工程计算手册(桥梁工程)
路桥施工计算手册pdf
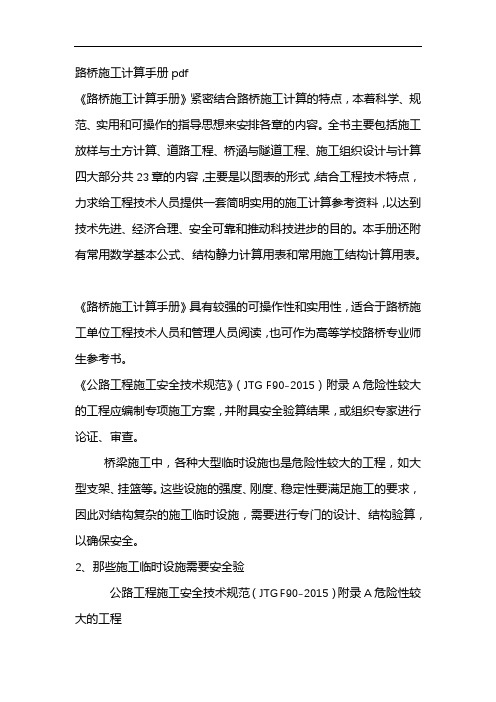
路桥施工计算手册pdf《路桥施工计算手册》紧密结合路桥施工计算的特点,本着科学、规范、实用和可操作的指导思想来安排各章的内容。
全书主要包括施工放样与土方计算、道路工程、桥涵与隧道工程、施工组织设计与计算四大部分共23章的内容,主要是以图表的形式,结合工程技术特点,力求给工程技术人员提供一套简明实用的施工计算参考资料,以达到技术先进、经济合理、安全可靠和推动科技进步的目的。
本手册还附有常用数学基本公式、结构静力计算用表和常用施工结构计算用表。
《路桥施工计算手册》具有较强的可操作性和实用性,适合于路桥施工单位工程技术人员和管理人员阅读,也可作为高等学校路桥专业师生参考书。
《公路工程施工安全技术规范》(JTG F90-2015)附录A危险性较大的工程应编制专项施工方案,并附具安全验算结果,或组织专家进行论证、审查。
桥梁施工中,各种大型临时设施也是危险性较大的工程,如大型支架、挂篮等。
这些设施的强度、刚度、稳定性要满足施工的要求,因此对结构复杂的施工临时设施,需要进行专门的设计、结构验算,以确保安全。
2、那些施工临时设施需要安全验公路工程施工安全技术规范(JTG F90-2015)附录A危险性较大的工程大型临时工程:大型临时设施会用到钢结构、模板工程、脚手架、贝雷架等各种材料,验算时要满足相关规范的要求。
《建筑施工扣件式钢管脚手架安全技术规范》JGJ 130-2001;《建筑施工碗扣式脚手架安全技术规范》》JGJ166-2016;《建筑施工承插型盘扣式钢管支架安全技术规程》JGJ231-2010;《建筑工程大模板技术规范》JGJ 74-2003;《建筑施工模板安全技术规程》JGJ 162-2008;《路桥施工计算手册》、《建筑施工计算手册》;《公路桥涵施工技术规范》JTG/T F50-2011;《钢结构设计规范》GB50017-2017.。
桥梁工程量计算表
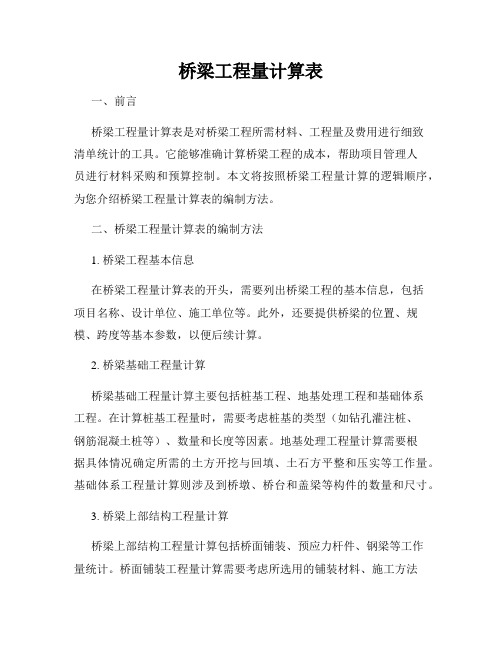
桥梁工程量计算表一、前言桥梁工程量计算表是对桥梁工程所需材料、工程量及费用进行细致清单统计的工具。
它能够准确计算桥梁工程的成本,帮助项目管理人员进行材料采购和预算控制。
本文将按照桥梁工程量计算的逻辑顺序,为您介绍桥梁工程量计算表的编制方法。
二、桥梁工程量计算表的编制方法1. 桥梁工程基本信息在桥梁工程量计算表的开头,需要列出桥梁工程的基本信息,包括项目名称、设计单位、施工单位等。
此外,还要提供桥梁的位置、规模、跨度等基本参数,以便后续计算。
2. 桥梁基础工程量计算桥梁基础工程量计算主要包括桩基工程、地基处理工程和基础体系工程。
在计算桩基工程量时,需要考虑桩基的类型(如钻孔灌注桩、钢筋混凝土桩等)、数量和长度等因素。
地基处理工程量计算需要根据具体情况确定所需的土方开挖与回填、土石方平整和压实等工作量。
基础体系工程量计算则涉及到桥墩、桥台和盖梁等构件的数量和尺寸。
3. 桥梁上部结构工程量计算桥梁上部结构工程量计算包括桥面铺装、预应力杆件、钢梁等工作量统计。
桥面铺装工程量计算需要考虑所选用的铺装材料、施工方法以及路面的面积和厚度等因素。
预应力杆件和钢梁的工程量计算则需要根据具体设计方案确定其数量和长度。
4. 桥梁附属设施工程量计算桥梁附属设施工程量计算主要包括护栏、栏杆、人行道和排水系统等。
护栏和栏杆的工程量计算需要考虑其材料、长度和安装方式等因素。
人行道的工程量计算则需根据其面积和厚度等参数来进行统计。
排水系统工程量计算涉及到桥梁上的雨水口、排水管和排水沟等。
5. 其他工程量计算在完成以上主要工程量计算后,还需对桥梁工程中的其他部分进行统计。
这些部分包括临时支撑、施工附属设备、交通安全设施等。
根据具体情况,结合工程实际需求,进行详细的工程量计算。
6. 总结及填表最后,根据以上工程量计算的结果,将所有数据归纳并依次填入桥梁工程量计算表中。
确保各项数据的准确性和一致性,以便后续的预算控制和材料采购工作。
工程计算手册(桥梁工程)课件.doc
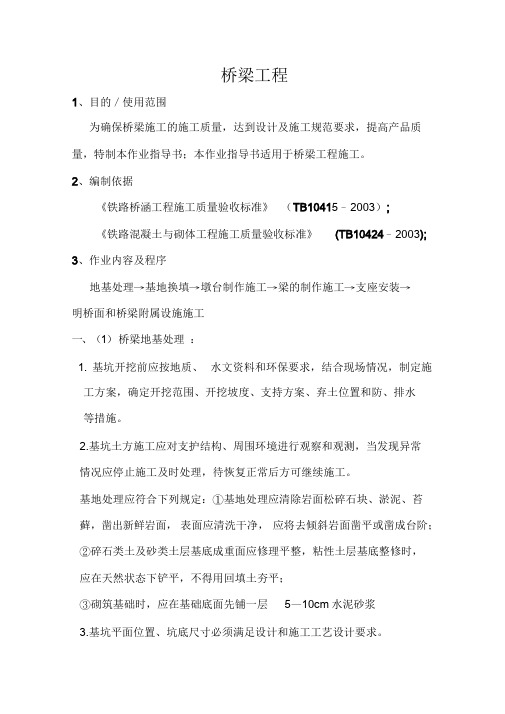
桥梁工程1、目的/使用范围为确保桥梁施工的施工质量,达到设计及施工规范要求,提高产品质量,特制本作业指导书;本作业指导书适用于桥梁工程施工。
2、编制依据《铁路桥涵工程施工质量验收标准》(TB10415–2003);《铁路混凝土与砌体工程施工质量验收标准》(TB10424–2003);3、作业内容及程序地基处理→基地换填→墩台制作施工→梁的制作施工→支座安装→明桥面和桥梁附属设施施工一、(1)桥梁地基处理:1. 基坑开挖前应按地质、水文资料和环保要求,结合现场情况,制定施工方案,确定开挖范围、开挖坡度、支持方案、弃土位置和防、排水等措施。
2.基坑土方施工应对支护结构、周围环境进行观察和观测,当发现异常情况应停止施工及时处理,待恢复正常后方可继续施工。
基地处理应符合下列规定:①基地处理应清除岩面松碎石块、淤泥、苔藓,凿出新鲜岩面,表面应清洗干净,应将去倾斜岩面凿平或凿成台阶;②碎石类土及砂类土层基底成重面应修理平整,粘性土层基底整修时,应在天然状态下铲平,不得用回填土夯平;③砌筑基础时,应在基础底面先铺一层5—10cm 水泥砂浆3.基坑平面位置、坑底尺寸必须满足设计和施工工艺设计要求。
4. 基坑开挖方式和支护必须满足设计要求。
5.基地地质条件必须满足设计要求。
基底高程的允许偏差和检验方法:序号地质类别允许偏差检验方法1 土±50测量检查+502 石—200(2)、基坑回填填料1.基坑回填填料应符合设计要求,夯实应符合规定。
2.换填地基所用材料必须符合下列规定:换填用砂应为中粗砂,有机质和泥量均不得大于5%;碎石粒径不得大于100mm,含泥量不得大于5%;石灰等级不得小于Ⅲ级。
3.换填范围必须符合设计要求。
4 填料比例必须符合设计要求。
5.填筑和压实工艺必须符合设计和施工技术方案的要求。
6.压实密度必须符合设计要求。
换填地基和顶部高程允许偏差为±50 mm。
二、墩台制作施工(1)钢筋加工绑扎1.钢筋在运输、加工和储存过程中应防止锈蚀、污染和变形。
桥梁设计常用数据手册

桥梁设计常用数据手册一、前言桥梁设计是土木工程中的重要组成部分,其涉及的数据与参数种类繁多,需要工程师在设计过程中经常使用。
为了方便工程师们能够更快捷、准确地获取和应用相关数据,编制一份桥梁设计常用数据手册是十分必要的。
本手册将包括桥梁设计中常用的材料性能、桥梁结构计算参数、设计规范等内容,力求全面、实用,为桥梁设计工程师提供一份便捷的参考资料。
二、常用材料性能数据1. 钢材性能数据:包括强度、屈服点、弹性模量等指标;2. 混凝土性能数据:包括抗压强度、抗拉强度、弹性模量等指标;3. 橡胶支座性能数据:包括承载能力、压缩性能、弹性模量等指标;4. 沥青材料性能数据:包括黏度、温度变化性能等指标;5. 锚杆材料性能数据:包括抗拉强度、弯矩承载能力等指标。
以上材料性能数据是桥梁设计中常用的基础数据,设计者需要根据具体工程需求进行选取和应用。
三、桥梁结构计算相关参数1. 桥梁荷载参数:包括静载和动载情况下的车辆荷载、行人荷载等;2. 结构受力参数:包括桥梁自重、横向风荷载、温度变化引起的应力等;3. 桥梁挠度和扭曲参数:包括跨中挠度允许值、横向扭曲应变等;4. 桥梁抗震参数:包括地震作用下的设计加速度、地震反应系数等;5. 桥梁基础承载力设计参数:包括土壤承载力、桩基承载力等。
以上桥梁结构计算相关参数是桥梁设计中常用的计算数据,设计者需要合理地应用这些参数进行结构计算和验证。
四、设计规范1. 桥梁设计规范:包括国家桥梁设计规范、国际桥梁设计规范等;2. 建筑法规标准:包括有关桥梁设计的建筑法规标准;3. 材料标准:包括钢材、混凝土、橡胶支座等材料的标准规范;4. 施工规范:包括桥梁施工过程中的相关规范标准;5. 安全规范:包括桥梁设计和施工中的安全操作规范。
设计规范是桥梁设计工程中不可或缺的指导性文件,设计者需要充分了解并遵守相关规范要求。
五、总结桥梁设计常用数据手册是桥梁设计工程师在实际设计中的重要参考资料,涵盖了材料性能数据、结构计算相关参数和设计规范等内容。
桥梁工程圆柱墩模板计算书
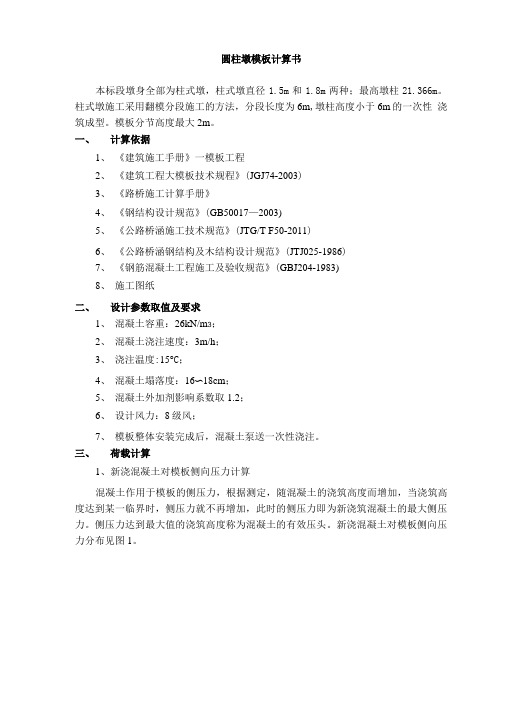
圆柱墩模板计算书本标段墩身全部为柱式墩,柱式墩直径1.5m和1.8m两种;最高墩柱21.366m。
柱式墩施工采用翻模分段施工的方法,分段长度为6m,墩柱高度小于6m的一次性浇筑成型。
模板分节高度最大2m。
一、计算依据1、《建筑施工手册》一模板工程2、《建筑工程大模板技术规程》(JGJ74-2003)3、《路桥施工计算手册》4、《钢结构设计规范》(GB50017—2003)5、《公路桥涵施工技术规范》(JTG/T F50-2011)6、《公路桥涵钢结构及木结构设计规范》(JTJ025-1986)7、《钢筋混凝土工程施工及验收规范》(GBJ204-1983)8、施工图纸二、设计参数取值及要求1、混凝土容重:26kN/m3;2、混凝土浇注速度:3m/h;3、浇注温度:15°C;4、混凝土塌落度:16〜18cm;5、混凝土外加剂影响系数取1.2;6、设计风力:8级风;7、模板整体安装完成后,混凝土泵送一次性浇注。
三、荷载计算1、新浇混凝土对模板侧向压力计算混凝土作用于模板的侧压力,根据测定,随混凝土的浇筑高度而增加,当浇筑高度达到某一临界时,侧压力就不再增加,此时的侧压力即为新浇筑混凝土的最大侧压力。
侧压力达到最大值的浇筑高度称为混凝土的有效压头。
新浇混凝土对模板侧向压力分布见图1。
图1新浇混凝土对模板侧向压力分布图按照《建筑工程大模板技术规程》(JGJ74-2003)附录B,新浇筑的混凝土作用于模板的最大侧压力,可按下列两式计算,并取其最小值:F = 0.227 t P P 心/2 F =丫Hc 0 1 2 c式中:F -----新浇筑混凝土对模板的最大侧压力(KN/m2)。
Y ----混凝土的重力密度(kN/m3),根据设计图纸取26kN/m3。
ct0---------- 新浇混凝土的初凝时间(h),可按实测确定,当缺乏实验资料时,可采用t=200/(T+15)计算,取t=5h。
0T -----混凝土的温度(25° C)。
大桥支架计算书(完整经典版)
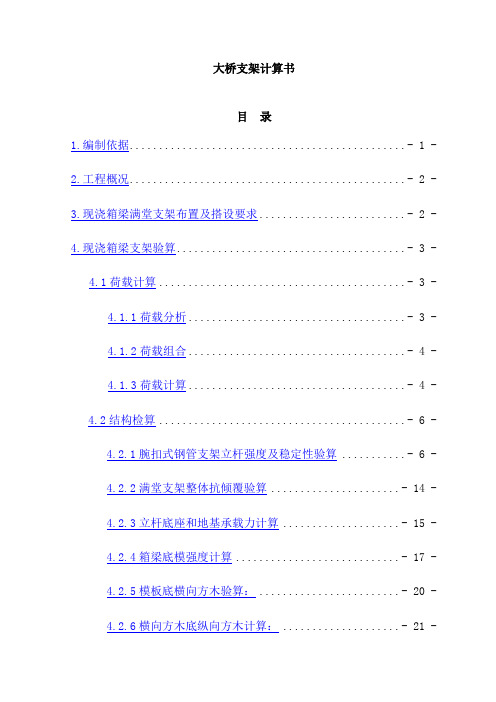
大桥支架计算书目录1.编制依据............................................... - 1 -2.工程概况............................................... - 2 -3.现浇箱梁满堂支架布置及搭设要求......................... - 2 -4.现浇箱梁支架验算....................................... - 3 -4.1荷载计算.......................................... - 3 -4.1.1荷载分析..................................... - 3 -4.1.2荷载组合..................................... - 4 -4.1.3荷载计算..................................... - 4 -4.2结构检算.......................................... - 6 -4.2.1腕扣式钢管支架立杆强度及稳定性验算 ........... - 6 -4.2.2满堂支架整体抗倾覆验算...................... - 14 -4.2.3立杆底座和地基承载力计算.................... - 15 -4.2.4箱梁底模强度计算............................ - 17 -4.2.5模板底横向方木验算:........................ - 20 -4.2.6横向方木底纵向方木计算:.................... - 21 -西一大桥主梁现浇箱梁模板及满堂支架方案计算书1.编制依据1.1亚行贷款酒泉市城市环境综合治理项目的有关投标文件。
桥梁工程课程设计报告计算书

桥梁工程课程设计及计算书设计题目: 桥梁工程课程设计学院:土木与建筑学院指导老师:汪峰姓名:学号:班级:2014年6月一、基本资料1.标准跨径:20 m计算跨径:19.50 m主梁全长:19.96 m2.桥面净宽:净7.5 m+2×0.25 m3. 车辆荷载:公路— 级4. 人群荷载:3.0 KN/m²5. 选用材料:钢筋:采用HRB300钢筋,HRB335钢筋。
混凝土:主梁C40人行道及栏杆:C25桥面铺装:C25(重度24KN/m)6. 课程设计教材及主要参考资料:《桥梁工程》.玲森编.人民交通出版社,1990年《桥梁工程》.邵旭东等编.人民交通出版社,2007年《桥梁工程》.立础编.人民交通出版社,2001年《简支梁桥示例集》.易建国编.人民交通出版社,2000年《桥梁工程课程设计指导书》.桥梁教研室.工业大学教材科,2002年《梁桥设计手册》.桥梁编辑组.人民交通出版社,1990年《公路桥涵设计通用规》(JTG D60-2004)人民交通出版社北京《拱桥设计手册(上、下)》.桥梁编辑组.人民交通出版社,1990年《配筋混凝土结构设计原理》袁国干主编,同济大学出版社二、桥梁尺寸拟定1.主梁高度:h=1.5m梁间距:采用5片主梁,间距1.8m。
2.横隔梁:采用五片横隔梁,间距为4×4.85m,梁高1.0m, 横隔梁下缘为15cm,上缘为16cm。
3.主梁梁肋宽:梁肋宽度为18cm。
4.桥面铺装:分为上下两层,上层为沥青砼厚2.0cm, 下层为C25防水混凝土垫层厚10.0cm。
桥面采用1.5%横坡。
5.桥梁横断面及具体尺寸:(见作图)6.桥梁纵断面及具体尺寸:(见作图)三、桥梁计算 一、主梁的计算1、主梁的抗弯及抗扭惯性矩x I 、TiI求主梁界面的重心位置x a (图3) 、平均板厚:H=1/2(10+18)=14(cm )cm x 93.452005141)12081(20512005124141)20081(a =⨯+⨯-⨯⨯+⨯⨯-=442323m 415481019.0cm 94.1548101445.93-21501502015020121214-45.931416014160121==⨯⨯+⨯⨯+⨯⨯+⨯⨯=x I43313517m 00.081.0.1613036.00.141.803333.0=⨯⨯+⨯⨯==∑=i i Mi i Ti t b c I2.计算结构自重集度(表1)结构自重集度计算表表13.结构自重力计算(表2)边中主梁自重产生的力 表2主:括号()值为中主梁力3.汽车、人群荷载力计算(1)支点处荷载横向分布系数(杠杆原理法)汽车荷载距人行道边缘不小于0.5m 。
Bridge engineering handbook桥梁工程手册-19斜拉桥
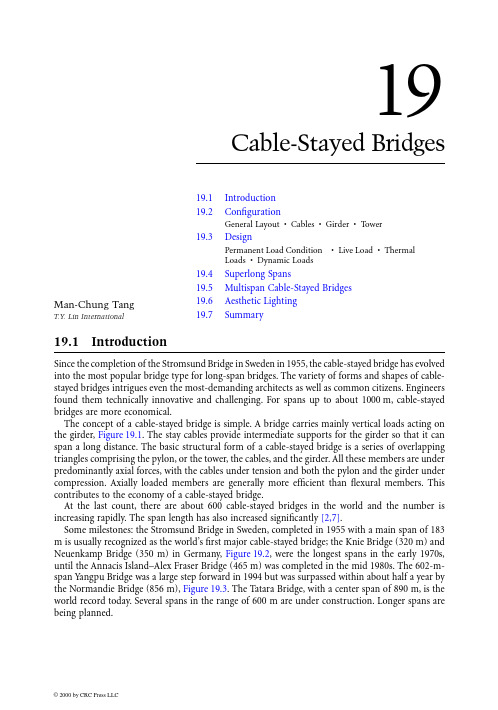
19Cable-Stayed Bridges19.1 Introduction19.2 ConfigurationGeneral Layout •Cables •Girder •Tower19.3 DesignPermanent Load Condition•Live Load •ThermalLoads •Dynamic Loads 19.4 Superlong Spans 19.5 Multispan Cable-Stayed Bridges 19.6 Aesthetic Lighting 19.7SummarySince the completion of the Stromsund Bridge in Sweden in 1955, the cable-stayed bridge has evolved into the most popular bridge type for long-span bridges. The variety of forms and shapes of cable-stayed bridges intrigues even the most-demanding architects as well as common citizens. Engineers found them technically innovative and challenging. For spans up to about 1000 m, cable-stayed bridges are more economical.The concept of a cable-stayed bridge is simple. A bridge carries mainly vertical loads acting on the girder, Figure 19.1. The stay cables provide intermediate supports for the girder so that it can span a long distance. The basic structural form of a cable-stayed bridge is a series of overlapping triangles comprising the pylon, or the tower, the cables, and the girder. All these members are under predominantly axial forces, with the cables under tension and both the pylon and the girder under compression. Axially loaded members are generally more efficient than flexural members. This contributes to the economy of a cable-stayed bridge.At the last count, there are about 600 cable-stayed bridges in the world and the number is increasing rapidly. The span length has also increased significantly [2,7].Some milestones: the Stromsund Bridge in Sweden, completed in 1955 with a main span of 183m is usually recognized as the world’s first major cable-stayed bridge; the Knie Bridge (320 m) and Neuenkamp Bridge (350 m) in Germany, Figure 19.2, were the longest spans in the early 1970s,until the Annacis Island–Alex Fraser Bridge (465 m) was completed in the mid 1980s. The 602-m-span Yangpu Bridge was a large step forward in 1994 but was surpassed within about half a year by the Normandie Bridge (856 m), Figure 19.3. The Tatara Bridge, with a center span of 890 m, is the world record today. Several spans in the range of 600 m are under construction. Longer spans are being planned.Man-Chung TangT.Y. Lin InternationalFIGURE 19.1Concept of a cable-stayed bridge.FIGURE 19.2Neuenkamp Bridge.FIGURE 19.3Normandie Bridge.19.2.1General LayoutAt the early stage, the idea of a cable-stayed bridge was to use cable suspension to replace the piers as intermediate supports for the girder so that it could span a longer distance. Therefore, early cable-stayed bridges placed cables far apart from each other based on the maximum strength of the girder.This resulted in rather stiff girders that had to span the large spacing between cables, in addition to resisting the global forces.The behavior of a cable-stayed girder can be approximately simulated by an elastically supported girder. The bending moment in the girder under a specific load can be thought of as consisting of a local component and a global component. The local bending moment between the cables is proportional to the square of the spacing. The global bending moment of an elastically supported girder is approximately [5](19.1)where a is a coefficient depending on the type of load p, I is the moment of inertia of the girder,and k is the elastic support constant derived from the cable stiffness. The global moment decreases as the stiffness of girder , I, decreases.Considering that the function of the cables is to carry the loads on the bridge girder, which remains the same, the total quantity of cables required for a bridge is practically the same indepen-dent of the number of cables, or cable spacing, Figure 19.4. But if the cable spacing is smaller, the local bending moment of the girder between the cables is also smaller. A reduction of the local bending moment allows the girder to be more flexible. A more flexible girder attracts in turn less global moment. Consequently, a very flexible girder can be used with closely spaced cables in many modern cable-stayed bridges. The Talmadge Bridge, Savannah, Figure 19.5, is 1.45 m deep for a 335m span, The ALRT Skytrain Bridge, Vancouver, Figure 19.6, is 1.1 m deep for a 340 m span and the design of the Portsmouth Bridge had a 84-cm-deep girder for a span of 286 m.Because the girder is very flexible, questions concerning buckling stability occasionally arose at the beginning. However, as formulated by Tang [4], Eq. (19.2), using the energy method,(19.2)where E is modulus of elasticity, I is moment of inertia, A is area, L is length , w is deflection, and ( )′is derivative with respect to length s. The buckling load depends more on the stiffness of the cables than on the stiffness of the girder. Theoretically, even if the stiffness of the girder is neglected, a cable-stayed bridge can still be stable in most cases. Experience shows that even for the most flexible girder, the critical load against elastic buckling is well over 400% of the actual loads of the bridge.FIGURE 19.4Cable forces in relation to load on girder.P cr EIw ds EC Ac Lc Ps Pc w ds ()=′′+∗∗()′ ∑∫∫22The recently adopted design requirement that all cables be individually replaceable makes closely spaced cables more desirable. It is usually required that one cable can be detensioned, dismantled,and replaced under reduced traffic loading. The additional bending moment in the girder will not increase excessively if the cable spacing is small.Availability of ever more powerful computers also helps. The complexity of the analysis increases as the number of cables increases. The computer offers engineers the best tool to deal with this problem.Harp, radial, fan, Figure 19.7, or other cable configurations have all been used. However, except in very long span structures, cable configuration does not have a major effect on the behavior of the bridge.A harp-type cable arrangement offers a very clean and delicate appearance because an array of parallel cables will always appear parallel irrespective of the viewing angle. It also allows an earlier FIGURE 19.5Talmadge Bridge.FIGURE 19.6ALRT Skytrain Bridge.FIGURE 19.7Cable arrangements.start of girder construction because the cable anchorages in the tower begin at a lower elevation.The Hoechst Bridge and the Dames Point Bridge are examples that fully utilized this advantage.A fan-type cable arrangement can also be very attractive, especially for a single-plane cable system.Because the cable slopes are steeper, the axial force in the girder, which is an accumulation of all horizontal components of cable forces, is smaller. This feature is advantageous for longer-span bridges where compression in the girder may control the design. The Nord Bridge, Bonn,Figure 19.8, is one of the first of this type.A radial arrangement of cables with all cables anchored at a common point at the tower is quite efficient. However, a good detail is difficult to achieve. Unless it is well treated, it may look clumsy.The Ludwighafen Bridge, Germany, Figure 19.9, is a successful example. The Yelcho Bridge, Chile,with all cables anchored in a horizontal plane in the tower top, is an excellent solution, both technically and aesthetically.When the Stromsund Bridge was designed, long-span bridges were the domain of steel construc-tion. Therefore, most early cable-stayed bridges were steel structures. They retained noticeable features from other types of long-span steel bridges.In the 1960s, Morandi designed and built several relatively long span concrete cable-stayed bridges. His designs usually had few cables in a span with additional strut supports at the towers for the girder. They did not fully utilize the advantages of a cable-stayed system. The concrete cable-stayed bridge in its modern form started with the Hoechst Bridge in Germany, followed by the Brotone Bridge in France and the Dames Point Bridge in the United States, each representing a significant advance in the state of the art.FIGURE 19.8Nord Bridge.FIGURE 19.9Ludwighafen Bridge.19.2.2CablesCables are the most important elements of a cable-stayed bridge. They carry the load of the girder and transfer it to the tower and the back-stay cable anchorage.The cables in a cable-stayed bridge are all inclined, Figure 19.10. The actual stiffness of an inclined cable varies with the inclination angle, a, the total cable weight, G, and the cable tension force , T [3]:(19.3)where E and A are Young’s modulus and the cross-sectional area of the cable. And if the cable tension T changes from T 1 to T 2, the equivalent cable stiffness will be(19.4)In most cases, the cables are tensioned to about 40% of their ultimate strength under permanent load condition. Under this kind of tension, the effective cable stiffness approaches the actual values,except for very long cables. However, the tension in the cables may be quite low during some construction stages so that their effectiveness must be properly considered.A safety factor of 2.2 is usually recommended for cables. This results in an allowable stress of 45% of the guaranteed ultimate tensile strength (GUTS) under dead and live loads [9]. It is prudent to note that the allowable stress of a cable must consider many factors, the most important being the strength of the anchorage assemblage that is the weakest point in a cable with respect to capacity and fatigue behavior.There have been significant developments in the stay cable system. Early cables were mainly lock-coil strands. At that time, the lock-coil strand was the only cable system available that could meet the more stringent requirements of cable-stayed bridges.Over the years, many new cable systems have been successfully used. Parallel wire cables with Hi-Am sockets were first employed in 1969 on the Schumacher Bridge in Mannheim, Germany.Since then, the fabrication technique has been improved and this type cable is still one of the best cables commercially available today. A Hi-Am socket has a conical steel shell. The wires are parallel for the entire length of the cable. Each wire is anchored to a plate at the end of the socket by a button head. The space in the socket is then filled with epoxy mixed with zinc and small steel balls.The Hi-Am parallel wire cables are prefabricated to exact length in the yard and transported to the site in coils. Because the wires are parallel and therefore all of equal length, the cable may FIGURE 19.10An inclined cable.EA EA G EA a T eff ()=+(){}112223cos EA EA G EA a T T T T eff ()=++()(){}11224122222cossometimes experience difficulty in coiling. This difficulty can be overcome by twisting the cable during the coiling process. To avoid this problem altogether, the cables can be fabricated with a long lay. However, the long lay may cause a very short cable to twist during stressing. Threadbar tendons were used for some stay cables. The first one was for the Hoechst Bridge over the Main River in Germany. The Penang Bridge and the Dames Point Bridge also have bar cables. They all have a steel pipe with cement grout as corrosion protection. Their performance has been excellent. The most popular type of cable nowadays uses seven-wire strands. These strands, originally developed for prestressed concrete applications, offer good workability and economy. They can either be shop-fabricated or site-fabricated. In most cases, corrosion protection is provided by a high-density polyethylene pipe filled with cement grout. The technique of installation has progressed to a point where a pair of cables can be erected at the site in 1 day.In search of better corrosion protection, especially during the construction stage before the cables have been grouted, various alternatives, such as epoxy coating, galvanization, wax and grease have all been proposed and used. Proper coating of strands must completely fill the voids between the wires with corrosion inhibitor. This requires the wires to be loosened before the coating process takes place and then retwisted into the strand configuration.In addition to epoxy, grease, or galvanization, the strands may be individually sheathed. A sheathed galvanized strand may have wax or grease inside the sheathing. All three types of additional protection appear to be acceptable and should perform well. However, a long-term performance record is not yet available.The most important element in a cable is the anchorage. In this respect, the Hi-Am socket has an excellent performance record. Strand cables with bonded sockets, similar to the Hi-Am socket, have also performed very well. In a recently introduced unbonded anchorage, all strands are being held in place only by wedges. Tests have confirmed that these anchorages meet the design require-ments. But unbonded strand wedges are delicate structural elements and are susceptible to con-struction deviations. Care must be exercised in the design, fabrication, and installation if such an anchorage is to be used in a cable-stayed bridge. The advantage of an unbonded cable system is that the cable, or individual strands, can be replaced relatively easily.Cable anchorage tests have shown that, in a bonded anchor, less than half of the cyclic stress is transferred to the wedges. The rest is dissipated through the filling and into the anchorage directly by bond. This is advantageous with respect to fatigue and overloading.The Post Tensioning Institute’s “Recommendations for Stay Cable Design and Testing,” [9] was published in 1986. This is the first uniformly recognized criteria for the design of cables. In con-junction with the American Society of Civil Engineers’ “Guidelines for the Design of Cable-Stayed Bridges” [10], they give engineers a much-needed base to start their design.There have been various suggestions for using composite materials such as carbon fiber, etc. as stays and small prototypes have been built. However, actual commercial application still requires further research and development.19.2.3GirderAlthough the Stromsund Bridge has a concrete deck, most other early cable-stayed bridges have an orthotropic deck. This is because both cable-stayed bridge and orthotropic deck were introduced to the construction industry at about the same time. Their marriage was logical. The fact that almost all long-span bridges were built by steel companies at that time made such a choice more under-standable.A properly designed and fabricated orthotropic deck is a good solution for a cable-stayed bridge. However, with increasing labor costs, the orthotropic deck becomes less commercially attractive except for very long spans.Many concrete cable-stayed bridges have been completed. In general, there have been two major developments: cast-in-place construction and precast construction.Cast-in-place construction of cable-stayed bridges is a further development of the free cantilever construction method of box-girder bridges. The typical construction is by means of a form traveler.The box girder is a popular shape for the girder in early structures, such as the Hoechst Bridge, the Barrios de Luna Bridge, and the Waal Bridge. But simpler cross sections have proved to be attractive:the beam and slab arrangement in the Penang Bridge, the Dames Point Bridge, and the Talmadge Bridge, or the solid slab cross section as in the Y elcho Bridge, the Portsmouth Bridge, and the Diepold-sau Bridge are both technically sound and economical. Use of the newly developed cable-supported form traveler, Figure 19.11, makes this type girder much more economical to build [8].Precast construction can afford a slightly more complicated cross section because precasting is done in the yard. The segments, however, should all be similar to avoid adjustment in the precasting forms. The weight of the segment is limited by the transportation capability of the equipment used.Box is the preferred cross section because it is stiffer and easier to erect. The Brotonne Bridge, the Sunshine Skyway Bridge, Figure 19.12, and the Chesapeake and Delaware Canal Bridge are good examples. However, several flexible girder cable-stayed bridges have been completed successfully,notably the Pasco–Kennewick Bridge, the East Huntington Bridge, and the ALRT Skytrain Bridge.As concrete technology advances, today’s cable-stayed bridges may consider using high-strength lightweight concrete for the girder, especially in high seismic areas.Although the steel orthotropic deck is too expensive for construction in most countries at this time, the composite deck with a concrete slab on a steel frame can be very competitive. In the Stromsund Bridge, the concrete deck was not made composite with the steel girder. Such a construc-tion is not economical because the axial compressive force in the girder must be taken entirely by FIGURE 19.11Form traveler of the Dames Point Bridge.FIGURE 19.12Sunshine Skyway Bridge – precast box and erection.the steel girder. Making the deck composite with the steel girder by shear studs reduces the steel quantity of the girder significantly. The compressive stress in the concrete deck also improves the performance of the deck slab. The Hootley Bridge was the first major composite bridge designed,but the Annacis Island Bridge was completed first. The Yang Pu Bridge is the longest span today.The Baytown–LaPorte Bridge, Figure 19.13, has the largest deck area.Precast slab panels are usually used for composite bridges. Requiring the precast panels to be stored for a period of time, say, 90 days, before erection reduces the effect of creep and shrinkage significantly. The precast panels are supported by floor beams and the edge girders during erection.The gaps between the panels are filled with nonshrinking concrete. The detail for these closure joints must be carefully executed to avoid cracking due to shrinkage and other stresses.Most portions of the girder are under high compression, which is good for concrete members.However, tensile stresses may occur in the middle portion of the center span and at both ends of the end spans. Post-tensioning is usually used in these areas to keep the concrete under compression.Several hybrid structures, with concrete side spans and steel main span, such as the Flehe Bridge,Germany and the Normandie Bridge, have been completed. There are two main reasons for the hybrid combination: to have heavier, shorter side spans to balance the longer main span or to build the side spans the same way as the connecting approaches. The transition, however, must be carefully detailed to avoid problems.19.2.4TowerThe towers are the most visible elements of a cable-stayed bridge. Therefore, aesthetic considerations in tower design is very important. Generally speaking, because of the enormous size of the structure,FIGURE 19.13Baytown–LaPorte Bridge and Yang Pu Bridge.a clean and simple configuration is preferable. The free-standing towers of the Nord Bridge and the Knie Bridge look very elegant. The H towers of the Annacis Bridge, the Talmadge Bridge, and the Nan Pu Bridge are the most logical shape structurally for a two-plane cable-stayed bridge,Figure 19.14. The A shape (as in the East Hungtington Bridge), the inverted Y (as in the Flehe Bridge), and the diamond shape (as in the Baytown–LaPorte and Yang Pu Bridges) are excellent choices for long-span cable-stayed bridges with very flexible decks. Other variations in tower shape are possible as long as they are economically feasible. Under special circumstances, the towers can also serve as tourist attractions such as the one proposed for the Dan Chiang Bridge in Taiwan.FIGURE 19.14Tower shapes — H (Talmadge), inverted Y (Flehe), diamond (Glebe), double diamond (Baytown),and special configuration (Dan Chiang).Although early cable-stayed bridges all have steel towers, most recent constructions have concrete towers. Because the tower is a compression member, concrete is the logical choice except under special conditions such as in high earthquake areas.Cables are anchored at the upper part of the tower. There are generally three concepts for cable anchorages at the tower: crisscrossing, dead-ended, and saddle.Early cable-stayed bridges took their anchorage details from suspension bridges that have saddles.Those saddles were of the roller, fixed, or sector types. Roller-type saddles have a roller at the base similar to a bridge bearing. Fixed-type saddles are similar except the base is fixed instead of rolling.Sector-type saddles rotate around a pin. Each of these saddles satisfies a different set boundary condition in the structural system. Cable strands, basically lock-coil strands, were placed in the saddle trough as in a suspension bridge. To assure that the strands do not slide under unequal tension, a cover plate is usually clamped to the saddle trough to increase friction. This transverse pressure increases friction but reduces the strength of the cable. This type of saddle is very expensive and has not been used for recent cable-stayed bridges.A different type of saddle was used in the Brotonne Bridge and the Sunshine Skyway Bridge.Here the seven-wire strands were bundled into a cable and then pulled through a steel saddle pipe which was fixed to the tower. Grouting the cable fixed the strands to the saddle pipe. However, the very high contact pressure between the strand wires must be carefully considered and dealt with in the design. Because the outer wires of a strand are helically wound around a straight, center king wire, the strands in a curved saddle rest on each other with point contacts. The contact pressure created by the radial force of the curved strands can well exceed the yield strength of the steel wires.This can reduce the fatigue strength of the cable significantly.Crisscrossing the cables at the tower is a good idea in a technical sense. It is safe, simple, and economical. The difficulty is in the geometry. To avoid creating torsional moment in the tower column, the cables from the main span and the side span should be anchored in the tower in the same plane, Figure 19.15. This, however, is physically impossible if they crisscross each other. One solution is to use double cables so that they can pass each other in a symmetrical pattern as in the case of the Hoechst Bridge. If A-shaped or inverted-Y-shaped towers are used, the two planes of cables can also be arranged in a symmetrical pattern.If the tower cross section is a box, the cables can be anchored at the front and back wall of the tower, Figure 19.16. Post-tensioning tendons are used to prestress the walls to transfer the anchoring forces from one end wall to the other. The tendons can be loop tendons that wrap around three side walls at a time or simple straight tendons in each side wall independently. The Talmadge,Baytown–LaPorte, and Yang Pu Bridges all employ such an anchoring detail. During the design of the Yang Pu Bridge, a full-scale model was made to confirm the performance of such a detail.As an alternative, some bridges have the cables anchored to a steel member that connects the cables from both sides of the tower. The steel member may be a beam or a box. It must be connected FIGURE 19.15Crisscrossing cables at tower column.to the concrete tower by shear studs or other means. This anchorage detail simulates the function of the saddle. However, the cables at the opposite sides are independent cables. The design musttherefore consider the loading condition when only one cable exists.19.3.1Permanent Load ConditionA cable-stayed bridge is a highly redundant, or statically indeterminate structure. In the design of such a structure, the treatment of the permanent load condition is very important. This load condition includes all structural dead load and superimposed dead load acting on the structure, all prestressing effects as well as all secondary moments and forces. It is the load condition when all permanent loads act on the structure.Because the designer has the liberty to assign a desired value to every unknown in a statically indeterminate structure, the bending moments and forces under permanent load condition can be determined solely by the requirements of equilibrium, ΣH = 0, ΣV = 0, and ΣM = 0. The stiffness of the structure has no effect in this calculation. There are an infinite number of possible combi-nations of permanent load conditions for any cable-stayed bridge. The designer can select the one that is most advantageous for the design when other loads are considered.Once the permanent load condition is established by the designer, the construction has to reproduce this final condition. Construction stage analysis, which checks the stresses and stability of the structure in every construction stage, starts from this selected final condition backwards.However, if the structure is of concrete or composite, creep and shrinkage effect must be calculated in a forward calculation starting from the beginning of the construction. In such cases, the calcu-lation is a combination of forward and backward operations.The construction stage analysis also provides the required camber of the structure during con-struction.19.3.2Live LoadLive-load stresses are mostly determined by evaluation of influence lines. However, the stress at a given location in a cable-stayed bridge is usually a combination of several force components. The stress, f, of a point at the bottom flange, for example, can be expressed as:(19.5)where A is the cross-sectional area, I is the moment of inertia , y is the distance from the neutral axis, and c is a stress influence coefficient due to the cable force K anchored at the vicinity. P is the axial force and M is the bending moment. The above equation can be rewritten asFIGURE 19.16Tendon layout at the anchorage area.f A P y I M c K =()∗+()∗+∗1(19.6)where the constants a 1, a 2, and a 3 depend on the effective width, location of the point, and other global and local geometric configurations. Under live load, the terms P, M, and K are individual influence lines. Thus , f is a combined influence line obtained by adding up the three terms multiplied by the corresponding constants a 1, a 2, and a 3, respectively.In lieu of the combined influence lines, some designs substitute P, M, and K with extreme values,i.e., maximum and minimum of each. Such a calculation is usually conservative but fails to present the actual picture of the stress distribution in the structure.19.3.3Thermal LoadsDifferential temperature between various members of the structure, especially that between the cables and the rest of the bridge, must be considered in the design. Black cables tend to be heated up and cooled down much faster than the towers and the girder, thus creating a significant tem-perature difference. Light-colored cables, therefore, are usually preferred.Orientation of the bridge toward the sun is another factor to consider. One face of the towers and some group of cables facing the sun may be warmed up while the other side is in the shadow,causing a temperature gradient across the tower columns and differential temperature among the cable groups.19.3.4Dynamic Loads19.3.4.1Structural BehaviorThe girder of a cable-stayed bridge is usually supported at the towers and the end piers. Depending on the type of bearing or supports used, the dynamic behavior of the structure can be quite different.If very soft supports are used, the girder acts like a pendulum. Its fundamental frequency will be very low. Stiffening up the supports and bearings can increase the frequency significantly.Seismic and aerodynamics are the two major dynamic loads to be considered in the design of cable-stayed bridges. However, they often have contradictory demands on the structure. For aero-dynamic stability a stiffer structure is preferred. But for seismic design, except if the bridge is founded on very soft soil, a more flexible bridge will have less response. Some compromise between these two demands is required.Because the way these two dynamic loads excite the structure is different, special mechanical devices can be used to assist the structure to adjust to both load conditions. Aerodynamic responses build up slowly. For this type of load the forces in the connections required to minimize the vibration buildup are relatively small. Earthquakes happen suddenly. The response will be especially sudden if the seismic motion also contains large flings. Consequently, a device that connects the girder and the tower, which can break at a certain predetermined force will help in both events. Under aerodynamic actions, it will suppress the onset of the vibrations as the connection makes the structure stiffer. Under seismic load, the connection breaks at the predetermined load and the structure becomes more flexible. This reduces the fundamental frequency of the bridge.19.3.4.2Seismic DesignMost cable-stayed bridges are relatively flexible with long fundamental periods in the range of 3.0 s or longer. Their seismic responses are usually not very significant in the longitudinal direction. In the transverse direction, the towers are similar to a high-rise building. Their responses are also manageable.Experience shows that, except in extremely high seismic areas, earthquake load seldom controls the design. On the other hand, because most cable-stayed bridges are categorized as major structures,they are usually required to be designed for more severe earthquake loads than regular structures.f a P a M a K =∗+∗+∗123。
桥梁工程-拱桥计算2
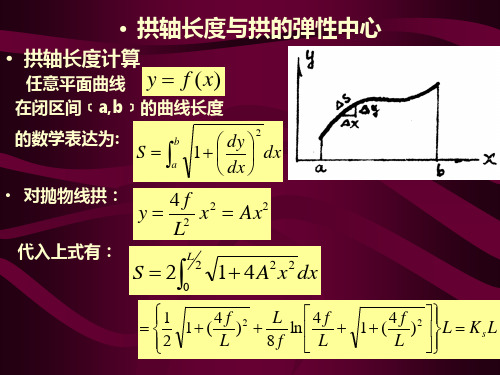
而
dx L dξ 1 1 ds = = , cos = = 2 cos 2 cos 1 + tg 1 + η 2 sh 2 kξ
1 α L2 2 2 2 2 故: 1 p = ∫ (1 ξ ) 1 + η sh kξ + (1 α ) ∫ 1 + η sh kξ dξ 4 EI α 0
S = 2∫
L
2
0
1 + 4 A x dx
2 2
1 4 f 2 L 4 f 4 f 2 = 1+ ( ) + ln + 1 + ( ) L = K s L L 8f L L 2
悬链线拱
dy L = ηshKξ , dx = dξ dx 2
1
1
S = 2∫
1
0
L 1 1 + η sh Kξ dξ = L 2 ν1
∫
∫
可分别查《拱桥》手册表Ⅲ , 可分别查《拱桥》手册表Ⅲ-9,Ⅲ-11 N = H g cos 主拱任意截面恒载弹性压缩影响内力为: 主拱任意截面恒载弹性压缩影响内力为: M = H g y
偏离内力
Q = H g sin
拱轴线偏离恒载压力线所产生的主拱内力称为偏离内力, 拱轴线偏离恒载压力线所产生的主拱内力称为偏离内力,对于大 中跨径空腹悬链拱应予考虑. 中跨径空腹悬链拱应予考虑.
分子可用分段总和法近似求取 11 MPy yi M Pi L yi M Pi L ∫ EI ds ≈ 2∑ EI cos i + i =∑ EI cos i i =1 0 ,12
拱顶控制弯矩为"+", 拱顶控制弯矩为" 拱脚为"-" 拱脚为"
桥梁设计常用数据手册

桥梁设计常用数据手册随着社会的不断发展和进步,桥梁作为连接城市和区域的重要交通设施,起着不可替代的作用。
桥梁设计的质量直接影响着交通的顺畅和人们的生活质量。
对于桥梁设计工作者来说,拥有一份常用数据手册是非常重要的。
本手册将介绍桥梁设计中常用的数据和参数,以及其应用。
一、桥梁基本参数1. 桥梁分类与结构形式- 按照功能划分:公路桥、铁路桥、人行桥等;- 按照材料划分:钢桥、混凝土桥、木桥等;- 按照结构形式划分:梁式桥、拱桥、索拉桥等。
2. 桥梁跨度与荷载- 桥梁跨度:单跨、连续梁、悬索桥等;- 桥梁荷载标准:设计车辆荷载、风荷载、地震荷载等。
3. 材料力学参数- 钢材:强度、屈服点、弹性模量等;- 混凝土:抗压强度、抗拉强度、收缩率等。
二、桥梁设计计算1. 结构设计参数- 桥墩与桥台:尺寸、基础形式、承载力等;- 桥面板设计:厚度、受力分析、变形控制等。
2. 荷载计算- 桥梁荷载计算:移动荷载、静荷载、特殊荷载等;- 桥梁挠度计算:跨径挠度、温度变形等。
3. 风荷载计算- 风荷载标准:设计风速、风载分布、桥梁抗风设计等;- 风振效应:风致振动频率、振动幅度等。
三、桥梁施工控制1. 施工材料参数- 混凝土质量控制:配合比、抗压强度、收缩裂缝控制等;- 钢材质量:材料标准、焊接质量、防锈措施等。
2. 施工工艺参数- 桥梁支座安装:支座尺寸、安装方法、调整控制等;- 预应力构件施工:张拉力控制、锚固长度、预应力损失计算等。
3. 安全防护措施- 施工现场安全:封闭施工区域、交通引导、安全标识等;- 桥梁施工监测:振动监测、动力响应监测、变形监测等。
四、桥梁运维保养1. 桥梁监测参数- 结构安全监测:裂缝监测、变形监测、钢材腐蚀监测等;- 桥梁荷载监测:车流量监测、车速监测、荷载频谱分析等。
2. 桥梁保养措施- 桥面防水维护:防水涂料、排水系统维护、伸缩缝保养等;- 涂装保护:防锈涂层、表面修复、更换防护层等。
6.桥工程量计算表格计算模板

柱高 φ8 φ20
m kg kg
φ22 kg
C25
m3
桥台 柱
柱高 φ8 φ20
m kg kg
φ22 kg
C25
m3
桩长
m
桥墩 φ8
kg
桩 φ12 kg
φ20 kg
φ22 kg
C25
m3
桩长
m
桥台 φ8
kg
桩 φ12 kg
φ20 kg
φ22 kg
C25
m3
桥墩 φ10 kg
盖梁 φ12 kg
φ25 kg
后封锚下部 40片梁 14.5cm,上部
18.5cm. 40片梁 螺旋筋
40片梁
其中钢纤 维:187.2kg
2处
其中钢纤 维:89kg
2处
2处
RG-80
型钢
伸缩
缝
钢板
kg
C25
m3
支承
垫石 φ8
kg
φ200×
42mm圆 板式橡胶
个
支座
支座 φ200×
42mm四 氟圆板式
个
橡胶支座
C25
m3
桥墩 柱
C25
m3
桥台 φ10 kg
盖梁 φ12 kg
φ25 kg
桥墩 挡块
C25 φ8 φ12
m3 kg kg
桥台 挡块
C25 φ8 φ12
m3 kg kg
桥台 C25
m3
背墙 φ12 kg
C25
m3
桥头 φ12 kg
搭板
2处
4个墩
2个台
4
4 4 4 2
2 2 2 4 8 4 4 4 4 2 4 2 2 2 2 4 4 4 4 2 2 2 2 4 4 4(请学员们有时间的提前计算,注意不要随意修改表格)
桥梁计算书——精选推荐
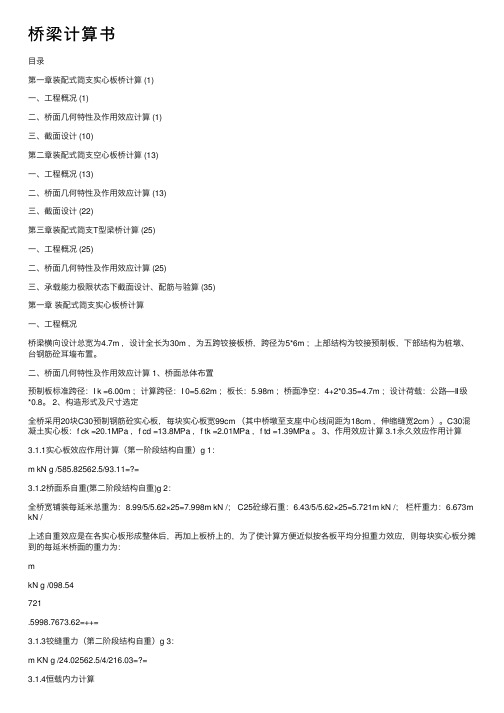
桥梁计算书⽬录第⼀章装配式简⽀实⼼板桥计算 (1)⼀、⼯程概况 (1)⼆、桥⾯⼏何特性及作⽤效应计算 (1)三、截⾯设计 (10)第⼆章装配式简⽀空⼼板桥计算 (13)⼀、⼯程概况 (13)⼆、桥⾯⼏何特性及作⽤效应计算 (13)三、截⾯设计 (22)第三章装配式简⽀T型梁桥计算 (25)⼀、⼯程概况 (25)⼆、桥⾯⼏何特性及作⽤效应计算 (25)三、承载能⼒极限状态下截⾯设计、配筋与验算 (35)第⼀章装配式简⽀实⼼板桥计算⼀、⼯程概况桥梁横向设计总宽为4.7m ,设计全长为30m ,为五跨铰接板桥,跨径为5*6m ;上部结构为铰接预制板,下部结构为桩墩、台钢筋砼⽿墙布置。
⼆、桥⾯⼏何特性及作⽤效应计算 1、桥⾯总体布置预制板标准跨径:I k =6.00m ;计算跨径:I 0=5.62m ;板长:5.98m ;桥⾯净空:4+2*0.35=4.7m ;设计荷载:公路—Ⅱ级*0.8。
2、构造形式及尺⼨选定全桥采⽤20块C30预制钢筋砼实⼼板,每块实⼼板宽99cm (其中桥墩⾄⽀座中⼼线间距为18cm ,伸缩缝宽2cm )。
C30混凝⼟实⼼板:f ck =20.1MPa ,f cd =13.8MPa ,f tk =2.01MPa ,f td =1.39MPa 。
3、作⽤效应计算 3.1永久效应作⽤计算3.1.1实⼼板效应作⽤计算(第⼀阶段结构⾃重)g 1:m kN g /585.82562.5/93.11=?=3.1.2桥⾯系⾃重(第⼆阶段结构⾃重)g 2:全桥宽铺装每延⽶总重为:8.99/5/5.62×25=7.998m kN /; C25砼缘⽯重:6.43/5/5.62×25=5.721m kN /;栏杆重⼒:6.673m kN /上述⾃重效应是在各实⼼板形成整体后,再加上板桥上的,为了使计算⽅便近似按各板平均分担重⼒效应,则每块实⼼板分摊到的每延⽶桥⾯的重⼒为:mkN g /098.54721.5998.7673.62=++=3.1.3铰缝重⼒(第⼆阶段结构⾃重)g 3:m KN g /24.02562.5/4/216.03=?=3.1.4恒载内⼒计算m kN g g /585.81Ⅰ==m kN g g g /34.524.0098.532=+=+=∏ m kN g g g /92.1334.5585.8Ⅰ=+=+=∏由此计算出简⽀实⼼板永久作⽤(⾃重)效应,计算结果见表1-1。
(完整版)市政道路及桥梁各专业设计说明计算书:双向六车道,沥青混凝土
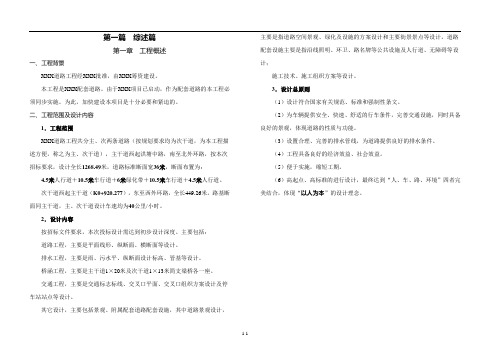
第一篇综述篇第一章工程概述一、工程背景XXX道路工程经XXX批准,由XXX筹资建设。
本工程是XXX配套道路。
由于XXX项目已启动,作为配套道路的本工程必须同步实施。
为此,加快建设本项目是十分必要和紧迫的。
二、工程范围及设计内容1.工程范围XXX道路工程共分主、次两条道路(按规划要求均为次干道,为本工程描述方便,称之为主、次干道),主干道西起洪塘中路,南至北外环路,按本次招标要求,设计全长1268.49米,道路标准断面宽36米,断面布置为:4.5米人行道+10.5米车行道+6米绿化带+10.5米车行道+4.5米人行道。
次干道西起主干道(K0+920.277),东至西外环路,全长449.26米。
路基断面同主干道,主、次干道设计车速均为40公里/小时。
2.设计内容按招标文件要求,本次投标设计需达到初步设计深度。
主要包括:道路工程,主要是平面线形、纵断面、横断面等设计。
排水工程,主要是雨、污水平、纵断面设计标高、管基等设计。
桥涵工程,主要是主干道1×20米及次干道1×13米简支梁桥各一座。
交通工程,主要是交通标志标线、交叉口平面、交叉口组织方案设计及停车站站点等设计。
其它设计,主要包括景观、附属配套道路配套设施,其中道路景观设计,主要是指道路空间景观、绿化及设施的方案设计和主要街景景点等设计。
道路配套设施主要是指沿线照明、环卫、路名牌等公共设施及人行道、无障碍等设计;施工技术、施工组织方案等设计。
3.设计总原则(1)设计符合国家有关规范、标准和强制性条文。
(2)为车辆提供安全、快速、舒适的行车条件,完善交通设施,同时具备良好的景观,体现道路的性质与功能。
(3)设置合理、完善的排水管线,为道路提供良好的排水条件。
(4)工程具备良好的经济效益、社会效益。
(5)便于实施,缩短工期。
(6)高起点、高标准的进行设计,最终达到“人、车、路、环境”四者完美结合,体现“以人为本”的设计理念。
第二章设计依据、标准和规范一、道路性质与技术标准1.设计依据(1)XXX。
桥梁设计常用数据手册
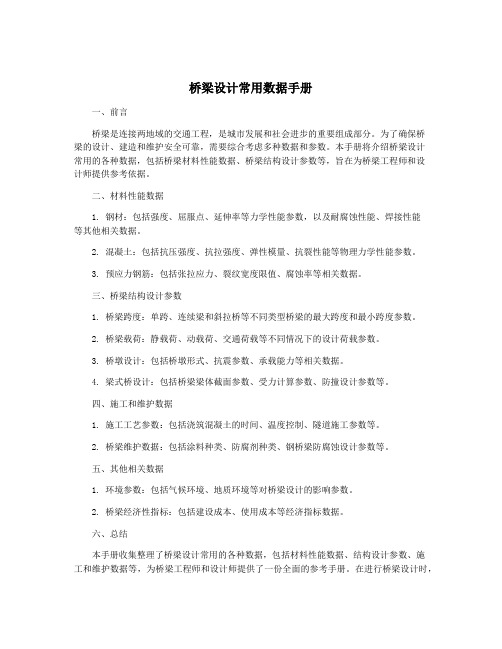
桥梁设计常用数据手册一、前言桥梁是连接两地域的交通工程,是城市发展和社会进步的重要组成部分。
为了确保桥梁的设计、建造和维护安全可靠,需要综合考虑多种数据和参数。
本手册将介绍桥梁设计常用的各种数据,包括桥梁材料性能数据、桥梁结构设计参数等,旨在为桥梁工程师和设计师提供参考依据。
二、材料性能数据1. 钢材:包括强度、屈服点、延伸率等力学性能参数,以及耐腐蚀性能、焊接性能等其他相关数据。
2. 混凝土:包括抗压强度、抗拉强度、弹性模量、抗裂性能等物理力学性能参数。
3. 预应力钢筋:包括张拉应力、裂纹宽度限值、腐蚀率等相关数据。
三、桥梁结构设计参数1. 桥梁跨度:单跨、连续梁和斜拉桥等不同类型桥梁的最大跨度和最小跨度参数。
2. 桥梁载荷:静载荷、动载荷、交通荷载等不同情况下的设计荷载参数。
3. 桥墩设计:包括桥墩形式、抗震参数、承载能力等相关数据。
4. 梁式桥设计:包括桥梁梁体截面参数、受力计算参数、防撞设计参数等。
四、施工和维护数据1. 施工工艺参数:包括浇筑混凝土的时间、温度控制、隧道施工参数等。
2. 桥梁维护数据:包括涂料种类、防腐剂种类、钢桥梁防腐蚀设计参数等。
五、其他相关数据1. 环境参数:包括气候环境、地质环境等对桥梁设计的影响参数。
2. 桥梁经济性指标:包括建设成本、使用成本等经济指标数据。
六、总结本手册收集整理了桥梁设计常用的各种数据,包括材料性能数据、结构设计参数、施工和维护数据等,为桥梁工程师和设计师提供了一份全面的参考手册。
在进行桥梁设计时,可以根据本手册提供的数据,结合实际情况进行分析和应用,以确保桥梁的设计、建造和维护工作更加安全可靠。
重庆交通大学桥梁工程Ⅱ课程设计计算书
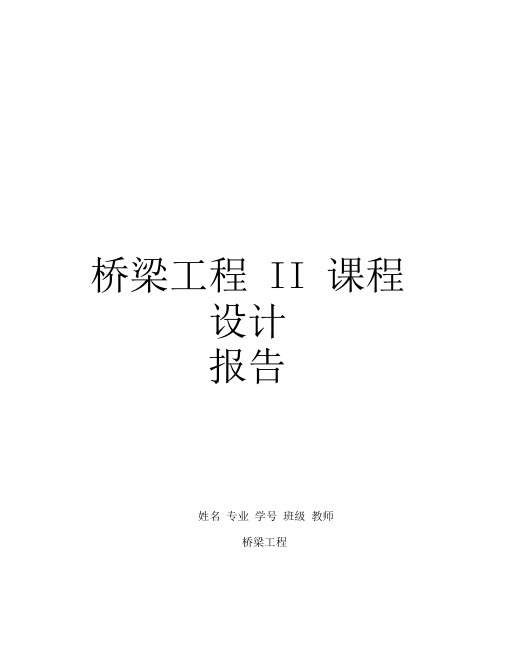
桥梁工程II 课程设计报告姓名专业学号班级教师桥梁工程2526正截面抗压强度验算自重剪力 汽车荷载剪力、设计资料二、拱圈几何性质 三、确定拱轴系数2015 年 6月目录四、拱圈弹性中心及弹性压缩系 数10五、主拱圈截面内力计算10恒载内力 11 活载内力11 汽车荷载 13 温度作用效应16六、主拱圈截面正截面强度验算2223拱脚截面直接抗剪强度验算用的荷载效应2425拱脚截面直接抗剪验算2526七、主拱圈稳定性验算27八、裸拱圈的强度和稳定性验算31截面内力整体强度 稳定性验算用的荷载 28 拱圈整体强度稳 定性验算30裸拱圈的强度及稳定性验算34321)中华人民共和国行业标准 . 公路圬工桥涵设计规范(课程设计实施指导根据选定题目的设计资料,即拱桥跨径、矢跨比、桥面宽度、荷载等级、设计资料、设计题目 1:等截面石拱桥设计与计算某空腹式悬链线石拱桥,净跨 L 0= 60m f 0= 12m f o / L 0=1/5,拱圈采用砂浆砌 筑MU50fi 料石,主拱圈厚度按照《桥梁工程(下册)》(上课用教材)自行取 值;腹拱圈为圆弧拱,采用 M10砂浆砌筑MU40块石,净跨L i =, f i =,厚度d=。
拱上横墙为横墙式,顺桥向宽,拱顶填料厚度平均为。
拱上侧墙顶宽,采用直立式 等宽构造。
主拱圈和拱上横墙砌筑完成后即拆除拱架。
大桥设计标准:公路—II ;桥面宽度:净7+2X 安全护栏。
已知:4)5)砂浆砌筑MU5(粗料石容重为25kN/卅;M10砂浆砌筑 MU40块石容重为24 kN/m 3;拱上横墙、拱上侧墙容重 24 kN/m 3; 拱顶填料、桥面铺装层换算容重 19 kN/m 3; 安全护栏: m 。
6)年平均最高温度28 r ,年平均最低气温2 C ,合龙温度15C 。
参考资料1)中华人民共和国行业标准 . 公路桥涵设计通用规范(JTG D60-2004);公路桥涵设计手册《拱桥》上册, 人民交通出版社, 1994 年 6月;桥梁计算示例集《拱桥》第二版,人民交通出版社, 2000年 10月;5) 顾安邦主编,《桥梁工程》(下) ,人民交通出版社,2000年;6)其他相关资料。
桥梁计算书(毕业设计)
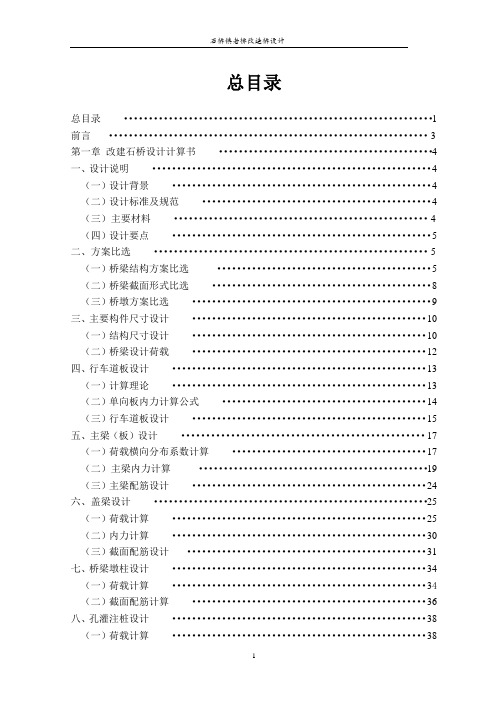
总目录总目录 (1)前言 (3)第一章改建石桥设计计算书 (4)一、设计说明 (4)(一)设计背景 (4)(二)设计标准及规范 (4)(三)主要材料 (4)(四)设计要点 (5)二、方案比选 (5)(一)桥梁结构方案比选 (5)(二)桥梁截面形式比选 (8)(三)桥墩方案比选 (9)三、主要构件尺寸设计 (10)(一)结构尺寸设计 (10)(二)桥梁设计荷载 (12)四、行车道板设计 (13)(一)计算理论 (13)(二)单向板内力计算公式 (14)(三)行车道板设计 (15)五、主梁(板)设计 (17)(一)荷载横向分布系数计算 (17)(二)主梁内力计算 (19)(三)主梁配筋设计 (24)六、盖梁设计 (25)(一)荷载计算 (25)(二)内力计算 (30)(三)截面配筋设计 (31)七、桥梁墩柱设计 (34)(一)荷载计算 (34)(二)截面配筋计算 (36)八、孔灌注桩设计 (38)(一)荷载计算 (38)(一)桩长设计 (39)(三)桩基配筋设计及强度验 (40)第二章改建石桥施工组织设计 (42)一、工程概况 (42)(一)工程简介 (42)(二)标准及规范 (42)(三)要技术指标 (42)(四)气候状况 (42)二、施工组织机构及工期安排 (42)(一)施工组织管理机构 (42)(二)工程进度计划 (43)三、机械、人员安排 (43)(一)钻孔桩施工工艺 (44)(二)钢筋混凝土空心板预制施工工艺 (46)(三)墩台施工工艺 (47)(四)盖梁施工工艺 (49)(五)桥梁安装施工工艺 (49)(六)桥面系施工工艺 (49)五、确保工程质量和工期的措施 (50)六、确保施工安全、文明施工、环境保护措施 (51)七、附图 (52)第三章改建石桥预算书 (59)结语 (65)谢辞 (66)参考文献 (67)前言毕业设计的主要目的是培养学生综合运用所学知识和技能,分析解决实际问题的能力。
工程量计算手册(全)
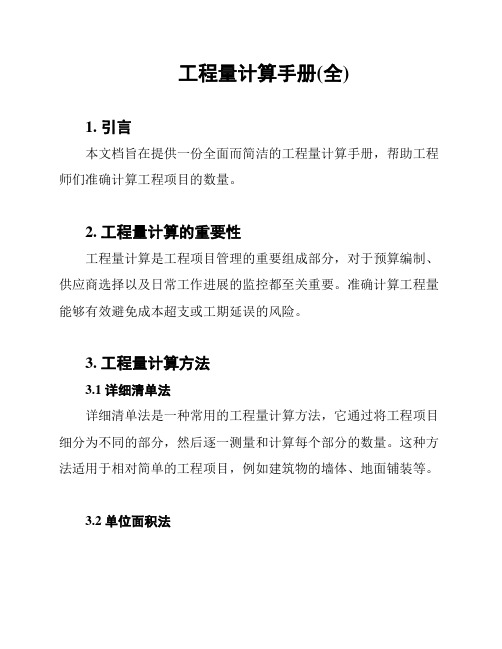
工程量计算手册(全)1. 引言本文档旨在提供一份全面而简洁的工程量计算手册,帮助工程师们准确计算工程项目的数量。
2. 工程量计算的重要性工程量计算是工程项目管理的重要组成部分,对于预算编制、供应商选择以及日常工作进展的监控都至关重要。
准确计算工程量能够有效避免成本超支或工期延误的风险。
3. 工程量计算方法3.1 详细清单法详细清单法是一种常用的工程量计算方法,它通过将工程项目细分为不同的部分,然后逐一测量和计算每个部分的数量。
这种方法适用于相对简单的工程项目,例如建筑物的墙体、地面铺装等。
3.2 单位面积法单位面积法是另一种常用的工程量计算方法,它将工程项目划分为不同的单位面积,并根据每个单位面积的特定要求计算数量。
这种方法适用于大型的土木工程项目,例如道路、桥梁等。
3.3 统计法统计法是一种基于统计数据的工程量计算方法,它通过分析历史数据和类似项目的实际情况,推算出当前项目的工程量数量。
这种方法适用于类似的工程项目,可以节省计算时间和精力。
4. 工程量计算的要点工程量计算过程中需要注意以下要点:- 精确测量:选择合适的测量工具和方法,确保数据的准确性。
- 细化划分:将工程项目细分为合适的部分,以便更准确地计算数量。
- 了解标准规范:熟悉相关的标准规范,遵循规范要求进行计算。
- 参考历史数据:参考过往的类似项目数据,可以提高计算的准确性。
5. 结论本文档介绍了工程量计算的重要性和常用的计算方法,并提供了一些注意事项。
合理、准确地计算工程量对于项目管理和控制非常重要,希望本手册能为工程师们提供一些帮助。
---以上为工程量计算手册的概要,详细内容请参考手册。
- 1、下载文档前请自行甄别文档内容的完整性,平台不提供额外的编辑、内容补充、找答案等附加服务。
- 2、"仅部分预览"的文档,不可在线预览部分如存在完整性等问题,可反馈申请退款(可完整预览的文档不适用该条件!)。
- 3、如文档侵犯您的权益,请联系客服反馈,我们会尽快为您处理(人工客服工作时间:9:00-18:30)。
工程计算手册(桥梁工程)本页仅作为文档页封面,使用时可以删除This document is for reference only-rar21year.March桥梁工程1、目的/使用范围为确保桥梁施工的施工质量,达到设计及施工规范要求,提高产品质量,特制本作业指导书;本作业指导书适用于桥梁工程施工。
2、编制依据《铁路桥涵工程施工质量验收标准》(TB10415–2003);《铁路混凝土与砌体工程施工质量验收标准》(TB10424–2003); 3、作业内容及程序地基处理→基地换填→墩台制作施工→梁的制作施工→支座安装→明桥面和桥梁附属设施施工一、(1)桥梁地基处理:1. 基坑开挖前应按地质、水文资料和环保要求,结合现场情况,制定施工方案,确定开挖范围、开挖坡度、支持方案、弃土位置和防、排水等措施。
2.基坑土方施工应对支护结构、周围环境进行观察和观测,当发现异常情况应停止施工及时处理,待恢复正常后方可继续施工。
基地处理应符合下列规定:①基地处理应清除岩面松碎石块、淤泥、苔藓,凿出新鲜岩面,表面应清洗干净,应将去倾斜岩面凿平或凿成台阶;②碎石类土及砂类土层基底成重面应修理平整,粘性土层基底整修时,应在天然状态下铲平,不得用回填土夯平;③砌筑基础时,应在基础底面先铺一层5—10cm水泥砂浆3.基坑平面位置、坑底尺寸必须满足设计和施工工艺设计要求。
4. 基坑开挖方式和支护必须满足设计要求。
5.基地地质条件必须满足设计要求。
基底高程的允许偏差和检验方法:(2)、基坑回填填料1.基坑回填填料应符合设计要求,夯实应符合规定。
2.换填地基所用材料必须符合下列规定:换填用砂应为中粗砂,有机质和泥量均不得大于5%;碎石粒径不得大于100mm,含泥量不得大于5%;石灰等级不得小于Ⅲ级。
3.换填范围必须符合设计要求。
4填料比例必须符合设计要求。
5.填筑和压实工艺必须符合设计和施工技术方案的要求。
6.压实密度必须符合设计要求。
换填地基和顶部高程允许偏差为±50 mm。
二、墩台制作施工(1)钢筋加工绑扎1.钢筋在运输、加工和储存过程中应防止锈蚀、污染和变形。
并按品种、规格和检验状态分别标识存放。
2.浇筑混凝土前,施工单位应对钢筋隐蔽前的下列内容进行检查,形成施工记录。
①钢筋品种、规格、数量位置和间距等②钢筋的连接方式、接头位置接头数量和接头面积百分率等③预埋件的规格数量等;④钢筋保护层厚度。
3.钢筋进场时,必须按批抽取试件做力学性能(屈服强度、抗拉强度和伸长率)和工艺性能(冷弯)试验,质量必须符合设计规范要求。
钢筋应平直、无损伤,表面无裂缝、油污、颗粒状或片状老锈。
4.钢筋加工应符合设计要求。
当设计未提出要求时,应符合下列规定:①受拉热轧光圆钢筋的末端应作180°弯钩,其弯曲直径d m不得小于钢筋直径的2.5倍,钩端应留有不小于钢筋直径3倍的直线段②受拉热轧光圆和带肋钢筋的末端,当设计要求采用直角形弯钩时,直钩的弯曲直径d m不得小于钢筋直径的5倍,钩端应留有不小于钢筋直径3倍的直线段③弯起钢筋应弯成平滑的曲线,其弯曲半径不得小于钢筋直径的10倍(光圆钢筋)或12倍(带肋钢筋)④用光圆钢筋制成的箍筋,其末端应作不小于90°的弯钩,有抗震等特殊要求的结构应作135°或180°的弯钩;弯钩的弯曲直径应大于受力钢筋直径,且不得小于箍筋直径的2.5倍;弯钩端直线段的长度,一般结构不得小于箍筋的5倍,有抗震等特殊要求的结构,不得小于箍筋直径的10倍。
钢筋加工允许偏差和检验方法钢筋接头应设置在承受应力较小处,并应分散布置。
配置在“同一截面”内受力钢筋接头的截面面积,占受力钢筋总截面面积的百分率,应符合设计要求。
当设计未提出要求时,应符合下列规定:1焊接接头在受弯构件的受拉区不得大于50%,轴心受拉构件不得大于25%;2 绑扎接头在构件的受拉区,不得大于25%,在受压区不得大于50%3 钢筋接头应避开钢筋弯曲处,距弯曲点的距离不得小于钢筋直径的10倍;4在同一根钢筋上应少设接头。
“同一截面”内,同一根钢筋上不得超过一个接头。
钢筋安装及钢筋保护层厚度允许偏差和检验方法:钢筋安装及钢筋保护层厚度允许偏差和检验方法(2)模板及支架安装和拆除1.模板及支架应具有足够的强度、刚度和稳定性;能承受所浇筑混凝土和砌体的重力、测压力及施工荷载;保证结构尺寸的正确,并根据工程结构形式、地基承载力、施工设备和材料等条件进行施工工艺设计,其弹性压缩、预拱度和沉降值应符合设计要求。
2.模板及支架必须安置于符合设计的可靠基底上,并有足够的支承面积和防、排水或防冻措施。
3.模板及支架与脚手架之间不得相互连接。
4.浇筑混凝土或砌体砌筑前,施工单位应对模板及支架进行交接检查,形成施工记录。
5.施工过程应对模板及支架进行观察和维护;发现跑模、变形等异常情况时,应停止施工,采取措施,并形成记录。
6.模板安装的材料质量及结构必须符合施工工艺设计要求。
模板安装必须稳固牢靠,接缝严密,不得漏浆。
模板与混凝土的接触面必须清理干净并涂刷隔离剂。
浇筑混凝土前,模板型内的积水和杂物应清理干净。
(3)混凝土施工1、当工地昼夜平均温度连续3d低于+5℃或最低气温低于-3℃时,应采取冬期施工措施;当工地平均气温高于30℃时,应采取夏季施工措施。
2、原材料进场必须对其进行检验,必须符合规范标准。
3、混凝土配合比必须符合设计规范要求。
4、结构混凝土强度等级和弹性模量必须符合设计要求。
混凝土抗压强度和弹性模量试件,在混凝土的浇筑地点随机抽样制作,其试件的取样与留置应符合下列规定:①抗压强度标准条件养护试件的取样与留置:1)没搅拌100盘且不超过100m3的同配合比的混凝土,取样不得少于一次;2)每工作班拌制的同一配合比的混凝土不足100盘时,取样不得少于一次;3)每次取样应至少留置一组②弹性模量试件的取样与留置按设计要求和实际需要确定。
③抗压强度同条件养护试件的取样、养护方式和留置数量应符合设计规范要求。
5、施工缝的位置和处理必须符合设计和施工技术方案的要求。
6、混凝土浇筑完毕后,应按施工技术方案及时采取有效的养护措施,并应符合下列要求:1)应在浇筑完毕后的12h一内混凝土加以覆盖并保湿养护。
2)混凝土浇水养护的时间:对采用硅酸盐水泥、普通硅酸盐水泥或矿渣硅酸盐水泥拌制的混凝土,不得少于7d;对掺用缓凝型外加剂或有抗渗等要求的混凝土,不得少于14d。
3)浇水次数应能保持混凝土处于湿润状态;混凝土养护用水应与拌合用水相同。
4)采用塑料布覆盖养护混凝土,其敞露的全部表面应覆盖严密,应保持塑料布内有凝结水。
5)混凝土强度达到1.2MPa前,不得在其上踩踏或安装模板及支架。
6)当日平均气温低于5℃时,不得浇水。
7)混凝土表面不便浇水或使用塑料布时,宜涂刷养护剂。
结构外形尺寸允许偏差和检验方法:7、混凝土结构表面应密实平整、颜色均匀,不得有露筋、蜂窝、孔洞、疏松、麻面和缺棱掉角等缺陷。
(4)模板拆除1、拆除承重模板及支架时的混凝土强度应符合设计要求。
当设计无要求时,除相关专业验收标准有特殊规定外,混凝土强度应符合表4.3.1的规定。
8.拆除非承重模板时,混凝土强度应保证其表面及棱角不受损伤。
(5)防水层及伸缩缝1、防水层及伸缩缝施工前,施工单位要按设计要求,编制施工方案,进行技术交底。
2、防水层严禁在雨雪或五级风及其以上时施工。
3、防水层隐蔽前,应进行防水层隐蔽工程验收,未经验收合格不得隐蔽。
防水层的基层应平整、清洁、干燥,不得有空鼓、松动、蜂窝麻面、浮土和油污。
4、防水层的表面质量应达到涂层厚薄一致,卷材粘贴牢固,搭接封口正确。
不得有滑移,翘边、气泡、损伤等现象。
坡度平顺,排水通畅。
5、保护层表面质量应达到与防水层粘结牢固,结合紧密,厚度均匀一致。
表面平整密实,不得有疏松、起砂、脱皮、损伤等现象。
6、防水层和保护层的材料称量的允许偏差为2%。
7、沥青胶结材料使用的允许偏差为-10℃.防水层允许偏差和检验方法保护层的允许偏差和检验方法1、伸缩缝所用材料的品种、规格、性能等必须符合设计要求。
2、伸缩缝位置、尺寸、构造形式和止水带的安装等必须符合设计要求。
3、沉降缝不得漏水。
4、沉降缝填塞前,缝内应清扫干净,保持干燥,不得有杂物和积水。
5、沉降缝的表面质量应达到缝宽均匀,缝身竖直,环向贯通,填塞密实,外表光洁。
(6)台后填土、锥体及其他台后及锥体填料种类和规格必须符合设计要求。
台后及锥体填筑范围必须符合设计要求。
台后及锥体填筑密实度必须符合设计要求。
锥体填筑浇筑后应刷坡,坡面平整圆顺。
二、梁的制作施工预应力梁制作(1)钢筋加工绑扎1.钢筋在运输、加工和储存过程中应防止锈蚀、污染和变形。
并按品种、规格和检验状态分别标识存放。
2.浇筑混凝土前,施工单位应对钢筋隐蔽前的下列内容进行检查,形成施工记录。
①钢筋品种、规格、数量位置和间距等②钢筋的连接方式、接头位置接头数量和接头面积百分率等③预埋件的规格数量等;④钢筋保护层厚度。
3.钢筋进场时,必须按批抽取试件做力学性能(屈服强度、抗拉强度和伸长率)和工艺性能(冷弯)试验,质量必须符合设计规范要求。
钢筋应平直、无损伤,表面无裂缝、油污、颗粒状或片状老锈。
4.钢筋加工应符合设计要求。
当设计未提出要求时,应符合下列规定:①受拉热轧光圆钢筋的末端应作180°弯钩,其弯曲直径d m不得小于钢筋直径的2.5倍,钩端应留有不小于钢筋直径3倍的直线段②受拉热轧光圆和带肋钢筋的末端,当设计要求采用直角形弯钩时,直钩的弯曲直径d m不得小于钢筋直径的5倍,钩端应留有不小于钢筋直径3倍的直线段③弯起钢筋应弯成平滑的曲线,其弯曲半径不得小于钢筋直径的10倍(光圆钢筋)或12倍(带肋钢筋)④用光圆钢筋制成的箍筋,其末端应作不小于90°的弯钩,有抗震等特殊要求的结构应作135°或180°的弯钩;弯钩的弯曲直径应大于受力钢筋直径,且不得小于箍筋直径的2.5倍;弯钩端直线段的长度,一般结构不得小于箍筋的5倍,有抗震等特殊要求的结构,不得小于箍筋直径的10倍。
钢筋接头应设置在承受应力较小处,并应分散布置。
配置在“同一截面”内受力钢筋接头的截面面积,占受力钢筋总截面面积的百分率,应符合设计要求。
当设计未提出要求时,应符合下列规定:1焊接接头在受弯构件的受拉区不得大于50%,轴心受拉构件不得大于25%;2 绑扎接头在构件的受拉区,不得大于25%,在受压区不得大于50%3 钢筋接头应避开钢筋弯曲处,距弯曲点的距离不得小于钢筋直径的10倍;4在同一根钢筋上应少设接头。
“同一截面”内,同一根钢筋上不得超过一个接头。
钢筋加工允许偏差和检验方法钢筋安装及钢筋保护层厚度允许偏差和检验方法(2)模板及支架安装和拆除1.模板及支架应具有足够的强度、刚度和稳定性;能承受所浇筑混凝土和砌体的重力、测压力及施工荷载;保证结构尺寸的正确,并根据工程结构形式、地基承载力、施工设备和材料等条件进行施工工艺设计,其弹性压缩、预拱度和沉降值应符合设计要求。