与疏浚有关的微量金属的移动
河道底泥污染对清淤疏浚水质影响分析
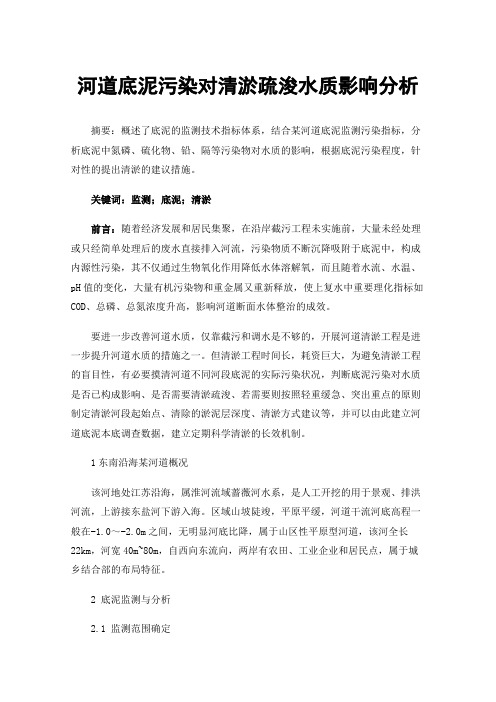
河道底泥污染对清淤疏浚水质影响分析摘要:概述了底泥的监测技术指标体系,结合某河道底泥监测污染指标,分析底泥中氮磷、硫化物、铅、隔等污染物对水质的影响,根据底泥污染程度,针对性的提出清淤的建议措施。
关键词:监测;底泥;清淤前言:随着经济发展和居民集聚,在沿岸截污工程未实施前,大量未经处理或只经简单处理后的废水直接排入河流,污染物质不断沉降吸附于底泥中,构成内源性污染,其不仅通过生物氧化作用降低水体溶解氧,而且随着水流、水温、pH值的变化,大量有机污染物和重金属又重新释放,使上复水中重要理化指标如COD、总磷、总氮浓度升高,影响河道断面水体整治的成效。
要进一步改善河道水质,仅靠截污和调水是不够的,开展河道清淤工程是进一步提升河道水质的措施之一。
但清淤工程时间长,耗资巨大,为避免清淤工程的盲目性,有必要摸清河道不同河段底泥的实际污染状况,判断底泥污染对水质是否已构成影响、是否需要清淤疏浚、若需要则按照轻重缓急、突出重点的原则制定清淤河段起始点、清除的淤泥层深度、清淤方式建议等,并可以由此建立河道底泥本底调查数据,建立定期科学清淤的长效机制。
1东南沿海某河道概况该河地处江苏沿海,属淮河流域蔷薇河水系,是人工开挖的用于景观、排洪河流,上游接东盐河下游入海。
区域山坡陡竣,平原平缓,河道干流河底高程一般在-1.0~-2.0m之间,无明显河底比降,属于山区性平原型河道,该河全长22km,河宽40m~80m,自西向东流向,两岸有农田、工业企业和居民点,属于城乡结合部的布局特征。
2 底泥监测与分析2.1 监测范围确定按照全面性、代表性的原则确定监测断面,本次监测断面包含该河段段的上、中、下游,并考虑了沿河排污口、支岔河、排水沟以及城镇建成区分布情况,并在连云港市沭新渠上设置1处背景对照断面。
2.2监测断面布设按照上述思路,本次调查监测断面共设置9处,其中河道中间段布设5处、上下游各布设3处、水质较好的沭新渠包庄桥附近布设背景对照断面1处,取样点布设自上游而下分别为W1、W2、W3、W4、W5、W6、W7、W8、W9共9个点位。
河道疏浚工程环境保护措施
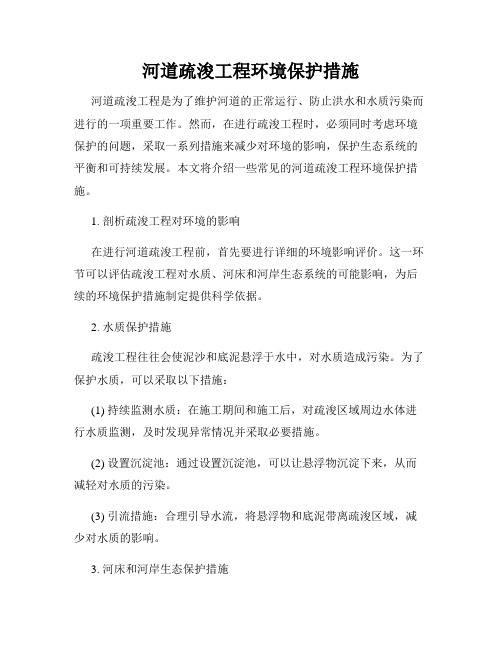
河道疏浚工程环境保护措施河道疏浚工程是为了维护河道的正常运行、防止洪水和水质污染而进行的一项重要工作。
然而,在进行疏浚工程时,必须同时考虑环境保护的问题,采取一系列措施来减少对环境的影响,保护生态系统的平衡和可持续发展。
本文将介绍一些常见的河道疏浚工程环境保护措施。
1. 剖析疏浚工程对环境的影响在进行河道疏浚工程前,首先要进行详细的环境影响评价。
这一环节可以评估疏浚工程对水质、河床和河岸生态系统的可能影响,为后续的环境保护措施制定提供科学依据。
2. 水质保护措施疏浚工程往往会使泥沙和底泥悬浮于水中,对水质造成污染。
为了保护水质,可以采取以下措施:(1) 持续监测水质:在施工期间和施工后,对疏浚区域周边水体进行水质监测,及时发现异常情况并采取必要措施。
(2) 设置沉淀池:通过设置沉淀池,可以让悬浮物沉淀下来,从而减轻对水质的污染。
(3) 引流措施:合理引导水流,将悬浮物和底泥带离疏浚区域,减少对水质的影响。
3. 河床和河岸生态保护措施疏浚工程对河床和河岸生态系统都会产生一定的影响,为了保护生态系统的平衡,可以采取以下措施:(1) 废弃物处理:对于疏浚过程中产生的废弃物,要进行妥善处理,可以采用分类、回收或安全填埋等方式。
(2) 植被保护:在工程施工过程中,应保护好河岸的植被,减少对植被的破坏。
(3) 动植物转移:如果疏浚工程将对当地动植物造成较大影响,可以采取转移的方式将它们安全地迁移至其他适宜的生境。
4. 废水和噪音控制措施在进行疏浚工程时,还应注意控制废水排放和噪音污染,以减少对周边环境和人居环境的不良影响。
(1) 废水处理:对于产生的废水,应依法进行处理,控制其中的污染物排放。
(2) 噪音控制:采用降噪设备、合理调整施工时间等措施,减少工程带来的噪音污染。
5. 整体效果评估在河道疏浚工程结束后,需要进行整体效果评估,检查环境保护措施的实施情况和效果,并根据评估结果进行必要的改进和完善。
总结:河道疏浚工程环境保护措施对于维护生态平衡和保护环境至关重要。
海港疏浚物处置方案
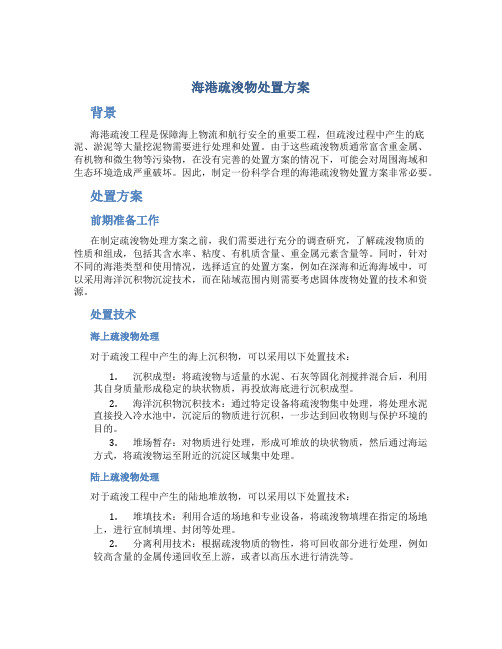
海港疏浚物处置方案背景海港疏浚工程是保障海上物流和航行安全的重要工程,但疏浚过程中产生的底泥、淤泥等大量挖泥物需要进行处理和处置。
由于这些疏浚物质通常富含重金属、有机物和微生物等污染物,在没有完善的处置方案的情况下,可能会对周围海域和生态环境造成严重破坏。
因此,制定一份科学合理的海港疏浚物处置方案非常必要。
处置方案前期准备工作在制定疏浚物处理方案之前,我们需要进行充分的调查研究,了解疏浚物质的性质和组成,包括其含水率、粘度、有机质含量、重金属元素含量等。
同时,针对不同的海港类型和使用情况,选择适宜的处置方案,例如在深海和近海海域中,可以采用海洋沉积物沉淀技术,而在陆域范围内则需要考虑固体废物处置的技术和资源。
处置技术海上疏浚物处理对于疏浚工程中产生的海上沉积物,可以采用以下处置技术:1.沉积成型:将疏浚物与适量的水泥、石灰等固化剂搅拌混合后,利用其自身质量形成稳定的块状物质,再投放海底进行沉积成型。
2.海洋沉积物沉积技术:通过特定设备将疏浚物集中处理,将处理水泥直接投入冷水池中,沉淀后的物质进行沉积,一步达到回收物则与保护环境的目的。
3.堆场暂存:对物质进行处理,形成可堆放的块状物质,然后通过海运方式,将疏浚物运至附近的沉淀区域集中处理。
陆上疏浚物处理对于疏浚工程中产生的陆地堆放物,可以采用以下处置技术:1.堆填技术:利用合适的场地和专业设备,将疏浚物填埋在指定的场地上,进行宣制填埋、封闭等处理。
2.分离利用技术:根据疏浚物质的物性,将可回收部分进行处理,例如较高含量的金属传递回收至上游,或者以高压水进行清洗等。
3.热化解技术:采用高温炉,通过水蒸气或加热的方式,将疏浚物完全分解、发酵并转化,获得新的生物质燃料等。
处置效果监控为保障处置效果的可控性和可检测性,我们需要在处置过程中建立完善的监测和检查体系。
其中,将重点监测疏浚物处置后的生态环境、水环境和空气环境,包括海水中的重金属元素含量、pH值和溶氧量等物理和化学指标,以及结核菌、80种致病生物和光合细胞的总计数等。
水域疏浚工程施工
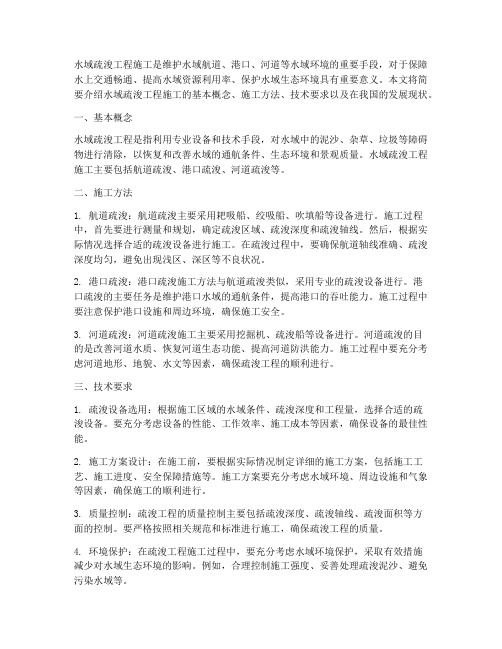
水域疏浚工程施工是维护水域航道、港口、河道等水域环境的重要手段,对于保障水上交通畅通、提高水域资源利用率、保护水域生态环境具有重要意义。
本文将简要介绍水域疏浚工程施工的基本概念、施工方法、技术要求以及在我国的发展现状。
一、基本概念水域疏浚工程是指利用专业设备和技术手段,对水域中的泥沙、杂草、垃圾等障碍物进行清除,以恢复和改善水域的通航条件、生态环境和景观质量。
水域疏浚工程施工主要包括航道疏浚、港口疏浚、河道疏浚等。
二、施工方法1. 航道疏浚:航道疏浚主要采用耙吸船、绞吸船、吹填船等设备进行。
施工过程中,首先要进行测量和规划,确定疏浚区域、疏浚深度和疏浚轴线。
然后,根据实际情况选择合适的疏浚设备进行施工。
在疏浚过程中,要确保航道轴线准确、疏浚深度均匀,避免出现浅区、深区等不良状况。
2. 港口疏浚:港口疏浚施工方法与航道疏浚类似,采用专业的疏浚设备进行。
港口疏浚的主要任务是维护港口水域的通航条件,提高港口的吞吐能力。
施工过程中要注意保护港口设施和周边环境,确保施工安全。
3. 河道疏浚:河道疏浚施工主要采用挖掘机、疏浚船等设备进行。
河道疏浚的目的是改善河道水质、恢复河道生态功能、提高河道防洪能力。
施工过程中要充分考虑河道地形、地貌、水文等因素,确保疏浚工程的顺利进行。
三、技术要求1. 疏浚设备选用:根据施工区域的水域条件、疏浚深度和工程量,选择合适的疏浚设备。
要充分考虑设备的性能、工作效率、施工成本等因素,确保设备的最佳性能。
2. 施工方案设计:在施工前,要根据实际情况制定详细的施工方案,包括施工工艺、施工进度、安全保障措施等。
施工方案要充分考虑水域环境、周边设施和气象等因素,确保施工的顺利进行。
3. 质量控制:疏浚工程的质量控制主要包括疏浚深度、疏浚轴线、疏浚面积等方面的控制。
要严格按照相关规范和标准进行施工,确保疏浚工程的质量。
4. 环境保护:在疏浚工程施工过程中,要充分考虑水域环境保护,采取有效措施减少对水域生态环境的影响。
河道疏浚底泥处理与资源利用方案研究
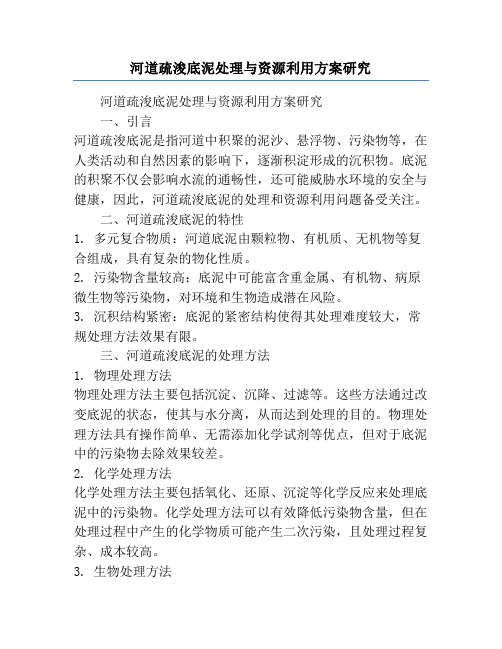
河道疏浚底泥处理与资源利用方案研究河道疏浚底泥处理与资源利用方案研究一、引言河道疏浚底泥是指河道中积聚的泥沙、悬浮物、污染物等,在人类活动和自然因素的影响下,逐渐积淀形成的沉积物。
底泥的积聚不仅会影响水流的通畅性,还可能威胁水环境的安全与健康,因此,河道疏浚底泥的处理和资源利用问题备受关注。
二、河道疏浚底泥的特性1. 多元复合物质:河道底泥由颗粒物、有机质、无机物等复合组成,具有复杂的物化性质。
2. 污染物含量较高:底泥中可能富含重金属、有机物、病原微生物等污染物,对环境和生物造成潜在风险。
3. 沉积结构紧密:底泥的紧密结构使得其处理难度较大,常规处理方法效果有限。
三、河道疏浚底泥的处理方法1. 物理处理方法物理处理方法主要包括沉淀、沉降、过滤等。
这些方法通过改变底泥的状态,使其与水分离,从而达到处理的目的。
物理处理方法具有操作简单、无需添加化学试剂等优点,但对于底泥中的污染物去除效果较差。
2. 化学处理方法化学处理方法主要包括氧化、还原、沉淀等化学反应来处理底泥中的污染物。
化学处理方法可以有效降低污染物含量,但在处理过程中产生的化学物质可能产生二次污染,且处理过程复杂、成本较高。
3. 生物处理方法生物处理方法通过利用微生物的生态功能,将底泥中的污染物降解为无害物质。
生物处理方法具有环境友好、能量耗费低等优点,但处理周期较长,且对处理条件有一定要求。
四、河道疏浚底泥的资源利用方案1. 土壤改良剂底泥中的有机质和无机物质可用作土壤改良剂。
通过确保其安全性和环境适应性,底泥可以被混合到农用土壤中,提高土壤肥力和保水性,促进植物生长。
2. 砖石材料制备底泥中的颗粒物可以通过加工成为砖石材料,用于道路建设或建筑材料。
这种方式可以实现对底泥的资源化利用,减少土地利用压力。
3. 生物质能源利用底泥中的有机质可以通过气化或厌氧发酵等方法,转化为生物质能源,如甲烷气体、生物质燃料等。
这样不仅可以减少对化石能源的依赖,还可以减少有机质的排放。
疏浚底泥重金属稳定化实验方案
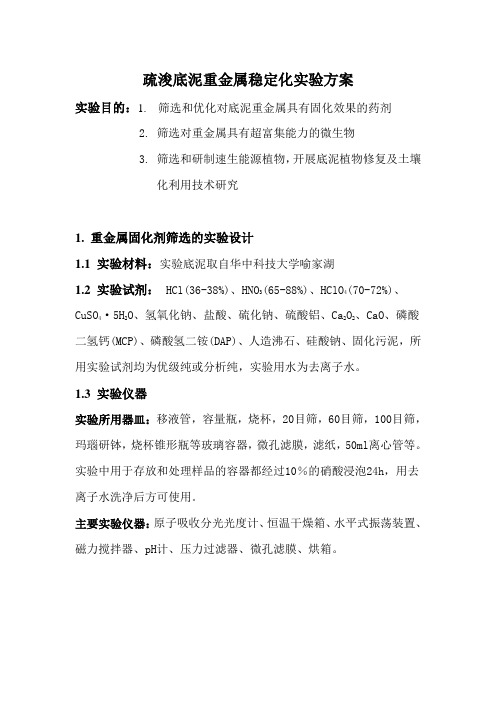
疏浚底泥重金属稳定化实验方案实验目的:1.筛选和优化对底泥重金属具有固化效果的药剂2. 筛选对重金属具有超富集能力的微生物3. 筛选和研制速生能源植物,开展底泥植物修复及土壤化利用技术研究1. 重金属固化剂筛选的实验设计1.1 实验材料:实验底泥取自华中科技大学喻家湖1.2 实验试剂:HCl(36-38%)、HNO3(65-88%)、HClO4(70-72%)、CuSO4·5H2O、氢氧化钠、盐酸、硫化钠、硫酸铝、Ca2O2、CaO、磷酸二氢钙(MCP)、磷酸氢二铵(DAP)、人造沸石、硅酸钠、固化污泥,所用实验试剂均为优级纯或分析纯,实验用水为去离子水。
1.3 实验仪器实验所用器皿:移液管,容量瓶,烧杯,20目筛,60目筛,100目筛,玛瑙研钵,烧杯锥形瓶等玻璃容器,微孔滤膜,滤纸,50ml离心管等。
实验中用于存放和处理样品的容器都经过10%的硝酸浸泡24h,用去离子水洗净后方可使用。
主要实验仪器:原子吸收分光光度计、恒温干燥箱、水平式振荡装置、磁力搅拌器、pH计、压力过滤器、微孔滤膜、烘箱。
1.4 实验步骤(1)现场观测与采样在华中科技大学喻家湖污染源、最深处的一条直线上及两边设置S1、S2、S3、S4、S5、S6、S7、S8、S9九个采样点,采样点多布设于水流缓慢,沉积稳定的地带,利用抓斗采泥器采集表层0-80cm底泥,保存于洁净塑料样品袋中(排出空气)。
迅速带回实验室,去除上层积水,拣除塑料、玻璃、石块和败叶等杂物, 经自然沉降1周,得到底泥样品。
图表 1 武汉喻家湖底泥采样点分布(2)底泥样品的预处理物理分析时,利用12000r/min离心机将底泥固液分离,得到间隙水和固体底泥。
固体底泥自然风干,先取风干样品100-200g,用玛瑙研钵研磨,然后过20目筛,留在筛上的底泥再倒在研钵上重新碾碎,如此反复,使全部底泥过筛然后将底泥混匀后盛于广口瓶中,作为底泥颗粒分析及其他物理性质测定之用。
疏浚工程对环境影响及保护措施

疏浚工程对环境影响及保护措施
疏浚工程可能对环境造成负面影响,包括:
1. 悬浮固体物质的释放:疏浚作业会扰动水底,导致大量泥沙、悬浮固体物质进入水体中,影响水质,破坏水生态环境。
2. 水下噪声:疏浚作业会产生高强度、频率不同的噪声,对水下生物和人类造成噪声污染。
3. 残留污染物:疏浚作业会释放污染底泥,而且船只和设备使用燃油与润滑油也可能污染水体。
针对这些环境影响,疏浚工程需要采取以下保护措施:
1. 疏浚点的设立和选择:疏浚前应充分考虑疏浚点选取,有充分地调查、分析和评估,再做出选择,避免破坏环境。
2. 水下噪声降低措施:采用降噪器、吸音装置等技术来降低水下噪声。
3. 废弃物处置:废弃物的清理、处理、回收和处置要规范,确保不会进一步损害环境。
4. 水质监测和治理:在疏浚和后期工程中,需要对水质进行监测和治理,确保水体质量达到要求。
5. 疏浚船舶的环保设备:使用符合环保标准的疏浚船舶,在疏浚过程中加装环保设备,如油水分离器等。
河道疏浚的原因
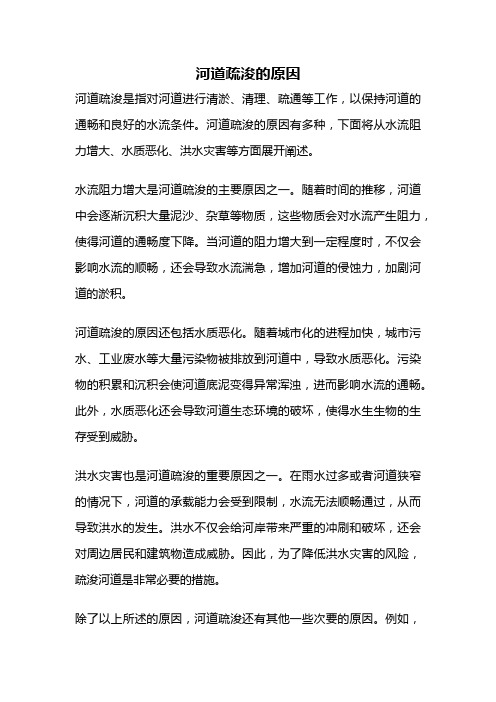
河道疏浚的原因河道疏浚是指对河道进行清淤、清理、疏通等工作,以保持河道的通畅和良好的水流条件。
河道疏浚的原因有多种,下面将从水流阻力增大、水质恶化、洪水灾害等方面展开阐述。
水流阻力增大是河道疏浚的主要原因之一。
随着时间的推移,河道中会逐渐沉积大量泥沙、杂草等物质,这些物质会对水流产生阻力,使得河道的通畅度下降。
当河道的阻力增大到一定程度时,不仅会影响水流的顺畅,还会导致水流湍急,增加河道的侵蚀力,加剧河道的淤积。
河道疏浚的原因还包括水质恶化。
随着城市化的进程加快,城市污水、工业废水等大量污染物被排放到河道中,导致水质恶化。
污染物的积累和沉积会使河道底泥变得异常浑浊,进而影响水流的通畅。
此外,水质恶化还会导致河道生态环境的破坏,使得水生生物的生存受到威胁。
洪水灾害也是河道疏浚的重要原因之一。
在雨水过多或者河道狭窄的情况下,河道的承载能力会受到限制,水流无法顺畅通过,从而导致洪水的发生。
洪水不仅会给河岸带来严重的冲刷和破坏,还会对周边居民和建筑物造成威胁。
因此,为了降低洪水灾害的风险,疏浚河道是非常必要的措施。
除了以上所述的原因,河道疏浚还有其他一些次要的原因。
例如,河道中的漂浮物、水生植物等也会对水流产生一定的阻力,影响河道的通畅性。
此外,河道中的底泥沉积还会使得水体中的氧气供应不足,对水生生物造成损害。
因此,及时疏浚河道,清除这些阻力物质,对于维护河道的生态环境和水质是非常重要的。
河道疏浚的原因主要包括水流阻力增大、水质恶化、洪水灾害等。
随着城市化的进程加快和人们对生态环境的重视,对河道进行疏浚已经成为一项重要的工作。
通过清淤、清理、疏通等措施,可以保持河道的通畅和良好的水流条件,维护河道的生态环境和水质,减少洪水灾害的风险,确保人们的生活和生产不受到河道问题的困扰。
河道疏浚措施

河道疏浚措施简介河道疏浚是指对河流、水道进行除淤、开浚、清淤等工程措施,以保持河道通畅,防止水患发生,并提高水运能力。
河道疏浚措施通常包括机械疏浚、人工疏浚和生态疏浚等多种手段。
本文将介绍各种河道疏浚措施的具体方法和适用情况。
机械疏浚措施机械疏浚是利用各种机械设备进行的河道疏浚措施。
常用的机械疏浚方法包括挖掘机疏浚、抽泥船疏浚、镇江机疏浚等。
挖掘机疏浚挖掘机疏浚是利用挖掘机将淤泥、杂草等物质从河床或河岸上清除的方法。
具体操作包括挖掘机铲斗切坡清除陡坡,铲斗翻砂清除粉沙,铲斗抓取清除淤泥等。
抽泥船疏浚抽泥船疏浚是利用抽泥船抽取河底淤泥的方法。
抽泥船通常配备有抽水泵和输送管路,可以方便地将淤泥抽取到抽泥船上,并通过管路输送至指定位置。
镇江机疏浚镇江机疏浚是将钢管插入河床,并利用镇江机旋转,通过钢管的摩擦力将淤泥推挤到外侧,从而清除淤泥的方法。
镇江机疏浚适用于淤泥较软的河道,能够高效地清除淤泥。
人工疏浚措施人工疏浚是利用人力进行的河道疏浚措施。
常用的人工疏浚方法包括人工清淤、人工开刷、人工拆渡等。
人工清淤人工清淤是利用工人用铁锹、铲子等工具进行的清淤工作。
工人通过挖掘、筛选和清除淤泥,将河床恢复到正常水深,以保持河道通畅。
人工开刷人工开刷是利用工人进行的开拓河道的工作。
工人使用铁锨、锤子等工具进行的河道开挖和疏通工作,以增加河道的水量和水流速度。
人工拆渡人工拆渡是指利用人力拆除河道上的障碍物,以恢复河道的畅通。
常见的障碍物包括枯木、倒塌的建筑物等。
通过人工拆除这些障碍物,可以恢复河道通畅。
生态疏浚措施生态疏浚是指通过生态手段进行的河道疏浚措施。
常用的生态疏浚方法包括河道修复、湿地恢复、植被恢复等。
河道修复河道修复是指通过退化的河道进行修复工作,以恢复其正常的功能。
工作包括修复河床和岸坡、重建生态系统、恢复水质等,使河道能够自然地保持通畅。
湿地恢复湿地恢复是指修复退化的湿地环境,以恢复其原有的水生态系统功能。
疏浚土的处理
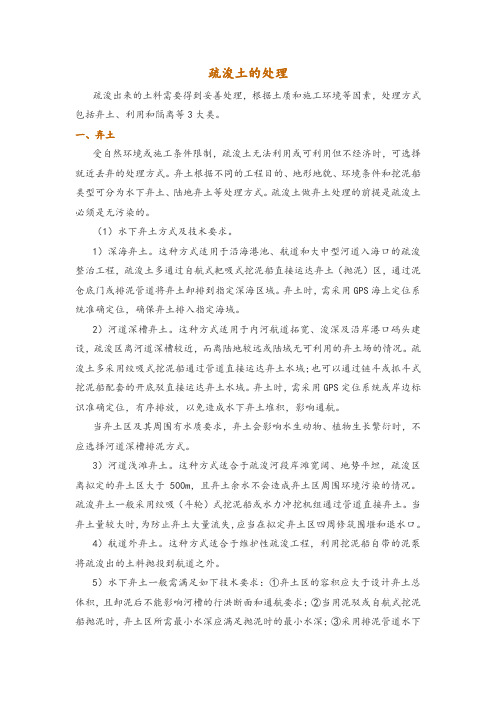
疏浚土的处理疏浚出来的土料需要得到妥善处理,根据土质和施工环境等因素,处理方式包括弃土、利用和隔离等3大类。
一、弃土受自然环境或施工条件限制,疏浚土无法利用或可利用但不经济时,可选择就近丢弃的处理方式。
弃土根据不同的工程目的、地形地貌、环境条件和挖泥船类型可分为水下弃土、陆地弃土等处理方式。
疏浚土做弃土处理的前提是疏浚土必须是无污染的。
(1)水下弃土方式及技术要求。
1)深海弃土。
这种方式适用于沿海港池、航道和大中型河道入海口的疏浚整治工程,疏浚土多通过自航式耙吸式挖泥船直接运达弃土(抛泥)区,通过泥仓底门或排泥管道将弃土卸排到指定深海区域。
弃土时,需采用GPS海上定位系统准确定位,确保弃土排入指定海域。
2)河道深槽弃土。
这种方式适用于内河航道拓宽、浚深及沿岸港口码头建设,疏浚区离河道深槽较近,而离陆地较远或陆域无可利用的弃土场的情况。
疏浚土多采用绞吸式挖泥船通过管道直接运达弃土水域;也可以通过链斗或抓斗式挖泥船配套的开底驳直接运达弃土水域。
弃土时,需采用GPS定位系统或岸边标识准确定位,有序排放,以免造成水下弃土堆积,影响通航。
当弃土区及其周围有水质要求,弃土会影响水生动物、植物生长繁衍时,不应选择河道深槽排泥方式。
3)河道浅滩弃土。
这种方式适合于疏浚河段岸滩宽阔、地势平坦,疏浚区离拟定的弃土区大于500m,且弃土余水不会造成弃土区周围环境污染的情况。
疏浚弃土一般采用绞吸(斗轮)式挖泥船或水力冲挖机组通过管道直接弃土。
当弃土量较大时,为防止弃土大量流失,应当在拟定弃土区四周修筑围堰和退水口。
4)航道外弃土。
这种方式适合于维护性疏浚工程,利用挖泥船自带的泥泵将疏浚出的土料抛投到航道之外。
5)水下弃土一般需满足如下技术要求:①弃土区的容积应大于设计弃土总体积,且卸泥后不能影响河槽的行洪断面和通航要求;②当用泥驳或自航式挖泥船抛泥时,弃土区所需最小水深应满足抛泥时的最小水深;③采用排泥管道水下直接排泥时,水面浮管及作业船舶不得影响正常通航,并要防止水下锚缆或排泥管口被淤埋;④弃土区周围水面须设置明显标志,标示出弃土范围与弃土顺序,以利于作业时控制;⑤对有通航要求的河道弃土区,应联系航政管理部门发布禁航或避让通告,施工船只应随时准备采取必要的避让措施。
疏浚工程常用施工方法自测
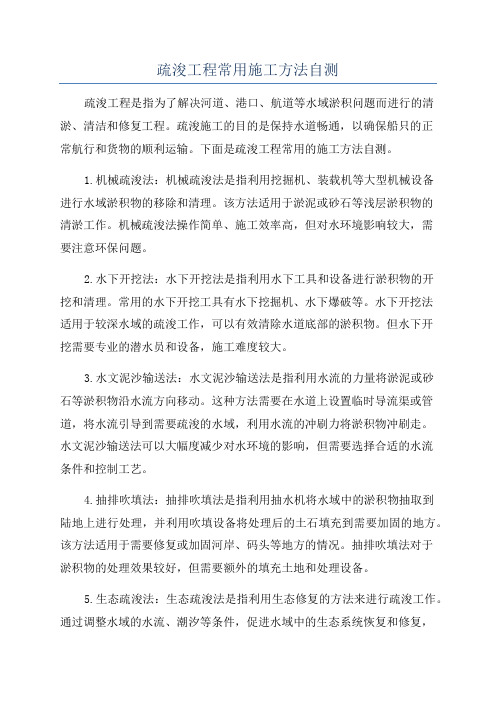
疏浚工程常用施工方法自测疏浚工程是指为了解决河道、港口、航道等水域淤积问题而进行的清淤、清洁和修复工程。
疏浚施工的目的是保持水道畅通,以确保船只的正常航行和货物的顺利运输。
下面是疏浚工程常用的施工方法自测。
1.机械疏浚法:机械疏浚法是指利用挖掘机、装载机等大型机械设备进行水域淤积物的移除和清理。
该方法适用于淤泥或砂石等浅层淤积物的清淤工作。
机械疏浚法操作简单、施工效率高,但对水环境影响较大,需要注意环保问题。
2.水下开挖法:水下开挖法是指利用水下工具和设备进行淤积物的开挖和清理。
常用的水下开挖工具有水下挖掘机、水下爆破等。
水下开挖法适用于较深水域的疏浚工作,可以有效清除水道底部的淤积物。
但水下开挖需要专业的潜水员和设备,施工难度较大。
3.水文泥沙输送法:水文泥沙输送法是指利用水流的力量将淤泥或砂石等淤积物沿水流方向移动。
这种方法需要在水道上设置临时导流渠或管道,将水流引导到需要疏浚的水域,利用水流的冲刷力将淤积物冲刷走。
水文泥沙输送法可以大幅度减少对水环境的影响,但需要选择合适的水流条件和控制工艺。
4.抽排吹填法:抽排吹填法是指利用抽水机将水域中的淤积物抽取到陆地上进行处理,并利用吹填设备将处理后的土石填充到需要加固的地方。
该方法适用于需要修复或加固河岸、码头等地方的情况。
抽排吹填法对于淤积物的处理效果较好,但需要额外的填充土地和处理设备。
5.生态疏浚法:生态疏浚法是指利用生态修复的方法来进行疏浚工作。
通过调整水域的水流、潮汐等条件,促进水域中的生态系统恢复和修复,逐步清除淤积物。
生态疏浚法对于水域生态环境的保护和修复效果显著,但施工周期相对较长。
以上是疏浚工程常用的施工方法自测,每种方法都有其适用的场景和限制条件,根据具体情况选择合适的施工方法是很重要的。
在进行疏浚工程时,还要注意环境保护,以最大程度减少对水环境的影响。
河道疏浚危险源辨识清单
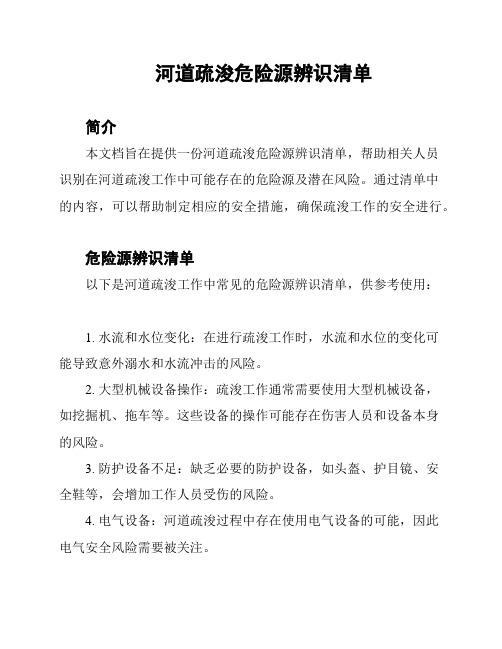
河道疏浚危险源辨识清单简介本文档旨在提供一份河道疏浚危险源辨识清单,帮助相关人员识别在河道疏浚工作中可能存在的危险源及潜在风险。
通过清单中的内容,可以帮助制定相应的安全措施,确保疏浚工作的安全进行。
危险源辨识清单以下是河道疏浚工作中常见的危险源辨识清单,供参考使用:1. 水流和水位变化:在进行疏浚工作时,水流和水位的变化可能导致意外溺水和水流冲击的风险。
2. 大型机械设备操作:疏浚工作通常需要使用大型机械设备,如挖掘机、拖车等。
这些设备的操作可能存在伤害人员和设备本身的风险。
3. 防护设备不足:缺乏必要的防护设备,如头盔、护目镜、安全鞋等,会增加工作人员受伤的风险。
4. 电气设备:河道疏浚过程中存在使用电气设备的可能,因此电气安全风险需要被关注。
5. 疏浚材料堆放:未妥善堆放疏浚材料可能导致坍塌和倾斜的风险。
6. 交通安全:疏浚工作现场的交通安全需要得到重视,以防止车辆碰撞和行人受伤。
安全措施建议在进行河道疏浚工作时,应采取以下安全措施以保障工作人员的安全:1. 提供适当的防护设备,如头盔、护目镜、安全鞋等,并确保每位工作人员正确使用。
2. 对工作人员进行必要的培训,确保其熟悉操作大型机械设备的方法及注意事项。
3. 在进行疏浚工作前,对工作现场进行安全检查,确保没有水流和水位异常以及其他危险源。
4. 对机械设备进行定期检查和维护,以确保其正常运行并降低故障风险。
5. 规划安全的疏浚材料堆放区域,并确保材料堆放牢固稳定。
6. 设置警示标志,提醒过往车辆和行人注意疏浚现场的存在,同时确保安全通行。
结论河道疏浚工作涉及许多潜在的危险源,但通过辨识这些危险源并采取相应的安全措施,可以减少事故发生的风险,保证工作人员的安全。
本文档提供了一份河道疏浚危险源辨识清单和建议的安全措施,可作为相关工作人员进行工作时的参考。
专用环保疏浚施工技术
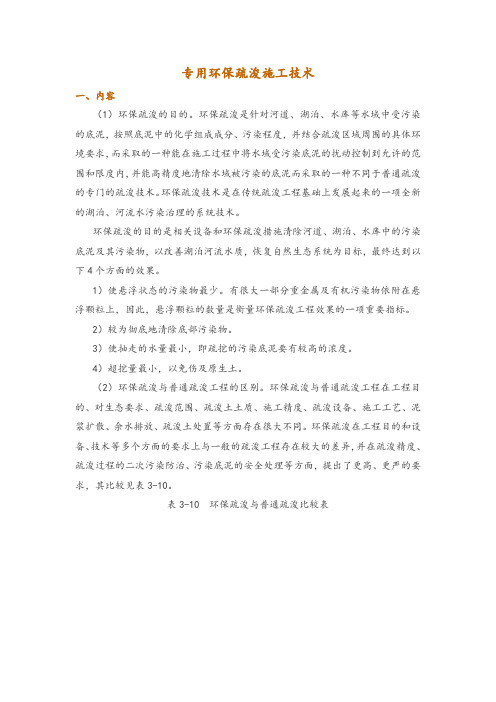
专用环保疏浚施工技术一、内容(1)环保疏浚的目的。
环保疏浚是针对河道、湖泊、水库等水域中受污染的底泥,按照底泥中的化学组成成分、污染程度,并结合疏浚区域周围的具体环境要求,而采取的一种能在施工过程中将水域受污染底泥的扰动控制到允许的范围和限度内,并能高精度地清除水域被污染的底泥而采取的一种不同于普通疏浚的专门的疏浚技术。
环保疏浚技术是在传统疏浚工程基础上发展起来的一项全新的湖泊、河流水污染治理的系统技术。
环保疏浚的目的是相关设备和环保疏浚措施清除河道、湖泊、水库中的污染底泥及其污染物,以改善湖泊河流水质,恢复自然生态系统为目标,最终达到以下4个方面的效果。
1)使悬浮状态的污染物最少。
有很大一部分重金属及有机污染物依附在悬浮颗粒上,因此,悬浮颗粒的数量是衡量环保疏浚工程效果的一项重要指标。
2)较为彻底地清除底部污染物。
3)使抽走的水量最小,即疏挖的污染底泥要有较高的浓度。
4)超挖量最小,以免伤及原生土。
(2)环保疏浚与普通疏浚工程的区别。
环保疏浚与普通疏浚工程在工程目的、对生态要求、疏浚范围、疏浚土土质、施工精度、疏浚设备、施工工艺、泥浆扩散、余水排放、疏浚土处置等方面存在很大不同。
环保疏浚在工程目的和设备、技术等多个方面的要求上与一般的疏浚工程存在较大的差异,并在疏浚精度、疏浚过程的二次污染防治、污染底泥的安全处理等方面,提出了更高、更严的要求,其比较见表3-10。
表3-10 环保疏浚与普通疏浚比较表续表(3)环保性疏浚土料特性。
1)环保性疏浚土料的物理特性。
环保性疏浚的对象是受污染的底泥,其特点是颗粒细、含水量高。
一般情况下,可以按密度变化范围大致划分为浮泥、流塑状淤泥、软塑状淤泥和淤泥质土共4个层面:①细颗粒泥沙经絮凝沉落到水底后,要经过很长时间才能变得比较密实,在尚未密实之前具有很强的流动性和悬浮性,称为浮泥,浮泥的密度范围为1.0~1.2g/cm3;②浮泥经进一步沉淀固结,流动性减小,当密度达到1.2~1.5g/cm3时,便成为流塑状淤泥;③当流塑状淤泥继续沉降,内部孔隙水被排走,密度增加到1.5~1.8g/cm3时,结构趋于稳定,在水流作用下不会直接悬扬、流动,已经属于软塑状淤泥的范畴;④当密度达到1.8g/cm3以上时,已成为淤泥质土。
疏浚工程知识点总结
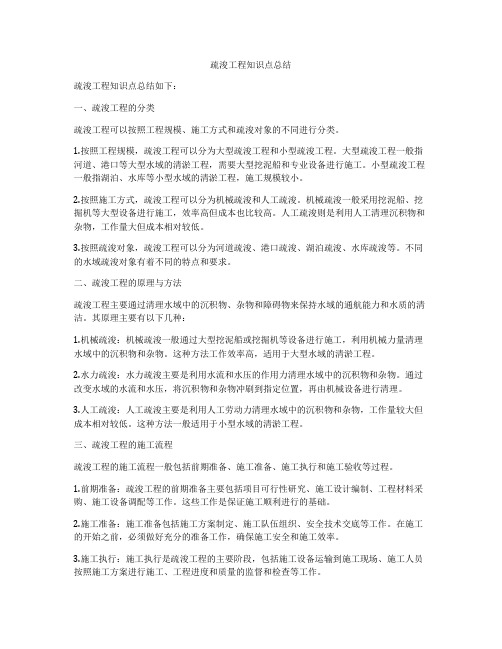
疏浚工程知识点总结疏浚工程知识点总结如下:一、疏浚工程的分类疏浚工程可以按照工程规模、施工方式和疏浚对象的不同进行分类。
1.按照工程规模,疏浚工程可以分为大型疏浚工程和小型疏浚工程。
大型疏浚工程一般指河道、港口等大型水域的清淤工程,需要大型挖泥船和专业设备进行施工。
小型疏浚工程一般指湖泊、水库等小型水域的清淤工程,施工规模较小。
2.按照施工方式,疏浚工程可以分为机械疏浚和人工疏浚。
机械疏浚一般采用挖泥船、挖掘机等大型设备进行施工,效率高但成本也比较高。
人工疏浚则是利用人工清理沉积物和杂物,工作量大但成本相对较低。
3.按照疏浚对象,疏浚工程可以分为河道疏浚、港口疏浚、湖泊疏浚、水库疏浚等。
不同的水域疏浚对象有着不同的特点和要求。
二、疏浚工程的原理与方法疏浚工程主要通过清理水域中的沉积物、杂物和障碍物来保持水域的通航能力和水质的清洁。
其原理主要有以下几种:1.机械疏浚:机械疏浚一般通过大型挖泥船或挖掘机等设备进行施工,利用机械力量清理水域中的沉积物和杂物。
这种方法工作效率高,适用于大型水域的清淤工程。
2.水力疏浚:水力疏浚主要是利用水流和水压的作用力清理水域中的沉积物和杂物。
通过改变水域的水流和水压,将沉积物和杂物冲刷到指定位置,再由机械设备进行清理。
3.人工疏浚:人工疏浚主要是利用人工劳动力清理水域中的沉积物和杂物,工作量较大但成本相对较低。
这种方法一般适用于小型水域的清淤工程。
三、疏浚工程的施工流程疏浚工程的施工流程一般包括前期准备、施工准备、施工执行和施工验收等过程。
1.前期准备:疏浚工程的前期准备主要包括项目可行性研究、施工设计编制、工程材料采购、施工设备调配等工作。
这些工作是保证施工顺利进行的基础。
2.施工准备:施工准备包括施工方案制定、施工队伍组织、安全技术交底等工作。
在施工的开始之前,必须做好充分的准备工作,确保施工安全和施工效率。
3.施工执行:施工执行是疏浚工程的主要阶段,包括施工设备运输到施工现场、施工人员按照施工方案进行施工、工程进度和质量的监督和检查等工作。
航道疏浚对水域生态的影响
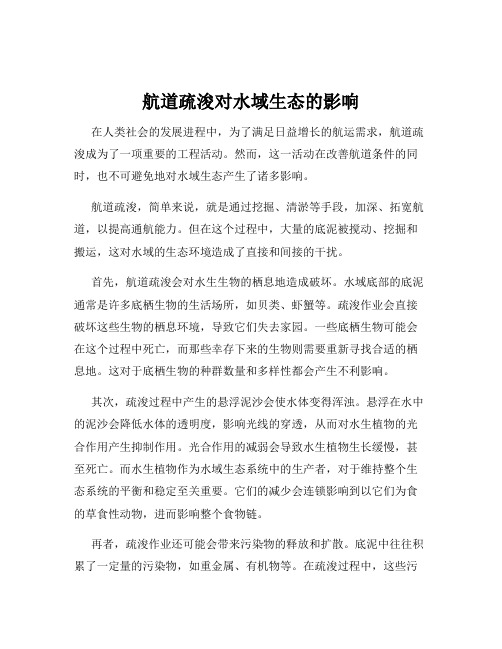
航道疏浚对水域生态的影响在人类社会的发展进程中,为了满足日益增长的航运需求,航道疏浚成为了一项重要的工程活动。
然而,这一活动在改善航道条件的同时,也不可避免地对水域生态产生了诸多影响。
航道疏浚,简单来说,就是通过挖掘、清淤等手段,加深、拓宽航道,以提高通航能力。
但在这个过程中,大量的底泥被搅动、挖掘和搬运,这对水域的生态环境造成了直接和间接的干扰。
首先,航道疏浚会对水生生物的栖息地造成破坏。
水域底部的底泥通常是许多底栖生物的生活场所,如贝类、虾蟹等。
疏浚作业会直接破坏这些生物的栖息环境,导致它们失去家园。
一些底栖生物可能会在这个过程中死亡,而那些幸存下来的生物则需要重新寻找合适的栖息地。
这对于底栖生物的种群数量和多样性都会产生不利影响。
其次,疏浚过程中产生的悬浮泥沙会使水体变得浑浊。
悬浮在水中的泥沙会降低水体的透明度,影响光线的穿透,从而对水生植物的光合作用产生抑制作用。
光合作用的减弱会导致水生植物生长缓慢,甚至死亡。
而水生植物作为水域生态系统中的生产者,对于维持整个生态系统的平衡和稳定至关重要。
它们的减少会连锁影响到以它们为食的草食性动物,进而影响整个食物链。
再者,疏浚作业还可能会带来污染物的释放和扩散。
底泥中往往积累了一定量的污染物,如重金属、有机物等。
在疏浚过程中,这些污染物可能会被重新释放到水体中,加剧水质的恶化。
这不仅对水生生物的生存造成威胁,还可能影响到周边地区的用水安全。
另外,航道疏浚会改变水流的速度和流向。
水流是水域生态系统中物质和能量传递的重要载体。
水流的改变可能会影响到鱼类等水生动物的洄游路线,干扰它们的繁殖和生长。
同时,水流的变化也可能会影响到营养物质的分布,进而对水域生态系统的结构和功能产生深远影响。
然而,我们也不能因噎废食,完全否定航道疏浚的积极作用。
在合理规划和科学管理的前提下,航道疏浚可以带来显著的经济和社会效益。
例如,它可以提高航运效率,促进贸易发展,为地区经济增长做出贡献。
2024年一级建造师《港口与航道工程》科目上半年模拟试题9
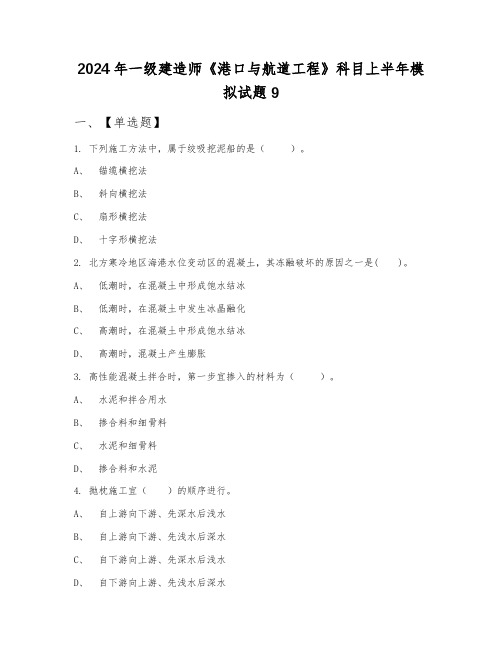
2024年一级建造师《港口与航道工程》科目上半年模拟试题9一、【单选题】1. 下列施工方法中,属于绞吸挖泥船的是()。
A、锚缆横挖法B、斜向横挖法C、扇形横挖法D、十字形横挖法2. 北方寒冷地区海港水位变动区的混凝土,其冻融破坏的原因之一是( )。
A、低潮时,在混凝土中形成饱水结冰B、低潮时,在混凝土中发生冰晶融化C、高潮时,在混凝土中形成饱水结冰D、高潮时,混凝土产生膨胀3. 高性能混凝土拌合时,第一步宜掺入的材料为()。
A、水泥和拌合用水B、掺合料和细骨料C、水泥和细骨料D、掺合料和水泥4. 抛枕施工宜()的顺序进行。
A、自上游向下游、先深水后浅水B、自上游向下游、先浅水后深水C、自下游向上游、先深水后浅水D、自下游向上游、先浅水后深水5. 挖泥船吹填施工中细砂在水面以上形成的坡度为()。
A、 1:25~1:50B、 1:50~1:100C、 1:15~1:50D、 1:30~l:1006. 港航工程设计变更可以由发包人、承包人、监理人中任何一方提出变更建议,但变更指示只能由()发出。
A、设计B、监理人C、业主D、施工单位7. 治理管涌与流沙(土)的原则是以防为主,大范围的流沙(土)险情出现时,首先应采取的有效措施是()。
A、截水防渗B、回土压顶C、土质改良D、人工降水8. 根据交通运输部《公路水运建设工程质量事故等级划分和报告制度》,因质量原因导致80万元直接经济损失的,属于()。
A、较大质量事故B、质量隐患C、一般质量事故D、质量问题9. 港口与航道工程的竣工验收前相关费属于()。
A、前期工作费B、建设管理费C、工程建设其他费用D、工程费用10. 海港工程的极端高水位应采用重现期为()年的年极值高水位。
A、 30B、 40C、 50D、 10011. 振冲挤密法适合于处理()。
A、粉质砂土B、砂土C、粉土D、黏性土12. 高桩码头工程施工组织设计编制中,主要施工方案的内容有:挖泥、测量、沉桩、构件预制及安装、模板工程、钢筋工程、()、土石方工程、设备安装工程、附属设施安装工程等。
疏浚污染
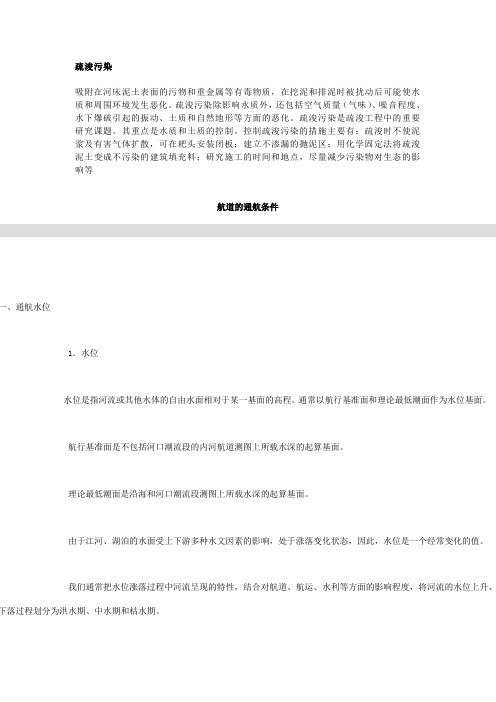
疏浚污染吸附在河床泥土表面的污物和重金属等有毒物质,在挖泥和排泥时被扰动后可能使水质和周围环境发生恶化。
疏浚污染除影响水质外,还包括空气质量(气味)、噪音程度、水下爆破引起的振动、土质和自然地形等方面的恶化。
疏浚污染是疏浚工程中的重要研究课题。
其重点是水质和土质的控制。
控制疏浚污染的措施主要有:疏浚时不使泥浆及有害气体扩散,可在耙头安装闭板;建立不渗漏的抛泥区;用化学固定法将疏浚泥土变成不污染的建筑填充料;研究施工的时间和地点,尽量减少污染物对生态的影响等航道的通航条件一、通航水位1.水位水位是指河流或其他水体的自由水面相对于某一基面的高程。
通常以航行基准面和理论最低潮面作为水位基面。
航行基准面是不包括河口潮流段的内河航道测图上所载水深的起算基面。
理论最低潮面是沿海和河口潮流段测图上所载水深的起算基面。
由于江河、湖泊的水面受上下游多种水文因素的影响,处于涨落变化状态,因此,水位是一个经常变化的值。
我们通常把水位涨落过程中河流呈现的特性,结合对航道、航运、水利等方面的影响程度,将河流的水位上升、下落过程划分为洪水期、中水期和枯水期。
2.设计最高通航水位设计最高通航水位是设计规定某一河段或具体部位允许标准船舶或船队正常通航的最高水位。
对船闸而言,当实际水位超过设计最高通航水位时,船舶便不能通航;对无船闸河段,它并不是断航水位,但是,对船舶水线以上高度和航速有限制。
3.设计最低通航水位设计最低通航水位是设计规定某一河段或具体部位允许标准船舶或船队正常通航的最低水位。
当实际水位低于设计最低通航水位时,航道不能保证标准维护水深,但吃水适宜的船舶可以照常航行,大型船舶(船队)需要减载控制吃水方可航行。
二、通航条件通航条件是指与通航有关的条件,包括通航尺度、水流条件、气象条件、河床边界条件和通航设施状况等的总称。
这里主要介绍通航尺度和通航水流条件。
1.通航尺度通航尺度是航道尺度、船闸有效尺度和通航净空尺度的总称。
疏浚工程师试题
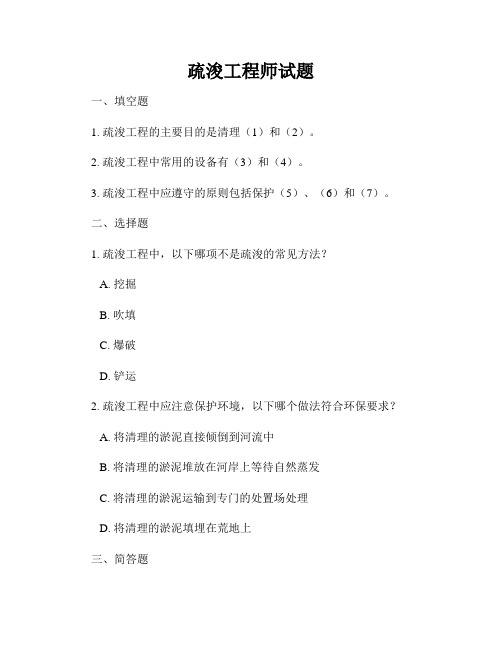
疏浚工程师试题
一、填空题
1. 疏浚工程的主要目的是清理(1)和(2)。
2. 疏浚工程中常用的设备有(3)和(4)。
3. 疏浚工程中应遵守的原则包括保护(5)、(6)和(7)。
二、选择题
1. 疏浚工程中,以下哪项不是疏浚的常见方法?
A. 挖掘
B. 吹填
C. 爆破
D. 铲运
2. 疏浚工程中应注意保护环境,以下哪个做法符合环保要求?
A. 将清理的淤泥直接倾倒到河流中
B. 将清理的淤泥堆放在河岸上等待自然蒸发
C. 将清理的淤泥运输到专门的处置场处理
D. 将清理的淤泥填埋在荒地上
三、简答题
1. 请简要描述疏浚工程中所遇到的主要困难及解决方法。
2. 谈谈你认为一个合格的疏浚工程师需要具备哪些技术能力和素质?
四、综合题
某城市的某河段淤积严重,需要进行疏浚工程。
请根据以下情况,
制定疏浚方案,并阐述具体实施步骤及预期效果。
情况:
1. 河段长度约1公里,淤积厚度约2米;
2. 淤泥质地较软,含有少量杂质;
3. 沿岸有部分建筑和植被需要保护;
4. 水流较缓,没有明显的水生生物栖息。
请根据以上试题完成答卷。
感谢您参与本次疏浚工程师试题考核!。
疏浚的名词解释
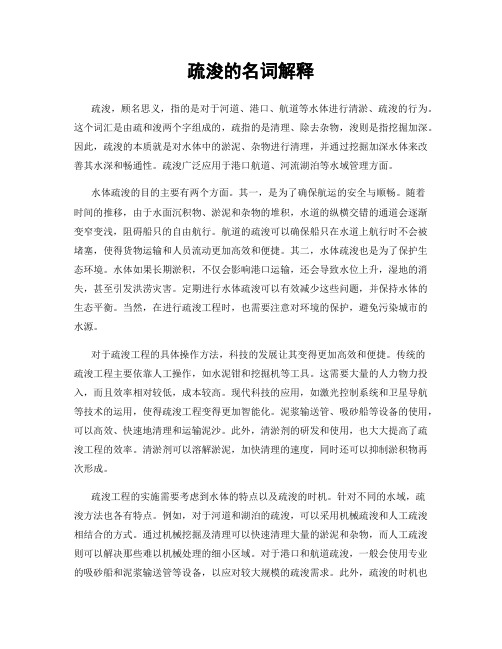
疏浚的名词解释疏浚,顾名思义,指的是对于河道、港口、航道等水体进行清淤、疏浚的行为。
这个词汇是由疏和浚两个字组成的,疏指的是清理、除去杂物,浚则是指挖掘加深。
因此,疏浚的本质就是对水体中的淤泥、杂物进行清理,并通过挖掘加深水体来改善其水深和畅通性。
疏浚广泛应用于港口航道、河流湖泊等水域管理方面。
水体疏浚的目的主要有两个方面。
其一,是为了确保航运的安全与顺畅。
随着时间的推移,由于水面沉积物、淤泥和杂物的堆积,水道的纵横交错的通道会逐渐变窄变浅,阻碍船只的自由航行。
航道的疏浚可以确保船只在水道上航行时不会被堵塞,使得货物运输和人员流动更加高效和便捷。
其二,水体疏浚也是为了保护生态环境。
水体如果长期淤积,不仅会影响港口运输,还会导致水位上升,湿地的消失,甚至引发洪涝灾害。
定期进行水体疏浚可以有效减少这些问题,并保持水体的生态平衡。
当然,在进行疏浚工程时,也需要注意对环境的保护,避免污染城市的水源。
对于疏浚工程的具体操作方法,科技的发展让其变得更加高效和便捷。
传统的疏浚工程主要依靠人工操作,如水泥钳和挖掘机等工具。
这需要大量的人力物力投入,而且效率相对较低,成本较高。
现代科技的应用,如激光控制系统和卫星导航等技术的运用,使得疏浚工程变得更加智能化。
泥浆输送管、吸砂船等设备的使用,可以高效、快速地清理和运输泥沙。
此外,清淤剂的研发和使用,也大大提高了疏浚工程的效率。
清淤剂可以溶解淤泥,加快清理的速度,同时还可以抑制淤积物再次形成。
疏浚工程的实施需要考虑到水体的特点以及疏浚的时机。
针对不同的水域,疏浚方法也各有特点。
例如,对于河道和湖泊的疏浚,可以采用机械疏浚和人工疏浚相结合的方式。
通过机械挖掘及清理可以快速清理大量的淤泥和杂物,而人工疏浚则可以解决那些难以机械处理的细小区域。
对于港口和航道疏浚,一般会使用专业的吸砂船和泥浆输送管等设备,以应对较大规模的疏浚需求。
此外,疏浚的时机也需要科学合理的选择。
一般来说,疏浚工程常在枯水期进行,这样能够尽可能减少水体对生态环境的影响。
- 1、下载文档前请自行甄别文档内容的完整性,平台不提供额外的编辑、内容补充、找答案等附加服务。
- 2、"仅部分预览"的文档,不可在线预览部分如存在完整性等问题,可反馈申请退款(可完整预览的文档不适用该条件!)。
- 3、如文档侵犯您的权益,请联系客服反馈,我们会尽快为您处理(人工客服工作时间:9:00-18:30)。
Wat.Res.Vol.35,No.8,pp.1979–1986,2001#2001Elsevier Science Ltd.All rights reservedPrinted in Great Britain0043-1354/01/$-see front matterPII:S0043-1354(00)00452-8DREDGING-RELATED MOBILISATION OF TRACE METALS:A CASE STUDY IN THE NETHERLANDSGERARD A.VAN DEN BERG 1*,GORGIAS G.A.MEIJERS 2,LAMBERTUS M.VANDER HEIJDT 1and JOHN J.G.ZWOLSMAN 11Ministry of Transport,Public Works and Water Management,Institute for Inland Water Management and Waste Water Treatment (RIZA),Van Leeuwenhoekweg 20,3316AV Dordrecht,The Netherlandsand 2Meijers Bodemadvies,Brederostraat 75,8023AP Zwolle,The Netherlands(First received 22August 1999;accepted in revised form 13September 2000)Abstract }Mobilisation of contaminants is an important issue in environmental risk assessment of dredging projects.This study has aimed at identifying the effects of dredging on mobilisation of trace metals (Zn,Cu,Cd and Pb).The intensities and time scales of trace metal mobilisation were investigated during an experimental dredging project conducted under field conditions.The loss of contaminated dredge spoil is mainly reflected by increasing levels of trace metals in the suspended matter;dissolved trace metal concentrations in the water column are not significantly influenced by the dredging activities.This indicates a strong binding mechanism of trace metals to the solid phase or a fast redistribution over sorptive phases in response to oxidation of e.g.trace metal sulphides.Given the differences in levels of reactive phases (Mn,Fe,sulphides and organic matter)between the riverine suspended matter and the sediments,changes in the levels of these parameters in the suspended matter upon dredging may give information on the processes influencing the behaviour of trace metals and on the potential loss of sediment during dredging operations.Therefore,we recommend to routinely measure these parameters in studies on contaminant behaviour related to dredging activities.#2001Elsevier Science Ltd.All rights reservedKey words }contaminant,dredging,dispersion,metal,sediment,suspended matterINTRODUCTIONDue to the sorptive behaviour of particulate matter,sediments act as important sinks of trace metals in aquatic environments (Calmano et al .,1996).The dynamics of sediment–water interactions should,therefore,be taken into account when examining the behaviour (i.e.mobilisation and bioavailability)of trace metals.Mobilisation is mainly controlled by physical transport (e.g.advection,turbulent mixing and diffusion),biological (bioturbation and bioirri-gation)and geochemical processes (adsorption/deso-rption and precipitation/dissolution),whereas the bioavailability of contaminants in aquatic ecosystems is generally attributed to the extent of their sorption to and (co)precipitation with the solid phase (Camp-bell,1995;Van Cappellen and Gaillard,1996).The environmental risks related to dredging of contaminated sediments,have been discussed by Goossens and Zwolsman (1996).Dredge-induced resuspension resulting from technical constraints of the dredging equipment,may result in dispersion ofbothparticulate matter and pore water in th e adjacent aquatic environment.The resulting shifts in particle–water interactions may affect the beha-viour of trace metals in the aquatic environment upon dredging;depending on the physico-chemical characteristics of the dredged sediment,trace metal levels in the water column (both dissolved and particulate concentrations)may potentially increase.It should be taken into account that most trace metals in sediments are present in the particulate phase;in contrast to the water column,only a relatively small amount of trace metals (50.1%)is generally present in the dissolved phase of the sediment (Van den Berg,1998).Therefore,dispersion of trace metals in the water column during dredging activities takes place predominantly by the particu-late phase and the direct contribution of the pore water to the overall trace metal mobilisation is probably negligible.A potential increase in dissolved concentrations of trace metals in the surface water in response to dredging is,therefore,primarily related to environ-mental conditions promoting the shift of trace metals from the particulate state to the dissolved state,e.g.by oxidation of reduced phases (Fo rstner et al .,1994;Morse,1995;Fo rstner and Calmano,1998).Thus,it*Author to whom all correspondence should be addressed.Tel.:+31-78-6322637;fax:+31-78-6315003;e-mail:g.vdberg@riza.rws.minvenw.nl1979may be concluded that the actual ecotoxicological risks depend primarily on changes in environmental conditions(Luoma,1995;Calmano et al.,1993). Several studies have addressed the effects of oxida-tion of reduced sediment phases(especially sulphides) on mobilisation and bioavailability of trace metals (Tramontano and Bohlen,1984).Laboratory-based studies generally indicate a release of trace metals to the water phase during oxidation(e.g.Petersen et al., 1997;Simpson et al.,1998).The purpose of this study was to identify the effects of dredging operations on trace metal mobilisation (bothsolid and dissolved ph ase).Data were collected during a large-scale experimental dredging project conducted underfield conditions(pilot project Remediation Spijkerboor).The results of this study provide quantitative information about the intensi-ties and time scales of dredging-related mobilisation of trace metals.MATERIALS AND METHODSStudy areaThe study area(Spijkerboor)is located in the southern part of the Biesbosch,an ecologically interesting freshwater tidal marsh area in the lower delta of the rivers Rhine and Meuse in The Netherlands(see Fig.1).This is an active sedimentation area withnet deposition offine-grained sediments.Before closure of the Haringvliet basin by sluices in1970,suspended matter(SPM)was transported mainly to the North Sea.In the tidal gullies in the Biesbosch,primarily accretion of sandy material took place during this period. After implementation of the Delta Project,the civil engineering project to protect the Dutch coastline and delta againstflooding,tidalfluctuations in the lower delta of the rivers Rhine and Meuse decreased considerably.The tidal range in the southern part of the Biesbosch was decreased from approximately2to0.2–0.3m.This invoked large-scale sedimentation of mainly Meuse-derived suspended matter in the former tidal gullies in this area and erosion of dry shoals and mudflats.Recent,mainlyfine-grained,sediments in the area are strongly contaminated,reflecting the serious metal pollution history of the river Meuse.Description of the pilot projectThe presence of contaminated sediments in the lower delta of the rivers Rhine and Meuse in The Netherlands poses a large potential ecotoxicological risk to the aquatic environment.The observed negative effects of contaminated sediments on this ecosystem(e.g.Den Besten et al.,1995) have revealed the need for environmental dredging. Spijkerboor,a former tidal gully in the Southern part of the Biesbosch,was chosen as a location for a pilot project.It is characterised as a semi-stagnant sedimentation basin with typicalflow rates lower than0.1m/s during average discharge of the river Meuse.The general purpose of the pilot project,carried out during the period August1995–February1996,was to study the physical,technical and logistic possibilities of environmental dredging and to investigate and quantify the effects of a large dredging operation on the physical and biological environment.The results of the pilot project in Spijkerboor and the monitor-ing program were expected to be indicative for alarge-scale Fig.1.Map of the study area(Spijkerboor)and the sampling locations.The experimental dredging site isindicated by dotted lines.The smallfigure shows the location of the study area in The Netherlands.Gerard A.van den Berg et al.1980restoration of the contaminated sediments in the southern part of the Biesbosch and to provide valuable information about the effects of dredging on the surrounding aquatic environment(see e.g.Flipsen et al.,1998).The boundary between layers offine-grained(muds)and coarse-grained deposits(sands)indicates the change in hydrological regime by the closure of the delta.It can serve as a visually recognisable depthto discriminate between contaminated and relatively uncontaminated material in the study area.During the dredging project,thefine-grained sediments were removed to a maximum depthof6m below Dutchsea level.During th e experiment,a total amount of 110.000m3of sludge was removed in an area of approxi-mately96.000m2using hydraulic cranes.This implies that a layer of sediment with a mean thickness of nearly1m has been removed.Sampling and analysisBefore and during the dredging experiment,suspended matter and surface water samples were taken at two locations in Spijkerboor(see Fig.1).Location A is situated close to the dredging site;location C is situated approxi-mately1100m north of the dredging site.During the experiment,samples were also taken at location E,situated approximately250m north of the dredging site.After the dredging experiment samples were collected at location N, situated near location A(in the framework of the monitoring program of the pilot project Remediation Spijkerboor).Surface water was sampled at half water depth.Samples for measuring,e.g.nutrients werefiltered on board with 0.45m m cellulose acetate membranefilters(Versapor)in stainless-steelfilter holders and stored at48C until analysis. Samples for dissolved trace metal analyses werefiltered through0.45m m cellulose nitratefilters(Sartorius)in Teflon filter holders and acidified to pH2with1M HNO3on board(filtration blocks,filters,storage containers,etc.,were acid cleaned before use withdiluted HNO3to assure metal-free conditions).Samples for chlorophyll-a analysis were stored at48C until analysis withoutfiltration(analysis took place within24h after sampling;in the event analysis did not take place within this period,measurements were dismissed).In the unacidified samples,analysis of nitrate (+nitrite),ammonium,ortho-phosphate,silicate and chlor-ide,took place photometrically with aflow-through system (SKALAR autoanalyser).Chlorophyll-a was measured spectrophotometrically(Perkin Elmer lambda40).Dis-solved concentrations of Cd,Cu and Pb were measured witha graph ite-furnace atomic absorption spectrometer (GF-AAS).Because no pre-concentration step has been applied,some low dissolved concentrations of trace metals (especially Cd and Pb)may be somewhat suspicious. Dissolved Fe,Mn and Zn concentrations were measured withinductively coupled plasma atomic emission spectro-scopy(ICP-AES).Dissolved organic carbon(DOC)was measured by high-temperature(6008C)catalytic oxidation withan IR analyser(Sh imadzu TOC-5000).Measurement of pH,oxygen and temperature took place on board with automatic measuring equipment.Suspended matter was collected at the same time by continuousflow-through centrifugation(sampling took place at half water depth as well).Samples were stored frozen until extraction and analysis.After freeze drying, suspended matter samples were digested in a mixture of concentrated HCl and HNO3(aqua regia).Concentrations of major elements(e.g.Fe,Mn and S)and trace metals(Cd, Cr,Cu,Ni,Pb and Zn)in the acid solution were determined by ICP-AES(Perkin Elmer Optima3000).Grain-size distribution was determined using the micropipet method. Total organic carbon and total nitrogen were determined using a CN-analyser(Carlo Erba NA1500).The inorganic carbon content was determined after acidification of the sample withconcentrated HCl(difference measurement), and used for calculation of CaCO3content.RESULTS AND DISCUSSIONNatural variation in surface water compositionThe surface water composition in the study area (Spijkerboor)is determined mainly by the discharge of the river Meuse.Given the pluvial character of the river Meuse,the lowest discharge(and highest Cl concentrations)is measured during summer months (Table2).In the study area significant primary production took place during the productive period (May–September),as can be implicitly concluded from high concentrations of chlorophyll-a(increase up to42m g/l during cruise#3),and low concentra-tions of nitrate,ammonium,phosphate,and silicate during the cruises#1–5with respect to cruise#6 (conducted during the non-productive period).Dur-ing the productive period,pH values are somewhat higher than during the non-productive period(the pH is in the range7.5–8.1during cruises#1–5and approximately7.4during cruise#6).SuchpH values are typical for the river Meuse.Characteristics of the sediment layer and the suspended matterIn Table1,some physico-chemical characteristics of the natural suspended matter,measured prior to dredging(average concentrations during cruises#1–3),and the dredged sediment layer(i.e.thefine-grained sediments deposited since1970;t0situation) in the study area(Spijkerboor)are shown.The characteristics of the SPM prior to dredging are comparable to those in the river Meuse,as is clearly shown in Fig.2for trace metals(Zn,Cd,Cu and Pb). The sediment layer is obviously coarser grained than the SPM(2m m fraction),which can be explained by preferential settling of the coarser fractions.Due to the association of organic matter with thefiner fractions and internal production of biomass in the water column,organic matter levels in the sediment Table1.Average characteristics of the riverine suspended matter (SPM)and the dredged sediment layer(t0situation;data from Anonymous,1995)in the study location(before dredging).Allcontents are expressed as unit dry weightSPM sed.(t0) 52m m%3422 OM%168.5 Fe% 4.3 3.6a Mn%0.250.11a Cd mg/kg1219Cr mg/kg107118Cu mg/kg94118Ni mg/kg5244Pb mg/kg212266Zn mg/kg12371527a Data from Van den Berg(1998).Dredging-related mobilisation of trace metals1981are lower than those in riverine SPM.Cycling of Mn between the sediment and the water column (see discussion in Davison,1993)results in relatively low Mn levels in the sediment layer in comparison with riverine SPM.The Mn level in SPM can,therefore,be used as an indicator for mixing of riverine SPM withresuspended sediments or dredge spoil.Due to differences between kinetics of Mn and Fe reduction (and oxidation),Fe levels do not show such obvious differences.As the dredged sediment is characterised by higher levels of trace metals than the riverine SPM,loss of dredged material and subsequent mixing could result in dispersion of trace metals in the surface water.Mixing of dredge spoil with riverine suspended matter A considerable part of the dredge spoil is lost to the water column during the experiment.This results in a turbidity increase and mixing of riverine suspended matter withresuspended dredge spoil.Highturbidity (>100mg/l SPM)was measured in the direct vicinity of active dredging vessels (see Table 2,cruise #4).Typically,suchh ighturbidity is apparent only during several hours (Pennekamp and Quaak,1990).Indeed,with the exception of short-lived turbidity in the zone of impact around active dredging vessels,SPM levels only slightly increase in this study.Nevertheless,mixing of riverine suspended matter withresuspended dredge spoil is clearly indicated by a decrease in organic matter and Mn levels (and to a lesser extent by an increase in Fe levels)and an increase in trace metal levels in SPM (Table 3).Most significant changes are observed for cruise #6,indicating the largest degree of mixing (unfortu-nately,SPM levels were not measured during this cruise).During high-turbidity periods,Mn,Fe and organic matter levels are even comparable to those in the dredged sediment layer (see Table 1).Table 3shows that the grain size distribution (52m m fraction)of the SPM is largely unaffected by the dredging activities,except for the high-turbidity period.This suggests that the finer (mud)fractions are preferentially kept in solution,while the coarser fractions of the dredge spoil are deposited rapidly upon resuspension.The remaining particulate matter in the water column can potentially be dispersed into the adjacent environment.The absence of significant differences in levels of trace metals between sampling locations (Fig.2)corroborates such(rapid)disper-sion of resuspended material.As trace metalsareFig.2.Levels of Zn,Cd,Cu en Pb in suspended matter in Spijkerboor (n location A;}location C;&location E;&location N).Trace metal levels in riverine (Meuse-derived)SPM are plotted as black dots.Metal levels are measured monthly at location K (Keizersveer)in the framework of the National monitoring program water quality.The horizontal straight line represents levels of metals in the dredgedsediment layer (t 0situation;data in Table 1).Gerard A.van den Berg et al.1982Table2.Characteristics of the surface water before(cruises#1and3)and during(cruises#4–6)the dredging experiment(outliers are printed in bold)aCruise Location Time T(8C)pH O2(mg/l)NO3/NO2(mgN/l)NH4(mgN/l)PO4(m gP/l)Si(mg/l)Chfl-a(m g/l)Cl(mg/l)DOC(mg/l)Cd(m g/l)Cu(m g/l)Pb(m g/l)Zn(m g/l)Fe(m g/l)Mn(m g/l)SPM(mg/l)1A10:3023.57.568.40 3.790.051010.562547 3.370.04 5.60.435343819.1 C12:1524.27.708.60 3.570.05940.391346 3.530.01 4.60.630135023.5 A14:0024.77.759.15 3.650.031080.452848 3.540.01 6.9 6.535183521.4C15:3024.77.978.91 3.610.051000.382347 3.440.05 4.80.933155625.3 A17:0024.98.079.85 3.660.031120.442748 3.620.09 5.20.437142620.1 3A10:1024.37.668.83 3.080.091070.151856 3.220.03 3.60.6512025nd C11:5524.57.768.22 2.880.12950.201456 3.750 3.4 1.0514052nd A13:2524.47.989.25 3.050.091070.162456 3.060 3.50.3512034nd C15:0524.47.638.99 3.000.111010.172056 3.030 3.60.42163039nd A16:3524.37.729.59 3.110.081100.164256 1.090.06 4.70.2513026nd C17:2524.37.757.98 2.860.14970.221756 2.950.02 2.9 1.3513058nd 4A8:4024.27.797.45 3.080.30900.20nd64 2.890 2.90.9102051121.8 E10:1524.07.50 6.72 2.780.11860.23nd63 3.240 3.00.97303727.4 C11:4023.97.47 6.38 2.630.14960.31nd63 3.330.08 2.6 1.311204421.1 A13:1024.27.73 6.98 2.680.13860.27nd62 3.500.01 2.80.89303826.3 E14:1024.37.63 6.55 2.610.14890.31nd62 2.460.04 2.50.912204720.6 C15:5023.87.71 6.08 2.540.13950.30nd62 3.460.01 3.0 1.214204417.2 5A8:4518.27.667.60 3.190.121080.252667 3.9950.04 3.150.935203926.0 E10:3018.57.607.22 3.130.121030.302068 3.760.03 2.90.422203923.7 C11:4018.57.657.22 3.020.101050.312066 4.220.11 3.050.122203720.2 A12:5019.27.908.28 3.400.091270.303168 3.710.02 3.40.730103127.0 E15:3019.07.788.15 3.320.141100.302867 4.020 3.10.765204630.8 C15:3018.87.707.78 3.150.131050.253167 3.990 2.950.164104323.7 6A9:359.17.189.95 4.740.40204 3.83nd60 3.430.14 4.00.9nd nd nd nd E11:088.47.209.81 4.840.40203 3.75nd59 3.300.04 2.950.130160106nd C13:047.97.369.50 4.720.42183 3.92nd60 3.090 2.80.4nd nd nd nd A14:258.47.509.67 4.810.40199 3.74nd60 3.440.02 2.80.1nd nd nd nd E16:168.57.659.70 4.830.39202 3.84nd57 3.370.06 3.00.52070100nd a nd:not determined.Dredging-related mobilisation of trace metals 1983preferentially bound to the finer fractions (De Groot,1995),levels of trace metals in SPM during dredging even exceed those in the dredge spoil.After comple-tion of the dredging activities,levels of trace metals in SPM again become more or less similar to those in Meuse-derived SPM.This suggests limited time scales of dispersion of resuspended dredge spoils in the surface water.Effects of dredging on dissolved concentrations of trace metalsThe sediments in the study area are characterised by high levels of organic matter,and become anoxic within a few mm to cm below the SWI due to strong microbial activity (Van den Berg et al .,2000).Relatively high sulphide levels (up to 50m mol AVS/g dry sediment)are measured in the study area (Van den Berg et al .,1998).Given the importance of sulphides for binding of trace metals,(re)oxidation processes may significantly affect trace metal beha-viour during the dredging activities;trace metals associated with sulphides in the dredge spoil are expected to be mobilised upon dispersion in the water column.Fig.3shows that the increase in levels of trace metals in SPM during dredging,as discussed before,does not notably influence dissolved concentrations of trace metals in the water column.It should be noted,however,that the analyses of dissolved and particulate constituents are not exactly from the same water sample.Similar observations were reported by Slotton and Reuter (1995)for resuspension experi-ments with anoxic sediments.It is not clear whether this is related to a relatively slow oxidation of metal sulphides or a fast resupply of liberated trace metals over e.g.freshly formed Mn-and Fe-(hydr)oxides.These may provide an efficient new sorptive phase for trace metals present in the turbidity cloud,thereby prohibiting extensive mobilisation of trace metals to the dissolved phase (Zhuang et al .,1994).Further-more,seasonal variability in biogeochemical pro-cesses in the water column (e.g.primary production),may result in significant variation of dissolved trace metals:low dissolved concentrations of e.g.Zn during the productive period (Fig.3)may be explained by sorption to algal biomass,as has been discussed by e.g.Sigg et al .(1987).Nevertheless,after settling,contaminated material can induce benthic fluxes of trace metals to the surface water over extended periods of time (Davi-son,1985;Kerner and Geisler,1995).In the Spijkerboor,an unconsolidated mud layer withan average thickness of 0.11m was measured afterTable 3.Characteristics of the suspended matter before (cruises #1–3)and during (cruises #4–6)the dredging experiment (outliers areprinted in bold)Cruise Location Date 52m m OM Tot N CaCO 3Tot S Fe MnCd Cr Cu Ni Pb Znyymmdd (%)(%)(g/kg)(%)(%)(%)(%)(mg/kg)(mg/kg)(mg/kg)(mg/kg)(mg/kg)(mg/kg)1A9507103815.513.3 2.90.34 3.90.311110085502001200C 9507102913.711.7 5.70.31 3.90.261110086522001200A 9507103217.513.7 3.10.32 3.70.27129884481901200C 9507103515.611.8 2.50.29 4.20.241211088532001200A 9507103218.113.8 3.60.31 3.70.241192804618012002A 9507273019.017.2 2.30.31 4.60.271210597502011215C 9507274216.713.7 1.90.29 4.60.251210497502301203A 9507273116.814.3 2.50.28 4.20.22129088471891139C 9507273218.715.1 1.80.29 4.60.2712117925521312313A 9508073315.312.1 3.20.29 4.90.2614116102562351327C 9508073613.810.7 3.20.29 5.00.2314114104562481307A 9508073415.111.2 2.70.27 4.80.231311599542041274C 9508073315.312.0 3.20.29 4.40.2614119103562541345A 9508073314.810.5 3.40.28 5.00.22141151025521313104A 950825219.9 4.4 2.60.25 4.00.11169497432241233E 9508253317.615.0 2.10.30 4.80.2715117103542561322C 9508253718.416.8 1.90.31 4.50.2615110101552701256A 9508253316.913.8 2.60.30 4.750.0116117107542741353E 9508253616.726.925.50.29 4.70.2415107103532531276C 9508253618.514.4 1.20.28 4.90.26161231075727113705A 9509063414.810.6 4.30.26 4.60.2119144129622621662E 9509063513.411.1 3.40.27 4.70.2019136125602731627C 9509063514.812.5 4.90.27 4.40.2117126115582581503A 9509063314.711.2 3.30.27 4.30.1819131125572561637E 9509063613.910.2 3.10.27 4.80.1721154151672991783C 9509063715.512.1 3.70.27 5.10.23191561326827017496A 951129318.5 4.7 2.90.24 5.80.1820146126622721636E 951129369.5 5.0 3.90.26 5.70.1624173144653121929C 951129439.1 5.2 3.10.26 4.60.1427152134593291783A 9511292811.1 4.8 2.90.27 4.80.1320153129632711750E9511294015.811.95.20.265.70.1626167148623541751Gerard A.van den Berg et al.1984dredging (Quist,1996).Therefore,we conclude that during dredging an extensive removal of contami-nated sediments is needed to reduce dispersion of trace metals.CONCLUSIONSLoss of contaminated dredge spoil by resuspension during dredging activities is reflected by increased levels of Cd,Cr,Cu,Pb and Zn in the suspended matter.The manganese,iron,sulphide and organic matter contents differ significantly between the sediment sludge and riverine suspended matter.Changes in the levels of these parameters in suspended matter upon dredging do not only provide essential information on the dispersion of trace metals,but also on the potential loss of material during dredging operations.Therefore,we recommend to routinely measure these parameters during large-scale dredging of contami-nated sediments.Due to either slow oxidation kinetics of metal sulphides in the dredge spoil or a fast resupply of trace metals over freshly formed sorptive phases,no distinct differences in dissolved trace metal concen-trations in the surface water before and during dredging are measured.Changes in dissolved metal concentrations (e.g.a distinct decrease in dissolvedZn during summer)may be explained by seasonal variability in biogeochemical processes in the water column (e.g.primary production).As contaminated dredge spoil can induce benthic fluxes of trace metals to the surface water over extended periods of time after settling,we conclude that an extensive removal of contaminated sediments is needed during dredging to reduce dispersion of trace metals.Acknowledgements }The pilot project Remediation Spijker-boor was directed by the Ministry of Transport,Public Works and Water Management,Directorate Zuid-Holland.REFERENCESAnonymous.(1995)Sanering Spijkerboor;Proefproject (Remediation Spijkerboor,pilot project).Report NAOZ WP.R.95001/400,Min.Transport,Public Works and Water Management (report in Dutch).Calmano W.,Ahlf W.and Fo rstner U.(1996)Sediment quality assessment:chemical and biological approaches.In Sediments and Toxic Substances:En v ironmental Effects and Ecotoxity ,ed.W.Calmano and U.Fo rstner,pp.1–35.Springer,Berlin.Calmano W.,Hong J.and Fo rstner U.(1993)Binding and mobilization of heavy metals in contaminated sediments affected by pH and redox potential.Water Sci.Tech.28(8–9),223–235.Campbell P.G.C.(1995)Interactions between trace metals and aquatic organisms:A critique of the free-ion activity model.In Metal Speciation and Bioa v ailability inAquaticFig.3.Dissolved concentrations of Zn,Cd,Cu en Pb in the study area before and during the experiment(mean concentrations and standard deviations are presented).Dredging-related mobilisation of trace metals 1985Systems,ed.A.Tessier and D.R.Turner,pp.45–102. Wiley,New York.Davison W.(1985)Conceptual models for transport at a redox boundary.In Chemical Processes in Lakes,ed. W.Stumm,pp.31–53.Wiley,New York.Davison W.(1993)Iron and manganese in lakes.Earth-Sci. Re v.34,119–163.De Groot A.J.(1995)Metals and sediments:A global perspective.In Metal Contaminated Aquatic Sediments, ed.H.Allen E.Allen,pp.1–20.Ann Arbor Press,Ann Arbor,MI.Den Besten P.J.,Schmidt C.A.,Ohm M.,Ruys M.M.,Van Berghem J.W.and Van de Guchte C.(1995)Sediment quality assessment in the delta of rivers Rhine and Meuse based onfield observations and food chain implications. J.Aquat.Ecosystems Health4,257–270.Flipsen E.M.T.E.,Houwing E.J.,KnijffL.M.and Van den Berg G. A.(1998)Proefsanering Spijkerboor,Nieuwe Merwede;Resultaten van het abiotisch monitoringsonder-zoek(Pilot project Remediation Spijkerboor,Nieuwe Merwede:Results of the abiotic monitoring program). Report98.152x.Institute of Water Management and Waste Water Treatment,The Netherlands(report in Dutch).Fo rstner U.and Calmano W.(1998)Characterisation of dredged materials.Water Sci.Tech.38(11),149–157.Fo rstner U.,Calmano W.,Hong J.and Kersten M.(1994) Effects of redox variations on metal speciation} Implications on sediment quality criteria assessment.In Special Publication,Vol.154pp.83–102.The Royal Society of Chemistry,London.Goossens H.and Zwolsman J.J.G.(1996)An evaluation of the behaviour of pollutants during dredging activities. Terra et Aqua62,20–28.Kerner M.and Geisler C.D.(1995)Dynamics of Cu release during early aerobic degradation in aggregated seston from the Elbe estuary.Mar.Chem.51,133–144. Luoma S.E.(1995)Prediction of metal toxicity in nature from bioassays:Limitations and researchneeds.In Metal Speciation and Bioa v ailability in Aquatic Systems,ed.A. Tessier and D.R.Turner,pp.609–659.Wiley,New York. Morse J.W.(1995)Dynamics of trace metal interactions withauth igenic sulfide minerals in anoxic sediments.In Metal Contaminated Aquatic Sediments,ed.H.E.Allen, pp.187–199.Ann Arbor Press,Ann Arbor,MI. Pennekamp J.and Quaak M.(1990)Impact on the environment of turbidity caused by dredging.Terra et Aqua42,10–20.Petersen W.,Willer E.and Willamowski C.(1997) Remobilization of trace elements from polluted anoxic sediments after resuspension in oxic water.Water Air Soil Pollut.99,515–522.Quist A.(1996)Proefsanering Spijkerboor Biesbosch: Evaluatie van de civiel-technische uitvoering(Pilot project Remediation Spijkerboor Biesbosch:Evaluation of the civil-technical execution).Report USW-SPIJ-R-96034,Min.Transport,Public Works and Water Management(report in Dutch).Sigg L.,Sturm M.and Kistler D.(1987)Vertical transport of heavy metals by settling particles in Lake Zurich. Limnol.Oceano g r.32(1),112–130.Simpson S.L.,Apte S.C.and Batley G.E.(1998)Effect of short-term resuspension events on trace metal speciation in polluted anoxic sediments.En v iron.Sci.Technol.32, 620–625.Slotton D.G.and Reuter J.E.(1995)Heavy metals in intact and resuspended sediments of a California Reservoir; withemph asis on potential bioavailability of copper and zinc.Mar.Freshwater Res.46,257–265.Tramontano J.M.and Bohlen W.F.(1984)The nutrient and trace metal geochemistry of a dredge plume. Estuarine Coastal Shelf Sci.18,385–401.Van Cappellen P.and Gaillard J.F.(1996)Biogeochemical dynamics in aquatic sediments.In Reacti v e Transport in Porous Media,eds P.C.Lichtner,C.I.Steefel and E.H.Oelkers,pp.335–376.Reviews in Mineralogy, Vol.34,Mineralogical Society of America,Washington, DC.Van den Berg G.A.(1998)Geochemical behaviour of heavy metals in a sedimentation area of the rivers Rhine and Meuse.Ph.D.Thesis,Utrecht University,The Nether-lands.Van den Berg G.A.,LochJ.P.G.,Van der Heijdt L.M. and Zwolsman J.J.G.(1998)Vertical distribution of acid volatile sulfide and simultaneously extracted metals in a recent sedimentation area of the river Meuse in The Netherlands.En v iron.Toxicol.Chem.17(4), 758–763.Van den Berg G.A.,LochJ.P.G.,Van der Heijdt L.M. and Zwolsman J.J.G.(2000)Redox processes in recent sediments of the river Meuse,The Netherlands.Bio g eo-chemistry48,217–235.Zhuang Y.,Allen H.E.and Fu G.(1994)Effect of aeration of sediment on cadmium binding.En v iron.Toxicol. Chem.13(5),717–724.Gerard A.van den Berg et al. 1986。