逻辑门回路设计生物传感器
生物医学工程中的生物传感器设计
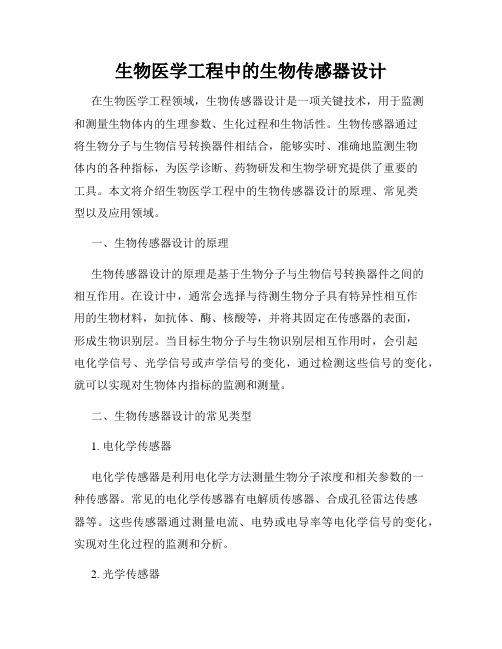
生物医学工程中的生物传感器设计在生物医学工程领域,生物传感器设计是一项关键技术,用于监测和测量生物体内的生理参数、生化过程和生物活性。
生物传感器通过将生物分子与生物信号转换器件相结合,能够实时、准确地监测生物体内的各种指标,为医学诊断、药物研发和生物学研究提供了重要的工具。
本文将介绍生物医学工程中的生物传感器设计的原理、常见类型以及应用领域。
一、生物传感器设计的原理生物传感器设计的原理是基于生物分子与生物信号转换器件之间的相互作用。
在设计中,通常会选择与待测生物分子具有特异性相互作用的生物材料,如抗体、酶、核酸等,并将其固定在传感器的表面,形成生物识别层。
当目标生物分子与生物识别层相互作用时,会引起电化学信号、光学信号或声学信号的变化,通过检测这些信号的变化,就可以实现对生物体内指标的监测和测量。
二、生物传感器设计的常见类型1. 电化学传感器电化学传感器是利用电化学方法测量生物分子浓度和相关参数的一种传感器。
常见的电化学传感器有电解质传感器、合成孔径雷达传感器等。
这些传感器通过测量电流、电势或电导率等电化学信号的变化,实现对生化过程的监测和分析。
2. 光学传感器光学传感器是通过测量光学信号的变化,来实现对生物分子的检测和测量的一种传感器。
光学传感器基于光学原理,通过光的吸收、发射、散射或干扰等现象,来检测目标生物分子的存在和浓度变化。
常见的光学传感器有荧光传感器、表面等离子共振传感器等。
3. 声学传感器声学传感器是利用声学原理测量声波的传播和变化,来实现对生物分子的检测和测量的一种传感器。
声学传感器可以通过测量声波的频率、幅度、相位等变化,来获取生物体内的生理参数和生物活性信息。
常见的声学传感器有超声波传感器、压电传感器等。
三、生物传感器设计的应用领域生物传感器在生物医学工程领域有着广泛的应用。
一方面,在医学诊断中,生物传感器可以用于测量血液中的生物指标,如血糖、血氧饱和度、血压等,可用于糖尿病管理、血液透析监测等。
生物医学工程中的生物传感器设计与应用
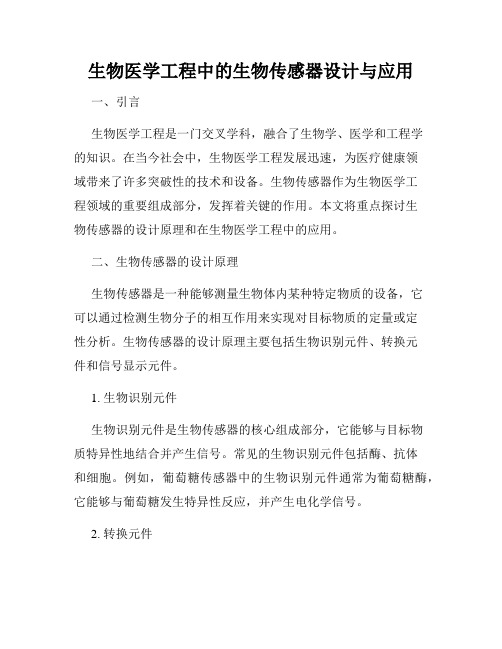
生物医学工程中的生物传感器设计与应用一、引言生物医学工程是一门交叉学科,融合了生物学、医学和工程学的知识。
在当今社会中,生物医学工程发展迅速,为医疗健康领域带来了许多突破性的技术和设备。
生物传感器作为生物医学工程领域的重要组成部分,发挥着关键的作用。
本文将重点探讨生物传感器的设计原理和在生物医学工程中的应用。
二、生物传感器的设计原理生物传感器是一种能够测量生物体内某种特定物质的设备,它可以通过检测生物分子的相互作用来实现对目标物质的定量或定性分析。
生物传感器的设计原理主要包括生物识别元件、转换元件和信号显示元件。
1. 生物识别元件生物识别元件是生物传感器的核心组成部分,它能够与目标物质特异性地结合并产生信号。
常见的生物识别元件包括酶、抗体和细胞。
例如,葡萄糖传感器中的生物识别元件通常为葡萄糖酶,它能够与葡萄糖发生特异性反应,并产生电化学信号。
2. 转换元件转换元件负责将生物识别元件与测量设备之间的信号转换。
常见的转换元件包括电化学传感器、光学传感器和声学传感器。
电化学传感器是应用最为广泛的一种转换元件,它通过测量电流、电荷或电压的变化来实现信号的转换。
3. 信号显示元件信号显示元件将转换后的信号以可视化或数字化的形式显示出来,以便进行分析和判断。
常见的信号显示元件包括显微镜、光电二极管和计算机界面等。
显微镜用于观察生物传感器中的生物反应,并将显微图像转化为数字信号。
三、生物传感器在生物医学工程中的应用生物传感器在生物医学工程中有着广泛的应用,本节将介绍生物传感器在多个领域的应用案例。
1. 健康监测生物传感器能够监测个体的生理参数,如心率、血氧饱和度和呼吸频率等。
通过将传感器与智能手表或智能手机等便携设备相结合,可以实时监测个体的健康状况,并进行远程监护。
2. 疾病诊断生物传感器在疾病诊断中发挥着重要作用。
以癌症为例,生物传感器可以检测癌细胞的特异性标志物,提前发现癌症的存在和发展趋势。
这为疾病的早期诊断和治疗提供了重要的依据。
分子生物逻辑门电路设计及应用
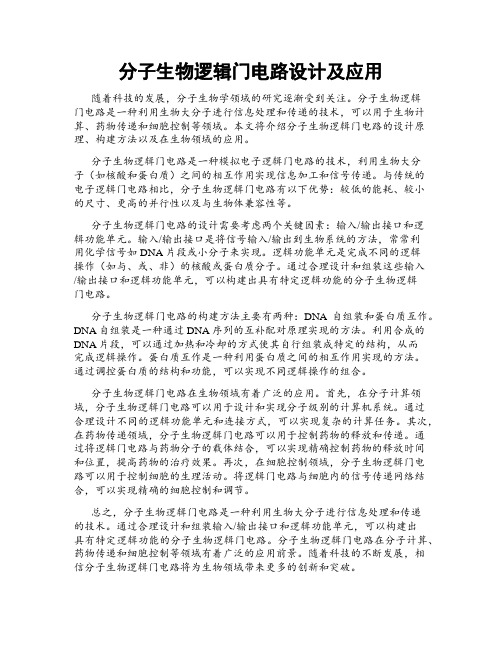
分子生物逻辑门电路设计及应用随着科技的发展,分子生物学领域的研究逐渐受到关注。
分子生物逻辑门电路是一种利用生物大分子进行信息处理和传递的技术,可以用于生物计算、药物传递和细胞控制等领域。
本文将介绍分子生物逻辑门电路的设计原理、构建方法以及在生物领域的应用。
分子生物逻辑门电路是一种模拟电子逻辑门电路的技术,利用生物大分子(如核酸和蛋白质)之间的相互作用实现信息加工和信号传递。
与传统的电子逻辑门电路相比,分子生物逻辑门电路有以下优势:较低的能耗、较小的尺寸、更高的并行性以及与生物体兼容性等。
分子生物逻辑门电路的设计需要考虑两个关键因素:输入/输出接口和逻辑功能单元。
输入/输出接口是将信号输入/输出到生物系统的方法,常常利用化学信号如DNA片段或小分子来实现。
逻辑功能单元是完成不同的逻辑操作(如与、或、非)的核酸或蛋白质分子。
通过合理设计和组装这些输入/输出接口和逻辑功能单元,可以构建出具有特定逻辑功能的分子生物逻辑门电路。
分子生物逻辑门电路的构建方法主要有两种:DNA自组装和蛋白质互作。
DNA自组装是一种通过DNA序列的互补配对原理实现的方法。
利用合成的DNA片段,可以通过加热和冷却的方式使其自行组装成特定的结构,从而完成逻辑操作。
蛋白质互作是一种利用蛋白质之间的相互作用实现的方法。
通过调控蛋白质的结构和功能,可以实现不同逻辑操作的组合。
分子生物逻辑门电路在生物领域有着广泛的应用。
首先,在分子计算领域,分子生物逻辑门电路可以用于设计和实现分子级别的计算机系统。
通过合理设计不同的逻辑功能单元和连接方式,可以实现复杂的计算任务。
其次,在药物传递领域,分子生物逻辑门电路可以用于控制药物的释放和传递。
通过将逻辑门电路与药物分子的载体结合,可以实现精确控制药物的释放时间和位置,提高药物的治疗效果。
再次,在细胞控制领域,分子生物逻辑门电路可以用于控制细胞的生理活动。
将逻辑门电路与细胞内的信号传递网络结合,可以实现精确的细胞控制和调节。
生物传感器的设计及其生命检测应用
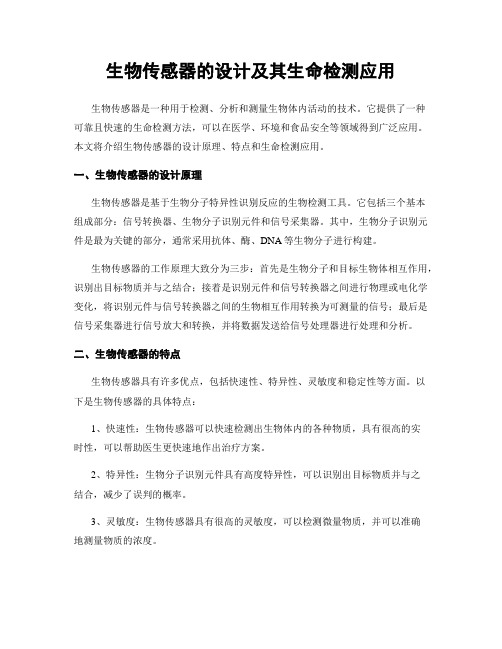
生物传感器的设计及其生命检测应用生物传感器是一种用于检测、分析和测量生物体内活动的技术。
它提供了一种可靠且快速的生命检测方法,可以在医学、环境和食品安全等领域得到广泛应用。
本文将介绍生物传感器的设计原理、特点和生命检测应用。
一、生物传感器的设计原理生物传感器是基于生物分子特异性识别反应的生物检测工具。
它包括三个基本组成部分:信号转换器、生物分子识别元件和信号采集器。
其中,生物分子识别元件是最为关键的部分,通常采用抗体、酶、DNA等生物分子进行构建。
生物传感器的工作原理大致分为三步:首先是生物分子和目标生物体相互作用,识别出目标物质并与之结合;接着是识别元件和信号转换器之间进行物理或电化学变化,将识别元件与信号转换器之间的生物相互作用转换为可测量的信号;最后是信号采集器进行信号放大和转换,并将数据发送给信号处理器进行处理和分析。
二、生物传感器的特点生物传感器具有许多优点,包括快速性、特异性、灵敏度和稳定性等方面。
以下是生物传感器的具体特点:1、快速性:生物传感器可以快速检测出生物体内的各种物质,具有很高的实时性,可以帮助医生更快速地作出治疗方案。
2、特异性:生物分子识别元件具有高度特异性,可以识别出目标物质并与之结合,减少了误判的概率。
3、灵敏度:生物传感器具有很高的灵敏度,可以检测微量物质,并可以准确地测量物质的浓度。
4、稳定性:生物传感器具有很高的稳定性,可以长期使用而不会影响性能,且易于维护和使用。
三、生物传感器的生命检测应用生物传感器在生命检测中的应用非常广泛,主要包括临床医学、环境检测和食品安全等方面。
以下是各个领域中的生物传感器应用举例:1、临床医学生物传感器可以检测血糖、血脂、血氧、血红蛋白等指标,帮助医生及时发现生命体征异常,提高治疗效果。
2、环境检测生物传感器可以检测空气中的有害物质、水质中的重金属和污染物等,可用于环境监测和生态监测。
3、食品安全生物传感器可以检测农药残留、嗅味剂、抗生素等食品中的添加剂及重金属等有害物质,帮助消费者购买更加安全的食品。
生物传感器设计制备及其检测方法
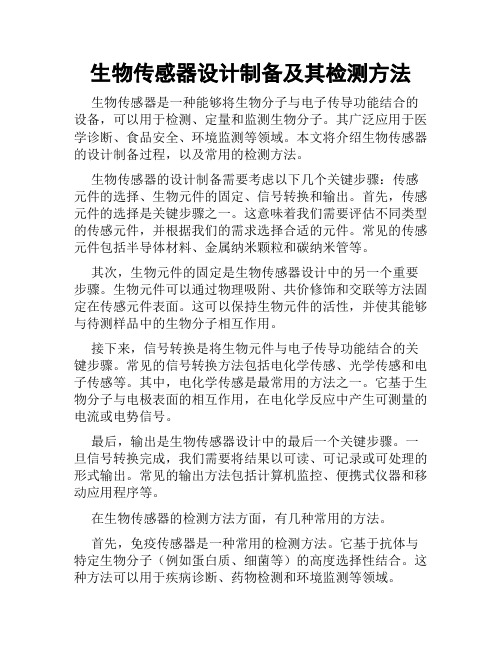
生物传感器设计制备及其检测方法生物传感器是一种能够将生物分子与电子传导功能结合的设备,可以用于检测、定量和监测生物分子。
其广泛应用于医学诊断、食品安全、环境监测等领域。
本文将介绍生物传感器的设计制备过程,以及常用的检测方法。
生物传感器的设计制备需要考虑以下几个关键步骤:传感元件的选择、生物元件的固定、信号转换和输出。
首先,传感元件的选择是关键步骤之一。
这意味着我们需要评估不同类型的传感元件,并根据我们的需求选择合适的元件。
常见的传感元件包括半导体材料、金属纳米颗粒和碳纳米管等。
其次,生物元件的固定是生物传感器设计中的另一个重要步骤。
生物元件可以通过物理吸附、共价修饰和交联等方法固定在传感元件表面。
这可以保持生物元件的活性,并使其能够与待测样品中的生物分子相互作用。
接下来,信号转换是将生物元件与电子传导功能结合的关键步骤。
常见的信号转换方法包括电化学传感、光学传感和电子传感等。
其中,电化学传感是最常用的方法之一。
它基于生物分子与电极表面的相互作用,在电化学反应中产生可测量的电流或电势信号。
最后,输出是生物传感器设计中的最后一个关键步骤。
一旦信号转换完成,我们需要将结果以可读、可记录或可处理的形式输出。
常见的输出方法包括计算机监控、便携式仪器和移动应用程序等。
在生物传感器的检测方法方面,有几种常用的方法。
首先,免疫传感器是一种常用的检测方法。
它基于抗体与特定生物分子(例如蛋白质、细菌等)的高度选择性结合。
这种方法可以用于疾病诊断、药物检测和环境监测等领域。
其次,DNA传感器是一种用于检测DNA序列的方法。
它基于DNA的互补配对原理,通过与待测样品中的目标DNA特异性结合来实现检测。
DNA传感器可以用于基因检测、病原体检测和基因工程领域等。
另外,生物传感器还可以通过酶或菌的活性来实现对底物的检测。
这种方法被称为酶传感器或细菌传感器。
它们可以检测底物的浓度、活性或毒性等。
这种方法广泛应用于食品安全、环境检测和生物燃料领域等。
生物传感器电路设计与优化
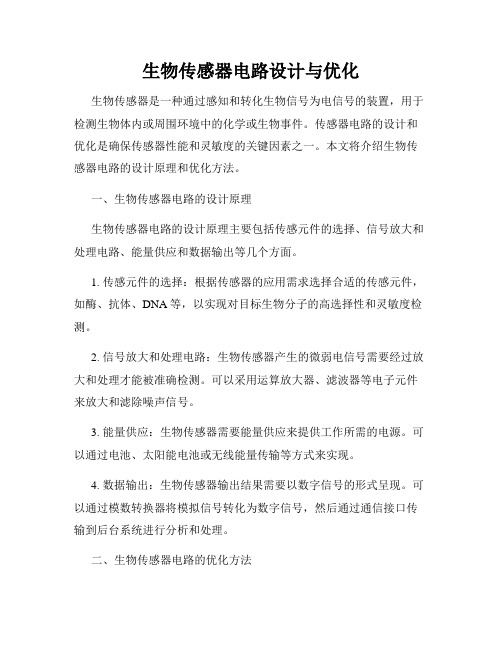
生物传感器电路设计与优化生物传感器是一种通过感知和转化生物信号为电信号的装置,用于检测生物体内或周围环境中的化学或生物事件。
传感器电路的设计和优化是确保传感器性能和灵敏度的关键因素之一。
本文将介绍生物传感器电路的设计原理和优化方法。
一、生物传感器电路的设计原理生物传感器电路的设计原理主要包括传感元件的选择、信号放大和处理电路、能量供应和数据输出等几个方面。
1. 传感元件的选择:根据传感器的应用需求选择合适的传感元件,如酶、抗体、DNA等,以实现对目标生物分子的高选择性和灵敏度检测。
2. 信号放大和处理电路:生物传感器产生的微弱电信号需要经过放大和处理才能被准确检测。
可以采用运算放大器、滤波器等电子元件来放大和滤除噪声信号。
3. 能量供应:生物传感器需要能量供应来提供工作所需的电源。
可以通过电池、太阳能电池或无线能量传输等方式来实现。
4. 数据输出:生物传感器输出结果需要以数字信号的形式呈现。
可以通过模数转换器将模拟信号转化为数字信号,然后通过通信接口传输到后台系统进行分析和处理。
二、生物传感器电路的优化方法生物传感器电路的优化是为了提高传感器的性能和稳定性,减少噪声和干扰信号的影响,从而提高传感器的检测灵敏度和精度。
以下是几种常见的优化方法:1. 噪声抑制:通过合理的电路设计和布局,降低电路中的噪声干扰。
可以采用屏蔽和隔离技术、微弱信号放大技术等来减小噪声对传感器输出的影响。
2. 敏感度增强:通过改进传感元件的特性或结构设计,提高传感器对目标生物分子的灵敏度。
例如,改变传感元件的结构、优化酶的反应速率等。
3. 电源管理:合理管理传感器所需的能量供应,延长电池寿命或提高太阳能电池的能量转化效率。
可以采用功耗优化技术、能量回收技术等来实现。
4. 信号处理算法优化:通过优化信号处理算法,提高传感器数据的采样率和分析速度,减少误差和延迟,进一步提高传感器的性能。
5. 系统集成:将生物传感器电路与其他系统集成,实现全自动化和实时检测。
生物传感器的设计和应用

生物传感器的设计和应用随着科学技术不断发展,生物传感器也因其广泛的应用领域和高精度的测量而逐渐成为研究热点。
生物传感器是一种能够将生理、化学和物理量转换成电信号的设备。
它利用感受器和信号处理器来将生物信号转化为可测量的电信号,从而使人们能够更好地了解人体和环境的情况。
本文将介绍生物传感器的设计和应用。
一、生物传感器的设计1. 感受器的选择生物传感器的感受器是将化学、生物或物理量转换成电信号的核心组件。
传感器的类型有许多种,如离子选择性电极、氨氮传感器、荧光传感器等。
为了选择适合特定应用的感受器,需要考虑多个因素,如1)测量的环境,如温度、湿度、流量等;2)分析的目标物质,如离子、分子、细胞等;3)所需的信噪比和灵敏度。
2. 信号传输和处理生物传感器的另一个重要组成部分是信号传输和处理系统。
由于生物传感器测量的信号小而且噪音大,需要合适的系统进行信号增强和噪音抑制。
传统的信号处理方法如滤波、放大和校准等,而现代技术如数字信号处理和特定算法能够更好地从复杂的背景信号中解析出目标信号。
3. 器件的集成生物传感器的器件集成是使其更加便携、可操控、可靠的一种方法。
因此,在设计生物传感器时,需要考虑如何将感受器和信号处理器集成在一起,并确保器件符合生物和医学的要求,如生物相容性和稳定性等。
二、生物传感器的应用生物传感器的应用领域极广,如环境监测、医疗诊断、食品安全等。
以下是其中一些领域的应用举例:1. 环境监测生物传感器可以用于监测空气、水、土壤等环境中的污染物,如重金属、有机化学物和生物毒素等。
传感器将环境中的化学物质转换为电信号,再用信号处理系统判断是否超过环境保护标准。
这种技术可用于及时检测污染源并采取有效的净化手段。
2. 医疗诊断生物传感器可以用于早期诊断和治疗疾病。
例如,通过测量人体液体中的生物指标如葡萄糖和白细胞计数等,可以提前发现和治疗二型糖尿病和白血病等疾病。
生物传感器还可以用于制定个性化的药物治疗计划,根据患者的生理特征和偏好,调整药物剂量和时间表。
生物分子逻辑门-概述说明以及解释
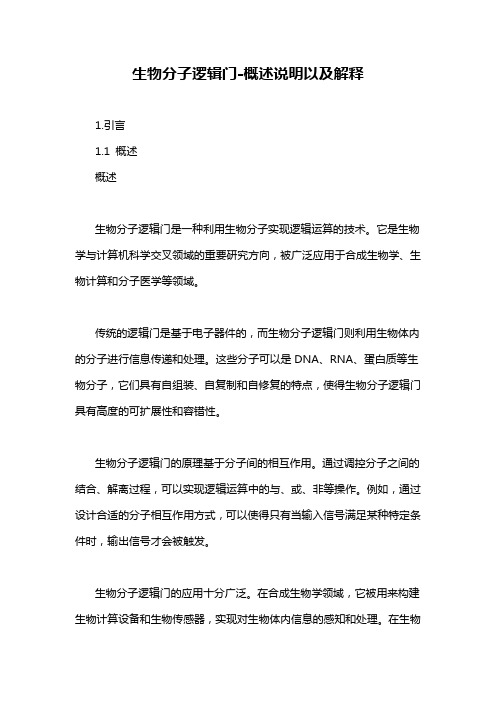
生物分子逻辑门-概述说明以及解释1.引言1.1 概述概述生物分子逻辑门是一种利用生物分子实现逻辑运算的技术。
它是生物学与计算机科学交叉领域的重要研究方向,被广泛应用于合成生物学、生物计算和分子医学等领域。
传统的逻辑门是基于电子器件的,而生物分子逻辑门则利用生物体内的分子进行信息传递和处理。
这些分子可以是DNA、RNA、蛋白质等生物分子,它们具有自组装、自复制和自修复的特点,使得生物分子逻辑门具有高度的可扩展性和容错性。
生物分子逻辑门的原理基于分子间的相互作用。
通过调控分子之间的结合、解离过程,可以实现逻辑运算中的与、或、非等操作。
例如,通过设计合适的分子相互作用方式,可以使得只有当输入信号满足某种特定条件时,输出信号才会被触发。
生物分子逻辑门的应用十分广泛。
在合成生物学领域,它被用来构建生物计算设备和生物传感器,实现对生物体内信息的感知和处理。
在生物医学领域,它被应用于基因治疗、药物筛选等方面,为疾病的治疗和诊断提供了新的思路和方法。
本文将从生物分子逻辑门的定义和原理出发,介绍其基本工作原理和设计方法。
然后,将重点探讨生物分子逻辑门在合成生物学和生物医学领域的应用。
最后,对生物分子逻辑门的发展进行总结,并展望其未来的研究方向和应用前景。
通过对生物分子逻辑门的全面介绍和分析,旨在促进这一领域的研究和应用,为生物信息处理和生物系统工程提供新的思路和方法。
文章结构部分的内容应该是对整篇文章的章节和内容进行介绍和概括。
以下是文章结构部分的内容示例:"1.2 文章结构"本文主要包含引言、正文和结论三个部分。
在引言部分,将对生物分子逻辑门的概述、文章的结构以及目的进行阐述。
在正文部分,将详细介绍生物分子逻辑门的定义和原理,以及其在不同领域的应用。
最后,在结论部分进行总结,概括所讨论的内容,并展望未来生物分子逻辑门的发展方向。
通过以上的文章结构,读者可以清楚地了解到本文将围绕生物分子逻辑门展开,并且获得目录中各个章节的预览。
生物传感器的设计与制备

生物传感器的设计与制备生物传感器是一种能够检测和测定生物体内或生物体外特定生物或非生物物质存在的生物化学传感器。
它具有高灵敏度、高选择性和实时检测等特点,广泛应用于医学诊断、环境监测、食品安全等领域。
是其性能优劣的决定因素,因此对其设计与制备进行深入研究至关重要。
生物传感器设计的关键在于选择合适的生物识别元件和传感器材料。
生物识别元件通常是酶、抗体、细胞等,在不同的应用领域需选择不同的生物识别元件。
例如,在医学诊断中,常用的酶有葡萄糖氧化酶、乳酸脱氢酶等,而在环境监测中则常用的是抗体等。
传感器材料也是影响生物传感器性能的重要因素,常用的传感器材料有金属、半导体、纳米材料等。
金属材料具有良好的导电性和稳定性,半导体材料具有可控性强和灵敏度高等特点,而纳米材料由于其表面积大、质量轻、尺寸小等特性,被广泛用于生物传感器的制备中。
在生物传感器的制备过程中,常见的方法有电化学沉积、溶液旋涂、薄膜沉积、光刻等。
电化学沉积是一种简单有效的制备方法,通过对电极表面施加电压或电流,在电极上生成一层合成的材料。
溶液旋涂是通过将液体旋转到晶片或玻璃片上,形成均匀的薄膜。
薄膜沉积是将薄膜材料溶于溶剂中,通过旋转涂覆等方式,在基底上沉积一层薄膜。
光刻是一种通过光照将光刻胶暴露在紫外线下,再通过显影的方式形成图案的制备方法。
生物传感器的设计与制备过程中还需考虑传感器的检测原理和信号采集系统。
传感器的检测原理包括电化学、光学、声学等,不同的原理影响着生物传感器的灵敏度、选择性和响应速度等性能。
信号采集系统是将生物传感器检测到的信号转换为数字信号,并通过处理器进行处理并输出结果。
常见的信号采集系统有电化学工作站、分光光度计、质谱仪等。
生物传感器的设计与制备涉及到多个学科的知识,如生物学、化学、物理学、材料学等。
生物传感器的优劣不仅取决于生物传感器的设计与制备过程,还受到环境因素、操作条件、样品性质等因素的影响。
因此,在生物传感器设计与制备的研究中,需要综合考虑各种因素,提高生物传感器的性能。
合成生物学在生物修复领域的应用
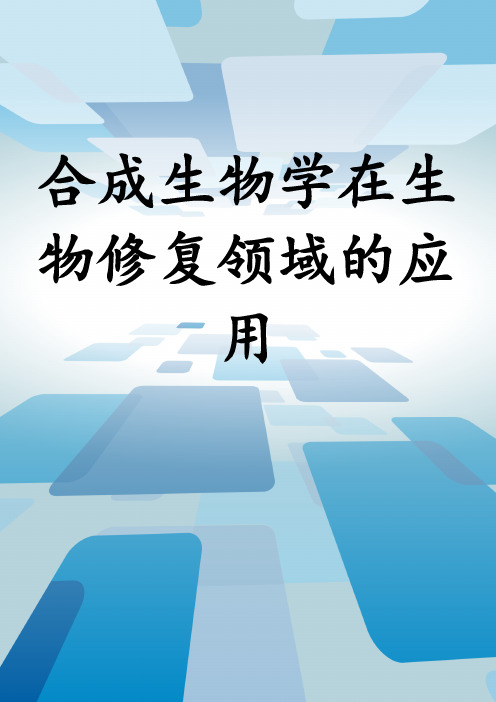
合成生物学在生物修复领域的应用合成生物学作为一门融合了生物学、工程学、信息学及计算机科学的交叉学科,正在逐步改变我们对生命科学的理解和应用方式。
在众多应用领域中,生物修复是其中一个极具前景的方向,它利用合成生物学的工具和策略来解决环境污染、生态恢复等重大问题。
以下是合成生物学在生物修复领域六个方面的具体应用概述:1. 定制微生物的设计与应用在生物修复过程中,通过合成生物学方法可以精准设计微生物,使其具备特定的降解能力。
例如,针对石油泄漏,科学家可以改造细菌,使其能够高效分解石油中的有害化合物,如多环芳烃。
这些定制的微生物不仅降解效率高,而且针对性强,能够在特定环境中快速响应和处理污染物,减少对生态系统的影响。
2. 基因电路的构建与调控合成生物学的核心之一是基因电路的设计,这在生物修复中尤为重要。
通过构建逻辑门控基因回路,可以实现对外界环境信号(如污染物浓度)的感知与响应。
例如,设计一个感应-响应系统,当检测到重金属离子时,启动特定基因表达,产生金属结合蛋白,从而有效捕获和去除水体中的重金属污染。
这种精准的调控机制,提高了生物修复的效率和可控性。
3. 代谢途径的重构与优化利用合成生物学技术,科研人员能够对微生物的代谢途径进行重新设计和优化,以增强其对特定污染物的转化能力。
例如,通过基因工程技术增强细菌对农药残留的降解能力,或者改造微生物以更有效地将塑料微粒转化为无害物质。
这种对代谢网络的精准编辑,使生物修复更加高效和广泛适用。
4. 生物传感器的开发合成生物学也为生物修复提供了先进的监测工具——生物传感器。
这些传感器基于活细胞,能够实时、准确地检测环境中污染物的类型和浓度。
例如,通过编程细菌使其在遇到特定污染物时发出荧光或产生可检测的信号,从而快速定位污染源,指导修复策略的实施。
生物传感器的开发极大提高了环境监测的灵敏度和准确性。
5. 生态系统的功能模拟与重建合成生物学在生态系统层面的应用,旨在通过模仿自然生态系统的功能,设计和构建人工生态模块,以恢复受损的生态环境。
生物传感器的设计与应用

生物传感器的设计与应用生物传感器是一种可以与生物分子互动的生物仿生系统,可以实现对生物分子的检测,具有高灵敏度、高选择性、快速响应、实时监测等优点,被广泛应用于医学诊断、食品安全、环境检测、生物合成等领域。
本文将介绍生物传感器的设计原理、制备方法和应用前景。
一、生物传感器的设计原理生物传感器由识别生物分子的生物元件和信号转换器件组成,其工作原理可以分为识别、转换和信号处理三个步骤。
识别:生物传感器的生物元件通常是一些生物分子,如抗体、酶、核酸等,用于与待检测样品中的目标分子特异性结合,并发生相应的反应。
例如,利用抗体与抗原结合的特异性,可以实现对感染病原体、癌细胞等的检测。
转换:生物传感器的信号转换器件可以将生物元件与目标分子的相互作用转化为可测量的电化学信号、荧光信号、质谱信号等,以便快速、准确地检测目标分子。
例如,利用酶的催化反应,可以将目标分子转化成电化学反应物,然后利用电化学传感器测量其电流、电位等信号。
信号处理:生物传感器的信号处理器件主要用于信号的放大、滤波、解码等,以提高检测灵敏度和选择性,并实现信号的实时监测和定量分析。
例如,利用微流控芯片、计算机辅助设计等,可以实现对多种目标分子的同时检测和高通量分析。
二、生物传感器的制备方法生物传感器的制备方法多种多样,可以根据不同的应用场景和检测要求选择适合的方法。
下面介绍几种常见的制备方法。
1.蛋白质工程技术:蛋白质工程技术是针对蛋白质的结构和性质进行修饰和改造,以获得更好的性能和功能。
可利用手性寡肽、多肽酰胺、蛋白质酶切割等技术将结构优异、活性较高的生物大分子固定到传感器上,以识别特定的分子。
2.基于纳米材料的传感器制备技术:现代材料学与纳米技术的进步,越来越多广泛利用于传感器的制备,并成为了促进全球传感领域快速发展的关键因素之一。
纳米材料具有大比表面积、高催化效率等特点,并可改变物质的光电性能和催化效率,因此可以增强传感器的灵敏度和选择性。
生物传感器的设计与制备

生物传感器的设计与制备生物传感器是一种能够将生物学事件转化为可测量信号的装置,可以应用于医学诊断、环境监测、食品安全等领域。
本文将着重介绍生物传感器的设计与制备过程,并讨论其在生物医学领域的应用。
一、背景介绍生物传感器是一种基于生物与物理化学原理相结合的传感技术,能够检测和测量生物活性物质或生物事件。
生物传感器的核心是生物识别元件,通常是由生物分子如抗体、酶或核酸等构建而成,用于与目标分子的相互作用。
二、生物传感器的设计1. 选择合适的生物材料:生物传感器的生物识别元件应选择具有高灵敏度和特异性的生物材料。
比如,在医学诊断中,选择具有高亲和性的抗体作为生物识别元件。
2. 设计传感器结构:传感器结构的设计需要考虑到生物材料的固定化、信号转导和信号放大等因素。
固定化方法可以采用吸附、共价键合或包埋等方式,以确保生物识别元件的稳定性和活性。
3. 优化传感器性能:为提高传感器的灵敏度和选择性,可以通过优化生物识别元件的程序,如优化抗体的亲和力或改进酶的催化效率。
此外,可以通过改变传感器的物理结构,如增大活性表面积或增加传感器的反应速率等,来提高传感器的性能。
三、生物传感器的制备1. 生物材料的制备:根据设计的要求,选择适当的方法制备生物材料。
比如,可以通过免疫动物获得目标抗体,或通过基因工程方法大量生产需要的酶。
2. 生物材料的修饰:为将生物材料固定在传感器表面,需要对其进行修饰处理。
可利用化学交联剂进行共价键合,或选择高亲和性的吸附剂将生物材料固定在传感器表面。
3. 传感器的组装:将生物材料固定在传感器表面后,需要将其他功能部件组装到传感器中,如信号转导器件、放大器件等。
这些部件的组装可以通过微流体技术、微纳加工技术等实现。
四、生物传感器在生物医学领域的应用1. 医学诊断:生物传感器在医学诊断中起到关键作用,如检测特定蛋白质标记物、细胞表面分子或基因突变等。
这些传感器可以用于早期癌症筛查、疾病诊断和药物疗效监测等。
生物传感器的设计与制备

生物传感器的设计与制备一、引言随着现代技术的发展,越来越多的传感器应用于生物医学等领域,并成为生命科学研究中的重要工具。
其中,生物传感器能够将化学、生物学、物理学等多种技术相结合,实现对生物体的检测和诊断。
本文将介绍生物传感器的设计和制备方法。
二、生物传感器概述生物传感器是一种能够将生物体内分子、细胞等生物体的信息转换为电信号的器具,通常由生物识别层、转换器和输出器组成。
其中生物识别层是生物传感器的核心部分,它可以非常精确地识别、分离特定的生物分子或细胞,并与其进行高效、特异的相互作用。
三、生物识别层的设计生物识别层的设计是生物传感器制备的重要部分。
在此,我们介绍两种常用的生物识别层设计方法:1.抗体/抗原识别:这种方法利用抗体与抗原的特异性结合来实现高效的分离和检测。
通过对抗体的选择和优化,可以实现对目标分子的高度特异性识别。
2.核酸识别:利用核酸互补杂交的原理,对特定的核酸序列进行识别。
与抗体/抗原识别相比,这种识别方法具有更高的灵敏度和特异性。
四、转换器的制备转换器是生物传感器中实现生物信息转换成电信号的部分。
转换器通常是利用物理、化学等方法,将生物信号转化为电信号。
1.生物发光转换器:该转换器利用荧光蛋白、荧光染料、酶反应产生的光信号等,将生物信号转化为光信号,再利用光电二极管等元件将其转化为电信号。
2.生物电化学转换器:该转换器通过氧化还原反应,将生物信号转化为电信号。
通常采用的底物是葡萄糖、乳酸等生物分子。
五、输出器的设计输出器是将转换器输出的电信号转化为人们能够接受的信号形式。
通常采用显示器、计算机等形式进行输出。
1.显示器:生物传感器通常采用液晶显示器、发光二极管等方式进行显示。
2.计算机:借助计算机的数据处理能力,可以实现对生物信息的实时监测和分析。
六、生物传感器的制备流程生物传感器的制备通常分为以下几个步骤:1.生物识别层的设计和合成。
2.生物识别层的表面修饰:通过化学方法将生物识别层连接到表面上,以提高其稳定性和灵敏度。
生物传感器的设计及其应用分析
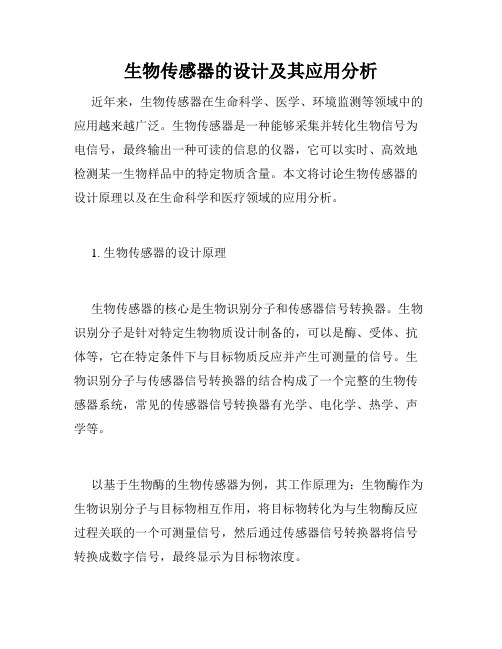
生物传感器的设计及其应用分析近年来,生物传感器在生命科学、医学、环境监测等领域中的应用越来越广泛。
生物传感器是一种能够采集并转化生物信号为电信号,最终输出一种可读的信息的仪器,它可以实时、高效地检测某一生物样品中的特定物质含量。
本文将讨论生物传感器的设计原理以及在生命科学和医疗领域的应用分析。
1. 生物传感器的设计原理生物传感器的核心是生物识别分子和传感器信号转换器。
生物识别分子是针对特定生物物质设计制备的,可以是酶、受体、抗体等,它在特定条件下与目标物质反应并产生可测量的信号。
生物识别分子与传感器信号转换器的结合构成了一个完整的生物传感器系统,常见的传感器信号转换器有光学、电化学、热学、声学等。
以基于生物酶的生物传感器为例,其工作原理为:生物酶作为生物识别分子与目标物相互作用,将目标物转化为与生物酶反应过程关联的一个可测量信号,然后通过传感器信号转换器将信号转换成数字信号,最终显示为目标物浓度。
2. 生物传感器在生命科学领域的应用生物传感器用于生命科学中的应用领域非常广泛,包括基因检测、蛋白质检测、细胞毒性检测、细胞激活状态检测等。
(1)基因检测:基因传感器可以检测人类基因中的单核苷酸多态性(SNP),实现基因疾病的诊断和基因多态性的分析。
(2)蛋白质检测:蛋白质是细胞内最重要的分子之一,是细胞的核心结构。
生物传感器可以对蛋白质的含量、结构、功能等方面进行检测,对诊断和治疗的帮助非常大。
(3)细胞毒性检测:通过测定生物样品中的细胞活性、凋亡等参数,可以分析细胞的毒性。
(4)细胞激活状态检测:生物传感器可以测定细胞在特定条件下的基因表达和蛋白质合成水平,对细胞活性、增殖、分泌等进行分析。
3. 生物传感器在医疗领域的应用随着人类生存环境的改变,新的疾病在不断涌现,传统的疾病治疗手段已经不足以满足医疗需求,而生物传感器正是解决这一问题的有效手段,它可以实现快速、准确的疾病检测和诊断,具有广泛的应用前景。
生物传感器的设计与制备
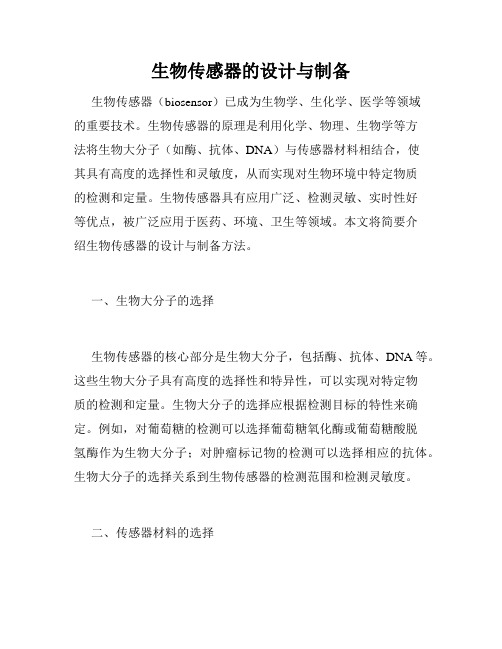
生物传感器的设计与制备生物传感器(biosensor)已成为生物学、生化学、医学等领域的重要技术。
生物传感器的原理是利用化学、物理、生物学等方法将生物大分子(如酶、抗体、DNA)与传感器材料相结合,使其具有高度的选择性和灵敏度,从而实现对生物环境中特定物质的检测和定量。
生物传感器具有应用广泛、检测灵敏、实时性好等优点,被广泛应用于医药、环境、卫生等领域。
本文将简要介绍生物传感器的设计与制备方法。
一、生物大分子的选择生物传感器的核心部分是生物大分子,包括酶、抗体、DNA等。
这些生物大分子具有高度的选择性和特异性,可以实现对特定物质的检测和定量。
生物大分子的选择应根据检测目标的特性来确定。
例如,对葡萄糖的检测可以选择葡萄糖氧化酶或葡萄糖酸脱氢酶作为生物大分子;对肿瘤标记物的检测可以选择相应的抗体。
生物大分子的选择关系到生物传感器的检测范围和检测灵敏度。
二、传感器材料的选择传感器材料是建立生物传感器的物质基础,应具有高度的灵敏度和选择性。
常用的传感器材料有电化学材料、光学材料、催化剂、纳米材料等。
例如,利用电化学材料制备的生物传感器可以实现快速、灵敏的电信号检测;光学材料可以实现对光信号的检测;利用催化剂可以加速反应速度,提高检测的灵敏度。
传感器材料的选择应考虑生物大分子的特性和反应机理。
三、生物大分子的修饰生物大分子与传感器材料的结合通常需要通过化学修饰的方法进行。
生物大分子的修饰可通过交联、化学修饰、共价键合等方式实现。
例如,将葡萄糖氧化酶修饰到电极表面,可采用交联法和共价键合法。
交联法通常利用聚乙二醇、凝胶等进行修饰,其优点是具有较强的稳定性和免疫原性;共价键合法则利用特定的交联剂,可实现较紧密的结合。
生物大分子的修饰应考虑到修饰方法对其活性的影响以及与传感器材料间的相容性。
四、传感器的制备传感器的制备是生物传感器的关键步骤,应考虑到传感器材料的特性和生物大分子的修饰情况。
传感器的制备通常涉及物理、化学、电化学等多个领域。
生物传感器的设计与制备
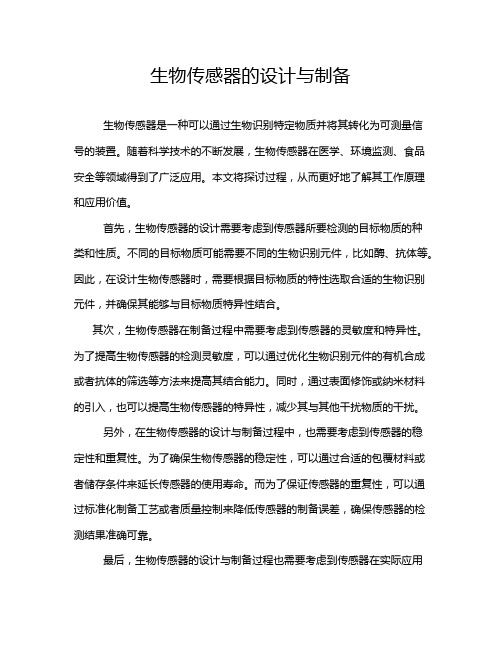
生物传感器的设计与制备生物传感器是一种可以通过生物识别特定物质并将其转化为可测量信号的装置。
随着科学技术的不断发展,生物传感器在医学、环境监测、食品安全等领域得到了广泛应用。
本文将探讨过程,从而更好地了解其工作原理和应用价值。
首先,生物传感器的设计需要考虑到传感器所要检测的目标物质的种类和性质。
不同的目标物质可能需要不同的生物识别元件,比如酶、抗体等。
因此,在设计生物传感器时,需要根据目标物质的特性选取合适的生物识别元件,并确保其能够与目标物质特异性结合。
其次,生物传感器在制备过程中需要考虑到传感器的灵敏度和特异性。
为了提高生物传感器的检测灵敏度,可以通过优化生物识别元件的有机合成或者抗体的筛选等方法来提高其结合能力。
同时,通过表面修饰或纳米材料的引入,也可以提高生物传感器的特异性,减少其与其他干扰物质的干扰。
另外,在生物传感器的设计与制备过程中,也需要考虑到传感器的稳定性和重复性。
为了确保生物传感器的稳定性,可以通过合适的包覆材料或者储存条件来延长传感器的使用寿命。
而为了保证传感器的重复性,可以通过标准化制备工艺或者质量控制来降低传感器的制备误差,确保传感器的检测结果准确可靠。
最后,生物传感器的设计与制备过程也需要考虑到传感器在实际应用中的可操作性和易用性。
为了提高生物传感器的可操作性,可以通过简化传感器的操作步骤或者设计便携式的传感器设备来降低传感器的使用门槛。
同时,也可以通过提供数据分析软件或者智能手机App等工具来简化传感器的数据处理过程,使用户能够更方便地获取传感器的检测结果。
梳理一下本文的重点,我们可以发现,生物传感器的设计与制备是一个复杂而又具有挑战性的过程。
只有通过深入研究和不断实践,我们才能不断提升生物传感器的性能和应用范围,真正实现生物传感器在健康监测、环境保护、食品安全等领域的广泛应用。
希望本文的内容能够为生物传感器的研究与应用提供一定的参考和借鉴,推动生物传感器技术的进一步发展和创新。
生物传感器的设计与应用
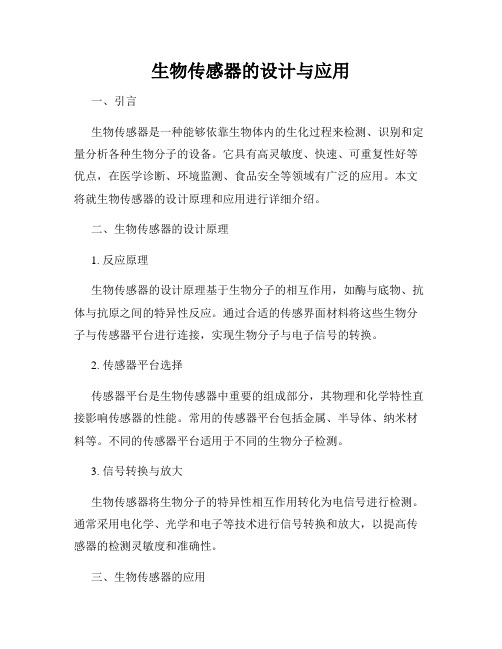
生物传感器的设计与应用一、引言生物传感器是一种能够依靠生物体内的生化过程来检测、识别和定量分析各种生物分子的设备。
它具有高灵敏度、快速、可重复性好等优点,在医学诊断、环境监测、食品安全等领域有广泛的应用。
本文将就生物传感器的设计原理和应用进行详细介绍。
二、生物传感器的设计原理1. 反应原理生物传感器的设计原理基于生物分子的相互作用,如酶与底物、抗体与抗原之间的特异性反应。
通过合适的传感界面材料将这些生物分子与传感器平台进行连接,实现生物分子与电子信号的转换。
2. 传感器平台选择传感器平台是生物传感器中重要的组成部分,其物理和化学特性直接影响传感器的性能。
常用的传感器平台包括金属、半导体、纳米材料等。
不同的传感器平台适用于不同的生物分子检测。
3. 信号转换与放大生物传感器将生物分子的特异性相互作用转化为电信号进行检测。
通常采用电化学、光学和电子等技术进行信号转换和放大,以提高传感器的检测灵敏度和准确性。
三、生物传感器的应用1. 医学诊断生物传感器在医学诊断中发挥着重要作用,尤其是早期疾病的检测。
例如,通过检测血液中的肿瘤标志物可以实现癌症的早期诊断。
生物传感器可以准确、快速地测定肿瘤标志物的浓度,为医生提供更准确的诊断依据。
2. 环境监测生物传感器在环境监测方面具有广泛应用。
例如,通过检测水中的重金属离子浓度可以评估水质的安全性。
生物传感器可以准确地测定水中重金属离子的浓度,及时预警水质超标问题,保障人民的饮用水安全。
3. 食品安全生物传感器在食品安全领域的应用也越来越重要。
例如,通过检测食品中的致病菌或农药残留可以评估食品的安全性。
生物传感器可以快速、准确地测定致病菌或农药残留的含量,确保食品质量安全。
4. 生物制药生物传感器在生物制药上的应用可以提高生产效率和产品质量。
例如,通过检测发酵液中的代谢产物可以调控发酵过程,提高生物制药的产量和质量。
生物传感器可以实时、快速地测定发酵液中的代谢产物,为生产厂家提供优化生产工艺的依据。
生物传感器的设计与性能研究
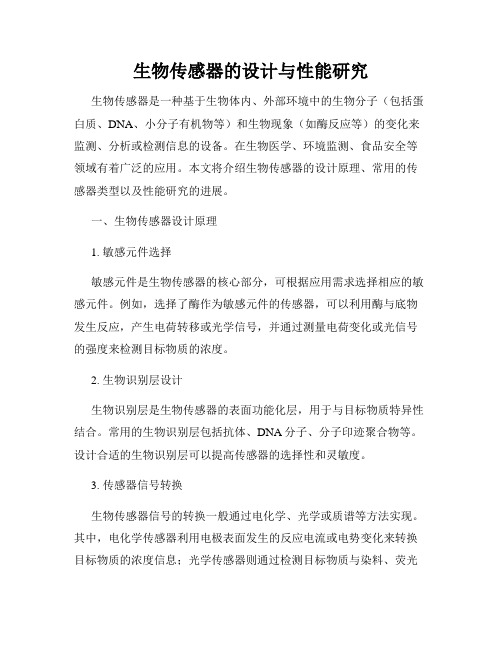
生物传感器的设计与性能研究生物传感器是一种基于生物体内、外部环境中的生物分子(包括蛋白质、DNA、小分子有机物等)和生物现象(如酶反应等)的变化来监测、分析或检测信息的设备。
在生物医学、环境监测、食品安全等领域有着广泛的应用。
本文将介绍生物传感器的设计原理、常用的传感器类型以及性能研究的进展。
一、生物传感器设计原理1. 敏感元件选择敏感元件是生物传感器的核心部分,可根据应用需求选择相应的敏感元件。
例如,选择了酶作为敏感元件的传感器,可以利用酶与底物发生反应,产生电荷转移或光学信号,并通过测量电荷变化或光信号的强度来检测目标物质的浓度。
2. 生物识别层设计生物识别层是生物传感器的表面功能化层,用于与目标物质特异性结合。
常用的生物识别层包括抗体、DNA分子、分子印迹聚合物等。
设计合适的生物识别层可以提高传感器的选择性和灵敏度。
3. 传感器信号转换生物传感器信号的转换一般通过电化学、光学或质谱等方法实现。
其中,电化学传感器利用电极表面发生的反应电流或电势变化来转换目标物质的浓度信息;光学传感器则通过检测目标物质与染料、荧光探针等发生的光学信号变化来转换浓度信息;质谱传感器则通过记录目标物质的质谱图谱来转换浓度信息。
二、常用的生物传感器类型1. 酶传感器酶传感器利用酶与底物的专一性催化作用,通过测量催化反应的速率、反应产物的电流或光信号强度等参数来确定目标物质浓度。
常见的酶传感器有葡萄糖传感器、乳酸传感器等。
2. DNA传感器DNA传感器利用DNA分子的特异性识别和杂交,通过测量目标DNA与DNA探针杂交反应引起的电化学、光学或质谱信号变化来检测目标DNA的序列、浓度或突变。
3. 免疫传感器免疫传感器利用抗体与抗原的特异性结合,通过测量抗原-抗体反应引起的电化学、光学或质谱信号变化来检测目标物质的存在和浓度。
三、性能研究的进展1. 提高选择性和灵敏度目前,研究者们致力于开发新型的生物识别层和信号转换方法,以提高传感器的选择性和灵敏度。
生物传感器的设计原理与应用领域
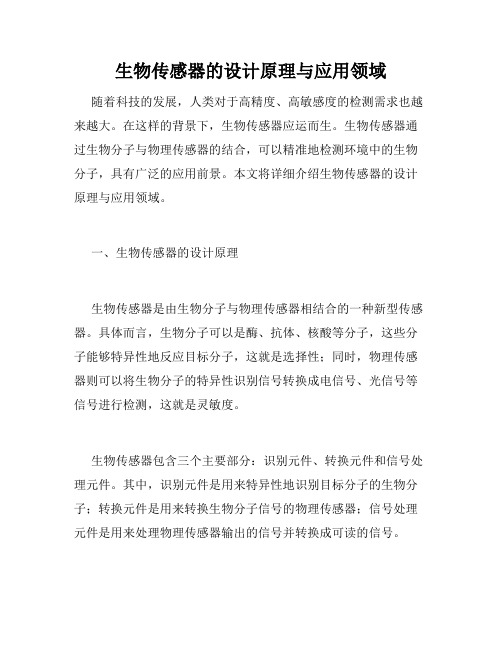
生物传感器的设计原理与应用领域随着科技的发展,人类对于高精度、高敏感度的检测需求也越来越大。
在这样的背景下,生物传感器应运而生。
生物传感器通过生物分子与物理传感器的结合,可以精准地检测环境中的生物分子,具有广泛的应用前景。
本文将详细介绍生物传感器的设计原理与应用领域。
一、生物传感器的设计原理生物传感器是由生物分子与物理传感器相结合的一种新型传感器。
具体而言,生物分子可以是酶、抗体、核酸等分子,这些分子能够特异性地反应目标分子,这就是选择性;同时,物理传感器则可以将生物分子的特异性识别信号转换成电信号、光信号等信号进行检测,这就是灵敏度。
生物传感器包含三个主要部分:识别元件、转换元件和信号处理元件。
其中,识别元件是用来特异性地识别目标分子的生物分子;转换元件是用来转换生物分子信号的物理传感器;信号处理元件是用来处理物理传感器输出的信号并转换成可读的信号。
二、生物传感器的应用领域生物传感器具有广泛的应用领域,下面将几个常见的应用场景进行介绍。
1、医学领域生物传感器可以用于医学领域的诊断、药物研发等方面。
例如,利用生物传感器可以检测病毒、细菌和肿瘤标志物等,从而实现疾病的快速检测和诊断。
此外,生物传感器还可以用于药物筛选、体内药物作用监测等方面。
2、环境监测生物传感器可以用于环境监测领域。
例如,利用生物传感器可以监测空气中的有害气体、水体中的有毒污染物等。
这种方法不仅准确度高,而且还可以监测场地更大范围内的污染而不仅仅是点测。
3、农业领域生物传感器可以用于农业领域。
例如,利用生物传感器可以检测植物病害、肥料中的营养成分等。
这种方法可以提高农业生产效率和降低损失。
4、食品安全生物传感器可以用于食品安全领域。
例如,利用生物传感器可以检测食品中的添加物、致病菌等,从而保证食品安全。
此外,生物传感器还可以监测食品在加工过程中的变化等。
三、生物传感器的未来前景生物传感器在未来的发展中,还面临着许多挑战和机遇。
其中一个挑战是如何提高生物传感器的灵敏度和选择性,这也是生物传感器技术发展的最大瓶颈。
生物传感器的设计与研究
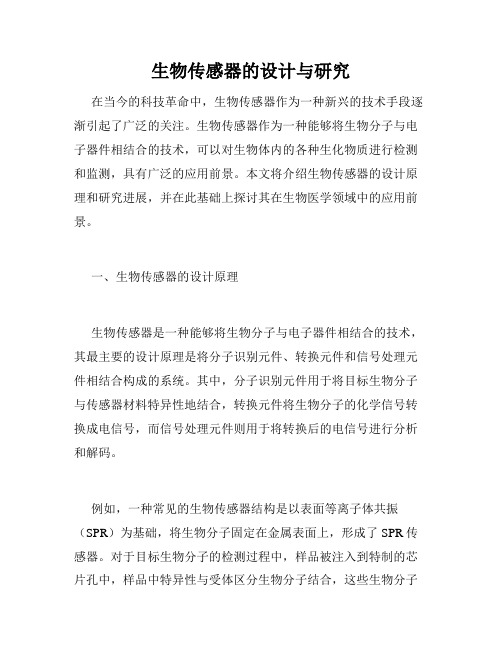
生物传感器的设计与研究在当今的科技革命中,生物传感器作为一种新兴的技术手段逐渐引起了广泛的关注。
生物传感器作为一种能够将生物分子与电子器件相结合的技术,可以对生物体内的各种生化物质进行检测和监测,具有广泛的应用前景。
本文将介绍生物传感器的设计原理和研究进展,并在此基础上探讨其在生物医学领域中的应用前景。
一、生物传感器的设计原理生物传感器是一种能够将生物分子与电子器件相结合的技术,其最主要的设计原理是将分子识别元件、转换元件和信号处理元件相结合构成的系统。
其中,分子识别元件用于将目标生物分子与传感器材料特异性地结合,转换元件将生物分子的化学信号转换成电信号,而信号处理元件则用于将转换后的电信号进行分析和解码。
例如,一种常见的生物传感器结构是以表面等离子体共振(SPR)为基础,将生物分子固定在金属表面上,形成了SPR传感器。
对于目标生物分子的检测过程中,样品被注入到特制的芯片孔中,样品中特异性与受体区分生物分子结合,这些生物分子与受体将生物分子结合在生物膜上,然后将此芯片置于SPR测量系统中。
利用SPR技术,测量系统能够实时监测绑定事件,并得到相应信号,这一信号反映了样品中生物分子相对于生物膜的吸附或解离动力学变化。
通过计算分析,可以得到目标生物分子的数量和质量浓度。
二、生物传感器研究进展随着科学技术的不断发展,生物传感器作为一种新兴的技术手段,近年来已经取得了很多重要的进展。
目前,生物传感器主要应用在生命科学、医疗诊断等领域。
随着相关研究的深入,可穿戴式个人监测生物传感器和非侵入性型传感器等新型传感器越来越受到研究人员的青睐。
例如,在生命科学中,生物传感器可用于检测生物体中的激素、肿瘤标志物、病毒和DNA等分子,这些检测的结果可以为相关疾病的诊断和治疗提供帮助。
同时,现代医药领域中的个性化医学和精准药物研究也与生物传感器联系紧密。
通过利用生物传感器技术实现生物分子的精确检测和监测,可为疾病预防和治疗带来新的突破。
- 1、下载文档前请自行甄别文档内容的完整性,平台不提供额外的编辑、内容补充、找答案等附加服务。
- 2、"仅部分预览"的文档,不可在线预览部分如存在完整性等问题,可反馈申请退款(可完整预览的文档不适用该条件!)。
- 3、如文档侵犯您的权益,请联系客服反馈,我们会尽快为您处理(人工客服工作时间:9:00-18:30)。
A modular cell-based biosensor using engineeredgenetic logic circuits to detect and integrate multiple environmental signalsBaojun Wang a ,b ,c ,n ,Mauricio Barahona a ,1,Martin Buck b ,2aDepartment of Mathematics,6M50Huxley Building,Imperial College London,London,SW72AZ,UK bDepartment of Life Sciences,Imperial College London,UK cCentre for Synthetic Biology and Innovation,Imperial College London,UKa r t i c l e i n f oAvailable online 23August 2012Keywords:Cellular biosensor Genetic circuits Logic gatesCell programming Synthetic biologya b s t r a c tCells perceive a wide variety of cellular and environmental signals,which are often processed combinatorially to generate particular phenotypic responses.Here,we employ both single and mixed cell type populations,pre-programmed with engineered modular cell signalling and sensing circuits,as processing units to detect and integrate multiple environmental signals.Based on an engineered modular genetic AND logic gate,we report the construction of a set of scalable synthetic microbe-based biosensors comprising exchangeable sensory,signal processing and actuation modules.These cellular biosensors were engineered using distinct signalling sensory modules to precisely identify various chemical signals,and combinations thereof,with a quantitative fluorescent output.The genetic logic gate used can function as a biological filter and an amplifier to enhance the sensing selectivity and sensitivity of cell-based biosensors.In particular,an Escherichia coli consortium-based biosensor has been constructed that can detect and integrate three environmental signals (arsenic,mercury and copper ion levels)via either its native two-component signal transduction pathways or synthetic signalling sensors derived from other bacteria in combination with a cell-cell communication module.We demonstrate how a modular cell-based biosensor can be engineered predictably using exchange-able synthetic gene circuit modules to sense and integrate multiple-input signals.This study illustrates some of the key practical design principles required for the future application of these biosensors in broad environmental and healthcare areas.&2012Elsevier B.V.All rights reserved.1.IntroductionBacterial cells live in an ever changing environment and must therefore be equipped with specific genetically-encoded sensors and signalling networks to continuously perceive and react to the various environmental signals.Analogous to a typical electrome-chanical sensor,a cellular signalling network normally consists of three interconnected modules –the input sensors,internal processing and regulatory circuits and output actuators to allow signal sensing and timely adaptations in cell physiology (Lim,2010;Wang and Buck,2012)(Fig.1).The input sensors are receptors,either embedded in the cell membrane (e.g.sensor kinases)or located freely in the cytoplasm (e.g.ligand responsive allosteric proteins)with which the cell can detect various extra-or intra-cellular signals,such as chemical molecules,metal ions,light,heat or antigens,and transduce them into differentialgene transcriptional levels.Downstream gene regulatory net-works process and integrate such signals combinatorially for a logic decision to be made,mimicking the digital logic circuit in electronic circuitry.Decisions are signified by changes in the expression of output actuators:the relevant proteins and chemi-cals responsible for the final phenotypic changes in motility,growth and morphology etc.Due to the inherent modular architecture of sensory systems,bacterial cells can be viewed as programmable living biosensors in which the three component modules are exchangeable (Fig.1).For example,specific synthetic sensors can be developed from either the host’s own genetic repertoire or that of other bacterial species with more relevant specialisations in sensing capabilities in order to detect for example particular environmental contami-nants or disease-related rmed by the advanced sen-sing capabilities of many environmental microbes,already a number of single-input bacterial biosensors (van der Meer and Belkin,2010)have been constructed to detect various toxic pollutant such as arsenic (Joshi et al.,2009;Stocker et al.,2003;Trang et al.,2005),xylene and toluene (Paitan et al.,2004),DNT explosive (De Las Heras et al.,2008)and the human pathogen Pseudomonas aeruginosa (Saeidi et al.,2011)with fluorescence,Contents lists available at SciVerse ScienceDirectjournal homepage:/locate/biosBiosensors and Bioelectronics0956-5663/$-see front matter &2012Elsevier B.V.All rights reserved./10.1016/j.bios.2012.08.011nCorresponding author.Tel.:þ447951759259;fax:þ442075948517.E-mail addresses:b.wang06@ (B.Wang),m.barahona@ (M.Barahona),m.buck@ (M.Buck).1Tel.:þ442075948528.2Tel.:þ442075945442.Biosensors and Bioelectronics 40(2013)368–376luminescence or colorimetric pigments as outputs.However,these biosensors have been typically developed utilizing only the cell native sensory elements without additional layers of genetic processing and regulation,and thuscan only detect one type of signal rather than a number of signals in a predefined combination.Instead,synthetic genetic logic circuits can be introduced through linking various cellular sensors and genetic actuators,to achieve customized cellular responses (Kobayashi et al.,2004)and sensing abilities (Khalil and Collins,2010)in a designed and logical manner.In particular,multi-input cellular biosensors are essential for recognizing complex conditions,such as the cancer microenvironment normally specified by several signals in combination,where an enhanced sensing specificity and accuracy of the output response are necessary.For example,several genetic logic AND gates have been constructed to link inputs to pathogenicity-related signal responsive promoters (Nissim and Bar-Ziv,2010),microRNAs (Xie et al.,2011)or proteins (Culler et al.,2010),and the output to a therapeutic suicide gene in order to achieve highly specific in vivo sensing and killing of diseased mammalian cells.These proof-of-concept examples demonstrate the great potential for using biological logic circuits to customize cell sensing and signalling for many useful applications (Khalil and Collins,2010;Wang and Buck,2012;Weber and Fussenegger,2012).Here,we employ both single cell type and multiple cell type populations,pre-programmed with engineered modular cell sig-nalling and sensing circuits,as processing units to sense and integrate multiple environmental contamination associated sig-nals.A modular,tightly-controlled and hypersensitive genetic AND gate was recently constructed in Escherichia coli that can integrate two genetic transcriptional inputs simultaneously (Wang et al.,2011).In the present work,we have engineered several E.coli -based logic-gated cellular biosensors by connecting the AND gate two inputs to a set of synthetic sensors for detecting and integrating the levels of arsenic,mercury,copper,zinc ions and bacterial quorum sensing molecules in an aqueous environment leading to a quantitative fluorescent output.The designed genetic logic circuits can act as a biological filter and an amplifier to enhance the sensing selectivity and sensitivity of whole cell-based biosensors.Moreover,a triple-input AND logic gated biosensor comprising two cell consortia was also constructed which can sense and integrate three environmental signals (As 3þ,Hg 2þand Cu 2þlevels)via a synthetic cell–cell communication module driving the cooperation between the two cell populations.2.Materials and methods2.1.Bacterial strains and growth conditionsPlasmid cloning work and characterisation of the circuit constructs were all performed in E.coli TOP10strain.Cells were cultured in LB (Luria-Bertani Broth)media (10g/L peptone,5g/L NaCl,5g/L yeast extract).The antibiotic concentrations used were 50m g/ml for kanamycin and 50m g/ml for ampicillin.Cells inocu-lated from single colonies on freshly streaked plates were grown overnight in 5ml LB in sterile 20ml universal tubes at 371C with shaking (200rpm).Overnight cultures were diluted into pre-warmed LB media at OD 600¼0.025for the day cultures,which were induced and grown for 6h at 371C prior to analysis,unless otherwise indicated.For the fluorescence assay by fluorometry,diluted cultures were loaded into a 96well microplate (Greiner Bio-One,chimney black,flat clear bottom)by a repetitive pipette and induced with 5m l (for single input induction)or 10m l (for double input induction)or 15m l (for triple input induction)inducers of varying concentrations to a final volume of 200m l per well by a multichannel pipette.The microplate was covered with a transparent lid to counteract evaporation and incubated in the fluorometer (BMG FLUOstar)with continuous shaking (200rpm,linear mode)between each cycle of repetitive measure-ments.All the chemical reagents used were analytical grade and purchased from Sigma Aldrich.2.2.Plasmid circuit constructionPlasmid construction and DNA manipulations were performed following standard molecular biology techniques.The arsenic (J23101-rbs32-arsR -B0015-P arsR )and mercury (J23115-rbs32-merR -B0015-P merT )sensor fragments,hrpR-B0015and hrpS-B0015fragments and hrpL promoter were synthesised by GEN-EART and have been designed following the BioBrick standard ( ),i.e.,eliminating the four restriction sites (EcoRI,XbaI,SpeI and PstI)exclusive for this standard by synonymous codon exchange and flanking with prefix and suffix sequences containing the appropriate restriction sites and RBS.The constitutive promoters J23101and J23115( )were used to drive gene expression continuously as indicated.The double terminator B0015was used to terminate gene transcription in all cases.The copper responsive P cusC ,zinc sensitive P zraP and cadmium responsive P zntA promotersmetal ions chemicals light temperature antigensInputs P 1P 2Fig.1.Architecture of a synthetic modular the input sensors,the internal genetic information processing as sensor kinases or intracellular receptor proteins to detect multiple input signals.The programmed cells can then initiate customized responses by activating different output genes according to the logic decision transmitted upstream.Adapted with permission from Wang and Buck (2012).B.Wang et al./Biosensors and Bioelectronics 40(2013)368–376369were amplified by colony PCR from the E.coli MG1655genome with EcoRI/SpeI sites.The 3OC 6HSL sensor (P tet -rbs34-luxR -B0015-P luxI ,BBa_F2620(Canton et al.,2008)),gfp (green fluor-escent protein,BBa_E0840)and rfp (red fluorescent protein,BBa_I13507)reporters and luxI gene (BBa_K081008)were obtained from the Registry of Standard Biological Parts ( ).Plasmid pSB3K3(Shetty et al.,2008)(p15A ori,Kan r )was used to carry the six single-input biosensors.The six chemical sensor fragments (cut by EcoRI/SpeI)and the gfp or P.syringae hrpR /hrpS expression constructs (cut by XbaI/PstI)were ligated into pSB3K3(cut by EcoRI/PstI)following a three way ligation protocol in one pool.Plasmid pSB3K3was also used to harbour the two-input AND gated biosensor constructs con-structed following a similar three way ligation method (Fig.S1).The hrpL promoter from Pseudomonas syringae followed by a gfp ,rfp or a luxI gene construct was carried on pSB4A3(Shetty et al.,2008)(pSC101ori,Amp r )using the same cloning strategy.The various RBS sequences (Table 1)for each gene construct were introduced with PCR primers if necessary (amplification utilized high-fidelity Phusion DNA polymerase from NEB and an Eppen-dorf Mastercycler gradient thermal cycler).All constructs were verified by DNA sequencing (Beckman Coulter Genomics at UK)prior to their use in the target cell strains.Primers were synthe-sised by Sigma Life Science.More information can be found in Fig.S1Supporting methods,with plasmid maps describing some representative circuit constructs used.2.3.Gene expression assayFluorescence outputs representing gene expression levels were measured by fluorometry at the whole cell population level.Cells grown in 96-well plates were monitored and assayed using a BMG FLUOstar fluorometer for repeated absorbance (OD 600)and green fluorescence (485nm for excitation,520710nm for emission,Gain ¼1200,bottom reading)or red fluorescence (584nm for excitation,620710nm for emission,Gain ¼2000,bottom reading)readings (20min/cycle).For fluorescent assay of the three-input biosensor comprising two cell consortia,the two cell populations were diluted from overnight culture to the same cell density at OD 600¼0.025and mixed using the same volume of each cell culture prior to being loaded into microtitre plate wells.The mixed cell culture was then subjected to chemical exposure and incubation in the fluorometer.The normalised total fluores-cence 10h after induction was used for the three-input biosensor assay.This longer time growth allows for sufficient quorum sensing molecules synthesised by the first cell consortium to accumulate for activation of the second cell consortium under the specified conditions (see Fig.4legends).2.4.Mathematical model and data analysisThe transfer functions for the single-input and double-input AND gated sensors were derived from a steady state assumption(Wang et al.,2011).The resulting data (Fig.2A–F)were fitted to a Hill function model for the environment responsive promoter steady state input-output response (transfer function)of the form (Alon,2007;Wang et al.,2011;Zoltan et al.,2006):f ð½I Þ¼k ða þ½I n =ðK n M þ½I nÞÞð1Þwhere [I ]is the concentration of the inducer,K M and n are the Hill constant and coefficient,respectively,relating to the promoter–regulator/inducer interaction,k is the maximum expression level due to induction,and a is a constant relating to the basal level of the promoter due to leaky expression (Table 2).A detailed derivation of the transfer functions is provided in Supplementary methods .The two dimensional data of the AND gated sensors (Fig.3A and B)were used to parameterise the normalised transfer function model of the AND gate of the form:f ð½R ,½S Þ¼½G =½G max ¼ð½R =K R Þn R ð½S =K S Þn S =ðð1þð½R =K R Þn R Þð1þð½S =K S Þn S ÞÞð2ÞThe transfer function describes the normalised output of the AND gate as a function of the levels of the two activator proteins (½R for HrpR,½S for HrpS)at steady state.½G max is the maximum activity observed for the output.K R ,K S and n R ,n S are the Hill constants and coefficients for HrpR and HrpS,respectively (Sup-plementary methods,Fig.S3).The levels of the two activators were derived from the parameterised transfer functions of the two input promoters (Table 2)with the same RBSs as used in the AND gate construction.The nonlinear least square fitting function (cftool and sftool)in Matlab (MathWorks R2010a)was applied to fit the experimental data to parameterise the transfer function model.The fluorometry data of gene expression were first processed in BMG Omega Data Analysis Software (v2.10)and then exported into Microsoft Excel 2010and Matlab for analysis.The medium back-grounds of absorbance and fluorescence were determined from blank wells loaded with LB media and were subtracted from the readings of sample wells.The sample fluorescence/OD 600ratio (Fluo/OD 600)at a specific time was determined by subtracting the triplicate-averaged ratio corresponding to the negative control cultures (GFP-free)at the same time point.For the population-averaged assay by fluorometry,the fluorescence/OD 6006h growth post initial dilution and induction was treated as the output response of cells in the steady state during exponential growth.3.Results and discussion3.1.Design and construction of a set of single input cellular sensorsThrough examination of the reported native environmental sensing abilities of different bacterial organisms,we first designed a set of whole cell based biosensors to detect contamination related molecules and heavy metal ions (arsenic,mercury,copper,zinc,cadmium and bacterial quorum sensing (QS)molecules)in the aqueous environment (Fig.2).These biosensors were designed either by harnessing the natural signalling mechanisms of the host cells,such as the E.coli genetic resistant modules against high concentrations of arsenite (Diorio et al.,1995),copper (Munson et al.,2000),zinc (Leonhartsberger et al.,2001)and cadmium ions (Binet and Poole,2000),or by utilising synthetic sensory pathways from other bacteria such as the mercury sensitive MerR protein and its cognate regulated promoter P merT from Shigella flexneri R100plasmid (Misra et al.,1985),in addition to the QS signalling molecule 3OC 6HSL sensitive LuxR and its target P luxI promoter from Vibrio fischeri (Stevens and Greenberg,1997).A GFP (green fluor-escent protein)mutant with fast maturation time was used as the output reporter to indicate the levels of these chemicals.Table 1Ribosome binding sites used for characterising sensor dose responses.Identifier a Sequence of RBS bObserved strength rbs30TCTAGAGATTAAAGAGGAGAAATACTAG ATG very strong rbs31TCTAGAGTCACACAGGAAACCTACTAG ATG strong rbs32TCTAGAGTCACACAGGAAAGTACTAG ATG weakrbs33TCTAGAGTCACACAGGACTACTAG ATGvery weakaThe RBS sequences are from the Registry of Standard Biological Parts ()and are reported to have distinct strengths (Wang et al.,2011).bSequences of RBS are underlined and start codons are in bold.B.Wang et al./Biosensors and Bioelectronics 40(2013)368–376370Fig.2A shows the architecture of the genetically encoded arsenic sensor.The synthetic sensor circuit comprises the arsenite/arsenate sensitive ArsR repressor continuously expressed from a constitutive promoter J23101and its cognate P arsR pro-moter fused to a gfp reporter.When arsenic is absent,the P arsR promoter is bound and repressed by ArsR resulting in low fluorescent output.The circuit produces high fluorescence when arsenic exists and binds with ArsR to relieve its repression on the target promoter.The spatial separation of the repressor and its cognate promoter in this design is different from that intheirFig.2.The design and characterization of a set of single-input cellular biosensors.(A)The arsenic sensor were characterised under various arsenite concentrations (0,0.125,0.25,0.5,1.0,1.2,2.0,4.0,8.0,16.0,32m M NaAsO 2).(B)The copper ion cellular sensor was characterised at various CuSO 4levels (0,0.00137,0.0041,0.0123,0.037,0.11,0.33,0.5,1.0,1.5,2.0mM).(C)The mercury sensor was characterised under various HgCl 2levels (0,0.0625,0.125,0.25,0.5,1.0,2.0,4.0,5.0,6.0m M).(D)The quorum sensing signalling molecule sensor was characterised under various AHL levels (0,0.098,0.39,1.56,6.25,25.0,50,100,400,1600,6400nM).(E)The zinc or lead sensor was characterised under various ZnCl 2/PbCl 2levels (0,0.0041,0.0123,0.037,0.111,0.222,0.333,0.5,0.75,1,1.2mM).(F)The cadmium or zinc sensor was characterised under various levels of CdCl 2(0,0.00137,0.0041,0.0123,0.037,0.111,0.222,0.333,0.4mM)or ZnCl 2as the same as indicated in (E).Error bars,s.d.(n ¼4).a.u.,arbitrary units.B.Wang et al./Biosensors and Bioelectronics 40(2013)368–376371native context in order to reduce the sensor background basal expression level.The dose response of this arsenic sensors (Fig.2A)shows that the sensor output is proportional to the arsenic levels in the media with detection limit as low as 0.1m M.The characterization data were fitted to the derived transfer function model of the sensor (see Section 2)and the fitted model parameters are shown in Table 2.Fig.2B shows the design and characterisation of the copper responsive sensor.The sensor circuit comprises simply the P cusC promoter fused to the gfp reporter by utilizing the E.coli native CusSR two component signal transduction pathway (Munson et al.,2000).The histidine sensor kinase CusS is embedded in the cell membrane to sense extracellular copper ions and can phosphorylate its response regulator CusR under high Cu 2þconditions.The phosphorylated CusR can then activate the target P cusC promoter to turn on the GFP production.Therefore the output fluorescence level is in proportion to the copper ion level in the environment.The dose response curve of the sensor (Fig.2B)shows that the sensor can detect Cu 2þbetween micro-and milli-molar range with a detection limit as low as 12m M.The characterised dose responses were next fitted to the transfer function model (Eq.(1),parameters shown in Table 2).Fig.2C and D shows the design and characterisation of the mercury and QS molecule 3OC 6HSL sensors respectively.These two sensors were designed using the orthologous sensory pro-teins MerR and LuxR and their cognate target promoters P merT and P luxI from other bacteria.The two sensor circuits have similar genetic architectures:the receptor proteins are continuously expressed from a constitutive promoter (J23115or P tet )with their cognate regulated promoters fused to the gfp reporter.The two sensory proteins can bind specifically to their cognate mercury and 3OC 6HSL molecules and the bound complexes then activate their target promoters to turn on the GFP reporter production.The characterised dose response curves of the two sensors (Fig.2C and D)show that they have similar digital-like responses with a steep transition from the OFF state to the ON state.The fitted Hill function models (Table 2)also indicate that the two sensors have high cooperative Hill coefficients (4.84for the mercury sensor and 6.64for the 3OC 6HSL sensor)but with distinctly different Hill constants (2.3m M for mercury and 31.6nM for 3OC 6HSL),which might be due to the different binding affinities between the two receptor proteins and their cognate signalling molecules.Fig.2E and F shows the design and characterisation of the zinc and cadmium responsive sensors.The zinc sensor comprises of the P zraP promoter fused to the gfp reporter by utilizing the E.coli native ZraSR two component signal transduction pathway (Leonhartsberger et al.,2001).The zinc responsive sensor kinase ZraS senses extracellular metal ions and can phosphorylate its response regulator ZraR under high Zn 2þor Pb 2þconditions.The phosphorylated ZraR can then activate the target P zraP promoter leading to GFP production.The cadmium sensor comprises the P zntA promoter fused to the gfp reporter by utilizing the E.colinative metal exporting ATPase pathway for Cd 2þor Zn 2þ(Binet and Poole,2000).The dose response curves show that the zinc sensor can sense both Zn 2þand Pb 2þthough with a decreased dynamic range for Pb 2þ(Fig.2E)while the cadmium sensor can sense both Cd 2þand Zn 2þthough at a less sensitive level for Zn 2þin the micro-and milli-molar range (Fig.2F).The charac-terised dose responses were fitted to the transfer function model (Eq.(1),Table 2).The input-output dose responses show that the output strengths of these sensors are different,i.e.,some regulated promoters like P merT and P luxI are strong while others like P arsR and P cusC are relatively weak.Thus we used different RBS (ribo-some binding site)to tune the maximum fluorescence output of these sensors by regulating the translational initiate rate of the gfp reporter (Table 1).Normally,using strong RBS can increase the sensitivities of the sensor but will slightly increase the back-ground basal level.Because these single input sensors will also be connected to the genetic logic gates in the next step,strong RBSs were used for characterizing arsenic and copper sensors and weak RBSs were used for characterizing mercury and 3OC 6HSL sensors (Fig.2)in order to balance their maximum output and default leaky levels.The detection thresholds of these sensors can also be tuned by varying the receptor protein levels in the cytoplasm.Typically,high level of the repressor receptor protein (e.g.,ArsR)in the cell will decrease the sensitivity of the sensor while high level of the activator receptor protein (e.g.,LuxR)will increase the sensitivity of the sensor.The constructed synthetic sensors have been developed to exhibit very high selectivity only for their target signalling molecules but with almost no or little recogni-tion specificity for a broad range of non-target molecules,as indicated in Fig.S2,with the exception of moderate multiple metal responses in the P zraP (responsive to Zn 2þand Pb 2þ)and P zntA (responsive to Cd 2þ,Zn 2þand Hg 2þ)sensors.3.2.Design and construction of modular double-input AND logic gated sensorsBased on the single input cell-based biosensors constructed,we next sought to construct an advanced biosensor that can detect two input signals at the same time and integrate them following a designed logic before producing the output response.To design a logic AND-gated cellular biosensor,we connected two transcriptional inputs of the single input sensors to a modular and orthogonal genetic AND gate we have engineered in E.coli recently (Wang et al.,2011)and used gfp or rfp as the output readout.The modular two-input AND gate comprises two heterologous genes,hrpR and hrpS ,and one s 54-dependent out-put promoter,hrpL ,from the hrp (hypersensitive response and pathogenecity)regulatory system of the plant pathogen P.syr-ingae (Hutcheson et al.,2001;Wang et al.,2011).The hrpR and hrpS encode two regulatory enhancer binding proteins that act synergistically by forming a heteromeric protein complex to co-activate the tightly regulated hrpL promoter (Jovanovic et al.,Table 2The best fits for characterised dose responses of the single-input biosensors with 95%confidence.Sensor reporter Target k (a.u.)n K M (m M)aR 2P arsR -rbs30-gfp Arsenic 371974311.11570.2512.89970.632À0.018570.04380.9971P cusC -rbs31-gfp Copper 2.0e471.383e4 1.34370.868 1.04e371.18e30.027770.05030.9883P merT -rbs32-gfp Mercury 85637594 4.8472.166 2.26770.1910.010670.02410.9983P luxI -rbs32-gfp 3OC 6HSL 1.571e470.056e4 6.63572.01131.64e À372.57e À30.018870.02420.9986P zraP -rbs30-gfp Zinc 57807392 2.76270.5850.335e370.027e30.010770.02700.9979P zraP -rbs30-gfp Lead21147548 1.74970.9330.334e370.123e30.030670.06680.9883P zntA -rbs31-gfp Cadmium 73267579 2.19270.5810.074e370.012e30.035970.03750.9980P zntA -rbs31-gfpZinc1.12e471.62e42.08571.6411.117e371.513e30.005970.03910.9821B.Wang et al./Biosensors and Bioelectronics 40(2013)368–3763722011).Both the inputs and output of the AND gate were designed to be promoters to facilitate their connection to different upstream and downstreamtranscriptional modules.Due to this modularity,the inputs can be rewired to different input sensors and the output can be used to drive various cellular responses.Fig.3A shows the schematic design of a two-input AND gated biosensor that can detect both arsenic and mercury ions with gfp as the output indicator.The synthetic sensor circuit comprises the arsenic and mercury sensors connected to the inputs of the modular AND gate to drive hrpR and hrpS respectively and the gfp fused to the hrpL output promoter of the AND gate.The constructed sensor behaviour was then systematically char-acterized in a 96well microtitre plate by measuring the output responses under 81combinations of the two input chemical inducers of varying concentrations.Fig.3A shows that the device behaves as expected,with clear digital-like logic AND character-istics:the sensor output is high only when both arsenic and mercury are above a certain threshold,similar to that of the single input sensors,sufficient to activate the two inputs of the genetic AND gate.Fig.3.Two-input AND logic gated cellular biosensors.(A)The arsenite and mercury sensitive AND logic gated sensor was measured using 81combinations of the two input metals (0,0.125,0.25,0.5,1.0,2.0,4.0,8.0,16.0m M arsenite by 0,0.0625,0.125,0.25,0.5,1.0,2.0,4.0,5.0m M mercury).(B)The 3OC 6HSL and copper sensitive AND logic gated sensor was measured using 72combinations of two input chemicals (0,0.39,1.56,6.25,25.0,50,100,400nM 3OC 6HSL by 0,0.00137,0.0041,0.0123,0.037,0.11,0.33,1.0,2.0mM CuSO 4).(C)The AND logic gated zinc sensor was measured using various levels of ZnCl 2or PbCl 2(0,0.0041,0.0123,0.037,0.111,0.222,0.333,0.5,0.75,1,1.2mM)or CdCl 2(0,0.00137,0.0041,0.0123,0.037,0.111,0.222,0.333,0.4mM).(D)Behaviour of the AND gated sensor for arsenic and mercury (Fig.3A)in the presence of other competing metals.The sensor responses are assayed under four logic combinations of the two target metal ions (16m M NaAsO 2plus 2m M HgCl 2,16m M NaAsO 2only,2m M HgCl 2only,and no target metals)either in the absence of other metals (Pure)or in the presence of two other competing chemicals with each metal added at 0.1mM and 3OC 6HSL at 100nM.Error bars,s.d.(n ¼4).In A and B,all data are the average of three repeats with variations less than 10%between biological replicates.B.Wang et al./Biosensors and Bioelectronics 40(2013)368–376373。