Modeling uncertainty in cutter wear prediction for tunnel boring machines(2012)-C.Frenzel
介绍微雕技艺作文英文
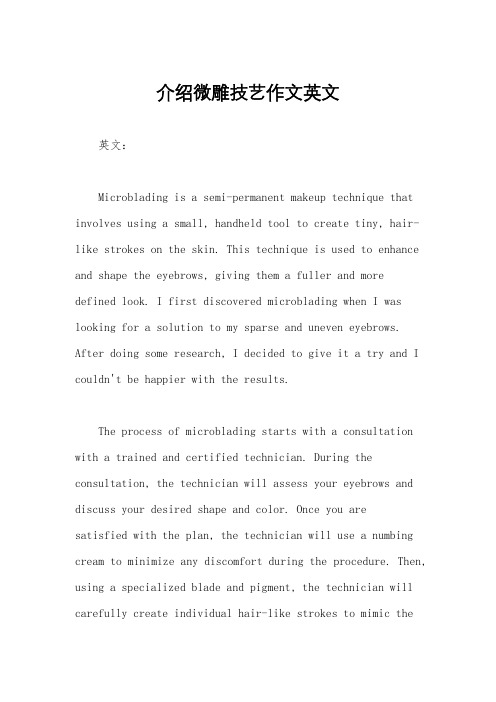
介绍微雕技艺作文英文英文:Microblading is a semi-permanent makeup technique that involves using a small, handheld tool to create tiny, hair-like strokes on the skin. This technique is used to enhance and shape the eyebrows, giving them a fuller and more defined look. I first discovered microblading when I was looking for a solution to my sparse and uneven eyebrows. After doing some research, I decided to give it a try and I couldn't be happier with the results.The process of microblading starts with a consultation with a trained and certified technician. During the consultation, the technician will assess your eyebrows and discuss your desired shape and color. Once you aresatisfied with the plan, the technician will use a numbing cream to minimize any discomfort during the procedure. Then, using a specialized blade and pigment, the technician will carefully create individual hair-like strokes to mimic thenatural pattern of your eyebrows. The entire process takes about 2-3 hours, including the initial consultation.After the procedure, there is a healing process of about 4-6 weeks, during which the pigment will settle and the skin will fully recover. It's important to follow the aftercare instructions provided by the technician to ensure the best results. Once the healing process is complete, the results are amazing. My eyebrows look natural and full, and I no longer have to spend time filling them in with makeup every day.Microblading has truly changed my life. I feel more confident and put-together with my new eyebrows, and I no longer have to worry about smudging or fading makeup throughout the day. I would highly recommend microblading to anyone who is looking for a long-lasting solution to sparse or uneven eyebrows.中文:微雕是一种半永久性化妆技术,它使用小型手持工具在皮肤上创建微小的、类似头发的笔触。
仿生减阻原理英文
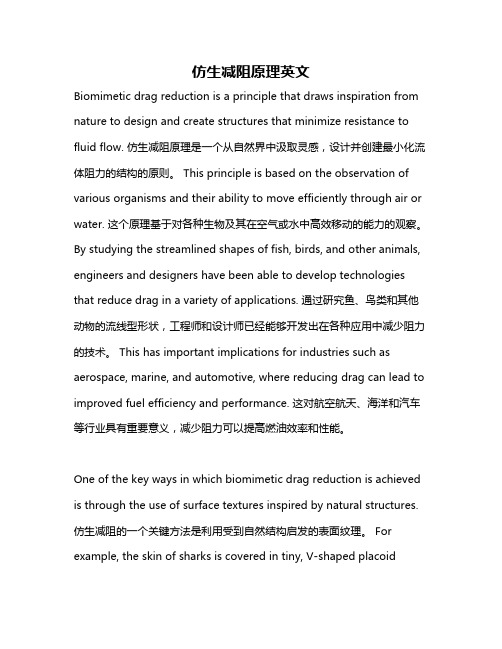
仿生减阻原理英文Biomimetic drag reduction is a principle that draws inspiration from nature to design and create structures that minimize resistance to fluid flow. 仿生减阻原理是一个从自然界中汲取灵感,设计并创建最小化流体阻力的结构的原则。
This principle is based on the observation of various organisms and their ability to move efficiently through air or water. 这个原理基于对各种生物及其在空气或水中高效移动的能力的观察。
By studying the streamlined shapes of fish, birds, and other animals, engineers and designers have been able to develop technologies that reduce drag in a variety of applications. 通过研究鱼、鸟类和其他动物的流线型形状,工程师和设计师已经能够开发出在各种应用中减少阻力的技术。
This has important implications for industries such as aerospace, marine, and automotive, where reducing drag can lead to improved fuel efficiency and performance. 这对航空航天、海洋和汽车等行业具有重要意义,减少阻力可以提高燃油效率和性能。
One of the key ways in which biomimetic drag reduction is achieved is through the use of surface textures inspired by natural structures. 仿生减阻的一个关键方法是利用受到自然结构启发的表面纹理。
介绍微雕技艺作文英语
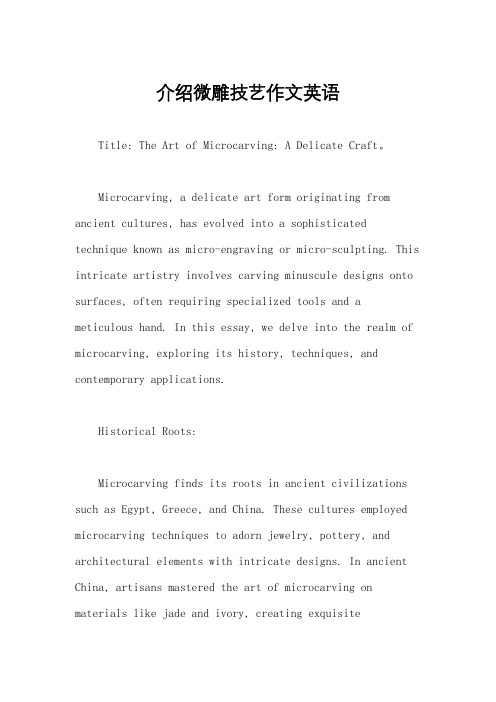
介绍微雕技艺作文英语Title: The Art of Microcarving: A Delicate Craft。
Microcarving, a delicate art form originating from ancient cultures, has evolved into a sophisticated technique known as micro-engraving or micro-sculpting. This intricate artistry involves carving minuscule designs onto surfaces, often requiring specialized tools and a meticulous hand. In this essay, we delve into the realm of microcarving, exploring its history, techniques, and contemporary applications.Historical Roots:Microcarving finds its roots in ancient civilizations such as Egypt, Greece, and China. These cultures employed microcarving techniques to adorn jewelry, pottery, and architectural elements with intricate designs. In ancient China, artisans mastered the art of microcarving on materials like jade and ivory, creating exquisitesculptures and ornaments that are revered to this day.Techniques:Modern microcarving techniques have advanced significantly, owing to technological innovations and refined craftsmanship. Artisans utilize specialized tools such as microscopes, engraving needles, and lasers to achieve precision in their work. The process begins with selecting a suitable material, often gemstones like diamonds or rubies due to their hardness and clarity. The artisan then meticulously sketches the design before delicately carving it onto the surface of the material. The intricacy of microcarving requires immense patience and skill, as even the slightest error can ruin the entire piece.Challenges and Rewards:Microcarving presents a unique set of challenges compared to traditional carving methods. Working on such a small scale demands steady hands, acute vision, and anunderstanding of the material's properties. Moreover, the fragility of the medium means that one must exercise caution throughout the entire process to avoid accidental damage. However, the rewards of mastering microcarving are unparalleled. The ability to breathe life into minute details and create awe-inspiring pieces of art is immensely gratifying for artisans and viewers alike.Contemporary Applications:In the modern era, microcarving has found diverse applications across various industries. In the realm of jewelry, micro-engraving allows designers to adorn pieces with intricate motifs, adding a touch of elegance and sophistication. Beyond adornment, microcarving has also found utility in security features for banknotes and identification documents. Microscopically engraved patterns and images serve as anti-counterfeiting measures, enhancing the security and authenticity of valuable documents.Conclusion:In conclusion, microcarving stands as a testament to the ingenuity and skill of artisans throughout history. From its humble beginnings in ancient civilizations to its contemporary applications in technology and art, microcarving continues to captivate and inspire. As technology advances and artistic boundaries expand, the art of microcarving will undoubtedly evolve, pushing the limits of what is possible on a minuscule scale.。
MPI TITAN RF Probe Selection Guide

MPI Probe Selection GuideWith a critical understanding of the numerous measurement challenges associated with today’s RF ap-plications, MPI Corporation has developed TITAN™ RF Probes, a product series specifically optimized for these complex applications centered upon the requirements of advanced RF customers.TITAN™ Probes provide the latest in technology and manufacturing advancements within the field of RF testing. They are derived from the technology transfer that accompanied the acquisition of Allstron, then significantly enhanced by MPI’s highly experienced RF testing team and subsequently produced utilizing MPI’s world class MEMS technology. Precisely manufactured, the TITAN™ Probes include matched 50 Ohm MEMS contact tips with improved probe electrical characteristics which allow the realization of unmat -ched calibration results over a wide frequency range. The patented protrusion tip design enables small passivation window bond pad probing, while significantly reducing probe skate thus providing the out -standing contact repeatability required in today’s extreme measurement environments. TITAN TM Probes with all their features are accompanied by a truly affordable price.The TITAN™ Probe series are available in single-ended and dual tip configurations, with pitch range from 50 micron to 1250 micron and frequencies from 26 GHz to 110 GHz. TITAN™ RF Probes are the ideal choice for on-wafer S-parameter measurements of RF, mm-wave devices and circuits up to 110 GHz as well as for the characterization of RF power devices requiring up to 10 Watts of continuous power. Finally, customers can benefit from both long product life and unbeatable cost of ownership which they have desired foryears.Unique design of the MEMS coplanar contacttip of the TITAN™ probe series.DC-needle-alike visibility of the contact point and the minimal paddamage due to the unique design of the tipAC2-2 Thru S11 Repeatability. Semi-Automated System.-100-80-60-40-200 S 11 E r r o r M a g n i t u d e (d B )Frequency (GHz)Another advantage of the TITAN™ probe is its superior contact repeatability, which is comparable with the entire system trace noise when measured on the semi-automated system and on gold contact pads.CROSSTALKCrosstalk of TITAN™ probes on the short and the bare ceramic open standard of 150 micron spacing compared to conventional 110 GHz probe technologies. Results are corrected by the multiline TRL calibration. All probes are of GSG configuration and 100 micron pitch.-80-60-40-200Crosstalk on Open. Multiline TRL Calibration.M a g (S21) (d B )Frequency (GHz)-80-60-40-200Crosstalk on Short. Multiline TRL Calibration.M a g (S21) (d B )Frequency (GHz)The maximal probe c ontac t repeatability error of the c alibrate S11-parameter of the AC2-2 thru standard by T110 probes. Semi-automated system. Ten contact circles.Cantilever needle material Ni alloy Body materialAl alloy Contact pressure @2 mils overtravel 20 g Lifetime, touchdowns> 1,000,000Ground and signal alignment error [1]± 3 µm [1]Planarity error [1] ± 3 µm [1]Contact footprint width < 30 µm Contact resistance on Au < 3 mΩThermal range-60 to 175 °CMechanical CharacteristicsAC2-2 Thru S21 Repeatability. Manual TS50 System.-100-80-60-40-200S 21 E r r o r M a g n i t u d e (d B )Frequency (GHz)MECHANICAL CHARACTERISTICSThe maximal probe c ontac t repeatability error of the c alibrate S21-parameter of the AC2-2 thru standard by T50 probes. Manual probe system TS50.26 GHZ PROBES FOR WIRELESS APPLICATIONSUnderstanding customer needs to reduce the cost of development and product testing for the high competitive wireless application market, MPI offers low-cost yet high-performance RF probes. The specifically developed SMA connector and its outstanding transmission of electro-magnetic waves through the probe design make these probes suitable for applications frequencies up to 26 GHz. The available pitch range is from 50 micron to 1250 micron with GS/SG and GSG probe tip configurations. TITAN™ 26 GHz probes are the ideal choice for measurement needs when developing components for WiFi, Bluetooth, and 3G/4G commercial wireless applications as well as for student education.Characteristic Impedance 50 ΩFrequency rangeDC to 26 GHz Insertion loss (GSG configuration)1< 0.4 dB Return loss (GSG configuration)1> 16 dB DC current ≤ 1 A DC voltage ≤ 100 V RF power, @10 GHz≤ 5 WTypical Electrical Characteristics26 GHz Probe Model: T26Connector SMAPitch range50 µm to 1250 µm Standard pitch step from 50 µm to 450 µm from 500 µm to 1250 µm25 µm step 50 µm stepAvailable for 90 µm pitch Tip configurations GSG, GS, SG Connector angleV-Style: 90-degree A-Style: 45-degreeMechanical CharacteristicsT26 probe, A-Style of the connectorTypical Electrical Characteristics: 26 GHz GSG probe, 250 micron pitchPROBES FOR DEVICE AND IC CHARACTERIZATION UP TO 110 GHZTITAN™ probes realize a unique combination of the micro-coaxial cable based probe technology and MEMS fabricated probe tip. A perfectly matched characteristic impedance of the coplanar probe tips and optimized signal transmission across the entire probe down to the pads of the device under test (DUT) result in excellent probe electrical characteristics. At the same time, the unique design of the probe tip provides minimal probe forward skate on any type of pad metallization material, therefo -re achieving accurate and repeatable measurement up to 110 GHz. TITAN™ probes are suitable for probing on small pads with long probe lifetime and low cost of ownership.The TITAN™ probe family contains dual probes for engineering and design debug of RF and mm-wave IC’s as well as high-end mm-wave range probes for S-parameter characterization up to 110 GHz for modeling of high-performance microwave devices.Characteristic Impedance 50 ΩFrequency rangeDC to 40 GHz Insertion loss (GSG configuration)1< 0.6 dB Return loss (GSG configuration)1> 18 dB DC current ≤ 1 A DC voltage ≤ 100 V RF power, @10 GHz≤ 5 WTypical Electrical Characteristics40 GHz Probe Model: T40Connector K (2.92 mm)Pitch range50 µm to 500 µmStandard pitch step For GSG configuration:from 50 µm to 450 µm from 500 µm to 800 µmFor GS/SG configuration:from 50 µm to 450 µm 25 µm step 50 µm stepAvailable for 90 µm pitch25 µm stepAvailable for 90/500 µm pitch Tip configurations GSG, GS, SG Connector angleV-Style: 90-degree A-Style: 45-degreeMechanical CharacteristicsTypical Electrical Characteristics: 40 GHz GSG probe, 150 micron pitchT40 probe, A-Style of the connectorCharacteristic Impedance50 ΩFrequency range DC to 50 GHz Insertion loss (GSG configuration)1< 0.6 dB Return loss (GSG configuration)1> 17 dBDC current≤ 1 ADC voltage≤ 100 VRF power, @10 GHz≤ 5 W Typical Electrical Characteristics Connector Q (2.4 mm)Pitch range50 µm to 250 µm Standard pitch stepFor GSG configuration: from 50 µm to 450 µm For GS/SG configuration: from 50 µm to 450 µm 25 µm stepAvailable for 90/500/550 µm pitch 25 µm stepAvailable for 90/500 µm pitchTip configurations GSG, GS, SG Connector angle V-Style: 90-degreeA-Style: 45-degreeMechanical CharacteristicsT50 probe, A-Style of the connectorTypical Electrical Characteristics: 50 GHz GSG probe, 150 micron pitchCharacteristic Impedance50 ΩFrequency range DC to 67 GHz Insertion loss (GSG configuration)1< 0.8 dB Return loss (GSG configuration)1> 16 dBDC current≤ 1 ADC voltage≤ 100 VRF power, @10 GHz≤ 5 W Typical Electrical Characteristics Connector V (1.85 mm)Pitch range50 µm to 250 µm Standard pitch stepFor GSG configuration: from 50 µm to 400 µm For GS/SG configuration: from 50 µm to 250 µm 25 µm step Available for 90 µm pitch25 µm step Available for 90 µm pitchTip configurations GSG Connector angle V-Style: 90-degreeA-Style: 45-degreeMechanical CharacteristicsT67 probe, A-Style of the connectorTypical Electrical Characteristics: 67 GHz GSG probe, 100 micron pitchCharacteristic Impedance 50 ΩFrequency rangeDC to 110 GHz Insertion loss (GSG configuration)1< 1.2 dB Return loss (GSG configuration)1> 14 dB DC current ≤ 1 A DC voltage ≤ 100 V RF power, @10 GHz≤ 5 WTypical Electrical CharacteristicsMechanical CharacteristicsTypical Electrical Characteristics: 110 GHz GSG probe, 100 micron pitchT110 probe, A-Style of the connectorCharacteristic impedance50 ΩFrequency range DC to 220 GHz Insertion loss (GSG configuration)1< 5 dB Connector end return loss(GSG configuration)1> 9 dBTip end return loss(GSG configuration)1> 13 dBDC current≤ 1.5 ADC voltage≤ 50 V Typical Electrical CharacteristicsConnector Broadband interface Pitch range50/75/90/100/125 µm Temperature range -40 ~ 150 ºC Contact width15 µmquadrant compatible(allowing corner pads)Yes recommended pad size20 µm x 20 µm recommended OT (overtravel)15 µmcontact resistance(on Al at 20 ºC using 15 µm OT)< 45 mΩlifetime touchdowns(on Al at 20 ºC using 15 µm OT)> 200,000Mechanical CharacteristicsT220 probe, broadband interface Typical Performance (at 20 ºC for 100 µm pitch)BODY DIMENSIONS PROBES Single-Ended V-StyleT220 GHz Probe1.161.1628.328437.455.6512.5527.73Single-Ended A-StyleCALIBRATION SUBSTRATESAC-series of calibration standard substrates offers up to 26 standard sets for wafer-level SOL T, LRM probe-tip cali -bration for GS/SG and GSG probes. Five coplanar lines provide the broadband reference multiline TRL calibration as well as accurate verification of conventional methods. Right-angled reciprocal elements are added to support the SOLR calibration of the system with the right-angled configuration of RF probes. A calibration substrate for wide-pitch probes is also available.Material Alumina Elements designCoplanarSupported calibration methods SOLT, LRM, SOLR, TRL and multiline TRL Thickness 635 µmSizeAC2-2 : 16.5 x 12.5 mm AC3 : 16.5 x 12.5 mm AC5 : 22.5 x 15 mm Effective velocity factor @20 GHz0.45Nominal line characteristic impedance @20 GHz 50 ΩNominal resistance of the load 50 ΩTypical load trimming accuracy error ± 0.3 %Open standardAu pads on substrate Calibration verification elements Yes Ruler scale 0 to 3 mm Ruler step size100 µmCalibration substrate AC2-2Probe Configuration GSGSupported probe pitch100 to 250 µm Number of SOL T standard groups 26Number of verification and calibration lines5Calibration substrate AC-3Probe Configuration GS/SG Supported probe pitch50 to 250 µm Number of SOL T standard groups 26Number of verification and calibration lines5Calibration substrate AC-5Probe Configuration GSG, GS/SG Supported probe pitch250 to 1250 µm Number of SOL T standard groups GSG : 7GS : 7SG : 7Open standardOn bare ceramic Number of verification and calibration linesGSG : 2GS : 1Typical characteristics of the coplanar line standard of AC2-2 calibration substrate measured using T110-GSG100 probes, and methods recommended by the National Institute of Standard and Technologies [2, 3].2468(d B /c m )F requency (G Hz)α-6-4-202I m a g (Z 0) ()F requency (G Hz)AC2-2 W#006 and T110A-GSG100Ω2.202.222.242.262.282.30 (u n i t l e s s )F requency (G Hz)β/βо4045505560R e a l (Z 0) ()F requency (G Hz)ΩTypical Electrical CharacteristicsMPI QAlibria® RF CALIBRATION SOFTWAREMPI QAlibria® RF calibration software has been designed to simplify complex and tedious RF system calibration tasks. By implementing a progressive disclosure methodology and realizing intuitive touch operation, QAlibria® provides crisp and clear guidance to the RF calibration process, minimizing con-figuration mistakes and helping to obtain accurate calibration results in fastest time. In addition, its concept of multiple GUI’s offers full access to all configuration settings and tweaks for advanced users. QAlibria® offers industry standard and advanced calibration methods. Furthermore, QAlibria® is integrated with the NIST StatistiCal™ calibration packages, ensuring easy access to the NIST mul-tiline TRL metrology-level calibration and uncertainty analysis.MPI Qalibria® supports a multi-language GUI, eliminating any evitable operation risks and inconvenience.SpecificationsRF AND MICROWAVE CABLESMPI offers an excellent selection of flexible cables and acces-sories for RF and mm-wave measurement applications forcomplete RF probe system integration.CablesHigh-quality cable assemblies with SMA and 3.5 mm connectorsprovide the best value for money, completing the entry-level RFsystems for measurement applications up to 26 GHz. Phase stab-le high-end flexible cable assemblies with high-precision 2.92, 2.4, 1.85 and 1 mm connectors guarantee high stability, accuracy and repeatability of the calibration and measurement for DC applications up to 110 GHz.MPI offers these cable assemblies in two standard lengths of 120 and 80 cm, matching the probe system’s footprint and the location of the VNA.Cables Ordering InformationMRC-18SMA-MF-80018 GHz SMA flex cable SMA (male) - SMA (female), 80 cmMRC-18SMA-MF-120018 GHz SMA flex cable SMA (male) - SMA (female), 120 cmMRC-26SMA-MF-80026 GHz SMA flex cable SMA (male) - SMA (female), 80 cmMRC-26SMA-MF-120026 GHz SMA flex cable SMA (male) - SMA (female), 120 cmMRC-40K-MF-80040 GHz flex cable 2.92 mm (K) connector, male-female, 80 cm longMRC-40K-MF-120040 GHz flex cable 2.92 mm (K) connector, male-female, 120 cm longMRC-50Q-MF-80050 GHz flex cable 2.4 mm (Q) connector, male-female , 80 cm longMRC-50Q-MF-120050 GHz flex cable 2.4 mm (Q) connector, male-female , 120 cm longMRC-67V-MF-80067 GHz flex cable 1.85 mm (V) connector, male-female, 80 cm longMRC-67V-MF-120067 GHz flex cable 1.85 mm (V) connector, male-female, 120 cm longMMC-40K-MF-80040 GHz precision flex cable 2.92 mm (K) connector, male-female, 80 cm long MMC-40K-MF-120040 GHz precision flex cable 2.92 mm (K) connector, male-female, 120 cm long MMC-50Q-MF-80050 GHz precision flex cable 2.4 mm (Q) connector, male-female , 80 cm long MMC-50Q-MF-120050 GHz precision flex cable 2.4 mm (Q) connector, male-female , 120 cm long MMC-67V-MF-80067 GHz precision flex cable 1.85 mm (V) connector, male-female, 80 cm long MMC-67V-MF-120067 GHz precision flex cable 1.85 mm (V) connector, male-female, 120 cm long MMC-110A-MF-250110 GHz precision flex cable 1 mm (A) connector, male-female, 25 cm longMPI Global PresenceDirect contact:Asia region: ****************************EMEA region: ******************************America region: ********************************MPI global presence: for your local support, please find the right contact here:/ast/support/local-support-worldwide© 2023 Copyright MPI Corporation. All rights reserved.[1] [2][3] REFERENCESParameter may vary depending upon tip configuration and pitch.R. B. Marks and D. F. Williams, "Characteristic impedance determination using propagation constant measu -rement," IEEE Microwave and Guided Wave Letters, vol. 1, pp. 141-143, June 1991.D. F. Williams and R. B. Marks, "Transmission line capacitance measurement," Microwave and Guided WaveLetters, IEEE, vol. 1, pp. 243-245, 1991.AdaptersHigh-In addition, high-quality RF and high-end mm-wave range adapters are offered to address challenges ofregular system reconfiguration and integration with different type of test instrumentation. MRA-NM-350F RF 11 GHz adapter N(male) - 3.5 (male), straight MRA-NM-350M RF 11 GHz adapter N(male) - 3.5 (female), straightMPA-350M-350F Precision 26 GHz adapter 3.5 mm (male) - 3.5 mm (female), straight MPA-350F-350F Precision 26 GHz adapter 3.5 mm (female) - 3.5 mm (female), straight MPA-350M-350M Precision 26 GHz adapter 3.5 mm (male) - 3.5 mm (male), straight MPA-292M-240F Precision 40 GHz adapter 2.92 mm (male) - 2.4 mm (female), straight MPA-292F-240M Precision 40 GHz adapter 2.92 mm (female) - 2.4 mm (male), straight MPA-292M-292F Precision 40 GHz adapter 2.92 mm (male) - 2.92 mm (female), straight MPA-292F-292F Precision 40 GHz adapter 2.92 mm (female) - 2.92 mm (female), straight MPA-292M-292M Precision 40 GHz adapter 2.92 mm (male) - 2.92 mm (male), straight MPA-240M-240F Precision 50 GHz adapter 2.4 mm (male) - 2.4 mm (female), straight MPA-240F-240F Precision 50 GHz adapter 2.4 mm (female) - 2.4 mm (female), straight MPA-240M-240M Precision 50 GHz adapter 2.4 mm (male) - 2.4 mm (male), straight MPA-185M-185F Precision 67 GHz adapter 1.85 mm (male) -1.85 mm (female), straight MPA-185F-185F Precision 67 GHz adapter 1.85 mm (female) -1.85 mm (female), straight MPA-185M-185M Precision 67 GHz adapter 1.85 mm (male) -1.85 mm (male), straight MPA-185M-100FPrecision 67 GHz adapter 1.85 mm (male) -1.00 mm (female), straightDisclaimer: TITAN Probe, QAlibria are trademarks of MPI Corporation, Taiwan. StatistiCal is a trademark of National Institute of Standards and Technology (NIST), USA. All other trademarks are the property of their respective owners. Data subject to change without notice.。
鲨鱼表皮的仿生原理对人类的作用英语作文
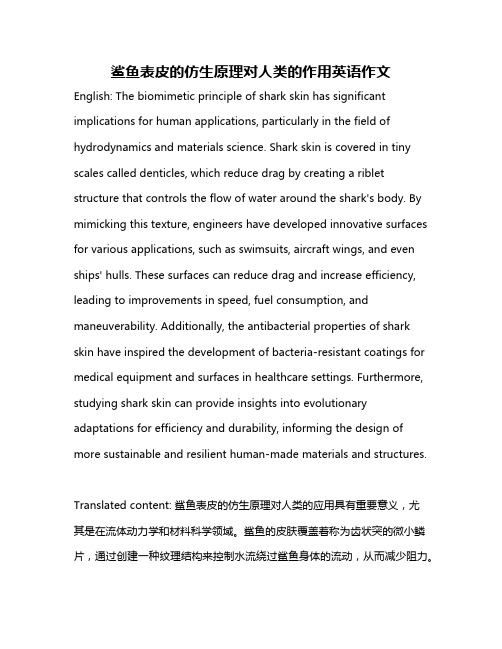
鲨鱼表皮的仿生原理对人类的作用英语作文English: The biomimetic principle of shark skin has significant implications for human applications, particularly in the field of hydrodynamics and materials science. Shark skin is covered in tiny scales called denticles, which reduce drag by creating a riblet structure that controls the flow of water around the shark's body. By mimicking this texture, engineers have developed innovative surfaces for various applications, such as swimsuits, aircraft wings, and even ships' hulls. These surfaces can reduce drag and increase efficiency, leading to improvements in speed, fuel consumption, and maneuverability. Additionally, the antibacterial properties of shark skin have inspired the development of bacteria-resistant coatings for medical equipment and surfaces in healthcare settings. Furthermore, studying shark skin can provide insights into evolutionary adaptations for efficiency and durability, informing the design of more sustainable and resilient human-made materials and structures.Translated content: 鲨鱼表皮的仿生原理对人类的应用具有重要意义,尤其是在流体动力学和材料科学领域。
关于微雕技术的作文英语

关于微雕技术的作文英语As a relatively new technique in the field of cosmetic surgery, micro-sculpting has gained increasing popularityin recent years. Micro-sculpting refers to the use of minimally invasive procedures to enhance one's facial features, such as the nose, chin, and cheeks. Thistechnique has been widely adopted by celebrities andordinary people alike, as it offers a more natural andsubtle way to improve one's appearance.Micro-sculpting is a highly precise and intricate procedure, requiring the use of specialized tools and equipment. The surgeon uses a small needle to injectfillers into the targeted areas, which can be made of hyaluronic acid, calcium hydroxyapatite, or other materials. These fillers are carefully chosen and customized to suit the patient's individual needs and preferences, ensuring a natural and harmonious look.One of the major advantages of micro-sculpting is itsminimally invasive nature. Unlike traditional plastic surgery, which often involves cutting and suturing, micro-sculpting requires only small incisions or injections. This means that there is less pain, swelling, and scarring, and a shorter recovery time. Patients can usually return to their normal activities within a few days, without any major disruptions to their daily lives.Another benefit of micro-sculpting is its versatility. It can be used to correct a wide range of facial imperfections, from asymmetrical features to wrinkles and sagging skin. The fillers used in micro-sculpting can also be adjusted over time, allowing the patient to fine-tune their appearance as needed. This makes micro-sculpting a highly customizable and personalized procedure, tailored to the patient's unique goals and desires.Despite its many benefits, micro-sculpting is not without its risks and limitations. As with any medical procedure, there is always a risk of complications, such as infection, bleeding, or allergic reactions. Patients should also be aware that the effects of micro-sculpting are notpermanent, and may require touch-ups or repeat treatments over time. It is important to discuss these risks and limitations with a qualified and experienced surgeon before undergoing micro-sculpting.In conclusion, micro-sculpting is a promising and innovative technique in the field of cosmetic surgery. Its minimally invasive nature, versatility, and customization make it an attractive option for those seeking to enhance their facial features. However, patients should approach micro-sculpting with caution and careful consideration, and choose a qualified and experienced surgeon to ensure the best possible results.。
字迹材料耐久性中色差测定不确定度评估及分析
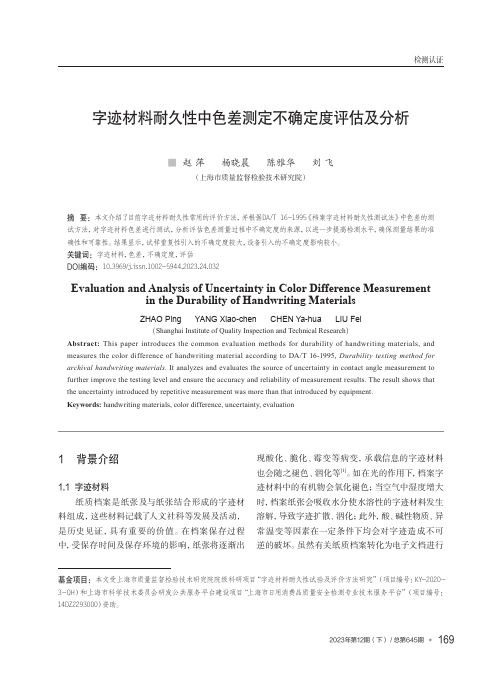
检测认证字迹材料耐久性中色差测定不确定度评估及分析■ 赵 萍 杨晓晨 陈雅华 刘 飞(上海市质量监督检验技术研究院)摘 要:本文介绍了目前字迹材料耐久性常用的评价方法,并根据DA/T 16-1995《档案字迹材料耐久性测试法》中色差的测试方法,对字迹材料色差进行测试,分析评估色差测量过程中不确定度的来源,以进一步提高检测水平,确保测量结果的准确性和可靠性。
结果显示,试样重复性引入的不确定度较大,设备引入的不确定度影响较小。
关键词:字迹材料,色差,不确定度,评估DOI编码:10.3969/j.issn.1002-5944.2023.24.032Evaluation and Analysis of Uncertainty in Color Difference Measurement in the Durability of Handwriting MaterialsZHAO Ping YANG Xiao-chen CHEN Ya-hua LIU Fei(Shanghai Institute of Quality Inspection and Technical Research)Abstract:This paper introduces the common evaluation methods for durability of handwriting materials, and measures the color difference of handwriting material according to DA/T 16-1995, Durability testing method for archival handwriting materials. It analyzes and evaluates the source of uncertainty in contact angle measurement to further improve the testing level and ensure the accuracy and reliability of measurement results. The result shows that the uncertainty introduced by repetitive measurement was more than that introduced by equipment.Keywords: handwriting materials, color difference, uncertainty, evaluation1 背景介绍1.1 字迹材料纸质档案是纸张及与纸张结合形成的字迹材料组成,这些材料记载了人文社科等发展及活动,是历史见证,具有重要的价值。
纳米外衣作文英语

纳米外衣作文英语Nano-clothing。
With the rapid development of science and technology, the application of nanotechnology in various fields has become more and more extensive. One of the most promising applications is the development of nano-clothing. Nano-clothing refers to clothing that has been treated with nanotechnology to improve its performance in terms of durability, comfort, and functionality. This technology has the potential to revolutionize the textile industry and change the way we think about clothing.One of the key benefits of nano-clothing is its durability. By treating the fabric with nanomaterials, it becomes more resistant to wear and tear, making it last longer than traditional clothing. This is especially important in today's fast fashion industry, where clothing is often discarded after only a few wears. Nano-clothing has the potential to reduce the environmental impact of thefashion industry by creating longer-lasting, more sustainable clothing.In addition to durability, nano-clothing also offers improved comfort. Nanotechnology allows for the creation of fabrics that are more breathable, moisture-wicking, and temperature-regulating. This means that nano-clothing can keep the wearer cool in hot weather and warm in cold weather, making it more versatile and comfortable to wear in a variety of conditions. This is especially importantfor outdoor enthusiasts and athletes who rely on their clothing to perform under demanding conditions.Furthermore, nano-clothing can also offer additional functionality. For example, nanotechnology can be used to create fabrics that are water-repellent, stain-resistant, and even antibacterial. This means that nano-clothing can stay clean and fresh for longer, reducing the need for frequent washing and extending the life of the garment. Additionally, nano-clothing can be designed with built-in UV protection, making it ideal for outdoor activities and protecting the wearer from the harmful effects of the sun.Overall, the development of nano-clothing has the potential to revolutionize the textile industry and change the way we think about clothing. By improving durability, comfort, and functionality, nano-clothing has the potential to create longer-lasting, more sustainable clothing that meets the needs of today's consumers. As nanotechnology continues to advance, we can expect to see even more innovative applications of nano-clothing in the future.。
介绍微雕技艺作文英文
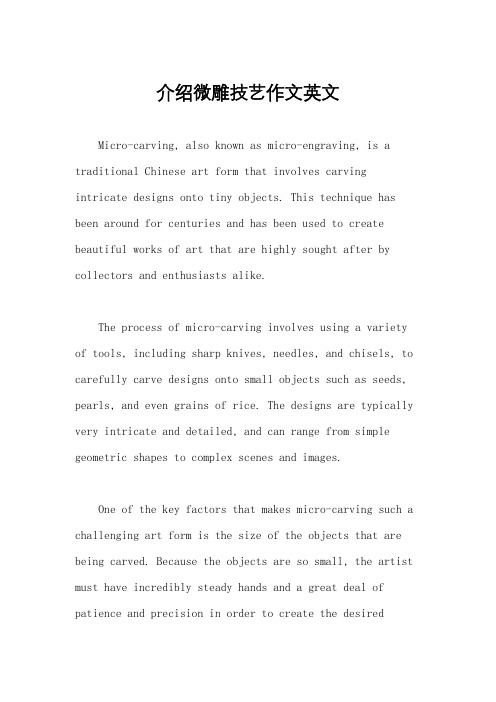
介绍微雕技艺作文英文Micro-carving, also known as micro-engraving, is a traditional Chinese art form that involves carvingintricate designs onto tiny objects. This technique has been around for centuries and has been used to create beautiful works of art that are highly sought after by collectors and enthusiasts alike.The process of micro-carving involves using a variety of tools, including sharp knives, needles, and chisels, to carefully carve designs onto small objects such as seeds, pearls, and even grains of rice. The designs are typically very intricate and detailed, and can range from simple geometric shapes to complex scenes and images.One of the key factors that makes micro-carving such a challenging art form is the size of the objects that are being carved. Because the objects are so small, the artist must have incredibly steady hands and a great deal of patience and precision in order to create the desireddesign. Additionally, the tools used in micro-carving are often very delicate and require a great deal of skill touse properly.Despite the challenges involved, micro-carving has become increasingly popular in recent years, with manyartists and enthusiasts around the world working to perfect their skills in this unique art form. Some of the most impressive micro-carvings can sell for thousands of dollars, making this a highly lucrative field for those who are skilled enough to succeed.Overall, micro-carving is a fascinating and highly intricate art form that requires a great deal of skill and patience to master. Whether you are a collector or simplyan admirer of beautiful art, there is no denying the incredible beauty and complexity of these tiny masterpieces.。
发明纳米涂层眼睛作文四百字
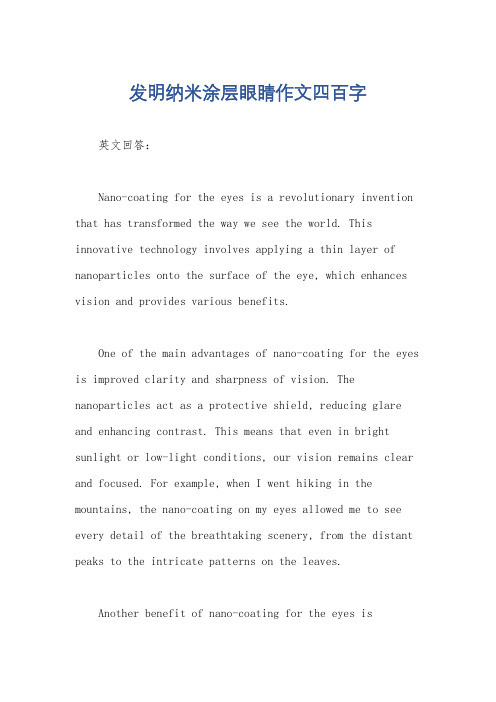
发明纳米涂层眼睛作文四百字英文回答:Nano-coating for the eyes is a revolutionary invention that has transformed the way we see the world. This innovative technology involves applying a thin layer of nanoparticles onto the surface of the eye, which enhances vision and provides various benefits.One of the main advantages of nano-coating for the eyes is improved clarity and sharpness of vision. The nanoparticles act as a protective shield, reducing glare and enhancing contrast. This means that even in bright sunlight or low-light conditions, our vision remains clear and focused. For example, when I went hiking in the mountains, the nano-coating on my eyes allowed me to see every detail of the breathtaking scenery, from the distant peaks to the intricate patterns on the leaves.Another benefit of nano-coating for the eyes isincreased resistance to dust and dirt. The nanoparticles create a hydrophobic layer that repels water and prevents particles from sticking to the eye's surface. This is particularly useful in dusty or polluted environments, as it reduces the need for constant eye rubbing or cleaning. For instance, when I visited a construction site, the nano-coating on my eyes prevented dust particles from irritating my eyes, allowing me to focus on my work without any discomfort.Furthermore, nano-coating for the eyes offersprotection against harmful UV rays. The nanoparticles have the ability to absorb and block UV radiation, reducing the risk of eye damage and conditions such as cataracts. This is especially important for individuals who spend a lot of time outdoors or in sunny climates. For example, when I went to the beach, the nano-coating on my eyes provided an extra layer of protection against the intense UV rays, ensuring that my eyes remained safe and healthy.In addition to these benefits, nano-coating for the eyes can also be customized to address specific visionproblems. By adjusting the composition and properties ofthe nanoparticles, it is possible to correct refractive errors such as nearsightedness or farsightedness. This eliminates the need for traditional eyeglasses or contact lenses, providing a more convenient and comfortable solution. For instance, my friend had a mild case of astigmatism, and after getting the nano-coating treatment, his vision improved significantly, allowing him to see clearly without the need for corrective lenses.中文回答:纳米涂层眼睛是一项革命性的发明,改变了我们看世界的方式。
哈里伯顿仪器和服务明细
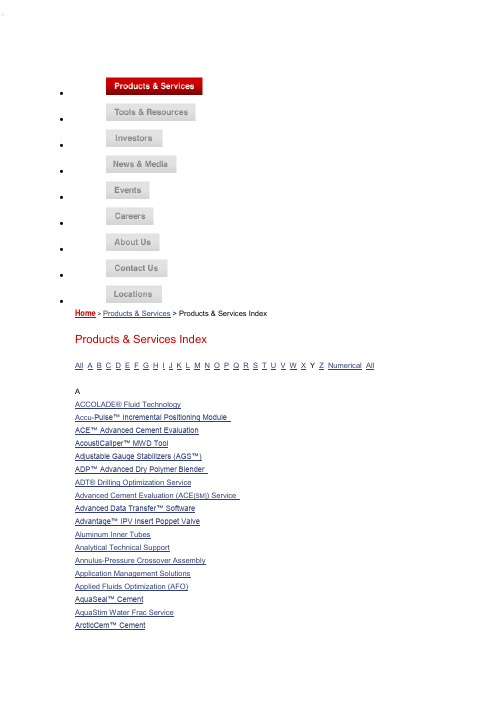
Bi-modal AcousTic Sensor (BAT™)
Bioassay Technical Support
BMR 100-Barrel Batch-Mixer Trailer
BondCem™ Cements
BondSeal™ Cement
Borate Crosslinked Fluids
AssetJournal™ Project Documentation Tool
AssetObserver™ real-time field surveillance
AssetPlanner™ Field Development Planning Software
AssetSolver™ real-time field management
CleanStream® Service
CleanWave™ Water Treatment System
CoalStim® Service
Cobra Frac® Service
CobraJet Frac® Service
CobraMax® H Fracturing Service
CobraMax® V Fracturing Service
Constant Volume Extractor (CVE )
Constrictor® Annular Barrier Tool
Control Line Clamps and Protectors
Conventional Full Closure System
Core Freezing
Core Gamma Logger
AutoLatch™ and Release Perforating Gun Connector
西门子PLM软件Parasolid 产品介绍说明书
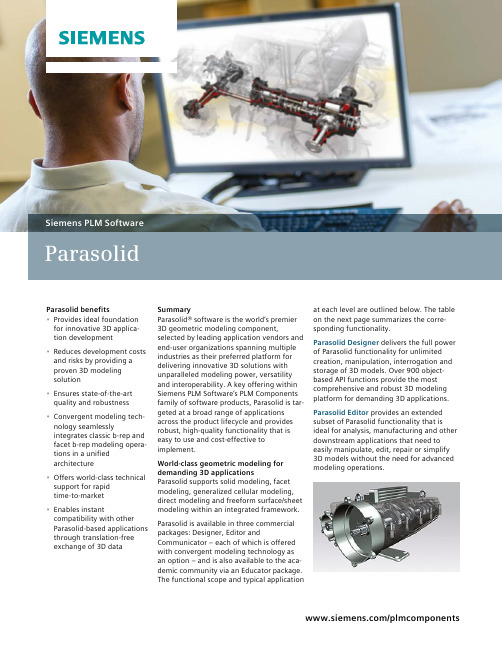
Siemens PLM Software ParasolidSummaryParasolid® software is the world’s premier 3D geometric modeling component, selected by leading application vendors and end-user organizations spanning multiple industries as their preferred platform for delivering innovative 3D solutions with unparalleled modeling power, versatility and interoperability. A key offering within Siemens PLM Software’s PLM Components family of software products, Parasolid is tar-geted at a broad range of applications across the product lifecycle and provides robust, high-quality functionality that is easy to use and cost-effective to implement.World-class geometric modeling for demanding 3D applications Parasolid supports solid modeling, facet modeling, generalized cellular modeling, direct modeling and freeform surface/sheet modeling within an integrated framework. Parasolid is available in three commercial packages: Designer, Editor and Communicator – each of which is offered with convergent modeling technology as an option – and is also available to the aca-demic community via an Educator package. The functional scope and typical application at each level are outlined below. The table on the next page summarizes the corre-sponding functionality.Parasolid Designer delivers the full power of Parasolid functionality for unlimited creation, manipulation, interrogation and storage of 3D models. Over 900 object-based API functions provide the most comprehensive and robust 3D modeling platform for demanding 3D applications. Parasolid Editor provides an extended subset of Parasolid functionality that is ideal for analysis, manufacturing and other downstream applications that need to easily manipulate, edit, repair or simplify 3D models without the need for advanced modeling operations.Parasolid benefits• Provides ideal foundationfor innovative 3D applica-tion development• Reduces development costsand risks by providing aproven 3D modelingsolution• Ensures state-of-the-artquality and robustness• Convergent modeling tech-nology seamlesslyintegrates classic b-rep andfacet b-rep modeling opera-tions in a unifiedarchitecture• Offers world-class technicalsupport for rapidtime-to-market• Enables instantcompatibility with otherParasolid-based applicationsthrough translation-freeexchange of 3D data/plmcomponentsParasolidPLM COMPONENTSParasolid Communicator comprises versatile base functionality, including interoper a bility, visualization and data interro g ation capabili-ties, that provides a platform for applications to consume existing 3D models.Parasolid Educator, complementing the above commercial packages, provides academic institutions with the full power of Parasolid functionality for teaching,research and industrial collaboration.Parasolid facts• Fully integrated modeling of 3D curves, surfaces and solids with over 900 API functions • Modeling foundation for hundreds of the world’s leading CAD, CAM and CAE applications• Corporate standard forSiemens’ NX™, Solid Edge®, Femap™ and Teamcenter® software solutions • Used in over 3.5 million seats of application soft-ware globally• Licensed by 170 software vendors for integration into more than 350 applications • Provides industry-leading robustness with over a mil-lion quality tests run daily • Provides unmatched two-way data compatibility via Parasolid native XT format Parasolid usageParasolid is the component of choice for both cloud-based solutions and traditional stand-alone workstations. Parasolid is deployed across a wide range of PLM application domains, including:• Mechanical CAD • CAM/manufacturing • CAE/validation • AEC• Visualization • Data exchange • Interoperability • Knowledge-based engineering • CMM/inspection • CNC/machine tools • Corporate R&D • Academic R&DPLM COMPONENTSFoundation capabilitiesParasolid is built on critical foundation capabilities that enable Parasolid to be deployed successfully in a wide variety of software applications. Enabled across all relevant functionality, Parasolid foundation capabilities include:• Tolerant modeling for intrinsically reliable modeling with imported data• Convergent modeling technology, available as a licensed option, seamlessly integrates classic b-rep and facet b-rep modeling operations in a unified architecture• Attributes and callbacks for application-specific character-istics and behavior• Session and partitioned rollback for flexible history and undo/redo implementation• Data management and tracking for managing models and associated data as they evolve• Thread safety and symmetric multi-processing support for optimal performance on multi-processor machines• Model storage in forwards and backwards compatible native XT format• .NET Binding to integrate Parasolid into .NET applications written in C#• Broad platform coverage including comprehensive support for Windows, Linux, Unix and MacGetting startedParasolid is delivered with a compre h ensive set of documen-tation and developer resources, including a complete Jumpstart Kit of tools that promote easy integration of Parasolid into new and existing applications:• Full Product Documentation Suite in html and pdf formats • Parasolid Workshop prototypingenvironment for Windows• Example Application Resourcesto get you up and running• Code Example Suite illustratesbest implementation practice• Parasolid ‘Getting Started’ Guideanswers your questions• Parasolid Overview summarizesParasolid capabilities• Parasolid API Training Materialsto educate the team Support, training and consultingParasolid has a renowned technical support, training and consulting team, dedicated to helping customers achieve the best possible implementation by providing expert advice on all matters related to Parasolid usage.Responsive telephone and email support is backed by an online support center that provides round-the-clock access to frequent product updates, as well as customer-specific issue reporting and tracking.In addition, specialized training and consulting services are available that can be tailored to customer requirements. Whether you are starting fresh, extending an existing appli-cation or transitioning from other modeling technology, the Parasolid support, training and consulting team is with you every step of the way.Interoperability productsThe Parasolid product suite is augmented by a range of add-on products that provide high-quality interoperability with third-party CAD data. These include Parasolid Bodyshop, a specialized tool for boosting the success of 3D data exchange by cleaning and repairing imported models, and Parasolid Translator toolkits for converting model data between Parasolid and other major standard and proprietary CAD formats, including STEP, IGES, Catia V4, Catia V5, Pro/ Engineer and ACIS(SAT).Siemens PLM Software partners with Tech Soft 3D to offer Hoops Exchange. This highly-integrated and industry-proven 3D data collaboration solution for Parasolid provides high performance import, export, healing and visualization toolsfor a wide range of 3D file formats.Siemens PLM SoftwareAmericas +1 314 264 8499Europe +44 (0) 1276 413200Asia-Pacific +852 2230 3308/plmrespective holders.5661-Y7 3/16 BConvergent modeling: facetmodel of knee joint withb-rep surgical guide, mod-eled in single architecture.。
鲨鱼表皮的仿生原理对人类的作用英语作文
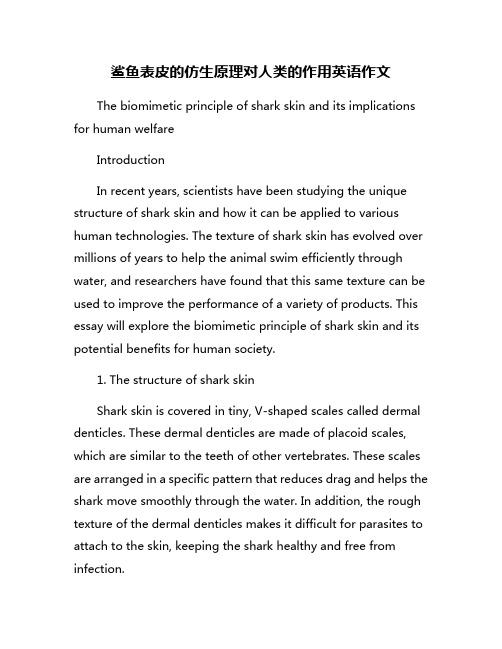
鲨鱼表皮的仿生原理对人类的作用英语作文The biomimetic principle of shark skin and its implications for human welfareIntroductionIn recent years, scientists have been studying the unique structure of shark skin and how it can be applied to various human technologies. The texture of shark skin has evolved over millions of years to help the animal swim efficiently through water, and researchers have found that this same texture can be used to improve the performance of a variety of products. This essay will explore the biomimetic principle of shark skin and its potential benefits for human society.1. The structure of shark skinShark skin is covered in tiny, V-shaped scales called dermal denticles. These dermal denticles are made of placoid scales, which are similar to the teeth of other vertebrates. These scales are arranged in a specific pattern that reduces drag and helps the shark move smoothly through the water. In addition, the rough texture of the dermal denticles makes it difficult for parasites to attach to the skin, keeping the shark healthy and free from infection.2. Biomimicry in human technologyInspired by the structure of shark skin, scientists and engineers have developed a variety of products that mimic its properties. One of the most notable examples is the development of sharkskin-inspired swimsuits. These swimsuits are designed with a textured surface that reduces drag in the water, allowing swimmers to move more quickly and efficiently. In addition, the swimsuits are resistant to bacteria growth, making them ideal for competitive athletes.3. Other applications of shark skin biomimicryIn addition to swimsuits, shark skin biomimicry has been used in a variety of other products. For example, researchers have developed anti-bacterial coatings for medical equipment that mimic the texture of shark skin. These coatings help prevent the growth of harmful bacteria on the surface of the equipment, reducing the risk of infection for patients. In addition, shark skin-inspired coatings have been applied to ships and submarines to reduce drag and increase fuel efficiency.4. Implications for human societyThe biomimetic principle of shark skin has the potential to revolutionize a wide range of industries, from sports andrecreation to healthcare and transportation. By harnessing the unique properties of shark skin, researchers are developing products that are more efficient, durable, and environmentally friendly. In addition, biomimicry helps us better understand the natural world and how we can learn from it to improve our own technologies.ConclusionThe biomimetic principle of shark skin has opened up a world of possibilities for human society. By studying and replicating the unique structure of shark skin, scientists and engineers are creating products that are faster, more durable, and more sustainable. As we continue to explore the natural world and learn from its innovations, we can look forward to a future filled with exciting new technologies inspired by the wonders of nature.。
纳米变形衣下雨也不怕的作文

纳米变形衣下雨也不怕的作文英文回答:In the realm of technological advancements, scientists have unlocked the potential of nanotechnology to create groundbreaking materials with exceptional properties. One such innovation is the emergence of shape-shifting fabrics, commonly known as morphing textiles. These extraordinary materials possess the ability to adapt their shape and structure in response to external stimuli, paving the way for a multitude of applications.Morphing textiles are meticulously engineered using advanced nanomaterials. These materials are meticulously structured to exhibit unique molecular configurations that enable them to respond to environmental cues such as temperature, humidity, and electromagnetic fields. By precisely manipulating the molecular arrangements, scientists can design fabrics that morph into specific shapes or perform tailored functions.One of the most remarkable applications of morphing textiles lies in the realm of protective clothing. Researchers have developed innovative garments that can seamlessly adapt to the wearer's movements and provide enhanced protection in various environments. These shape-shifting clothes can be programmed to adjust their porosity, allowing for optimal breathability during physical exertion while maintaining water resistance in rainy conditions.Imagine a scenario where you encounter a sudden downpour while wearing a nanomorphing garment. The fabric, embedded with moisture-sensitive nanomaterials, wouldswiftly respond to the increased humidity. The molecular structure of the fabric would reconfigure, reducing its porosity to prevent rainwater from penetrating through the garment. As a result, you would remain dry and comfortable, unfazed by the relentless rain.中文回答:纳米变形服,雨天也不怕。
模仿鲸鱼发明潜水服的英语作文
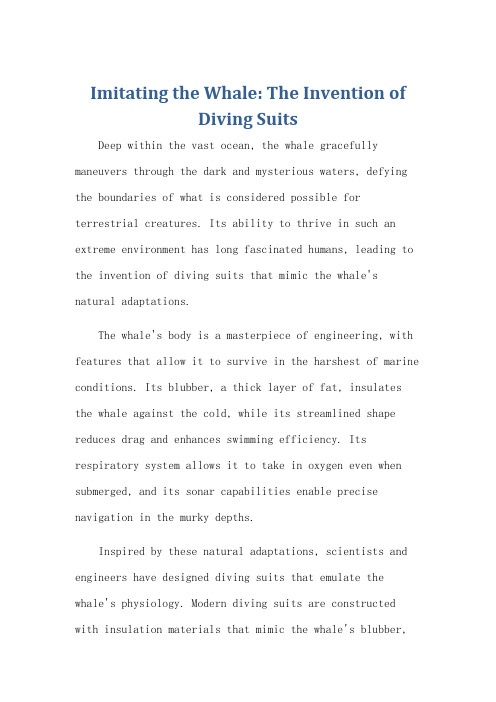
Imitating the Whale: The Invention ofDiving SuitsDeep within the vast ocean, the whale gracefully maneuvers through the dark and mysterious waters, defying the boundaries of what is considered possible forterrestrial creatures. Its ability to thrive in such an extreme environment has long fascinated humans, leading to the invention of diving suits that mimic the whale's natural adaptations.The whale's body is a masterpiece of engineering, with features that allow it to survive in the harshest of marine conditions. Its blubber, a thick layer of fat, insulates the whale against the cold, while its streamlined shape reduces drag and enhances swimming efficiency. Its respiratory system allows it to take in oxygen even when submerged, and its sonar capabilities enable precise navigation in the murky depths.Inspired by these natural adaptations, scientists and engineers have designed diving suits that emulate the whale's physiology. Modern diving suits are constructed with insulation materials that mimic the whale's blubber,keeping divers warm even in frigid water temperatures. The suits are shaped to reduce drag and enhance maneuverability, allowing divers to move with the same ease as the whale.Moreover, the invention of self-contained breathing apparatuses has allowed divers to breathe underwater for extended periods, much like the whale's ability to take in oxygen while submerged. These breathing apparatuses, often referred to as scuba tanks, provide a continuous supply of oxygen, enabling divers to explore deeper and for longer durations.Another remarkable aspect of whale biology that hasbeen replicated in diving suits is the whale's sonar capabilities. Sonar, which stands for sound navigation and ranging, is a technique used by whales to detect objects in their environment. Similarly, modern diving suits are equipped with sonar devices that allow divers to navigate accurately in low-visibility conditions. These devices emit sound waves that bounce off objects and return to the suit, providing information about the surrounding environment.The imitation of the whale's sonar capabilities indiving suits has revolutionized underwater exploration. Ithas enabled divers to navigate through caves, reefs, and other complex underwater landscapes with unprecedented precision. This technology has also been crucial in search and rescue operations, allowing divers to locate missing persons or objects even in the most challenging conditions. In addition to these technological advancements, the whale's biology has also influenced the design of diving suits in terms of comfort and ergonomics. The suits are designed to fit snugly, yet comfortably, allowing divers to move freely without restriction. The materials used are also chosen for their durability and flexibility, ensuring that the suits can withstand the rigors of underwater exploration.In conclusion, the imitation of the whale's adaptations in the invention of diving suits represents a remarkable feat of human ingenuity. By mimicking the whale's natural abilities, we have been able to extend our reach into the depths of the ocean, revealing its secrets and wonders. As technology continues to advance, we can expect further innovations in diving suits that will allow us to exploreeven deeper and further into the mysterious realm of the sea.**模仿鲸鱼:潜水服的发明**在浩瀚的海洋深处,鲸鱼优雅地穿梭在黑暗而神秘的水域中,打破了陆地生物所能达到的极限。
介绍微雕手艺的作文
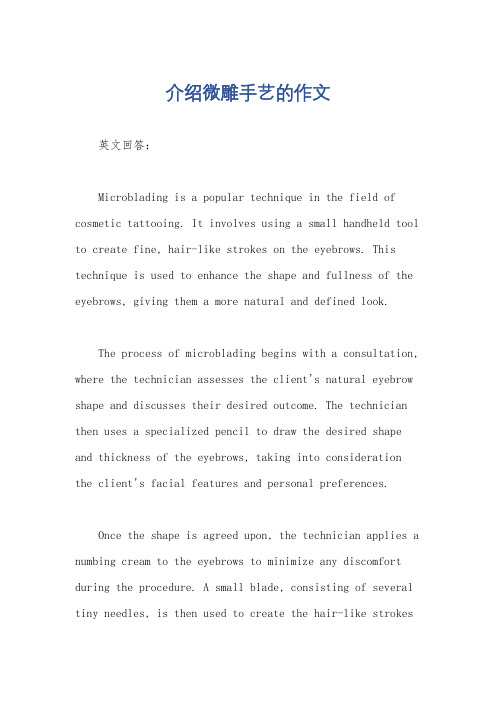
介绍微雕手艺的作文英文回答:Microblading is a popular technique in the field of cosmetic tattooing. It involves using a small handheld tool to create fine, hair-like strokes on the eyebrows. This technique is used to enhance the shape and fullness of the eyebrows, giving them a more natural and defined look.The process of microblading begins with a consultation, where the technician assesses the client's natural eyebrow shape and discusses their desired outcome. The technician then uses a specialized pencil to draw the desired shape and thickness of the eyebrows, taking into consideration the client's facial features and personal preferences.Once the shape is agreed upon, the technician applies a numbing cream to the eyebrows to minimize any discomfort during the procedure. A small blade, consisting of several tiny needles, is then used to create the hair-like strokesby depositing pigment into the superficial layers of the skin. The strokes are carefully placed to mimic the natural direction and growth pattern of eyebrow hairs.The entire process usually takes about two hours, with the initial appointment including the consultation and the actual microblading procedure. After the procedure, the client may experience some redness and mild swelling, but this typically subsides within a few hours or days. It is important to follow the aftercare instructions provided by the technician to ensure proper healing and long-lasting results.One of the advantages of microblading is its longevity. The results can last anywhere from one to three years, depending on factors such as skin type, lifestyle, and exposure to sunlight. Touch-up appointments are recommended every six to twelve months to maintain the desired shape and color of the eyebrows.中文回答:微雕是美容纹身领域中一种受欢迎的技术。
仿生假肢英语作文

仿生假肢英语作文Bionic limbs are truly amazing technological advancements that have transformed the lives of many individuals. Imagine someone who's lost a limb due to an accident or illness. Before the invention of bionic limbs, they might have felt helpless and limited. But now, with these innovative prosthetics, they can regain their confidence and lead a normal life.One of the coolest things about bionic limbs is how they mimic the functions of natural limbs. They're designed to move and feel like real limbs, allowing users to perform tasks that were once unimaginable. Whether it's picking up a cup or playing a sport, bionic limbs make it possible for people to do what they love without limitations.Another great thing about these prosthetics is how customizable they are. Each bionic limb is tailored to fit the unique needs and preferences of the user. This ensures that they feel comfortable and natural, like an extensionof their own body. Plus, with advancements in technology, bionic limbs are becoming more and more realistic, making it harder to distinguish them from the real thing.Of course, bionic limbs aren't perfect. They can be expensive, and some users may experience discomfort or difficulty adapting to them. But despite these challenges, the benefits of having a bionic limb far outweigh the drawbacks. They give people a sense of freedom and independence that they might have never thought they'd。
介绍眼镜的英语作文
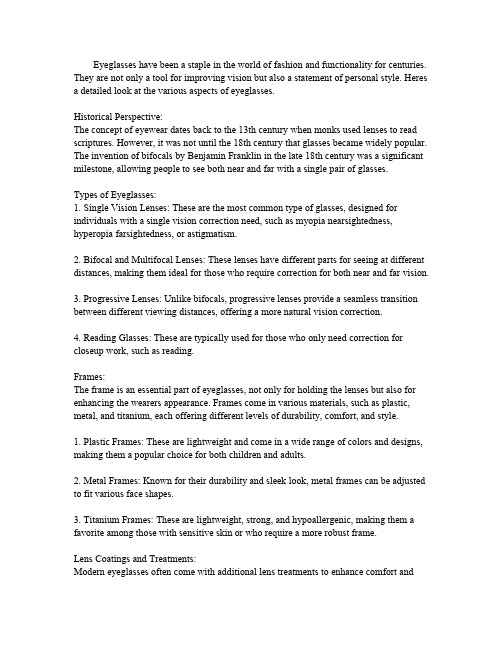
Eyeglasses have been a staple in the world of fashion and functionality for centuries. They are not only a tool for improving vision but also a statement of personal style.Heres a detailed look at the various aspects of eyeglasses.Historical Perspective:The concept of eyewear dates back to the13th century when monks used lenses to read scriptures.However,it was not until the18th century that glasses became widely popular. The invention of bifocals by Benjamin Franklin in the late18th century was a significant milestone,allowing people to see both near and far with a single pair of glasses.Types of Eyeglasses:1.Single Vision Lenses:These are the most common type of glasses,designed for individuals with a single vision correction need,such as myopia nearsightedness, hyperopia farsightedness,or astigmatism.2.Bifocal and Multifocal Lenses:These lenses have different parts for seeing at different distances,making them ideal for those who require correction for both near and far vision.3.Progressive Lenses:Unlike bifocals,progressive lenses provide a seamless transition between different viewing distances,offering a more natural vision correction.4.Reading Glasses:These are typically used for those who only need correction for closeup work,such as reading.Frames:The frame is an essential part of eyeglasses,not only for holding the lenses but also for enhancing the wearers appearance.Frames come in various materials,such as plastic, metal,and titanium,each offering different levels of durability,comfort,and style.1.Plastic Frames:These are lightweight and come in a wide range of colors and designs, making them a popular choice for both children and adults.2.Metal Frames:Known for their durability and sleek look,metal frames can be adjusted to fit various face shapes.3.Titanium Frames:These are lightweight,strong,and hypoallergenic,making them a favorite among those with sensitive skin or who require a more robust frame.Lens Coatings and Treatments:Modern eyeglasses often come with additional lens treatments to enhance comfort andfunctionality.1.AntiReflective Coating:Reduces glare from light sources,improving vision in lowlight conditions and reducing eye strain.2.UV Protection:Protects the eyes from harmful ultraviolet rays,which can cause longterm damage to the eyes.3.Blue Light Blocking:Helps to reduce the effects of digital eye strain by filtering out highenergy visible HEV light emitted by digital screens.Prescription and Fitting:Getting the right prescription is crucial for optimal vision and comfort.An eye exam by an optometrist or ophthalmologist is necessary to determine the correct lens power and any additional treatments needed.Fashion and Personal Style:Eyeglasses have transcended their functional purpose and have become a fashion accessory.They can be chosen to complement ones face shape,skin tone,and personal style.Designer brands offer a range of options,from classic to avantgarde,allowing individuals to express their personality through their eyewear.Maintenance and Care:Proper care of eyeglasses is essential for maintaining their effectiveness and appearance. This includes cleaning the lenses regularly with a microfiber cloth,storing them in a protective case when not in use,and handling them with care to avoid damage.In conclusion,eyeglasses are more than just a necessity for those with vision impairments they are a reflection of ones personal style and a testament to the advancements in technology and design.Whether for practical use or as a fashion statement,eyeglasses have become an integral part of our daily lives.。
隐形眼镜和带框眼镜对比英语作文
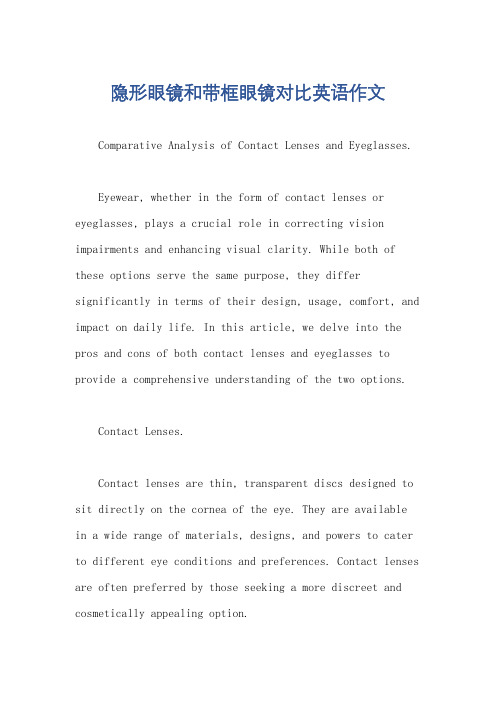
隐形眼镜和带框眼镜对比英语作文Comparative Analysis of Contact Lenses and Eyeglasses.Eyewear, whether in the form of contact lenses or eyeglasses, plays a crucial role in correcting vision impairments and enhancing visual clarity. While both of these options serve the same purpose, they differ significantly in terms of their design, usage, comfort, and impact on daily life. In this article, we delve into the pros and cons of both contact lenses and eyeglasses to provide a comprehensive understanding of the two options.Contact Lenses.Contact lenses are thin, transparent discs designed to sit directly on the cornea of the eye. They are availablein a wide range of materials, designs, and powers to cater to different eye conditions and preferences. Contact lenses are often preferred by those seeking a more discreet and cosmetically appealing option.Advantages:1. Cosmetic Appeal: Contact lenses offer a more natural look, as they are not visible from the outside. This is especially beneficial for those who do not want their eyewear to affect their appearance.2. Convenience: Contact lenses are easily inserted and removed, providing flexibility in terms of usage. They are also suitable for sports and other activities that might be hindered by traditional eyeglasses.3. Wider Field of Vision: Contact lenses do not have frames that can obstruct the peripheral vision, providing a wider and clearer field of view.Disadvantages:1. Maintenance: Contact lenses require regular cleaning and disinfecting to prevent eye infections. Improper care can lead to serious eye problems.2. Higher Cost: Contact lenses tend to be more expensive than eyeglasses, especially when considering the cost of replacements and maintenance supplies.3. Not Suitable for Everyone: Contact lenses may not be suitable for people with certain eye conditions or allergies.Eyeglasses.Eyeglasses, on the other hand, consist of two lenses mounted on a frame that sits on the ears and nose. They are available in a variety of styles, materials, and lens options to cater to different needs and preferences. Eyeglasses are often chosen for their ease of use and affordability.Advantages:1. Ease of Use: Eyeglasses are relatively easy to use and maintain. They do not require special cleaningsolutions or disinfection, making them a more convenient option for many.2. Affordability: Eyeglasses are generally more affordable than contact lenses, especially when considering the long-term costs of replacements and maintenance.3. Suitable for Everyone: Eyeglasses are suitable for a wide range of eye conditions and are often recommended by ophthalmologists for children and those with specific eye diseases.Disadvantages:1. Appearance: Eyeglasses can be visually obtrusive, affecting the wearer's appearance. Some people may find them uncomfortable or unfashionable.2. Limited Field of Vision: Eyeglasses can have frames that obstruct the peripheral vision, especially for those with larger frames or heavy lenses.3. Inconvenience: Eyeglasses can be inconvenient in certain situations, such as when playing sports or engaging in activities that require a helmet or goggles.In conclusion, both contact lenses and eyeglasses have their unique advantages and disadvantages. The choice between the two depends largely on personal preferences, eye conditions, lifestyle, and budget. It is advisable to consult an ophthalmologist or optician to determine the best eyewear option for each individual.。
雕刻橡皮人作文英语

雕刻橡皮人作文英语Title: The Art of Carving Rubber Figures。
Carving rubber figures is a delicate art form that requires patience, precision, and creativity. Whether it's crafting intricate details or conveying emotions through simple gestures, the process of sculpting rubber figures is both challenging and rewarding. In this essay, we'll explore the techniques and nuances involved in this fascinating craft.To begin with, the choice of materials is crucial in rubber figure carving. High-quality rubber blocks are preferred, as they offer the right balance of flexibility and durability. Additionally, specialized carving tools such as fine blades and sculpting knives are essential for achieving intricate details and smooth surfaces.One of the key techniques in rubber figure carving is sketching the design onto the rubber block before makingany cuts. This initial outline serves as a guide throughout the carving process, ensuring that the final figure retains its intended shape and proportions. Attention to detail at this stage is crucial, as any mistakes can be difficult to correct later on.Once the design is sketched, the carving process begins. Using sharp carving tools, the artist carefully removes layers of rubber to reveal the desired shape. This requires steady hands and a keen eye for detail, as even theslightest deviation from the original design can alter the final outcome significantly.As the figure starts to take shape, attention is paidto adding texture and depth to the surface. This can be achieved through various carving techniques, such as cross-hatching, stippling, or gouging. Each method creates a different effect, allowing the artist to enhance therealism and expression of the figure.In addition to technical skill, creativity plays a significant role in rubber figure carving. Artists oftendraw inspiration from a wide range of sources, including nature, mythology, and everyday life. By infusing their creations with personal style and imagination, they bring their rubber figures to life in unique and captivating ways.Furthermore, the process of carving rubber figures can be a deeply meditative and therapeutic experience. As the artist immerses themselves in the intricacies of the craft, they enter a state of flow where time seems to stand still. This sense of focus and concentration not only enhances creativity but also provides a welcome escape from the stresses of daily life.Moreover, rubber figure carving is not just about creating visually appealing sculptures; it's also about storytelling and emotional expression. Through subtle gestures and expressions, artists can convey a wide rangeof emotions, from joy and laughter to sadness and contemplation. This ability to evoke feelings and provoke thought is what truly sets apart a masterful rubber figure from a mere work of art.In conclusion, carving rubber figures is a multifaceted art form that requires technical skill, creativity, and emotional depth. From the careful selection of materials to the final touches of detail, every step in the process contributes to the creation of a unique and captivating sculpture. Whether it's a simple figurine or a complex masterpiece, each rubber figure tells a story and invites the viewer to experience the world through the eyes of the artist.。
- 1、下载文档前请自行甄别文档内容的完整性,平台不提供额外的编辑、内容补充、找答案等附加服务。
- 2、"仅部分预览"的文档,不可在线预览部分如存在完整性等问题,可反馈申请退款(可完整预览的文档不适用该条件!)。
- 3、如文档侵犯您的权益,请联系客服反馈,我们会尽快为您处理(人工客服工作时间:9:00-18:30)。
Modeling uncertainty in cutter wear prediction for tunnel boring machinesC. Frenzel 11Department of Mining Engineering, Colorado School of Mines, 1600 Illinois St,Golden, CO, 80401; PH (303) 273-3714; FAX (303) 273-3719; email:cfrenzel@ABSTRACTWear of cutting tools and the excavation performance of tunnel boringmachines (TBM) is influenced by a number of geotechnical parameters. Theprediction model of the Colorado School of Mines uses Uniaxial CompressiveStrength, Brazilian Tensile Strength, and Cerchar Abrasivity Index. Due to thecomplex nature of rock each of these parameters shows variance and there arecorrelations between these parameters.The usual approach to performance and wear prediction is to use averageinput parameters to estimate the “average” result. It is shown that this is not alwayscorrect because of the statistical nature of the input parameters.The approach described in this paper establishes a statistical model for theperformance and wear prediction based on the previously developed model. Since ananalytical solution for variance prediction is not possible for this model a Monte-Carlo simulation is used.PREDICTION OF DISC CUTTER WEARA broadly used model for prediction tunnel boring machine performance and disccutter wear has been developed at the Colorado School of Mines. Its first version waspublished by Ozdemir et al. 1977 and the last version was published by Rostami1997.To predict the wear of disc cutters two steps need to be followed:1. Prediction of the penetration rate2. Prediction of disc cutter lifeThe reason is that cutter life has been shown to be proportional to the rolling distanceof a disc cutter (Frenzel 2010).Penetration Rate. The prediction of the penetration rate is an iterative process sincethere are three basic limitations:• Thrust limit: Depending on the size of the disc cutter the bearing capacity of the disc cutters varies and limits the maximum thrust force that a disc cuttercan withstand.• Torque limit: The main drive of a tunnel boring machine can overcome only a certain maximum torque at a given rotational speed.• Geometrical limit: The geometry of disc cutter rings does not support infinite penetration rates because the shoulders of the cutter ring will eventually touchthe rock surface.D o w n l o a d e d f r o m a s c e l i b r a r y .o r g b y T o n g j i U n i v e r s i t y o n 04/13/14. C o p y r i g h t A S CE .F o r p e r s o n a l u s e o n l y ; a l l r i g h t s r e s e r ve d .Based on geometrical considerations the opening angle ϕ of the contact area betweenthe disc cutter and the rock can be calculated bywhere R is the radius of the disc cutter and p is the penetration rate.The average stress P* in the contact area can be estimated bywhere σc is the uniaxial compressive strength, σt is the Brazilian Tensile Strength(BTS), S is the spacing between adjacent disc cutter positions on the cutterhead, andT is the width of the tip of the cutter ring.The resulting cutting force F on the disc cutter is given byThis resulting force can be separated into a normal and a rolling component. Therolling component is critical because the sum of all rolling forces times their distancefrom the center of the cutterhead yields the required torque for the main drive of theTBM:where nc is the number of disc cutters on the cutterhead and Dc is the excavationdiameter of the cutterhead.These calculations are repeated until one of the three limits as shown abovehas been reached.Disc Cutter Life. The maximum rolling distance until a disc cutter has to be changeddue to primary wear iswhere CAI is the Cerchar Abrasivity index and R the radius of the disc cutter in mm.The average rolling distance of a disc cutter during one revolution of the cutterheadcan be approximated byD o w n l o a d e d f r o m a s c e l i b r a r y .o r g b y T o n g j i U n i v e r s i t y o n 04/13/14. C o p y r i g h t A S CE .F o r p e r s o n a l u s e o n l y ; a l l r i g h t s r e s e r ve d .where D c is the diameter of the cutterhead. Further analysis by Frenzel 2010revealed that actual cutter life was less than the expected value based on primarywear. Hence, a correctionis introduced where g i are the relative weights of the number of disc cutters in thecenter, face, and gage area. The corrections k k and k v can be determined fromTable 1.Table 1. Correction factors for cutter life at different cutterhead positionsCorrection k k Correction k vCentre cutters 0.561.0Face cutters 0.76 1.0Gage cutters 0.92 0.54The maximum number of cutterhead revolutions U cuntil a cutter changeoccurs can be calculated byand net cutter life V c is computed to bewhere S is the spacing of the disc cutters. Based on net cutter life the number of disccutter changes for a given tunnel can be predicted.INPUT PARAMETERSRock Strength. Due to inhomogeneity repeated tests for rock strengths yielddifferent results. If the number of tests is large enough (sufficient sample size) theresults can be used to estimate the (unknown) statistical distribution. That includesthe type of distribution and its parameters.Of course, measuring rock strengths already introduces a certain bias sinceany damage or imperfection of the sample will reduce its (apparent) strength. Bydefinition UCS should be the intact rock strength which means that there is a biastowards underestimating rock strengths using standard laboratory tests.Examples for histograms of UCS tests of rock samples from Hong Kong areshown in figures 1 and 2.D o w n l o a d e d f r o m a s c e l i b r a r y .o r g b y T o n g j i U n i v e r s i t y o n 04/13/14. C o p y r i g h t A S CE .F o r p e r s o n a l u s e o n l y ; a l l r i g h t s r e s e r ve d .Figure 1: Histogram of Uniaxial Compressive Strength of Hong Kong granite (after: Frenzel 2010).Figure 2: Histogram of Uniaxial Compressive Strength of Hong Kong tuff (after: Frenzel 2010).Neither of these two examples represents a normal distribution, but both showa distinct asymmetry. That could be explained by geology: The Hong Kong granitehad its highest strength after being created and weathering started to degrade the freshrock which led to lower rock strength. That results in a skew towards the left (i.e.lower rock strengths).On the other hand the Hong Kong tuff is metamorphic which eventuallyincreased its strength, but not in a perfectly homogenous manner. That explains theskew towards the right (i.e. higher rock strengths).D o w n l o a d e d f r o m a s c e l i b r a r y .o r g b y T o n g j i U n i v e r s i t y o n 04/13/14. C o p y r i g h t A S CE .F o r p e r s o n a l u s e o n l y ; a l l r i g h t s r e s e r ve d .The difficulty to estimate statistical distributions is to obtain a sufficientsample size. This could be substituted by a small number of tests if the type ofdistribution was linked to the geology of the rock. That should be subject of furtherresearch.Cerchar Abrasivity Index. Abrasivity of rock can be characterized by index tests orby its mineralogy. The most common index test is the Cerchar Abrasivity Index(CAI). However, since its results are influenced by a number of rock properties like:• Mineralogy • Strength of grain bounds• Grain size • Shape of grainsthere is an inherent variability to this parameter as well. In addition, several issues have been identified and discussed in regards to the test itself (Plinninger 2002,Rostami 2005, Käsling et al. 2007).Correlations. UCS and BTS are correlated for many specific rock types and this hasbeen shown as a ratio (Gunsallus & Kulhawy 1987, Heyne 1980). Since the ratiobetween UCS and BTS easily ranges from as low as 5 up to 20 there is the questionhow strong this correlation is.A correlation analysis for UCS, BTS, and CAI has been conducted to evaluatevariances and correlations between these parameters. The data were taken from 20different tunneling projects including a range of different rock types. The results aredepicted in table 2.Table 2: Empirical standard deviations and correlation coefficient for UCS,BTS, and CAI from 20 tunneling projects.UCS BTS CAIUCS s =63.3 MPa R =0.79 R =0.75BTS s = 5.6 MPa R =0.75CAI s = 1.5Although the dataset included many different rock types ranging fromsedimentary through metamorphic to igneous and volcanic there are clear positivecorrelations. This means that there is a bigger probability that a sample with a highUCS value also exhibits a high BTS and a high CAI value. The opposite also holdstrue. This has implications on the prediction of cutter life since using average valueswould underestimate the probability of extreme combinations of UCS, BTS, and CAI.STOCHASTIC EXTENSION OF THE PREDICTION MODELThe stochastic model of cutter life prediction can be expressed as the followingfunctionD o w n l o a d e d f r o m a s c e l i b r a r y .o r g b y T o n g j i U n i v e r s i t y o n 04/13/14. C o p y r i g h t A S CE .F o r p e r s o n a l u s e o n l y ; a l l r i g h t s r e s e r ve d .where the penetration p and abrasivity CAI are the random variables. Randomvariables are printed in grey to distinguish them from non-random input parameters.In addition, the penetration p can be expressed as a function of the random variablesUCS and BTS:The stochastic model reflects the variances σ and correlations ρ of the random variables and assumes normal distributions as an approximation:For linear problems an analytical approach can be taken to predict thevariance of the results. However, in this specific case the prediction model is iterativeand non-linear which requires a different approach.Monte-Carlo-Simulation. A numerical solution to calculate the characteristics ofsuch a problem is the Monte-Carlo-Method. It is based on generating a large numberof input data sets and to calculate the result for each input data set. The results canthen be characterized using descriptive statistics.Generating input data sets requires some specific steps to ensure that the datasets exhibit the correct distribution functions and show the correct correlations.Typically, programming language only provide functions for pseudo-random numbergeneration that follow an equal distribution. Three of these random numbers areconverted into normal-distributed random numbers using the central limit theorem.Finally, correlations are introduced by using a Cholesky repartition of the variance-covariance matrix and transformation by the mean.Example. To analyze the consequences of the considerations above an example hasbeen studied. The input parameters are summarized in table 3.Table 3: Input parameters for cutter life predictionMean UCS 150 MPaExcavation diameter 9.0 mMean BTS 10 MPa Disc cutter diameter 432 mmMean CAI 3.8 Max cutter load 267 kNVariance of UCS 50 MPa Spacing 90 mmVariance of BTS 3 MPa Disc cutter tip width 19 mmVariance of CAI 0.5 Number of disc cutters 57Covariance UCS-BTS 0.78 Cutterhead speed 5.3 1/minCovariance UCS-CAI 0.7 Cutterhead torque 5.77 MNmCovariance BTS-CAI 0.7D o w n l o a d e d f r o m a s c e l i b r a r y .o r g b y T o n g j i U n i v e r s i t y o n 04/13/14. C o p y r i g h t A S CE .F o r p e r s o n a l u s e o n l y ; a l l r i g h t s r e s e r ve d .The simulation was run using 10,000 iterations. The resulting distribution ofpenetration and cutter life is shown in figures 3 and 4.Figure 3: Histogram of penetration rate of Monte-Carlo-SimulationFigure 4: Histogram of cutter life of Monte-Carlo-SimulationBoth histograms show a right-skewed distribution which is a result of the non-linearity of the prediction model. The peak at a penetration rate of 10 mm in figure 3D o w n l o a d e d f r o m a s c e l i b r a r y .o r g b y T o n g j i U n i v e r s i t y o n 04/13/14. C o p y r i g h t A S CE .F o r p e r s o n a l u s e o n l y ; a l l r i g h t s r e s e r ve d .can be explained by the transition between thrust and torque limitation as discussedearlier. For comparison purposes table 4 shows the comparison between a predictionusing average values versus the results obtained through Monte-Carlo simulation.Table 4: Comparison of Monte-Carlo simulation and static prediction usingmean input valuesPenetration Rate [mm] Cutter Life [m 3]Monte-Carlo simulation 9.1 486 Mean values without simulation 8.3 398DISCUSSIONThe differences between the Monte-Carlo simulation and the static prediction show adifference of 10% for the average penetration rate and a difference of 22% for cutterlife. The difference for penetration rate prediction is probably neglectable forpractical purposes. However, the difference of 22% for cutter life is significant andcannot be disregarded easily. However, it is important to run the simulation on bothvalues because the penetration rate influencing the cutter life.CONCLUSIONBased on the prediction model for penetration rate and cutter life prediction for tunnelboring machines developed at the Colorado School of Mines a methodologyaccounting for the inherent uncertainties of rock is introduced. The model usesUniaxial Compressive Strength, Brazilian Tensile Strength, and the CercharAbrasivity Index as geotechnical input parameters.Through extensive testing the statistical distribution of these parameters canbe determined. Typically, the type of distribution is a normal distribution, butdepending on geology this may be skewed significantly as shown on the example ofgranite and tuff from Hong Kong.In addition, there are high correlations between these three parameters whichmean that there is a higher probability of a high UCS value occurring along with ahigh BTS value and a high CAI value. This leads to an increased variance of theresulting penetration rate and cutter life compared to the variance of the inputparameters.The existing prediction model can be used as the basis for a Monte-Carlosimulation which incorporates the random nature of the input parameters and whichallows to study the characteristics of the results.Through a calculated example it becomes clear that although the differencesbetween a simulation and a static calculation using average input values may lead tosignificant differences for predicted cutter life.D o w n l o a d e d f r o m a s c e l i b r a r y .o r g b y T o n g j i U n i v e r s i t y o n 04/13/14. C o p y r i g h t A S CE .F o r p e r s o n a l u s e o n l y ; a l l r i g h t s r e s e r ve d .REFERENCESFrenzel, Christian (2010): Verschleisskostenprognose für Schneidrollen beimaschinellen Tunnelvortrieben in Festgesteinen , Münchner GeowissenschaftlicheAbhandlungen, Reihe B, Dr. Friedrich Pfeil, MünchenGunsallus, K.L.; Kulhawy, F. H. (1987): “A comparative evaluation of rock strengthmeasures.” International Journal of Rock Mechanics and Mining Sciences , 24 (3),193–196.Heyne, K.-H. (1980): “Mathematisch-statistische Zusammenhänge zwischengesteinsmechanischen und gesteinsphysikalischen Kennwerten.“ Zeitschrift fürangewandte Geologie, 26 (10), 519–523Käsling, Heiko; Thiele, Inke; Thuro, Kurosch (2007): "Abrasivitätsuntersuchungenmit dem Cerchar-Test - eine Evaluierung der Versuchsbedingungen.“ 16. Tagung fürIngenieurgeologie und Forum "Junge Ingenieurgeologen", Bochum, 229–235Ozdemir, Levent; Miller, Russell; Wang, Fun-Den (1977): Mechanical tunnel boringprediction and machine design . Annual report , Colorado School of Mines, NationalScience Foundation, Washington, DCPlinninger, Ralf J. (2002): Klassifizierung und Prognose von Werkzeugverschleiß beikonventionellen Gebirgslösungsverfahren im Festgestein. Münchner geologischeHefte, Reihe B, MünchenRostami, Jamal (1997): Development of a force estimation model for rockfragmentation with disc cutters through theoretical modeling and physicalmeasurement of crushed zone pressure, PhD thesis, Colorado School of Mines,Golden.Rostami, Jamal (2005): “CAI testing and its implications.” Tunnels & TunnellingInternational , October, 43–45D o w n l o a d e d f r o m a s c e l i b r a r y .o r g b y T o n g j i U n i v e r s i t y o n 04/13/14. C o p y r i g h t A S CE .F o r p e r s o n a l u s e o n l y ; a l l r i g h t s r e s e r v e d .。