内源性大马素系统综述
内源性大麻素系统用于骨质疏松症预防与治疗的研究进展

Tianjin Med J,September 2023,Vol.51No.9内源性大麻素系统用于骨质疏松症预防与治疗的研究进展王蕾1,杨涛1,耿立成1,孙天威2△摘要:骨质疏松症(OP )是一种以骨量减少和骨组织微结构改变为特征的全身性骨骼疾病,其发病率呈逐年上升趋势,已经成为严重的公共健康隐患。
成骨细胞和破骨细胞介导的骨代谢失衡是OP 的重要发病机制。
内源性大麻素系统(ECS )广泛分布于骨组织中,参与调节成骨细胞及破骨细胞的多种生物学功能,可能是OP 的潜在治疗靶点,靶向ECS 进行深入研究能够为OP 的临床治疗提供新的理论依据。
就ECS 在调控OP 发病过程中的具体作用进行了综述。
关键词:骨质疏松;受体,大麻酚;大麻素受体调节剂;成骨细胞;骨代谢中图分类号:R681.4,R614,R285文献标志码:A DOI :10.11958/20230510Research progress of endocannabinoid system for prevention and treatment of osteoporosisWANG Lei 1,YANG Tao 1,GENG Licheng 1,SUN Tianwei 2△1Department of Anesthesiology,2Department of Spine Surgery,Tianjin Union Medical Center,Tianjin 300121,China△Corresponding Author E-mail:Abstract:Osteoporosis (OP)is a kind of systemic bone disease characterized by reduced bone mass and changes of bone microstructure.The incidence rate of OP has been increasing gradually year by year,which has become a severe public health issue.The imbalance of bone metabolism mediated by osteoblasts and osteoclast is an important pathogenesis of OP.The endocannabinoid system (ECS)is widely distributed in bone tissue and participates in regulating multiple biological effects of osteoblasts and osteoclasts,suggesting that ECS may be a potential treatment target of OP.Further study on targeting ESC can provide a new theoretical basis for the clinical treatment of OP.This article reviews the specific role of ECS in regulating the pathogenesis of OP.Key words:osteoporosis;receptors,cannabinoid;cannabinoid receptor modulators;osteoblasts;bone metabolism基金项目:天津市科技计划项目(22JCZDJC00250)作者单位:1天津市人民医院麻醉科(邮编300121),2脊柱外科作者简介:王蕾(1972),男,主治医师,主要从事麻醉药理与骨质疏松症疼痛机制研究。
笔记
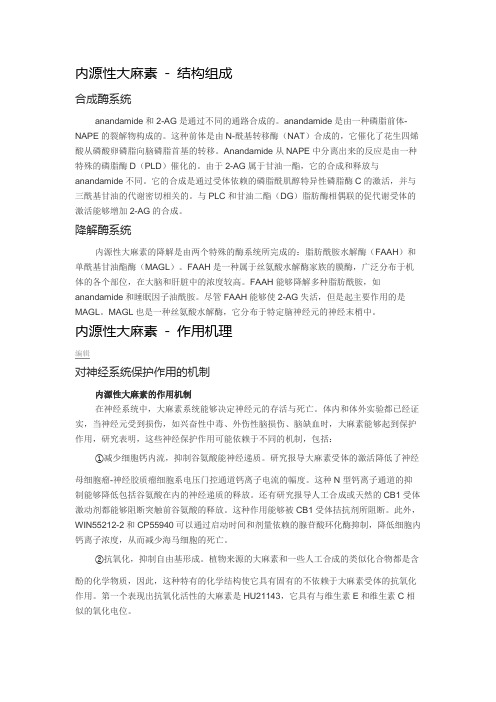
内源性大麻素- 结构组成合成酶系统anandamide和2-AG是通过不同的通路合成的。
anandamide是由一种磷脂前体-NAPE的裂解物构成的。
这种前体是由N-酰基转移酶(NAT)合成的,它催化了花生四烯酸从磷酸卵磷脂向脑磷脂首基的转移。
Anandamide从NAPE中分离出来的反应是由一种特殊的磷脂酶D(PLD)催化的。
由于2-AG属于甘油一酯,它的合成和释放与anandamide 不同。
它的合成是通过受体依赖的磷脂酰肌醇特异性磷脂酶C的激活,并与三酰基甘油的代谢密切相关的。
与PLC和甘油二酯(DG)脂肪酶相偶联的促代谢受体的激活能够增加2-AG的合成。
降解酶系统内源性大麻素的降解是由两个特殊的酶系统所完成的:脂肪酰胺水解酶(FAAH)和单酰基甘油酯酶(MAGL)。
FAAH是一种属于丝氨酸水解酶家族的膜酶,广泛分布于机体的各个部位,在大脑和肝脏中的浓度较高。
FAAH能够降解多种脂肪酰胺,如anandamide和睡眠因子油酰胺。
尽管FAAH能够使2-AG失活,但是起主要作用的是MAGL。
MAGL也是一种丝氨酸水解酶,它分布于特定脑神经元的神经末梢中。
内源性大麻素- 作用机理编辑对神经系统保护作用的机制内源性大麻素的作用机制在神经系统中,大麻素系统能够决定神经元的存活与死亡。
体内和体外实验都已经证实,当神经元受到损伤,如兴奋性中毒、外伤性脑损伤、脑缺血时,大麻素能够起到保护作用,研究表明,这些神经保护作用可能依赖于不同的机制,包括:①减少细胞钙内流,抑制谷氨酸能神经递质。
研究报导大麻素受体的激活降低了神经母细胞瘤-神经胶质瘤细胞系电压门控通道钙离子电流的幅度。
这种N型钙离子通道的抑制能够降低包括谷氨酸在内的神经递质的释放。
还有研究报导人工合成或天然的CB1受体激动剂都能够阻断突触前谷氨酸的释放。
这种作用能够被CB1受体拮抗剂所阻断。
此外,WIN55212-2和CP55940可以通过启动时间和剂量依赖的腺苷酸环化酶抑制,降低细胞内钙离子浓度,从而减少海马细胞的死亡。
人体的第十大系统内源性大麻素系统
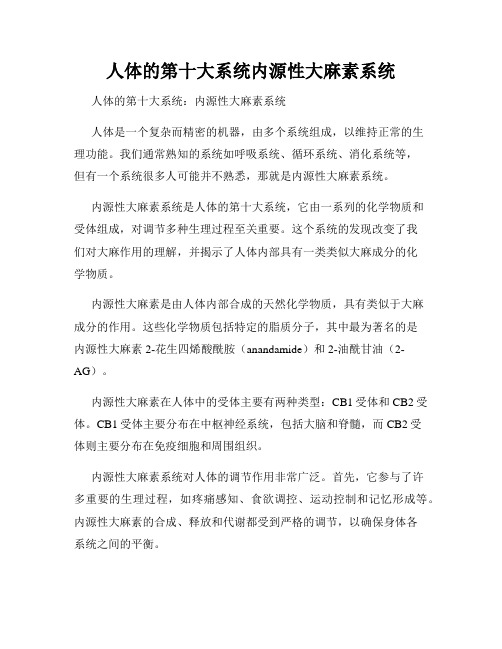
人体的第十大系统内源性大麻素系统人体的第十大系统:内源性大麻素系统人体是一个复杂而精密的机器,由多个系统组成,以维持正常的生理功能。
我们通常熟知的系统如呼吸系统、循环系统、消化系统等,但有一个系统很多人可能并不熟悉,那就是内源性大麻素系统。
内源性大麻素系统是人体的第十大系统,它由一系列的化学物质和受体组成,对调节多种生理过程至关重要。
这个系统的发现改变了我们对大麻作用的理解,并揭示了人体内部具有一类类似大麻成分的化学物质。
内源性大麻素是由人体内部合成的天然化学物质,具有类似于大麻成分的作用。
这些化学物质包括特定的脂质分子,其中最为著名的是内源性大麻素2-花生四烯酸酰胺(anandamide)和2-油酰甘油(2-AG)。
内源性大麻素在人体中的受体主要有两种类型:CB1受体和CB2受体。
CB1受体主要分布在中枢神经系统,包括大脑和脊髓,而CB2受体则主要分布在免疫细胞和周围组织。
内源性大麻素系统对人体的调节作用非常广泛。
首先,它参与了许多重要的生理过程,如疼痛感知、食欲调控、运动控制和记忆形成等。
内源性大麻素的合成、释放和代谢都受到严格的调节,以确保身体各系统之间的平衡。
其次,内源性大麻素系统对于免疫和炎症反应也起着重要作用。
CB2受体的激活可抑制炎症反应,并调节免疫系统的功能,从而对多种免疫相关疾病具有潜在的治疗价值。
此外,内源性大麻素系统还与情绪和精神疾病密切相关。
CB1受体的激活与快乐、奖赏等积极情绪相关,而CB1受体的异常活性则与焦虑、抑郁等精神疾病有关。
因此,内源性大麻素系统可能成为开发新型抗焦虑或抗抑郁药物的潜在靶点。
此外,一些研究还发现内源性大麻素系统与神经发育和神经保护相关。
大麻素对神经细胞的生长和重塑具有一定的调节作用,因此在神经退行性疾病的治疗方面也具有一定的潜力。
尽管内源性大麻素系统在多个方面对人体的调节至关重要,但我们对其了解仍然有限。
目前,许多研究正致力于揭示内源性大麻素系统的更多细节,以期进一步发掘其在健康和疾病中的作用。
探索人体内的“魔法药”——内源性大麻素
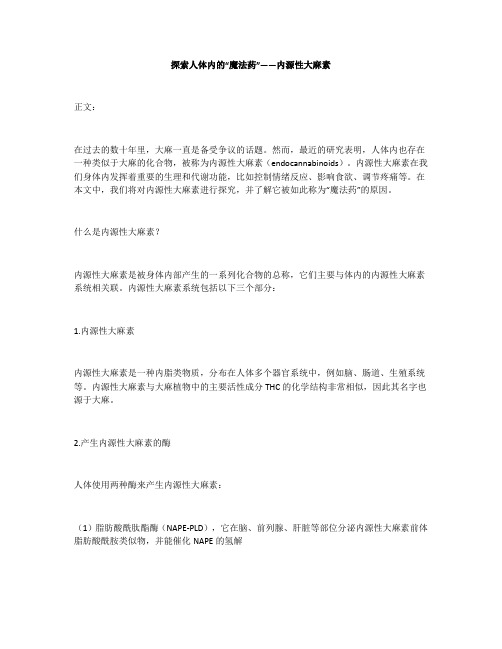
探索人体内的“魔法药”——内源性大麻素正文:在过去的数十年里,大麻一直是备受争议的话题。
然而,最近的研究表明,人体内也存在一种类似于大麻的化合物,被称为内源性大麻素(endocannabinoids)。
内源性大麻素在我们身体内发挥着重要的生理和代谢功能,比如控制情绪反应、影响食欲、调节疼痛等。
在本文中,我们将对内源性大麻素进行探究,并了解它被如此称为“魔法药”的原因。
什么是内源性大麻素?内源性大麻素是被身体内部产生的一系列化合物的总称,它们主要与体内的内源性大麻素系统相关联。
内源性大麻素系统包括以下三个部分:1.内源性大麻素内源性大麻素是一种内脂类物质,分布在人体多个器官系统中,例如脑、肠道、生殖系统等。
内源性大麻素与大麻植物中的主要活性成分THC的化学结构非常相似,因此其名字也源于大麻。
2.产生内源性大麻素的酶人体使用两种酶来产生内源性大麻素:(1)脂肪酸酰肽酯酶(NAPE-PLD),它在脑、前列腺、肝脏等部位分泌内源性大麻素前体脂肪酸酰胺类似物,并能催化NAPE的氢解(2)磷酸脂酰肌醇酰肽合成酶α(PITPNM1),它在脑、肝脏、卵巢中产生内源性大麻素前体磷脂酰肌醇酰胺3.内源性大麻素的作用位点内源性大麻素作用于人体内的多种不同的受体,包括脑内CBI和CB2受体、TRPV1受体、GPR55受体等。
内源性大麻素和健康的关系内源性大麻素的作用方式以及它们在人体内的分布表明,它们在整个身体的生命过程中发挥重要的生理和代谢功能。
在此介绍几个与内源性大麻素相关的健康方面:1.控制情绪反应大麻是一种能够影响情绪的药物。
内源性大麻素系统帮助调节人体内的神经律动性,从而控制情绪反应。
一些研究表明,当大脑中内源性大麻素水平较低时,人们往往更容易出现焦虑、恐惧和紧张感。
2.影响食欲内源性大麻素与食欲有着直接的关系。
当体内内源性大麻素水平升高时,食欲也会增加。
(CB1受体的)激活可以增加进食,而阻断内源性大麻素信号的实验则导致动物进食量减少。
内源性大麻素的疼痛调节机制
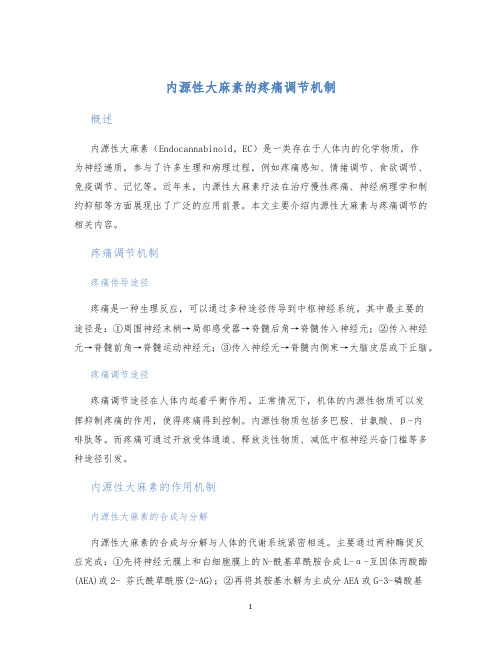
内源性大麻素的疼痛调节机制概述内源性大麻素(Endocannabinoid,EC)是一类存在于人体内的化学物质,作为神经递质,参与了许多生理和病理过程,例如疼痛感知、情绪调节、食欲调节、免疫调节、记忆等。
近年来,内源性大麻素疗法在治疗慢性疼痛、神经病理学和制约抑郁等方面展现出了广泛的应用前景。
本文主要介绍内源性大麻素与疼痛调节的相关内容。
疼痛调节机制疼痛传导途径疼痛是一种生理反应,可以通过多种途径传导到中枢神经系统。
其中最主要的途径是:①周围神经末梢→局部感受器→脊髓后角→脊髓传入神经元;②传入神经元→脊髓前角→脊髓运动神经元;③传入神经元→脊髓内侧束→大脑皮层或下丘脑。
疼痛调节途径疼痛调节途径在人体内起着平衡作用。
正常情况下,机体的内源性物质可以发挥抑制疼痛的作用,使得疼痛得到控制。
内源性物质包括多巴胺、甘氨酸、β-内啡肽等。
而疼痛可通过开放受体通道、释放炎性物质、减低中枢神经兴奋门槛等多种途径引发。
内源性大麻素的作用机制内源性大麻素的合成与分解内源性大麻素的合成与分解与人体的代谢系统紧密相连。
主要通过两种酶促反应完成:①先将神经元膜上和白细胞膜上的N-酰基草酰胺合成L-α-互因体丙酸酯(AEA)或2- 芬氏酰草酰胺(2-AG);②再将其胺基水解为主成分AEA或G-3-磷酸基酰胺 (G3PA)。
内源性大麻素的分解主要在胶质细胞中进行,主要由脑膜素酸途径完成。
内源性大麻素的作用机制内源性大麻素与二酚类(2-arachidonoyl glycerol)等众多物质,共同发挥着抑制疼痛的作用。
在人体内可以与两种不同的受体相结合:大麻素1型受体(CB1)和大麻素2型受体(CB2)。
1.CB1受体CB1受体主要分布在中枢神经系统中,主要与胆固醇共同构成受体,介导内源性大麻素等神经介质的作用。
在这里,内源性大麻素可以使钙离子离开突触前神经元,增加了突触前神经元的兴奋性,并抑制边缘神经元兴奋性的某些水平。
2.CB2受体CB2受体主要定位于免疫系统中,与抗炎作用和调节免疫反应有关。
内源性大麻素系统对肠黏膜屏障的保护作用及作用机制研究进展

内源性大麻素系统对肠黏膜屏障的保护作用及作用机制研究进展吴旭1,王美玲2,李德伟2,赵淑磊1,王京21 山东第一医科大学附属省立医院消化内科,济南250021;2 山东第一医科大学附属省立医院感染性疾病科摘要:肠黏膜屏障由机械屏障、化学屏障、免疫屏障和生物屏障构成,肠黏膜屏障功能失调会造成大量细菌及毒素进入人体引发疾病,保护肠黏膜屏障对人体健康至关重要。
内源性大麻素系统是一种内源性信号通路,由内源性大麻素受体、相应的配体和参与其合成及降解的酶组成。
内源性大麻素系统在消化系统广泛分布,不仅调节胃肠运动,还参与了肠黏膜屏障的保护。
内源性大麻素系统可通过上调肠道紧密连接蛋白的表达参与机械屏障的保护,通过减少肠液分泌参与化学屏障的保护,通过抑制免疫细胞异常活化和减少促炎细胞因子分泌参与免疫屏障的保护,通过改变肠道菌群丰度参与生物屏障的保护。
关键词:内源性大麻素系统;肠黏膜屏障;紧密连接蛋白;免疫细胞;肠道菌群doi:10.3969/j.issn.1002-266X.2023.09.024中图分类号:R574 文献标志码:A 文章编号:1002-266X(2023)09-0098-05肠黏膜屏障是指肠道防止肠内的有害物质如细菌、毒素等穿透肠黏膜进入人体内其他器官和血液循环的结构。
正常肠黏膜屏障由机械屏障、化学屏障、免疫屏障与生物屏障共同构成。
肠道内环境复杂,含有大量细菌和毒素,肠黏膜屏障的结构完整和功能健全对人体健康至关重要,肠黏膜屏障功能失调会导致许多疾病的发生,如肠易激综合征、炎症性肠病、乳糜泻等[1]。
内源性大麻素系统是一种分布广泛的信号系统,在肠神经系统和肠道免疫组织中大量表达,参与胃肠运动及胃酸分泌的调节[2]。
近年来研究[2]发现,内源性大麻素系统参与多种生理过程,如进食、情绪、认知、疼痛、呕吐等,在胃肠道中,激活CB1受体可抑制收缩性递质的释放,抑制胃肠蠕动延缓胃排空,还可抑制胃酸的分泌。
内源性大麻素系统还在许多疾病中发挥重要的作用,如肿瘤性疾病、神经退行性疾病、消化系统疾病及肝脏疾病等,内源性大麻素系统可以抵抗炎症及胃肠异常分泌,是治疗许多消化系统疾病如炎症性肠病、肠易激综合征和分泌/运动相关疾病的靶点[3-4]。
大麻素在神经系统中的作用及机制研究
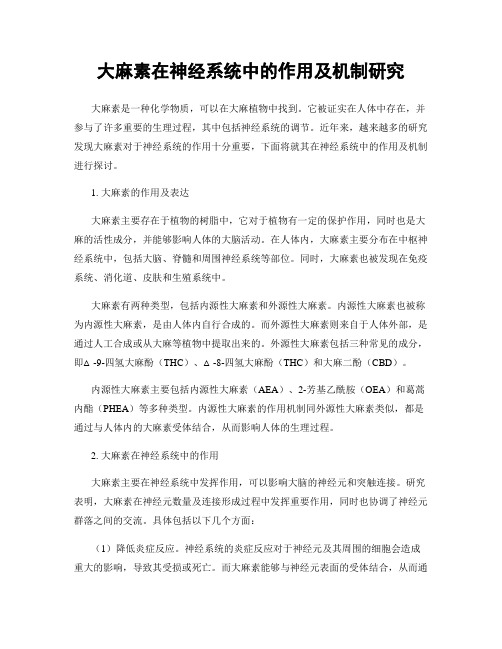
大麻素在神经系统中的作用及机制研究大麻素是一种化学物质,可以在大麻植物中找到。
它被证实在人体中存在,并参与了许多重要的生理过程,其中包括神经系统的调节。
近年来,越来越多的研究发现大麻素对于神经系统的作用十分重要,下面将就其在神经系统中的作用及机制进行探讨。
1. 大麻素的作用及表达大麻素主要存在于植物的树脂中,它对于植物有一定的保护作用,同时也是大麻的活性成分,并能够影响人体的大脑活动。
在人体内,大麻素主要分布在中枢神经系统中,包括大脑、脊髓和周围神经系统等部位。
同时,大麻素也被发现在免疫系统、消化道、皮肤和生殖系统中。
大麻素有两种类型,包括内源性大麻素和外源性大麻素。
内源性大麻素也被称为内源性大麻素,是由人体内自行合成的。
而外源性大麻素则来自于人体外部,是通过人工合成或从大麻等植物中提取出来的。
外源性大麻素包括三种常见的成分,即△-9-四氢大麻酚(THC)、△-8-四氢大麻酚(THC)和大麻二酚(CBD)。
内源性大麻素主要包括内源性大麻素(AEA)、2-芳基乙酰胺(OEA)和葛蒿内酯(PHEA)等多种类型。
内源性大麻素的作用机制同外源性大麻素类似,都是通过与人体内的大麻素受体结合,从而影响人体的生理过程。
2. 大麻素在神经系统中的作用大麻素主要在神经系统中发挥作用,可以影响大脑的神经元和突触连接。
研究表明,大麻素在神经元数量及连接形成过程中发挥重要作用,同时也协调了神经元群落之间的交流。
具体包括以下几个方面:(1)降低炎症反应。
神经系统的炎症反应对于神经元及其周围的细胞会造成重大的影响,导致其受损或死亡。
而大麻素能够与神经元表面的受体结合,从而通过减少细胞内的氧化应激反应和炎症介质的产生,抑制神经元的死亡,减轻炎症反应。
(2)调节神经元兴奋性。
大麻素能够影响神经元和突触连接的兴奋性,从而对神经元的兴奋性及其连接影响。
研究表明,大麻素可以通过促进钙离子进入体内,从而抑制突触间隙内的神经纤维释放,从而降低神经元的兴奋性,减少神经系统的过度兴奋反应,从而治疗一些神经性疾病。
你不“吸毒”,但你的身体里也有“大麻”!你信吗?
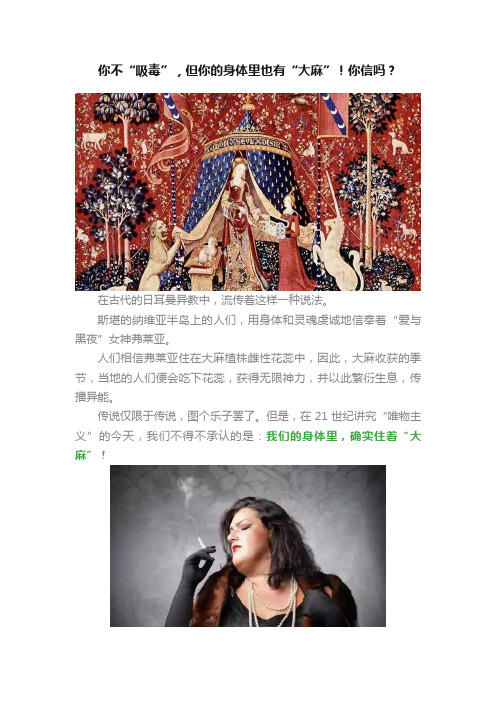
你不“吸毒”,但你的身体里也有“大麻”!你信吗?在古代的日耳曼异教中,流传着这样一种说法。
斯堪的纳维亚半岛上的人们,用身体和灵魂虔诚地信奉着“爱与黑夜”女神弗莱亚。
人们相信弗莱亚住在大麻植株雌性花蕊中,因此,大麻收获的季节,当地的人们便会吃下花蕊,获得无限神力,并以此繁衍生息,传播异能。
传说仅限于传说,图个乐子罢了。
但是,在21世纪讲究“唯物主义”的今天,我们不得不承认的是:我们的身体里,确实住着“大麻”!不要诧异,让我们先来举几个非常普遍的例子:▪当你吃了一根薯条时,你是否经常“停不下来”,直至消灭最后一根?▪当你在不饿的情况下,吃了一口非常“香”的食物,是否还想再多吃一口,甚至觉得饿了?可能很多人都会干掉所有的薯条,或直接跑到餐馆,大吃特吃一顿。
这种在生活中极为普遍的现象,究其根本原因,就在于我们体内的“内源性大麻素系统”。
不要怕怕,虽然“内源性大麻素系统”的名字听上去复杂又古怪,但运行的机制却十分简单。
一、“内源性大麻素系统”到底是啥?顾名思义:▪内源性,说的就是我们人体自身携带的东西,自打娘胎就拥有的。
▪大麻素,是一种大麻类化合物。
结合在一起,“内源性大麻素系统”就是人体自身拥有的大麻化合物系统。
俗话说,存在必有道理。
那么,这套十分玄乎的系统,在我们的身体里,有啥用呢?二、“内源性大麻素系统”到底有啥用?目前,“内源性大麻素系统”公认的受体有CB1和CB2。
CB1受体由473个氨基酸,7个跨膜结构域构成,主要分布在大脑和末梢神经组织中。
CB2受体由360个氨基酸,7个跨膜结构域构成,主要分布在免疫系统和造血细胞中。
CB1和CB2皆属于G蛋白偶联受体,共同的作用都是调节化学递质的释放。
这两个受体成功地占领了人类生存的高地,并且每日参与着我们的学习、记忆、快乐、食欲、运动、平衡、对时间的感知等等一系列反应。
同时,也对大脑的发育功能以及免疫系统功能有重要作用。
换句话说,CB1和CB2两兄弟各司其职,分别维持着我们的“精神”和“肉体”。
内源性大麻素系统神经保护作用及机制
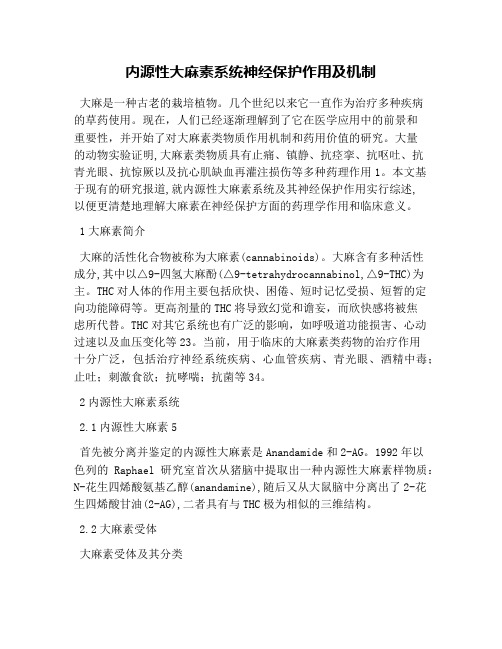
内源性大麻素系统神经保护作用及机制大麻是一种古老的栽培植物。
几个世纪以来它一直作为治疗多种疾病的草药使用。
现在,人们已经逐渐理解到了它在医学应用中的前景和重要性,并开始了对大麻素类物质作用机制和药用价值的研究。
大量的动物实验证明,大麻素类物质具有止痛、镇静、抗痉挛、抗呕吐、抗青光眼、抗惊厥以及抗心肌缺血再灌注损伤等多种药理作用1。
本文基于现有的研究报道,就内源性大麻素系统及其神经保护作用实行综述,以便更清楚地理解大麻素在神经保护方面的药理学作用和临床意义。
1大麻素简介大麻的活性化合物被称为大麻素(cannabinoids)。
大麻含有多种活性成分,其中以△9-四氢大麻酚(△9-tetrahydrocannabinol,△9-THC)为主。
THC对人体的作用主要包括欣快、困倦、短时记忆受损、短暂的定向功能障碍等。
更高剂量的THC将导致幻觉和谵妄,而欣快感将被焦虑所代替。
THC对其它系统也有广泛的影响,如呼吸道功能损害、心动过速以及血压变化等23。
当前,用于临床的大麻素类药物的治疗作用十分广泛,包括治疗神经系统疾病、心血管疾病、青光眼、酒精中毒;止吐;刺激食欲;抗哮喘;抗菌等34。
2内源性大麻素系统2.1内源性大麻素5首先被分离并鉴定的内源性大麻素是Anandamide和2-AG。
1992年以色列的Raphael研究室首次从猪脑中提取出一种内源性大麻素样物质:N-花生四烯酸氨基乙醇(anandamine),随后又从大鼠脑中分离出了2-花生四烯酸甘油(2-AG),二者具有与THC极为相似的三维结构。
2.2大麻素受体大麻素受体及其分类从九十年代初以来,THC就被公认为是通过模仿内源性大麻素而起作用的,而内源性大麻素则是通过结合并激活特殊细胞表面的大麻素受体发挥作用6。
迄今为止,两种大麻素受体-CB1(Cannabinoidreceptor1)和CB2(Cannabinoidreceptor2)都已经分别于1990年7和1993年8在哺乳动物组织中被成功克隆。
人体的第十大系统——内源性大麻素系统

人体的第十大系统——内源性大麻素系统在学校,你应该学过人体中有11个主要的器官系统。
包括循环系统、呼吸系统、泌尿系统、生殖系统、皮肤系统、骨骼系统、肌肉系统、神经系统、内分泌系统、淋巴系统和消化系统。
所有这些系统构成了人体。
但是你知道人体中还存在一个内源性大麻素系统(ECS)吗?很可能你没听说过,除非你是科学家或医用大麻专家。
内源性大麻素系统直到1992年才被发现。
然而,内源性大麻素系统非常重要,被认为是“建立和维持人体健康的最重要的生理系统”。
人体主要系统为什么大多数人不知道内源性大麻素系统(以下简称ECS)?我们对ECS的认识只有25年左右,由以色列科学家L.A. Matsuda 在1992发现。
在研究大麻素对人体影响的过程中,科学家惊奇地发现人体中存在一个复杂的大麻素受体(CBr)网络,广泛分布在中枢和外周神经系统中。
随后,其他的大麻素受体被发现,如CB-2受体,其主要分布在免疫系统、消化系统(胃肠道)和很多主要身体器官中。
CB-1和CB-2受体的发现,科学家产生了一个疑问,如果人体中没有大麻素,为什么会有与之结合的受体呢?这激励科学家去寻找人体中的类大麻素化合物,这些天然的化合物应该可以与受体相互作用。
内源性大麻素系统最先被发现的类大麻素化合物是花生四烯酸乙醇胺。
其可以与CB-1和CB-2受体相互作用,分别调节中枢和外周神经系统。
而外周神经系统的活动可以调节免疫系统功能。
ECS有什么用?我们对ECS的了解还不够完整和全面,但已经确定ECS有助于微调人体的大部分重要生理功能。
ECS可以促进体内平衡,影响睡眠、食欲、疼痛、炎症、记忆、情绪,甚至生殖。
因此,ECS有助于人体调节所有主要的身体系统的平衡,确保所有系统能彼此协同工作。
以下是7个有关内源性大麻素系统的事实:1. 所有动物都具有ECS作为人类,我们通常认为自己很特别,与动物不同,但事实上并没有,至少在ECS方面。
所有的脊椎动物都具有ECS。
内源性大麻素系统与炎症性皮肤病
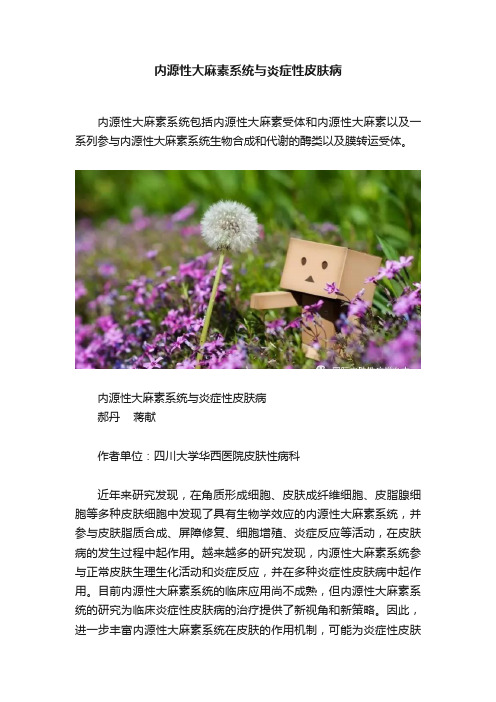
内源性大麻素系统与炎症性皮肤病
内源性大麻素系统包括内源性大麻素受体和内源性大麻素以及一系列参与内源性大麻素系统生物合成和代谢的酶类以及膜转运受体。
内源性大麻素系统与炎症性皮肤病
郝丹蒋献
作者单位:四川大学华西医院皮肤性病科
近年来研究发现,在角质形成细胞、皮肤成纤维细胞、皮脂腺细胞等多种皮肤细胞中发现了具有生物学效应的内源性大麻素系统,并参与皮肤脂质合成、屏障修复、细胞增殖、炎症反应等活动,在皮肤病的发生过程中起作用。
越来越多的研究发现,内源性大麻素系统参与正常皮肤生理生化活动和炎症反应,并在多种炎症性皮肤病中起作用。
目前内源性大麻素系统的临床应用尚不成熟,但内源性大麻素系统的研究为临床炎症性皮肤病的治疗提供了新视角和新策略。
因此,进一步丰富内源性大麻素系统在皮肤的作用机制,可能为炎症性皮肤
病治疗、皮肤屏障修复策略等方面提供新的切入点。
DOI:10.3760/cma.j.issn.1673-4173.2017.02.011
《国际皮肤性病学杂志》,2017,43(2):102-105。
ec的功能主治
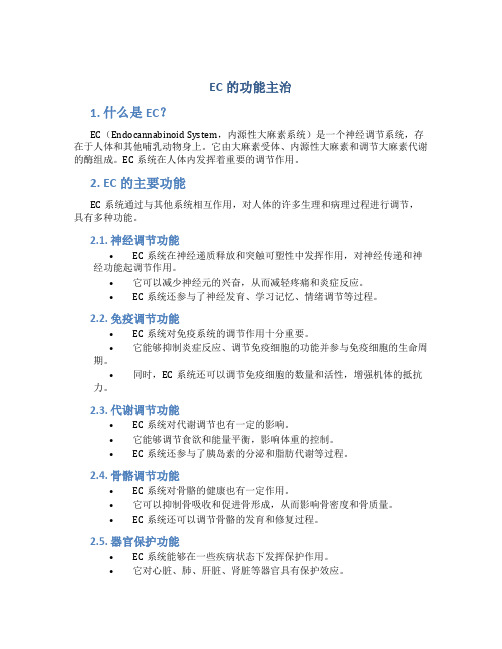
EC的功能主治1. 什么是EC?EC(Endocannabinoid System,内源性大麻素系统)是一个神经调节系统,存在于人体和其他哺乳动物身上。
它由大麻素受体、内源性大麻素和调节大麻素代谢的酶组成。
EC系统在人体内发挥着重要的调节作用。
2. EC的主要功能EC系统通过与其他系统相互作用,对人体的许多生理和病理过程进行调节,具有多种功能。
2.1. 神经调节功能•EC系统在神经递质释放和突触可塑性中发挥作用,对神经传递和神经功能起调节作用。
•它可以减少神经元的兴奋,从而减轻疼痛和炎症反应。
•EC系统还参与了神经发育、学习记忆、情绪调节等过程。
2.2. 免疫调节功能•EC系统对免疫系统的调节作用十分重要。
•它能够抑制炎症反应、调节免疫细胞的功能并参与免疫细胞的生命周期。
•同时,EC系统还可以调节免疫细胞的数量和活性,增强机体的抵抗力。
2.3. 代谢调节功能•EC系统对代谢调节也有一定的影响。
•它能够调节食欲和能量平衡,影响体重的控制。
•EC系统还参与了胰岛素的分泌和脂肪代谢等过程。
2.4. 骨骼调节功能•EC系统对骨骼的健康也有一定作用。
•它可以抑制骨吸收和促进骨形成,从而影响骨密度和骨质量。
•EC系统还可以调节骨骼的发育和修复过程。
2.5. 器官保护功能•EC系统能够在一些疾病状态下发挥保护作用。
•它对心脏、肺、肝脏、肾脏等器官具有保护效应。
•EC系统还可以减轻缺血再灌注损伤,促进组织修复和再生。
3. EC的主治领域EC系统在多个领域中的作用得到了研究和应用。
3.1. 疼痛管理•EC系统可以通过调节疼痛传导途径和减少神经兴奋来缓解疼痛。
•因此,EC系统在疼痛管理中具有重要作用。
•一些研究还表明,通过激活EC系统,可以减少对传统镇痛药物的使用。
3.2. 炎症调节•EC系统对炎症反应的调节作用已被广泛研究。
•它可以抑制免疫细胞的激活和炎症因子的释放,从而减轻炎症反应。
•一些研究还发现,激活EC系统可以减少炎症相关疾病的发生和发展。
【专业科普】ω-3脂肪酸抗炎新介质:内源性大麻素!|机理研究

【专业科普】ω-3脂肪酸抗炎新介质:内源性大麻素!|机理研究大麻素从印度大麻中发现,是一类可以与体内大麻素受体结合的萜酚类化合物,但也泛指其它可以与大麻素受体结合的物质,包括在人体内自然地由ω-3和ω-6脂肪酸衍生的内源性大麻素。
最为众所周知的大麻素,四氢大麻酚(tetrahydrocannabinol,THC),可以让人觉得兴奋(欣快的感觉),但同时也具有抗炎作用。
另一方面,临床研究表明,丰富的ω-3多不饱和脂肪酸(PUFA)的饮食提供有益的抗炎作用,部分原因是它们转化为生物活性代谢物。
那么,我们不禁要问了:既然都可以消炎,那么大麻素和ω-3脂肪酸是否有某种潜在联系呢?小科普 >>>大麻素的历史|1964年,以色列化学家Raphael Mechoulam率先从大麻中发现和分离THC。
为了测试他是否找到产生兴奋剂的化合物,他在蛋糕中加了10毫克的纯THC,并在派对上分派给了愿意尝试的朋友。
这些朋友的反应,从不间断的笑声、昏睡到贫嘴,证实了THC 是一类影响精神的大麻素。
直到1992年,研究人员发现我们的身体内会自然产生内源性大麻素。
从那时起,其他几种内源性大麻素也被发现,但各自的功能尚不完全清晰。
最新发现|2017年7月7日,来自于University of Illinois at Urbana-Champaign的研究人员在国际知名杂志《Proceedings of the National Academy of Sciences》上发表的最新研究揭示,ω-3脂肪酸可以转化为具有抗炎益处但没有精神药效的“ω-3内源性大麻素环氧化物”(EDP-EA和EEQ-EA),它们的浓度与其他内源性大麻素相当,预计在体内炎症过程中发挥关键作用。
研究结论与意义|大麻素与体内两种类型的大麻素受体结合,一类在神经系统(CB1),一类在免疫系统(CB2)。
一些大麻素,如大麻或内源性大麻素中的THC可以与这些受体结合,诱导消炎和镇痛作用。
- 1、下载文档前请自行甄别文档内容的完整性,平台不提供额外的编辑、内容补充、找答案等附加服务。
- 2、"仅部分预览"的文档,不可在线预览部分如存在完整性等问题,可反馈申请退款(可完整预览的文档不适用该条件!)。
- 3、如文档侵犯您的权益,请联系客服反馈,我们会尽快为您处理(人工客服工作时间:9:00-18:30)。
Mini-SymposiumFunctional Interactions between Stress and the Endocannabinoid System:From Synaptic Signalingto Behavioral OutputMatthew N.Hill,1Sachin Patel,2Patrizia Campolongo,3Jeffrey G.Tasker,4Carsten T.Wotjak,5and Jaideep S.Bains6,71Laboratory of Neuroendocrinology,The Rockefeller University,New York,New York10065,2Department of Psychiatry,Vanderbilt University,Nashville, Tennessee37232,3Department of Physiology and Pharmacology,Sapienza University of Rome,00185Rome,Italy,4Department of Cell and Molecular Biology,Tulane University,New Orleans,Louisiana70118,5Laboratory of Neuronal Plasticity,Max Planck Institute of Psychiatry,80804Munich,Germany, and6Department of Physiology and Pharmacology,and7The Hotchkiss Brain Institute,University of Calgary,Calgary,Alberta T2N4N1,Canada Endocannabinoid signaling is distributed throughout the brain,regulating synaptic release of both excitatory and inhibitory neurotrans-mitters.The presence of endocannabinoid signaling within stress-sensitive nuclei of the hypothalamus,as well as upstream limbic structures such as the amygdala,suggests it may play an important role in regulating the neuroendocrine and behavioral effects of stress. The evidence reviewed here demonstrates that endocannabinoid signaling is involved in both activating and terminating the hypothalamic-pituitary-adrenal axis response to both acute and repeated stress.In addition to neuroendocrine function,however, endocannabinoid signaling is also recruited by stress and glucocorticoid hormones to modulate cognitive and emotional processes such as memory consolidation and extinction.Collectively,these data demonstrate the importance of endocannabinoid signaling at multiple levels as both a regulator and an effector of the stress response.The endocannabinoid systemCannabis has been used as a recreational drug in a multitude of cultures for centuries(Russo,2007).Typically,cannabis pro-duces anxiolytic and mood-elevating effects that promote relax-ation and stress reduction,which likely contributes to its high level of recreational use(Green et al.,2003).In the late1980s,it was discovered that⌬9-tetrahydrocannabinol(THC),the psycho-active constituent of cannabis,bound to specific receptors within brain tissue(Devane et al.,1988),setting the stage for the subsequent discovery of the endogenous cannabinoid system.In the early1990s, the genetic characterization and neuroanatomical localization of the cannabinoid receptor(termed the CB1receptor)was mapped in the rodent brain(Matsuda et al.,1990;Herkenham et al.,1991).Soon after,the endogenous cannabinoid ligands[termed endocannabi-noids(eCBs)]N-arachidonylethanolamine(AEA)(named anand-amide,after anand,the Sanskrit word for bliss;Devane et al.,1992) and2-arachidonoylglycerol(2-AG)(Sugiura et al.,1995)were dis-covered.While quite distinct in structure from THC,both AEA and 2-AG share the lipophilicity of THC,being arachidonate-derived neuroactive signaling lipids.Unlike canonical neurotransmitters, both AEA and2-AG are postsynaptically synthesized signaling mol-ecules that are not stored in vesicles,but are generated on demand and then liberated to act in a retrograde fashion on presynaptically localized CB1receptors(Wilson and Nicoll,2002).The signaling lifespan of AEA and2-AG,in turn,is primarily regulated by yet ill-defined uptake processes and intracellular hydrolysis by fatty acid amide hydrolase(FAAH)and monoacylglycerol lipase(MAG-L), respectively(Hillard,2000).Recent observations also implicate serine hydrolase␣,-hydrolase domain6(ABHD6)as an essential regulator of2-AG and thus a key player in the control of eCB efficacy (Marrs et al.,2010).The CB1receptor is coupled to G i/o proteins and functions to suppress adenylate cyclase activity and calcium influx, resulting in the suppression of neurotransmitter release.Accord-ingly,the primary function of the eCB system is to gate and regulate neurotransmitter release,particularly GABA and glutamate,thus fine tuning the strength of a particular synapse(Freund et al.,2003). The stress response:a role for endocannabinoids?Stress is most readily defined as any stimulus that presents a challenge to homeostasis—typically a real or perceived threat to an organism’s well being.Activation of the hypothalamic-pituitary-adrenal(HPA)axis is a well characterized response to stress.Activation of the HPA axis results in the release of corticotropin-releasing hormone(CRH)from neurosecretory cells of the paraventricular nucleus of the hypothalamus(PVN) into the portal vessels,where it is then transported to the anterior pituitary gland and stimulates the release of adrenocorticotropic hormone(ACTH)into the general circulation(Herman et al., 2002).In turn,ACTH causes the release of glucocorticoid hor-mones(primarily corticosterone in rodents and cortisol in hu-mans)from the adrenal cortex into the circulation.A majorReceived Aug.16,2010;revised Sept.7,2010;accepted Sept.8,2010.The writing of this review,and the research described herein,was supported by an operating grant from the Canadian Institute of Health Research(CIHR)to J.S.B.and a postdoctoral fellowship from CIHR to M.N.H.,as well as National Institutes of Health Grant K08MH090412to S.P.and National Institute of Mental Health Grant2R01 MH066958to J.G.T.Additionally,funding support was provided by the Catherine and Hunter Pierson Chair in Neuroscience,Tulane University Research Enhancement Fund to J.G.T.Correspondence should be addressed to Dr.Matthew N.Hill,Laboratory of Neuroendocrinology,The Rockefeller University,1230York Avenue,Box165,New York,NY10065.E-mail:mhill@.DOI:10.1523/JNEUROSCI.4283-10.2010Copyright©2010the authors0270-6474/10/3014980-07$15.00/014980•The Journal of Neuroscience,November10,2010•30(45):14980–14986function of glucocorticoids during stressful experiences is to mobi-lize energy stores,which allows the organism to mount the appro-priate response to a threat.In addition,glucocorticoids produce a range of effects on cardiovascular,immune,metabolic,and neural systems that facilitate optimal responses to aversive stimuli (Pecoraro et al.,2006).Excessive and unchecked secre-tion of glucocorticoids,however,can produce detrimental effects on health and increase the susceptibility of the organism to an array of disease states,ranging from mood disorders to type II diabetes.To circumvent this,glucocorticoids exert potent nega-tive feedback inhibition of the HPA axis,such that elevated cir-culating levels of glucocorticoids rapidly suppress HPA axisactivity.Negative feedback of the HPA axis occurs at multiplelevels in the periphery (e.g.,adrenal and pituitary glands)and thebrain.Within the PVN,glucocorticoids exhibit both rapid anddelayed suppression of HPA axis activity (Dallman,2005).Inaddition,extrahypothalamic limbic structures that communicatewith the PVN exert both positive and negative regulation of theHPA axis (Ulrich-Lai and Herman,2009).The amygdala hasbeen identified as one of the primary limbic structures involvedin activating the HPA axis in response to stressful stimuli.Thehippocampus and prefrontal cortex,on the other hand,havebeen identified as inhibiting the HPA axis,and are also involvedin the extrahypothalamic glucocorticoid-mediated negative feed-back of the HPA axis.Given the pronounced effects of cannabis on emotionality andthe processing of stressful information,it is not surprising thateCB signaling is widely distributed throughout corticolimbic cir-cuits that are linked to the stress response.With respect to theCNS,CB 1receptors are expressed and both AEA and 2-AG aresynthesized in the hypothalamus,including locally within the PVN (Malcher-Lopes et al.,2006),as well as in upstream struc-tures such as the amygdala,hippocampus,and prefrontal cortex (Herkenham et al.,1991;Hill et al.,2008).As cannabis consump-tion is known to produce anxiolytic and stress-reducing effects in humans,the question arose as to whether eCB signaling itself was involved in regulating the stress response and subsequent changes in neuroendocrine function and emotional behavior.Following is a review of a mini-symposium that summarizes the current state of knowledge regarding how eCB signaling integrates into the regula-tion of the HPA axis in response to stress and,in turn,how stress-induced regula-tion of eCB signaling is involved in both glucocorticoid feedback and changes in emotional behavior.Endocannabinoids and activation of the HPA axisThe presence of CB 1receptors within cor-ticolimbic circuits regulating the HPA axis and the anti-stress properties of can-nabis consumption suggest that eCB sig-naling should inhibit the stress response.Both genetic disruption and pharmaco-logical blockade of CB 1receptor signaling result in an increase in HPA axis activity under basal conditions and following ex-posure to acute stress (Patel et al.,2004;Wade et al.,2006;Cota et al.,2007;Steiner and Wotjak,2008).These data indicate that an eCB tone provides a steady-stateinhibition of HPA axis activity,which,when disrupted,results in activation of the HPA axis.This gate-keeper model was supported by subsequent studies revealing adecrease in AEA content in the amygdala,through an increase in FAAH-mediated hydrolysis,in response to acute stress (Patel et al.,2005b;Rademacher et al.,2008;Hill et al.,2009).Preventing this decline in amygdalar AEA by local administration of a FAAHinhibitor into the basolateral nucleus (BLA)attenuates stress-induced activation of the HPA axis (Hill et al.,2009).Similarly,local administration of a CB 1receptor agonist directly into theBLA dramatically reduces stress-induced activation of the HPA axis (Ganon-Elazar and Akirav,2009;Hill et al.,2009).Given that administration of a CB 1receptor antagonist to unstressed ani-mals increases neuronal activation of the BLA (Singh et al.,2004;Patel et al.,2005a),and that CB 1receptors regulate the release of glutamate within the amygdala (Azad et al.,2003;Domenici et al.,2006;Kodirov et al.,2009;Karst et al.,2010),we propose (Fig.1)that under steady-state conditions,a tonic level of AEA/CB 1receptor signaling within the BLA gates glutamatergic inputs to principal neurons in the BLA.In response to stress,FAAH activity increases and AEA levels decline,resulting in a disinhibition of BLA principal neurons and thereby an increase in the outflow of amygdalar neu-rons and downstream targets of the amygdala,such as the HPA axis (Hill et al.,2009).In support of this model,genetic work has deter-mined that CB 1receptors localized on glutamatergic,and not on GABAergic,neurons are responsible for the ability of eCBs to re-strain activation of the HPA axis (Steiner et al.,2008).Stress and glucocorticoid-mediated regulation of endocannabinoid signaling in the hypothalamus:a role in glucocorticoid feedback In addition to contributing to the regulation of the HPA axis,eCB signaling is also regulated by the HPA axis.In vitro studies havedemonstrated that direct application of glucocorticoids to hypo-thalamic slices results in a rapid (ϳ10min)elevation in the tissue content of both AEA and 2-AG (Malcher-Lopes et al.,2006).In vivo studies have supported these findings by demonstrating that peripheral administration to rats of corticosterone results in a rapid (ϳ10min)increase in both AEA and 2-AG levels in the hypothalamus (Hill et al.,2010a).Similarly,exposure to 30minFigure 1.A ,Under steady-state conditions,AEA signaling within the BLA restrains glutamatergic signaling and helps maintain non-stress conditions.B ,In response to stress,FAAH activity increases,reducing AEA content and disinhibiting glutamate release onto BLA neurons,facilitating an adequate stress response.Hill et al.•Endocannabinoids and the Stress Response J.Neurosci.,November 10,2010•30(45):14980–14986•14981of restraint stress results in a significant elevation in hypothalamic 2-AG content,while having no effect on tissue levels of AEA (Evanson et al.,2010).Thus,stress increases hypothalamic eCB signaling through a glucocorticoid-mediated path-way.Furthermore,given the rapid effectsof glucocorticoids on hypothalamic eCB signaling,these effects are likely not medi-ated by the canonical actions of glucocor-ticoids on gene transcription.The function of this increase in hypo-thalamic eCB signaling in response to stress has been highlighted by a series ofin vitro and in vivo studies demonstrat-ing a critical role of this process inglucocorticoid-mediated negative feed-back.Following activation of the HPAaxis in response to stress,elevated circu-lating glucocorticoids feed back onto the brain to suppress stress-induced HPA ac-tivation via both a rapid,transcription-independent inhibitory mechanism and a delayed,transcription-dependent regula-tion of HPA output.Intracellular record-ings from in vitro brain slices have demonstrated a rapid glucocorticoid-induced suppression of glutamatergic ex-citatory synaptic inputs to CRH neurons of the PVN that is mediated by a putative membrane glucocorticoid receptor (Di et al.,2003).This suppression of excitatory inputs to CRH neurosecretory cells may represent a mechanism of fast-feedback inhibition of the HPA axis,whereby glucocorti-coids can rapidly decrease incoming excitatory input and de-crease activation of the HPA axis at the level of the PVN.This rapid glucocorticoid suppression of PVN neurons is blocked by inhibiting postsynaptic G-protein activity and by blocking CB 1receptors (Di et al.,2003).The glucocorticoid effect is also blocked by inhibiting the activity of diacylglycerol lipase,a synthetic enzyme of 2-AG (C.C.Harris and J.G.Tasker,unpublished obser-vation),indicating that the glucocorticoid suppression of excitation is mediated by the eCB 2-AG.These findings are consistent with the ability of glucocorticoids to induce 2-AG synthesis rapidly inthe hypothalamus in vitro and in vivo (Malcher-Lopes et al.,2006;Hill et al.,2010a).These observations implicate a rapidglucocorticoid-induced suppression of the excitatory synaptic driveto CRH neurons via the dendritic release and retrograde actions ofeCBs in the PVN,providing a potential mechanism for the rapidnegative feedback effects of glucocorticoids on the HPA axis via amembrane-associated glucocorticoid receptor and noncanonicalglucocorticoid signaling mechanism (Fig.2).The postulated glucocorticoid-eCB mechanism of rapidHPA axis feedback inhibition has recently been tested in vivo instudies in which glucocorticoids and cannabinoid analogs wereadministered directly into the PVN.Local bilateral PVN admin-istration of glucocorticoids,including a dexamethasone-BSAconjugate that is membrane-impermeant and restricted to theextracellular environment,dampens the restraint stress-inducedsecretion of ACTH and corticosterone,suggesting a rapid glu-cocorticoid inhibition of the HPA axis directly at the level of thePVN (Evanson et al.,2010).The rapid glucocorticoid-mediatedsuppression of HPA axis activation is prevented by the coapplicationinto the PVN of the CB 1receptor antagonist AM251[N -(piperidin-1-yl)-5-(4-iodophenyl)-1-(2,4-dichlorophenyl)-4-methyl-1H -pyrazole-3-carboxamide],indicating that the rapid glucocorticoid effect is mediated by eCB actions in the PVN.Again,this is consistentwith the observation that both restraint stress and glucocorticoids cause a rapid increase in the level of 2-AG in the hypothalamus (Evanson et al.,2010;Hill et al.,2010a).These findings support a rolefor the glucocorticoid-induced eCB release in the PVN in the rapid glucocorticoid feedback regulation of the HPA axis.It has recently been demonstrated that exposure of young ratsto repetitive,but not acute,immobilization stress (30min/d for 5d)results in a loss of eCB-mediated retrograde signaling in parvocellu-lar neurosecretory cells (PNCs)of the PVN (Wamsteeker et al.,2010).Specifically,following repeated stress,application of dexa-methasone to hypothalamic slices no longer causes an eCB-mediated suppression of excitatory inputs to PNCs (Wamsteekeret al.,2010),as was described above.Confirming that this loss of glucocorticoid-mediated suppression of excitation in the PVN is due to an impairment of eCB signaling,bath application of a CB 1receptor agonist to PVN slices from repeatedly stressed animals does not suppress excitatory transmission to PNCs (Wamsteeker et al.,2010).Moreover,the ability of a CB 1receptor agonist toinhibit GABA transmission to PNCs is also compromised follow-ing repeated stress (Wamsteeker et al.,2010).This conundrum may underscore the notion that following stress,GABA does not necessarily function as an inhibitory transmitter.In fact,follow-ing stress-induced changes in chloride homeostasis in PNCs,GABA can act in an excitatory capacity when synapses are acti-vated by bursts of activity (Hewitt et al.,2009).This loss of CB 1receptor function in the PVN has important implications for glucocorticoid-mediated feedback inhibition of the Figure 2.Fast-feedback inhibition of the HPA axis via glucocorticoid-induced eCB release in the hypothalamus.Left,StressactivationoftheHPAaxisconsistsofCRHsecretionfromPVNneuronsandCRH-evokedACTHsecretionfromthepituitary,which,inturn,stimulatescorticosteroid(CORT)releasefromtheadrenalcortexandCORTfeedbackontothePVN.Right,InPVNCRHneurons,CORT binds to a membrane-associated glucocorticoid receptor (mGR)(1),which causes eCB synthesis in CRH neurons (2)and retrograde eCB release (3);eCB binds to presynaptic CB1receptors on glutamate terminals (4)and inhibits glutamate release (5)onto the CRH neurons,suppressing the excitatory synaptic drive and decreasing CRH neuron activity and CRH release (6),which suppresses HPA axis activation.14982•J.Neurosci.,November 10,2010•30(45):14980–14986Hill et al.•Endocannabinoids and the Stress ResponseHPA.As glucocorticoid-mediated induction of eCB signaling within the PVN is integral for fast-feedback inhibition of the HPA axis (see above and Fig.2),this process may be compromised following chronic stress.In line with this hypothesis,in vivo studies haveshown that chronic stress exposure results in impairedglucocorticoid-mediated fast-feedback inhibition of the HPAaxis (Young et al.,1990).Together,these data indicate that glucocorticoids induce eCBsignaling through a rapid,nongenomic process in CRH neurons ofthe PVN.This induction of eCB signaling inhibits glutamatergicinputs to CRH neurons,and thus decreases the excitatory drive tothe HPA axis.This process is believed to subserve glucocorticoid-mediated fast-feedback inhibition of the HPA axis.Followingchronic stress,eCB signaling in the PVN is compromised,whichresults in impaired fast-feedback inhibition of the HPA axis,andlikely contributes to the hypersecretion of glucocorticoids followingchronic stress (Hill et al.,2010b).Stress and glucocorticoid-mediated regulation ofendocannabinoid signaling in the amygdala:a role inemotional memoryGlucocorticoid-mediated induction of eCB signaling occurs notonly in the hypothalamus,but also in the amygdala.While expo-sure to stress rapidly reduces tissue levels of AEA in the amygdala(Patel et al.,2005b;Rademacher et al.,2008;Hill et al.,2009),administration of corticosterone in the absence of stress results ina rapid increase in tissue levels of AEA in the amygdala (Hill et al.,2010a).These apparently contradictory findings have lead to thehypothesis that stress,through a glucocorticoid-independentmechanism,rapidly decreases amygdalar AEA content,which isreversible by increasing levels of glucocorticoids following acti-vation of the HPA axis (Hill and McEwen,2010).At the functional level,there is growing interest in the putativeinteractions between glucocorticoids and eCBs within the amyg-dala.Accumulating evidence demonstrates that glucocorticoidhormones enhance long-term consolidation of emotionally arous-ing experiences involving rapid actions on intracellular signalingcascades in the BLA (Roozendaal,2000;McGaugh,2002).Given the ability of glu-cocorticoids to rapidly mobilize eCB sig-naling,the eCB system seems to be a plausible target to mediate these glu-cocorticoid effects.Bilateral post-training infusions of the CB 1receptor agonist WIN55,212-2[(R )-(ϩ)-[2,3-dihydro-5-methyl-3-(4-morpholinylmethyl)pyr-rolo-[1,2,3-d ,e ]-1,4-benzoxazin-6-yl]-1-naphthalenylmethanone]into the BLA induced dose-,time-,and site-dependent enhancement of inhibitory avoidance retention performance that was compa-rable to performance seen following post-training glucocorticoid adminis-tration (Campolongo et al.,2009).In line with these parallels between CB 1re-ceptor activation and glucocorticoids,intra-BLA administration of a CB 1re-ceptor antagonist,at a dose ineffective,per se,on memory consolidation,is ca-pable of blocking the ability of systemi-cally administered corticosterone to facilitate aversive memory consolida-tion (Campolongo et al.,2009).Together,these findings indicate that glucocorticoids recruit eCB signaling in the BLA to modulate aversive memory consolidation.It is well established that activation of CB 1receptors decreasesGABA release (Ohno-Shosaku et al.,2001;Freund et al.,2003)via a rapid inhibition of Ca 2ϩentry into the terminals (Hoffman and Lupica,2000;Wilson et al.,2001).Furthermore,it has been re-ported that the amygdalar GABAergic system modulates memory storage (McGaugh,2002)and that inhibition of GABAergic ac-tivity within the BLA enhances memory consolidation by increas-ing the release of norepinephrine (NE)(Hatfield et al.,1999),a neurotransmitter involved in mediating emotional arousal ef-fects on memory consolidation (McGaugh,2000,2004).In view of this evidence,the following model is proposed:corticosterone binds to a membrane-bound glucocorticoid receptor (as dis-cussed above),which activates the Gs–cAMP/protein kinase A pathway to induce eCB synthesis,similar to the rapid glucocor-ticoid signaling in the PVN (Malcher-Lopes et al.,2006).eCBs arereleased into the synapse,where they bind to CB 1receptors on GABAergic terminals to inhibit GABA release.This inhibition of GABA release leads to the disinhibition of NE release and increases noradrenergic activation of postsynaptic adrenoreceptors,in-creasing the consolidation of emotionally aversive memories (for model,see Hill and McEwen,2009;Campolongo et al.,2009).As such,these data provide functional evidence of glucocorticoid-mediated recruitment of eCB signaling in the amygdala,demon-strating that this phenomenon is important for complex behavioral processes in addition to glucocorticoid-mediated feedback.A role for amygdalar endocannabinoid signaling in adaptation to stress and aversive stimuli Unlike the hypothalamus,which exhibits a robust increase in 2-AG content following stress or glucocorticoid treatment (Malcher-Lopes et al.,2006;Evanson et al.,2010;Hill et al.,2010a),the amygdala shows no change in 2-AG content in re-sponse to an acute stressor (Patel et al.,2005b;Rademacher et al.,2008;Hill et al.,2009).However,following repeated presentation of an aversive stimulus,2-AG signaling within the amygdala pro-Figure3.A ,Inresponsetoacutestress,thereislittletonochangein2-AGwithintheBLA.B ,Repeatedstressexposure,however,primes 2-AGsignalingwithintheBLAsuchthatinresponsetostressorpresentation,thereisaphasicandlimitedincreasein2-AGsignalingwithin the BLA which suppresses glutamatergic inputs to the BLA,decreasing the outflow of the amygdala and driving habituation of the stress response.Asimilarphenomenonmayalsosubservebehavioralplasticity,suchasfearextinction,toaversivestimuli.Hill et al.•Endocannabinoids and the Stress Response J.Neurosci.,November 10,2010•30(45):14980–14986•14983gressively increases(Patel et al.,2005b,2009;Rademacher et al., 2008;Hill et al.,2010b).This response is temporally restricted, with elevations in2-AG found within20–30min of the onset of stress(Patel et al.,2009;Hill et al.,2010b),but waning within1h after the onset of stress(Patel et al.,2009)and reversing com-pletely within24h of exposure to stress(Hill et al.,2010b).Based on these data,it has been suggested that repeated stress exposure results in an enhanced capacity to elevate2-AG levels following subsequent stress exposures(Patel et al.,2009).Given the prominent role of2-AG in mediating retrograde eCB signaling at central synapses(Kano et al.,2009),a clear ex-tension of the above findings is that eCB-mediated synaptic sup-pression would be enhanced after chronic stress exposure.An inherent assumption in this hypothesis is that tissue levels of 2-AG correlate with the magnitude of eCB-mediated synaptic signaling,one that had not been previously tested.In accor-dance with this hypothesis,a prototypic form of eCB signaling, depolarization-induced suppression of inhibition(DSI),was found to be significantly enhanced in BLA neurons of mice ex-posed to10d of restraint stress(Patel et al.,2009).One day of restraint stress exposure did not affect DSI,consistent with a lack of change in2-AG levels seen after acute stress exposure(Hill et al.,2009;Patel et al.,2009).These findings support the notion that tissue levels of2-AG correlate with the magnitude of eCB signaling.With regard to the functional implications of repeated stress-induced elevations in amygdala2-AG signaling,Hill et al. (2010b)have recently shown that this process is critical for the habituation of the HPA axis that occurs in response to repeated homotypic stressor exposure.Specifically,the elevation of2-AG within the amygdala following repeated stress correlated with the magnitude of HPA axis suppression,and local administration of a CB1receptor antagonist into the BLA reversed the expression of stress habituation(Hill et al.,2010b).Accordingly,increased re-cruitment of2-AG signaling within the BLA appears to be essen-tial for adaptation of the HPA axis to repeated stress.Given the aforementioned model by which eCB signaling in the BLA gates glutamatergic inputs to principal neurons,the most parsimoni-ous explanation of these data is that a transient augmentation of 2-AG signaling upon repeated stressor exposure dampens excita-tory inputs to the BLA,thereby decreasing the outflow of the amygdala,which would include stimulation of the HPA axis(Hill et al.,2010b)(see Fig.3for model).This model is in accordance with a recent report demonstrating that corticosterone inhibits glutamatergic inputs to the BLA through an eCB-mediated mechanism,but only in animals that have a history of previous stress exposure(Karst et al.,2010).In addition to contributing to adaptation of the HPA axis to stressful stimuli,eCB signaling in the amygdala is also important for behavioral adaptation to aversive stimuli.In response to an acutely presented aversive stimulus,an organism must recognize a potential threat and adequately react to it,including fear and anxiety responses.These behavioral responses rapidly vanish when the threat is removed.Such cognitive-emotional flexibility allows an organism to both respond and adapt to threats.Recent observations suggest an important role of the eCB system in this complex adaptive ing classical associative fear condi-tioning and extinction paradigms,it was revealed that during extinction training,but not initial fear conditioning,eCB levels in the amygdala,but not in the prefrontal cortex,were elevated (Marsicano et al.,2002).The relevance of this increase in eCB signaling was highlighted by subsequent behavioral studies dem-onstrating that mice lacking the CB1receptor exhibited pro-longed expression of fear behaviors during extinction training, indicating that this augmentation of amygdalar eCB signaling is essential for the adaptation of fear behaviors(Marsicano et al., 2002).This observation was subsequently extended to nonassocia-tive fear,where mice exposed to a brief inescapable electric foot shock showed a considerable fear response to a subsequently pre-sented neutral tone(Kamprath and Wotjak,2004).However, whereas this fear behavior was rapidly suppressed in wild-type mice,CB1receptor-deficient mice failed to suppress the condi-tioned fear response(Kamprath et al.,2006).Specifically,mice lacking CB1receptors showed persistent fear on repeated tone exposures,indicating that the CB1receptor is essential not only for within-,but also for between-session habituation(Kamprath et al.,2006).The fear dampening effects of eCBs become evident only in highly aversive situations and are independent of CRH and corticosterone action(Kamprath et al.,2009).Similar to the hypothesized mechanism of HPA axis regula-tion,experiments with conditional mouse CB1receptor mutants reveal that eCBs exert their effects on fear adaptation primarily via CB1receptors expressed on cortical glutamatergic neurons (Kamprath et al.,2009).As increased eCB signaling within the amygdala is coupled to both fear extinction(Marsicano et al., 2002)and HPA axis habituation(Hill et al.,2010b),these data suggest that CB1receptor-mediated regulation of cortical gluta-matergic inputs to BLA neurons is likely the nexus of the eCB contribution to both neuroendocrine and behavioral adaptation to stress.SummaryThe material reviewed in this mini-symposium demonstrates an increasingly complex role of eCB signaling in multiple facets of the stress response.With respect to the HPA axis,eCB regulation of glutamatergic transmission in both the PVN and the BLA ap-pears to be critical for the suppression of the HPA axis under conditions of fast-feedback inhibition and habituation following repeated stress,respectively.Temporal studies demonstrate an interesting dual component role of eCBs within the HPA axis. Specifically,a rapid loss of AEA within the BLA following stress exposure promotes activation of the HPA axis,whereas a glucocorticoid-mediated elevation in2-AG in the PVN contrib-utes to fast-feedback inhibition of the HPA axis.Adding to this complexity is the emerging role of eCB signaling in the BLA in the habituation of the HPA axis response to repeated stress.The eCB system,therefore,is clearly intertwined with HPA axis function, under both acute and chronic conditions.In addition to regulation of the HPA axis,however,eCB sig-naling also contributes to higher-order responses to stress,such as emotional and cognitive responses.As an extension of the role of eCB signaling in the adaptation of the HPA axis to repeated stress,eCBs are also required for the suppression of behavioral responses to aversive stimuli following their removal or repeated presentation.Furthermore,the recruitment of eCB signaling within the amygdala by glucocorticoids mediates their effects on the consolidation of emotional memory.Together,these data clearly point to the integration of eCBs into the stress response.Ongoing research will help us to delineate the extent to which eCB signaling is engaged by stress in different stress-related CNS structures,and how deficits in this system may contribute to stress-related mental disorders,such as de-pression and post-traumatic stress disorder(Hill and Gorzalka, 2009).14984•J.Neurosci.,November10,2010•30(45):14980–14986Hill et al.•Endocannabinoids and the Stress Response。