 2.3 3.7 1.1120.80 1.3973.631.336.4 2.5 3.8 6.913 1.58 2.1091.549.858.0 3.2 3.67.6Mean 0.86 1.5573.030.443.2 2.8 3.8 5.4LSD a0.060.5021.84.55.00.71.21.1aLSD=least signi®cant di erence.Minimum and maximum values are in bold.Table 3Ergosterol in m g g À1soil,in %of the total sum and the ergosterol-to-biomass C ratio in the sample fractions bulk soil (<2mm),rhizosphere material,and root material No.Ergosterol (m g g À1)Ergosterol (%sum)Ergosterol-to-biomass C (%)SoilRhizosphere Root Soil Rhizosphere Root Soil Rhizosphere Root (1) 6.888.9666.591.3 3.3 5.50.400.440.11(2) 2.44 6.9863.081.213.0 5.80.240.360.06(3) 5.327.5799.183.07.49.60.450.390.20(4) 4.47 5.9075.580.110.09.90.520.500.09(5) 1.57 2.3323.386.110.1 3.80.270.220.03(6) 3.24 6.8834.369.621.78.70.460.500.02(7) 1.99 2.8785.345.08.846.20.470.240.13(8) 2.49 5.2281.076.0 4.020.00.480.350.09(9) 3.3712.9895.572.814.213.00.600.660.23(10) 2.98 5.55124.167.811.820.30.680.690.32(11) 2.05 3.7234.380.8 6.113.10.260.230.18(12) 2.23 3.6378.473.610.116.30.280.260.11(13) 4.72 5.68104.574.115.510.30.300.270.11Mean 3.37 6.0274.275.510.514.00.420.390.13LSD a0.681.8922.215.59.97.20.130.130.18aLSD=least signi®cant di erence.Minimum and maximum values are in bold.R.G.Joergensen /Soil Biology &Biochemistry 32(2000)647±652650sphere organisms than do fungi(Vancura and Kunc, 1977).Another important component of rhizosphere organisms are mycorrhizal fungi which in grassland soil consist almost exclusively of the vesicular±arbuscu-lar type(Frey et al.,1994).They estimated an ergos-terol concentration of0.2mg gÀ1dry weight in living vesicular±arbuscular mycorrhizal mycelia,10times less than in di erent ectomycorrhizal fungi(Salmanowicsz and Nylund,1988).However,Frey et al.(1994) measured roughly®ve times smaller ergosterol concen-trations in root material than in another experiment published earlier(Frey et al.,1992).Other ergosterol concentrations in vesicular±arbuscular mycorrhizal fungi have rarely been reported and are in most cases hampered by an insu cient sample preparation such as drying(Beilby,1980;Schmitz et al.,1991).More work needs to be done to identify the origin of the ergosterol found in roots and in the rhizosphere of grassland soils.However,the combination of fumi-gation extraction and ergosterol determination clearly reveals that rhizosphere and root colonising fungi and other microorganisms contribute a signi®cant percen-tage to the total microbial biomass of grassland eco-systems,an aspect which should not be neglected in the future.AcknowledgementsThis study was carried out at the Institute of Soil Science in Go ttingen.I would like to thank Ingrid Ostermeyer,Karin Schmidt and especially Ulrike Hill for their help with the analyses.ReferencesAnderson,J.P.E.,Domsch,K.H.,1978.A physiological method for quantitative measurement of microbial biomass in soils.Soil Biology&Biochemistry10,519±525.Beilby,J.P.,1980.Fatty acid and sterol composition of ungermi-nated spores of the vesicular arbuscular mycorrhizal fungus Acaulospora laevis.Lipids15,949±952.Cheng,W.,Zhang,Q.,Coleman,D.C.,Carroll,C.R.,Ho man,C.A.,1996.Is available carbon limiting microbial respiration inthe rhizosphere?Soil Biology&Biochemistry28,1283±1288. Djajakirana,G.,Joergensen,R.G.,Meyer,B.,1996.Ergosterol and microbial biomass relationship in soil.Biology&Fertility of Soils 22,299±304.Frey,B.,Buser,H.-R.,Schu epp,H.,1992.Identi®cation of ergos-terol in vesicular±arbuscular mycorrhizae.Biology&Fertility of Soils13,229±234.Frey,B.,Vilarino,A.,Schu epp,H.,Arines,J.,1994.Chitin and ergosterol content of extraradical and intraradical mycelium of the vesicular±arbuscular mycorrhizal fungus Glomus intraradices.Soil Biology&Biochemistry26,711±717.Jenkinson,D.S.1988.The determination of microbial biomass car-bon and nitrogen in soil.In:Wilson,J.R.(Ed.),Advances in Nitrogen Cycling in Agricultural Ecosystems.CAB International, Wallingford,pp.368±386.Jenkinson,D.S.,Powlson,D.S.,1976.The e ects of biocidal treat-ments on metabolism in soil.V.A method for measuring soil bio-mass.Soil Biology&Biochemistry8,209±213. Joergensen,R.G.,anic matter and element dynamics of the litter layer on a forest Rendzina under beech.Biology&Fertility of Soils11,163±169.Joergensen,R.G.,1995.The fumigation extraction method to esti-mate soil microbial biomass:extraction with0.01M CaCl2.Agribiological Research48,319±324.Joergensen,R.G.,1996.The fumigation extraction method to esti-mate soil microbial biomass:calibration of the k EC-factor.Soil Biology&Biochemistry28,25±31.Joergensen,R.G.,Scheu,S.,1999.Depth gradients of microbial and chemical properties in moder soils under beech and spruce.Pedobiologia43,134±144.Joergensen,R.G.,Meyer,B.,Mueller,T.,1994.Time-course of the soil microbial biomass under wheat:a1-year®eld study.Soil Biology&Biochemistry26,987±994.Table4Biomass C of root colonising microorganisms and root-derived CHCl3-labile C aNo.Root colonising microbial biomass C(m g gÀ1soil)Root-derived CHCl3-labile C(%E C b)(1)15.775(2)21.381(3)23.852(4)14.882(5)9.688(6)7.195(7)24.062(8)19.380(9)15.163(10)18.253(11)14.025(12)29.061(13)36.760Mean19.167a Minimum and maximum values are in bold.b E C=(organic C extracted from fumigated soil)À(organic C extracted from nonfumigated soil).R.G.Joergensen/Soil Biology&Biochemistry32(2000)647±652651Martin,J.L.,Foster,R.C.,1985.A model system for studying the biochemistry and biology of the root soil interface.Soil Biology &Biochemistry17,261±269.Mueller,T.,Joergensen,R.G.,Meyer,B.,1992.Estimation of soil microbial biomass C in the presence of fresh roots by fumi-gation±extraction.Soil Biology&Biochemistry24,179±181. Newell,S.Y.1992.Estimating fungal biomass and productivity in decomposing litter.In:Carroll,G.C.,Wicklow,D.T.(Eds.),The Fungal Community:its Organization and Role in the Ecosystem, 2nd ed.Marcel Dekker,New York,pp.521±561.Newell,S.Y.,1996.Established and potential impacts of eukaryotic mycelial decomposers in marine/terrestrial ecotones.Journal of Experimental Marine Biology and Ecology200,187±206. Norton,J.M.,Smith,J.L.,Firestone,M.K.,1990.Carbon¯ow in the rhizosphere of ponderosa pine seedlings.Soil Biology& Biochemistry22,999±1000.Nylund,J.E.,Wallander,H.,1992.Ergosterol analysis as a mean of quantifying mycorrhizal biomass.Methods in Microbiology24, 77±88.Ocio,J.A.,Brookes,P.C.,1990.An evaluation of methods for measuring the microbial biomass in soils following recent ad-ditions of wheat straw and the characterization of the biomass that develops.Soil Biology&Biochemistry22,685±694. Ohlfs,H.-W.,Scherer,H.W.,1996.Estimating microbial biomass N in soils with and without living roots:limitations of a pre-extrac-tion step.Biology&Fertility of Soils21,314±318.Padgett,D.E.,Posey,M.H.,1993.An evaluation of the e ciencies of several ergosterol extraction techniques.Mycological Research 97,1476±1480.Salmanowicsz,B.,Nylund,J.-E.,1988.High performance liquid chromatography determination of ergosterol as a measure ofectomycorrhiza infection of Scots pine.European Journal of Forest Pathology18,291±298.Schlichting, E.,Blume,H.-P.,Stahr,K.,1995.Bodenkundliches Praktikum,2nd ed.Blackwell,Berlin.Schmitz,O.,Danneberg,G.,Hundeshagen,B.,Klinger,A.,Bothe,H.,1991.Quanti®cation of vesicular±arbuscular mycorrhiza bybiochemical parameters.Journal of Plant Physiology139,106±114.Seitz,L.M.,Mohr,H.E.,Burroughs,R.,Sauer, D.B.,1977.Ergosterol as an indicator of fungal invasions in grains.Cereal Chemistry54,1207±1217.Sparling,G.P.,West,A.W.,Whale,K.N.,1985.Interference from plant roots in the estimation of soil microbial ATP,C,N and P.Soil Biology&Biochemistry17,275±278.Stahl,P.D.,Parkin,T.B.,1996.Relationship of soil ergosterol con-centration and fungal biomass.Soil Biology&Biochemistry28, 847±855.Vance,E.D.,Brookes,P.C.,Jenkinson,D.S.,1987.An extraction method for measuring soil microbial biomass C.Soil Biology& Biochemistry19,703±707.Vancura,V.,Kunc,F.,1977.The e ect of streptomycin and acti-dione on respiration in the rhizosphere and non-rhizosphere soil.Zentralblatt fuer Bakteriologie,Abteilung II132,472±478. Wallander,H.,Massicotte,H.B.,Nylund,J.-E.,1997.Seasonal vari-ation in protein,ergosterol and chitin in®ve morphotypes of Pinus sylvestris L.ectomycorrhizae in a mature Swedish forest.Soil Biology&Biochemistry29,45±53.West,A.W.,Grant,W.D.,Sparling,G.P.,e of ergosterol, diamino-pimelic acid and glucosamine content of soils to monitor changes in microbial populations.Soil Biology&Biochemistry 19,607±612.R.G.Joergensen/Soil Biology&Biochemistry32(2000)647±652 652。