3-Carotene triplet state absorption in the near-IR range
表面活性剂和抗氧化剂对三孢布拉霉合成β-胡萝卜素的影响
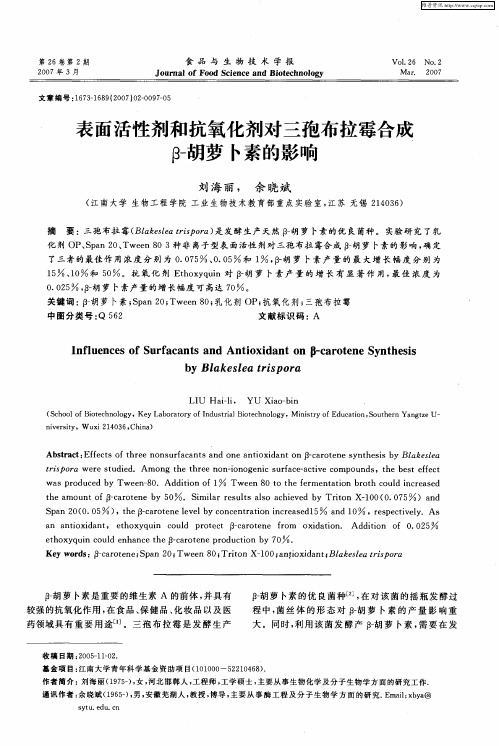
I fun e f u fcnsa dAnixd n n ̄c rtn y tei n le cso ra a t n t ia t S o o -aoe eS n h s s
b a s e r s r y Bl ke l a t i po a
1 、0 和 5 。抗 氧 化 剂 E h x q i 5 1 0/ 9 6 to y un对 p胡 萝 卜素 产 量 的 增 长 有 显 著 作 用 , 一 最佳 浓 度
0 0 5 ,一 . 2 % I胡萝 卜紊产 量的增 长 幅度 可 高达 7 。 3 0
关键词 : 一 萝 卜 ; p n2 ; e n8 ; p胡 素 S a 0 Tw e 0 乳化 剂 OP; 氧化 剂 ; 抗 三孢布拉 霉
维普资讯
第2 6卷 第 2期 2 0 年 3月 07
食 品 与 生 物 技 术 学 报
J u n lo o d S in e a d Bi tc n l g o r a fF o c e c n o e h o o y
பைடு நூலகம்
Vo . 6 No 2 12 .
M ar . 2 007
文 章 编 号 :631 8 (0 7 0 —0 70 17—6 9 2 0 )20 9—5
表 面活性剂和抗氧化剂对三孢布拉霉合成 I胡萝 卜 3 一 素的影响
刘 海 丽 余 晓斌 ’
( 江南 大学 生物 工程 学院 工 业生 物技术 教育部 重 点实验 室 , 江苏 无锡 2 4 3 ) 1 0 6 摘 要 :三孢 布拉 霉 ( lkse ip r ) 发酵 生产 天然 I胡萝 卜紊的优 良茵种 。 实验研 究 了 B a elat s oa 是 r 3 一
细胞信号转导通路
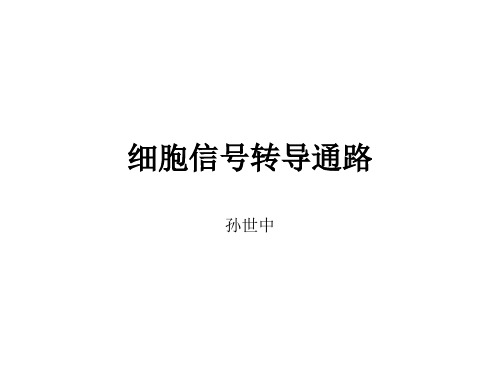
Chromatin/Epigenetics Resources
Overview of Chromatin / Epigenetics
Chromatin regulation refers to the events affecting chromatin structure and therefore, transcriptional control of gene expression patterns. Epigenetics, specifically, refers to the heritable modifications which result in altered gene expression and are not known to be encoded in DNA. The nucleosome, made up of four histone proteins (H2A, H2B, H3, and H4), is the primary building block of chromatin. Originally thought to function as a static scaffold for DNA packaging, histones have more recently been shown to be dynamic proteins, undergoing multiple types of post-translational modifications (PTMs) and interacting with regulatory proteins to control gene expression. Protein acetylation plays a crucial role in regulating chromatin structure and transcriptional activity. Histone hyperacetylation by histone acetyltransferases (HATs) is associated with transcriptional activation, whereas histone deacetylation by histone deacetylases (HDACs) is associated with transcriptional repression. Hyperacetylation can directly affect chromatin structure by neutralizing the positive charge on histone tails and disrupting nucleosome-nucleosome and nucleosomeDNA interactions. In addition, acetylation creates binding sites for bromodomain-containing chromatin regulatory proteins (histone modification readers). Unlike acetylation, methylation does not alter the charge of arginine and lysine residues and is unlikely to directly modulate nucleosomal interactions required for chromatin folding. Methylated arginine and lysine residues are major determinants for formation of active and inactive regions of the genome. Methylation facilitates binding of chromatin regulatory proteins/histone modification readers that contain various methyl-lysine or methyl-arginine binding domains (PHD, chromo, WD40, Tudor, MBT, Ankyrin repeats, PWWP domains). Recruitment of co-activator and co-repressor proteins is dependent on the specific lysine residue that is modified. The modulation of chromatin structure is an essential component in the regulation of transcriptional activation and repression. One strategy by which chromatin structure can be modulated is through disruption of histone-DNA contacts by ATP-dependent chromatin remodelers, such as the NuRD, Polycomb, and SWI/SNF complexes, which have been shown to regulate gene activation/repression, cell growth, the cell cycle, and differentiation. Chromatin structure is also modulated through other PTMs such as phosphorylation of histone proteins, which affects association with DNA-interacting proteins and has been recently identified to play a role in coordinating other histone modifications. Furthermore, methylation of DNA at cytosine residues in mammalian cells affects chromatin folding and is a heritable, epigenetic modification that is critical for proper regulation of gene silencing, genomic imprinting, and development. Three families of mammalian DNA methyl-transferases have been identified, DNMT1/2/3, that play distinct roles in embryonic stem cells and adult somatic cells. In addition to the core histone proteins, a number of histone variants exist that confer different structural properties to nucleosomes and play a number of specific functions such as DNA repair, proper kinetochore assembly and chromosome segregation during mitosis, and regulation of transcription. Chromatin and epigenetic regulation is crucial for proper programming of the genome during development and under stress conditions, as the misregulation of gene expression can lead to diseased states such as cancer.
酸性离子液体催化一三二羰基化合物与叔醇之间的反应
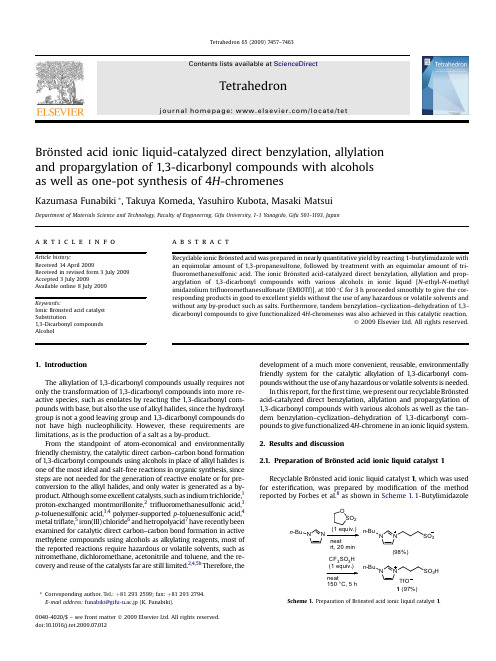
Bro¨nsted acid ionic liquid-catalyzed direct benzylation,allylation and propargylation of 1,3-dicarbonyl compounds with alcohols as well as one-pot synthesis of 4H -chromenesKazumasa Funabiki *,Takuya Komeda,Yasuhiro Kubota,Masaki MatsuiDepartment of Materials Science and Technology,Faculty of Engineering,Gifu University,1-1Yanagido,Gifu 501-1193,Japana r t i c l e i n f oArticle history:Received 14April 2009Received in revised form 3July 2009Accepted 3July 2009Available online 8July 2009Keywords:Ionic Bro¨nsted acid catalyst Substitution1,3-Dicarbonyl compounds Alcohola b s t r a c tRecyclable ionic Bro¨nsted acid was prepared in nearly quantitative yield by reacting 1-butylimidazole with an equimolar amount of 1,3-propanesultone,followed by treatment with an equimolar amount of tri-fluoromethanesulfonic acid.The ionic Bro¨nsted acid-catalyzed direct benzylation,allylation and prop-argylation of 1,3-dicarbonyl compounds with various alcohols in ionic liquid [N -ethyl-N -methyl imidazolium trifluoromethanesulfonate (EMIOTf)],at 100 C for 3h proceeded smoothly to give the cor-responding products in good to excellent yields without the use of any hazardous or volatile solvents and without any by-product such as salts.Furthermore,tandem benzylation–cyclization–dehydration of 1,3-dicarbonyl compounds to give functionalized 4H -chromenes was also achieved in this catalytic reaction.Ó2009Elsevier Ltd.All rights reserved.1.IntroductionThe alkylation of 1,3-dicarbonyl compounds usually requires not only the transformation of 1,3-dicarbonyl compounds into more re-active species,such as enolates by reacting the 1,3-dicarbonyl com-pounds with base,but also the use of alkyl halides,since the hydroxyl group is not a good leaving group and 1,3-dicarbonyl compounds do not have high nucleophilicity.However,these requirements are limitations,as is the production of a salt as a by-product.From the standpoint of atom-economical and environmentally friendly chemistry,the catalytic direct carbon–carbon bond formation of 1,3-dicarbonyl compounds using alcohols in place of alkyl halides is one of the most ideal and salt-free reactions in organic synthesis,since steps are not needed for the generation of reactive enolate or for pre-conversion to the alkyl halides,and only water is generated as a by-product.Although some excellent catalysts,such as indium trichloride,1proton-exchanged montmorillonite,2trifluoromethanesulfonic acid,3p -toluenesulfonic acid,3,4polymer-supported p -toluenesulfonic acid,4metal triflate,5iron(III)chloride 6and hetropolyacid 7have recently been examined for catalytic direct carbon–carbon bond formation in active methylene compounds using alcohols as alkylating reagents,most of the reported reactions require hazardous or volatile solvents,such as nitromethane,dichloromethane,acetonitrile and toluene,and the re-covery and reuse of the catalysts far are still limited.2,4,5b Therefore,thedevelopment of a much more convenient,reusable,environmentally friendly system for the catalytic alkylation of 1,3-dicarbonyl com-pounds without the use of any hazardous or volatile solvents is needed.In this report,for the first time,we present our recyclable Bro¨nsted acid-catalyzed direct benzylation,allylation and propargylation of 1,3-dicarbonyl compounds with various alcohols as well as the tan-dem benzylation–cyclization–dehydration of 1,3-dicarbonyl com-pounds to give functionalized 4H -chromene in an ionic liquid system.2.Results and discussion2.1.Preparation of Bro¨nsted acid ionic liquid catalyst 1Recyclable Bro¨nsted acid ionic liquid catalyst 1,which was used for esterification,was prepared by modification of the method reported by Forbes et al.8as shown in Scheme 1.1-ButylimidazoleNNn -Bu SO 2O TfO 150°C,5hNN 3H n -Bu rt,20min 1(97%)CF 3SO 3H (1equiv.)NN SO 3n-Bu(98%)Scheme 1.Preparation of Bro¨nsted acid ionic liquid catalyst 1.*Corresponding author.Tel.:þ812932599;fax:þ812932794.E-mail address:funabiki@gifu-u.ac.jp (K.Funabiki).0040-4020/$–see front matter Ó2009Elsevier Ltd.All rights reserved.doi:10.1016/j.tet.2009.07.012Tetrahedron 65(2009)7457–7463Contents lists available at ScienceDirectTetrahedronjournal homepage:www.elsevie /locate/tetwas allowed to react with an equimolar amount of 1,3-propane-sultone at room temperature to produce zwitterionic imidazolium salt in quantitative yield.Treatment of this zwitterionic imidazo-lium salt with an equimolar amount of trifluoromethanesulfonicacid at 150 C gave the Bro¨nsted acid ionic liquid catalyst 1in quantitative yield.2.2.Bro¨nsted acid ionic liquid 1-catalyzed direct benzylation,allylation and propargylation of 1,3-dicarbonyl compounds 2with alcohols 3The reaction of 2,4-pentanedione (2a )with 1-phenylethanol (3a )was conducted in the presence of 5mol %of the prepared Bro¨nsted acid ionic liquid catalyst 1in a commercially available ionic liquid,N -ethyl-N -methyl imidazolium trifluoromethanesulfonate (EMIOTf),at 100 C for 3h.After the mixture was allowed to cool to room temperature,repeated extraction with a mixed solvent of diethyl ether and hexane (v/v ¼1:1)from EMIOTf,evaporation under vacuum,and chromatography with silica gel gave 3-(1-phenyl-ethyl)pentane-2,4-dione (4aa )in 77%yield,together with a small amount (7%)of (E )-but-1-ene-1,3-diyldibenzene (5)(styrene dim-mer)(Table 1,entry 1).Other ionic liquids carrying other counter anions,such as N -butyl-N -methylimidazolium tetrafluoroborate (BMIBF 4)and N -butyl-N -methylimidazolium hexafluorophosphite (BMIPF 6),were used (entries 2and 3).As a result,in the case of BMIBF 4,only trace amount of the product 4aa was formed,and 1,10-oxybis-(ethane-1,1-diyl)dibenzene (bis(1-phenylethyl)ether)was obtained as a main product (33%yield,dr ¼50:50)(entry 2).The reaction in BMIPF 6proceeded smoothly to give 4aa in 94%yield (entry 3).Surprisingly,when the ionic liquid catalyst 1was not added,the reaction of diketone 2a with alcohol 3a also proceeded to give the corresponding product 4aa in lower yield (61%),to-gether with styrene dimmer 5(20%yield)(entry 4).9The use of an equimolar amount of diketone 2a resulted in significant decrease of the yield (39%)of 4aa (entry 5).Employing trifluoromethansulfonicacid in place of the Bro¨nsted acid ionic liquid catalyst 1gave the similar yield (74%)of 4aa ,together with styrene dimmer 5(8%)as well as 4-phenylpentan-2-one (20%)(entry 6).The results of the Bro¨nsted acid ionic liquid 1-catalyzed reaction of 1,3-diketones 2,such as 2,4-pentanedione (2a )and 1,3-diphenyl-propane-1,3-dione (2b ),with various alcohols,such as 1-phenyl-ethanol (3a ),diphenylmethanol (3b ),(E )-1,3-diphenylprop-2-en-1-ol (3c ),(E )-pent-3-en-2-ol (3d ),and 1,3-diphenylprop-2-yn-1-ol (3e )in EMIOTf,are summarized in Table 1.In the case of diphenylmethanol (3b ),the reaction also proceeded smoothly to give 3-benzhy-drylpentane-2,4-dione (4ab )in 94%yield (entry 7).Allylation and propargylation using (E )-1,3-diphenylprop-2-en-1-ol (3c ),(E )-pent-3-en-2-ol (3d )and 1,3-diphenylprop-2-yn-1-ol (3e )also proceeded to give the corresponding allylated and propargylated diketones 4ac ,4ad and 4ae in 47–90%yields (entries 8–10).1,3-Diphenylpropane-1,3-dione (2b )participated well in the reaction with 1-phenylethanol (3a )and diphenylmethanol (3b )to produce the corresponding ben-zylated diketones 4ba and 4bb in 81–98%yields,without formation of the styrene dimer 5(entries 11and 12).The results of the Bro¨nsted acid ionic liquid-catalyzed reactions of ethyl 3-oxobutanoate (2c ),ethyl 3-oxopentanoate (2d )and ethyl 3-oxo-3-phenylpropanoate (2e )with various alcohols are sum-marized in Table 2.The use of various alcohols 3a –c ,e gave the corresponding benzylated,allylated and propargylated products 4cb ,4cc and 4ce in 76–91%yields (entries 2–4).However,the re-action of 2c with 1-phenylethanol (3a )gave the corresponding ketoester 4ca in 30%yield,together with a moderate amount (40%)of styrene dimer 5,probably due to lower nucleophilicity of ketoester 2c than those of diketones 2a ,b .Other ketoesters 2d ,e also participated well in the catalytic reaction with alcohol 3b to give the corresponding products 4db and 4eb in 89–97%yields (entries 5and 6).Diastereoselectivities of the products 4ca ,4cc and 4ce are quite low.To confirm the reaction mechanism for the formation of styrenedimer 5,the Bro¨nsted acid ionic liquid-catalyzed reaction of 1-phenylethanol (3a )in EMIOTf in the absence of 1,3-dicarbonyl compound 2was carried out,as shown in Scheme 2.Table 1Bro¨nsted acid ionic liquid 1-catalyzed direct benzylation,allylation and prop-argylation of 1,3-diketones 2a ,b with various alcohols 3EMIOTf,100°C,3h1(5mol%)R 34OH R 1R 2O O +32R 1R 2O O R 4R 343a R 3=Ph,R 4=Me 3b R 3=R 4=Ph3c R 3=(E )-PhCH=CH,R 4=Ph 3d R 3=(E )-MeCH=CH,R 4=Me 3e R 3=PhC C,R 4=Ph 2a R 1=R 2=Me 2b R 1=R 2=PhNNEt TfO EMIOTfNNn -Bu BMIBF 4BF 4NNn -Bu BMIPF 6PF 6Yields of isolated products.Values in parentheses show the yields of styrene dimer 5.bBMIBF 4was used in place of EMIOTf.1,10-Oxybis (ethane-1,1-diyl)dibenzene was obtained in 33%yield.cBMIPF 6was used in place of EMIOTf.dIL catalyst 1was not added.eAn eqimolar amount of 2a was used.fTrifluoromethanesulfonic acid was used in place of IL catalyst 1.4-Phenylpentan-2-one was also obtained in 20%yield.PhPh5K.Funabiki et al./Tetrahedron 65(2009)7457–74637458As a result,styrene dimer 5was formed as a sole product in 50%yield.This result can be explained by the following mechanism:(1)protonation of the hydroxyl group of alcohol 3a and successive dehydration produces the benzyl cation,and subsequent deproto-nation gives styrene.(2)The obtained styrene attacks the other benzyl cation,and deprotonation at the b -carbon gives styrene dimer 5.After having successfully developed an efficient benzylation of 1,3-diketones 2a ,b and ketoesters 2c ,d ,e ,we then sought to apply this methodology to the synthesis of highly functionalized 4H -chromene 10via catalytic tandem benzylation,cyclization and de-hydration of the 2-(hydroxy(phenyl)methyl)phenol (3f ),prepared from salicylaldehyde and phenyllithium,as described in Table 3.This catalytic tandem reaction of 3f with diketones 2a ,b and ketoesters 2c ,d ,e proceeded smoothly to produce the corresponding 4H -chromenes,such as 1-(2-methyl-4-phenyl-4H -chromen-3-yl)-ethanone (6af ),(2,4-diphenyl-4H -chromen-3-yl)(phenyl)methanone (6bf ),ethyl 2-methyl-4-phenyl-4H -chromene-3-carboxylate (6cf ),ethyl 2-ethyl-4-phenyl-4H -chromene-3-carboxylate (6df )and ethyl 2,4-diphenyl-4H -chromene-3-carboxylate (6ef ),in good to excellent yields (77–98%),respectively.Furthermore,the Bro¨nsted acid ionic liquid-catalyzed reactions of 1,3-diphenylpropane-1,3-dione (2b )with an equimolar amount of a highly activated tertiary alkynol,1,1,3-triphenylprop-2-yn-1-ol (7),also proceeded smoothly to give not a propargylated product,but rather a dienyl product,1,3-diphenyl-2-(1,3,3-triphenylallyli-dene)propane-1,3-dione (8),in 66%yield,as shown in Scheme 3.Table 3Bro¨nsted acid ionic liquid 1-catalyzed tandem direct benzylation,cyclization and dehydration of 2with the alcohol 3f1(5mol%)R1R 2O O+3f2a R 1=R 2=Me 2b R 1=R 2=Ph2c R 1=Me,R 2=OEt 2d R 1=Et,R 2=OEt 2e R 1=Ph,R 2=OEtEMIOTf,100°C,3hO R 2O 6R 1R 1R 2O O OHOHOH2Yields of isolated products.Table 2Bro¨nsted acid ionic liquid 1-catalyzed direct benzylation,allylation and prop-argylation of ester 2with various alcohols 31(5mol%)R3R 4OH R1O O +32c R 1=Me 2d R 1=Et 2e R 1=PhOEtO O R 4R 343a R 3=Ph,R 4=Me 3b R 3=R 4=Ph3c R 3=(E )-PhCH=CH,R 4=Ph 3e R 3=PhC C,R 4=PhEMIOTf,100°C,3h2R 1Yields of isolated products.Values in parentheses show the yields of styrene dimer 5.bDetermined by GC.PhPhMe 5(50%)PhMeOH EMIOTf,120°C,3h1(5mol%)3a Scheme 2.Proposed reaction mechanism for the formation of 5.EMIOTf,100°C,24h1(5mol%)PhOH PhPhO O +72bPh PhO O Ph 8(66%)PhPh PhPhScheme 3.Bro¨nsted acid ionic liquid 1-catalyzed reaction of 1,3-diphenylpropane-1,3-dione (2b )with tertiary alkynol 7.K.Funabiki et al./Tetrahedron 65(2009)7457–74637459According to the previous report by Sanz et al.,3b this product could be produced by the tandem Meyer–Schuster rearrangement of tertiary alkynol 7,aldol condensation with diketone 2b and de-hydration,as shown in Scheme 4.2.3.Reuse of the Bro¨nsted acid ionic liquid catalyst 1Finally,reuse of the Bro¨nsted acid ionic liquid catalyst 1was carried out,as shown in Scheme 5.After the initial use of the catalyst 1in EMIOTf,the product 4aaand styrene dimer 5were extracted from EMIOTf three times with a mixed solvent of diethyl ether and hexane (1:1).Concentration of the mixed organic layer and purification by column chromatogra-phy gave the product 4aa with a trace amount of 5.Reuse of the catalyst in the second and third cycles gave the product 4aa in al-most the same yield along with a trace amount of 5.3.ConclusionIn conclusion,we have developed a new recyclable Bro¨nsted acid-catalyzed direct benzylation,allylation and propargylation of 1,3-dicarbonyl compounds with various alcohols in an ionic liquid,N -ethyl-N -methyl imidazolium trifluoromethanesulfonate (EMIOTf),without the use of any hazardous or volatile solvents and without any by-product such as salts.Furthermore,this method could also be applied to the tandem benzylation–cyclization–dehydration of 1,3-dicarbonyl compounds to give functionalized 4H -chromenes in good to excellent yields.4.Experimental4.1.General1H (400MHz)or 13C (100MHz)NMR spectra were measured with a JEOL a -400FT-NMR spectrometer in deuteriochloroform (CDCl 3)solution with tetramethylsilane (Me 4Si)as an internal stan-dard.Melting points were obtained on a Yanagimoto MP-S2micro melting point apparatus and are uncorrected.IR spectra were mea-sured on a SHIMADZU FT-IR 8100A spectrometer.HRMS were measured on a JEOL JMS-700mass spectrometer.LRMS were mea-sured on a JEOL JMS-K9mass spectrometer.The pure products were isolated by column chromatography using silica gel (Wakogel C-200,100–200mesh,Wako Pure Chemical Ind.,Ltd.).N -Ethyl-N -methyl imidazolium trifluoromethanesulfonate (EMIOTf)was a gift from the Central Glass Co.,Ltd.All chemicals were of reagent grade and,if necessary,purified in the usual manner prior to use.4.2.Preparation of 1-butyl-3-(3-sulfopropyl)-1H -imidazol-3-ium trifluoromethanesulfonate (1)To propanesultone (3.908g,31.97mmol)in a two-necked flask under argonwas slowly added 1-butylimidazole (4.005g,32.25mmol),and the mixture was stirred for 30min at room temperature.Repeated washing of the obtained solid with toluene (20ml Â5)and Et 2O (20ml Â5),and evaporation under vacuum at room temperature gave 3-(1-butyl-1H -imidazol-3-ium-3-yl)propane-1-sulfonate in 98%yield (7.797g).4.2.1.3-(1-Butyl-1H-imidazol-3-ium-3-yl)propane-1-sulfonateYield 98%;Mp 176.7–177.1 C;IR (KBr)1566(C ]C),1179(SO),1038(SO)cm À1;1H NMR (D 2O,400MHz)d 0.99(t,J ¼7.37Hz,3H,C H 3CH 2CH 2CH 2),1.38(sext,J ¼7.37Hz,2H,CH 3C H 2CH 2CH 2),1.88(quint,J ¼7.37Hz,2H,CH 3CH 2C H 2CH 2),2.32(quint,J ¼7.37Hz,2H,–C H 2CH 2SO 3À),2.80(t,J ¼7.37Hz,2H,–CH 2SO 3À),4.23(t,J ¼7.37Hz,2H,–C H 2N ]), 4.43(t,J ¼7.37Hz,2H,–CH 2N þ^),7.68(d,J ¼15.46Hz,1H,imidazolium-H),7.68(d,J ¼15.46Hz,1H,imidazo-lium-H),9.02(s,1H,imidazolium-H);13C NMR (D 2O,100MHz)d 24.2(s),30.4(s),36.7(s),42.8(s),58.8(s),59.3(s),61.0(s),133.9(s),134.2(s),147.0(s);HRMS found m /z 247.1112,calcd for C 10H 19N 2O 3S:M þH,247.1118.A mixture of 3-(1-butyl-1H -imidazol-3-ium-3-yl)propane-1-sulfo-nate (2.473g,10.0mmol)and trifluoromethanesulfonic acid (1.628g,10.85mmol)was heated to 150 C and stirred at the same temperature for 5h.After being allowed to cool to room temperature,the obtained ionic liquid was washed repeatedly with toluene (20ml Â5)and Et 2O (20ml Â5)to remove non-ionic residues,and dried under vacuum at room temperature to give 1-butyl-3-(3-sulfopropyl)-1H -imidazol-3-ium trifluoromethanesulfonate (1)(3.924g,99%).4.2.2.1-Butyl-3-(3-sulfopropyl)-1H-imidazol-3-ium trifluoromethanesulfonate (1)Yield 99%;IR (neat)3415(SO 3H),1566(C ]C),1227(SO),1170(SO),1030(SO)cm À1;1H NMR (D 2O,400MHz)d 0.92(t,3H,J ¼7.34Hz,Ph OH 7PhPh H +PhOHPhPh Ph -H +PhPhPh Ph O PhPhPh OO PhPhPh HO-H 2OPhPhOO Ph8PhPhPhPh O O2b Scheme 4.Proposed reaction mechanism for the formation of 8.PhOH MeMeO O +initial use 4aa (77), 5 (7)first reuse 4aa (77), 5 (7)second reuse 4aa (75), 5 (5)Product (%)aaYields of isolated productsScheme 5.Reuse of the Bro¨nsted acid ionic liquid catalyst 1.K.Funabiki et al./Tetrahedron 65(2009)7457–74637460C H3CH2CH2CH2),1.33(sext,2H,J¼7.34Hz,CH3C H2CH2CH2),1.87 (quint,J¼7.34Hz,CH3CH2C H2CH2), 2.34(quint,J¼7.34Hz,2H,–C H2CH2SO3À), 2.93(t,2H,J¼7.34Hz,–CH2SO3À), 4.22(t,2H, J¼7.34Hz,–CH2N]),4.38(t,2H,J¼7.34Hz,–CH2Nþ^),7.54(d, 1H,J¼9.42Hz,imidazolium-H),7.54(d,1H,J¼9.42Hz,imidazolium-H),8.82(s,1H,imidazolium-H);13C NMR(D2O,100MHz)d23.2(s),29.4(s),35.8(s),41.8(s),57.9(s),58.4(s),60.1(s),50.2(s),130.3(q, J¼317.9Hz),133.0(s),133.3(s),146.0(s);HRMS found m/z247.1123, calcd for C10H19N2O3S:MÀCF3SO3,247.1116.4.3.Typical procedure for the recyclable Bro¨nsted acid1-catalyzed direct carbon–carbon bond formation of1,3-dicarbonyl compounds with alcoholsA mixture of1-butyl-3-(3-sulfopropyl)-1H-imidazol-3-ium tri-fluoromethanesulfonate(1)(0.060g,0.151mmol),1-phenylethanol (3a)(0.370g,3.029mmol)and pentane-2,4-dione(2a)(1.503g, 15.01mmol)in1-ethyl-3-methyl-1H-imidazol-3-ium trifluorome-thanesulfonate(1ml)under argon was stirred at100 C for3h.The mixture was then cooled to room temperature and extracted from the ionic liquid with a mixed solvent of Et2O/hexane(1:1) (30mlÂ3).After the solvent was removed under reduced pressure, the product was purified by column chromatography on silica gel with hexane/EtOAc(20:1)to give3-(1-phenylethyl)pentane-2,4-dione(4aa)(0.478g,77%)and(E)-but-1-ene-1,3-diyldibenzene(5) (0.022g,7%).4.3.1.3-(1-Phenylethyl)pentane-2,4-dione(4aa)3aYield77%;Mp46.9–47.9 C(lit.43–45 C);R f0.38(hexane/ EtOAc¼5:1);IR(CHCl3)1697(C]O),1722(C]O)cmÀ1;1H NMR (CDCl3,400MHz)d1.16(d,J¼7.00Hz,3H,CHC H3),1.78(s,3H, COCH3),2.22(s,3H,COCH3),3.51–3.59(m,1H,C H CH3),3.99(d, J¼7.00Hz,1H,C H COCH3),7.13–7.26(m,5H,aryl H);13C NMR (CDCl3,100MHz)d21.6(s),30.4(s),30.5(s),41.1(s),77.4(s),127.7 (s),128.0(s),130.0(s),143.8(s),204.1(s),204.2(s);HRMS found m/z204.1151,calcd for C13H16O2:M,204.1154.4.3.2.(E)-But-1-ene-1,3-diyldibenzene(5)3aYield7%;R f0.38(hexane);IR(neat)1600(C]C)cmÀ1;1H NMR (CDCl3,400MHz)d1.38(d,J¼7.00Hz,3H,CHC H3),3.55(quint, J¼7.00Hz,1H,C H CH3),6.30–6.32(m,2H,2Âvinyl H),7.08–7.28(m, 10H,aryl H);13C NMR(CDCl3,100MHz)d21.4(s),42.7(s),126.3(s), 126.4(s),127.2(s),127.4(s),128.6(s),135.3(s),137.7(s),145.7(s); HRMS found m/z208.1259,calcd for C16H16:M,208.1253.4.3.3.1,10-Oxybis(ethane-1,1-diyl)dibenzeneYield33%;dr¼50:50;R f0.60(hexane/CH2Cl2¼1:1);1H NMR (CDCl3,400MHz)d1.31(d,6H,J¼6.52Hz,2ÂCHC H3),1.39(d,6H, J¼6.52Hz,2ÂCHC H3),4.18(q,2H,J¼6.52Hz,2ÂC H CH3),4.46(q, 2H,J¼6.52Hz,2ÂC H CH3),7.13–7.31(m,20H,aryl H);13C NMR (CDCl3,100MHz)d23.9(s),25.6(s),75.3(s),75.5(s),127.1(s),127.2 (s),128.0(s),128.3(s),129.1(s),129.4(s),145.0(s),145.1(s);MS(EI) m/z226(M,7.5%).4.3.4.4-Phenylpentan-2-one11Yield20%;R f0.29(hexane/CH2Cl2¼1:1);IR(neat)1716 (C]O)cmÀ1;1H NMR(CDCl3,400MHz)d1.29(d,3H,J¼7.00Hz, C H3CHPh), 2.09(s,3H,COCH3), 2.68(dd,1H,J¼7.00,16.18Hz, CH2CO), 2.78(dd,1H,J¼7.00,16.18Hz,CH2CO), 2.78(sext, J¼7.00Hz,1H,CH3C H Ph),7.20–7.35(m,5H,aryl H);13C NMR (CDCl3,100MHz)d21.9(s),30.5(s),35.3(s),51.9(s),126.2(s),126.6 (s),128.4(s),146.1(s),207.8(s);MS(EI)m/z162(M,34.3%).4.3.5.3-benzhydrylpentane-2,4-dione(4ab)3aYield94%;Mp114.9–116.1 C(lit.112–114 C);R f0.43(hexane/ CH2Cl2¼1:3);IR(CHCl3)1697(C]O),1719(C]O)cmÀ1;1H NMR (CDCl3,400MHz)d2.00(s,6H,2ÂCOCH3),4.81(d,J¼12.32Hz,1H,C H Ph),4.73(d,J¼12.32Hz,1H,C H COCH3),7.15–7.20(m,2H,aryl H),7.24–7.29(m,8H,arlyl H);13C NMR(CDCl3,100MHz)d30.5(s),52.1 (s),75.4(s),127.9(s),128.6(s),129.8(s),142.1(s),203.8(s);HRMS found m/z266.1308,calcd for C18H18O2:M,266.1307.4.3.6.(Z)-3-(1,3-Diphenylallyl)pentane-2,4-dione(4ac)1Yield90%;Mp83.0–83.8 C(lit.85 C);R f0.20(hexane/ Et2O¼5:1);IR(CHCl3)1682(C]O),1732(C]O)cmÀ1;1H NMR (CDCl3,400MHz)d1.92(s,3H,COCH3),2.25(s,3H,COCH3),4.30–4.37(m,2H,C H COCH3and CHPh),6.16–6.22(m,1H,PhCH]C H), 6.43(d,J¼15.70Hz,1H,PhC H]CH),7.20–7.33(m,10H,aryl H);13C NMR(CDCl3,100MHz)d30.4(s),30.7(s),49.8(s),127.0(s),127.9 (s),128.4(s),128.6(s),129.2(s),129.7(s),129.9(s),132.3(s),137.2 (s),140.7(s),141.9(s),203.4(s),203.5(s);HRMS found m/z 292.1475,calcd for C20H20O2:M,292.1464.4.3.7.(E)-3-(Pent-3-en-2-yl)pentane-2,4-dione(4ad)Yield47%;R f0.18(hexane/Et2O¼5:1);IR(neat)1698(C]O), 1722(C]O)cmÀ1;1H NMR(CDCl3,400MHz)d0.96(d,J¼7.19Hz, 3H,CHC H3),1.62(d,J¼7.19Hz,3H,C H3CH:CH),2.11(s,3H,COCH3), 2.19(s,3H,COCH3), 2.97(sext,J¼7.19Hz,1H,C H CH3), 3.56(d, J¼7.19Hz,1H,C H COCH3),5.19–5.25(m,1H,CH3CH]C H),5.46–5.55 (m,1H,CH3C H]CH);13C NMR(CDCl3,100MHz)d17.8(s),19.0(s), 29.5(s),30.0(s),37.7(s),75.8(s),126.4(s),132.3(s),204.0(s),204.0 (s);HRMS found m/z168.1158,calcd for C10H16O2:M,168.1151. 4.3.8.3-(1,3-Diphenylprop-2-ynyl)pentane-2,4-dione(4ae)3bYield88%;Mp95.4–96.0 C(lit.90–92 C);R f0.50(hexane/ CH2Cl2¼1:3);IR(CHCl3)1701(C]O),1733(C]O)cmÀ1;1H NMR (CDCl3,400MHz)d1.93(s,3H,COCH3),2.39(s,3H,COCH3),4.22(d, J¼10.87Hz,1H,C H Ph),4.67(d,J¼10.87Hz,1H,C H COCH3),7.25–7.42(m,10H,aryl H);13C NMR(CDCl3,100MHz)d28.7(s),31.1(s), 38.0(s),75.6(s),84.9(s),88.0(s),122.7(s),127.7(s),128.1(s),128.2 (s),128.3(s),128.9(s),131.6(s),138.2(s),201.6(s),201.6(s);HRMS found m/z290.1310,calcd for C20H18O2:M,290.1307.4.3.9.1,3-Diphenyl-2-(1-phenylethyl)propane-1,3-dione(4ba)3aYield81%;Mp126.1–126.8 C(lit.126–127 C);R f0.15(hexane/ EtOAc¼20:1);IR(KBr)1683(C]O),1733(C]O)cmÀ1;1H NMR (CDCl3,400MHz)d1.33(d,J¼7.00Hz,3H,CHC H3),4.03–4.11(m, 1H,C H Ph),5.63(d,J¼7.00Hz,1H,C H COPh),7.04(t,J¼7.35Hz,1H, aryl H),7.14(t,J¼7.35Hz,2H,aryl H),7.22–7.26(m,4H,aryl H), 7.35–7.42(m,3H,aryl H),7.52(t,J¼7.35Hz,1H,aryl H),7.73(d, J¼7.35Hz,2H,aryl H),8.02(d,J¼7.35Hz,2H,aryl H);13C NMR (CDCl3,100MHz)d20.5(s),41.5(s),65.0(s),126.9(s),128.0(s), 128.7(s),128.8(s),129.1(s),129.1(s),133.3(s),133.9(s),137.1(s), 137.4(s),144.1(s),194.9(s),195.3(s);HRMS found m/z328.1467, calcd for C23H20O2:M,328.1464.4.3.10.2-Benzhydryl-1,3-diphenylpropane-1,3-dione(4bb)12Yield98%;Mp221.6–222.3 C(lit.228.6–230.2 C);R f0.28 (hexane/CH2Cl2¼1:1);IR(KBr)1661(C]O),1683(C]O)cmÀ1;1H NMR(CDCl3,400MHz)d5.32(d,J¼11.71Hz,1H,C H COPh),6.35(d, J¼11.71Hz,1H,CHPh),7.05(t,J¼7.46Hz,2H,aryl H),7.15(t, J¼7.46Hz,4H,aryl H),7.24(s,4H,aryl H),7.33(t,J¼7.46Hz,4H,aryl H),7.47(t,J¼7.46Hz,2H,aryl H),7.83(d,J¼7.46Hz,4H,aryl H);13C NMR(CDCl3,100MHz)d52.4(s),62.3(s),126.6(s),128.3(s),128.5 (s),128.6(s),128.6(s),133.2(s),136.9(s),141.7(s),194.1(s);HRMS found m/z390.1618,calcd for C28H22O2:M,390.1621.4.3.11.Ethyl2-acetyl-3-phenylbutanoate(4ca)2bYield30%;R f0.63(hexane/EtOAc¼5:1);IR(neat)1717(C]O), 1747(C]O)cmÀ1;1H NMR(CDCl3,400MHz)d0.85(t,J¼7.10Hz, 3H,COOCH2C H3),1.16(d,J¼8.88Hz,3H,CHC H3),1.21(t,J¼7.10Hz, 3H,COOCH2C H3), 1.22(d,J¼8.88Hz,3H,CHC H3), 1.85(s,3H,K.Funabiki et al./Tetrahedron65(2009)7457–74637461COCH3),2.22(s,3H,COCH3),3.44–3.48(m,2H,PhCH),3.67(d, J¼8.88Hz,1H,C H COCH3),3.72(d,J¼8.88Hz,1H,C H COCH3),3.80(q, J¼7.10Hz,2H,COOC H2CH3),4.14(q,J¼7.10Hz,2H,COOC H2CH3), 7.11–7.21(m,10H,aryl H);13C NMR(CDCl3,100MHz)d13.7(s),14.2 (s),20.4(s),20.6(s),29.6(s),29.9(s),39.8(s),40.1(s),61.2(s),61.5 (s),67.0(s),67.6(s),76.8(s),77.1(s),77.4(s),126.8(s),126.9(s), 127.4(s),127.5(s),128.5(s),128.7(s),143.1(s),143.3(s),168.2(s), 168.6(s),202.4(s);HRMS found m/z234.1263,calcd for C14H18O3: M,234.1256.4.3.12.Ethyl2-benzhydryl-3-oxobutanoate(4cb)3aYield91%;Mp87.8–89.0 C(lit.84–86 C);R f0.38(hexane/ CH2Cl2¼1:1);IR(CHCl3)1716(C]O),1738(C]O)cmÀ1;1H NMR (CDCl3,400MHz)d1.00(t,J¼7.10Hz,3H,COOCH2C H3),2.09(s,3H, COCH3),3.98(q,J¼7.10Hz,2H,COOC H2CH3),4.52(d,J¼12.20Hz, 1H,CHPh),4.76(d,J¼12.20Hz,1H,C H COCH3),7.14–7.18(m,2H,arylH),7.23–7.30(m,8H,aryl H);13C NMR(CDCl3,100MHz)d13.4(s),29.7(s),50.5(s),61.2(s),64.9(s),126.5(s),126.6(s),127.4(s),127.5 (s),128.3(s),128.5(s),140.9(s),141.2(s),167.3(s),201.4(s);HRMS found m/z296.1419,calcd for C19H20O3:M,296.1413.Found:C, 76.91;H,6.87.C19H20O3requires C,77.00;H,6.80.4.3.13.(Z)-Ethyl2-acetyl-3,5-diphenylpent-4-enoate(4cc)7Yield76%;R f0.20(hexane/EtOAc¼20:1);IR(neat)1714(C]O), 1741(C]O)cmÀ1;1H NMR(CDCl3,400MHz)d0.93(t,J¼7.10Hz, 3H,COOCH2C H3),1.16(t,J¼7.10Hz,3H,COOCH2C H3),1.99(s,3H, COCH3),2.26(s,3H,COCH3),3.89(q,J¼7.10Hz,2H,COOC H2CH3),4.05(d,J¼10.99Hz,2H,C H COCH3), 4.08(d,J¼10.99Hz,2H,C H COCH3),4.12(q,J¼7.10Hz,2H,COOC H2CH3),4.26(t,J¼10.99Hz, 2H,CHPh),6.18–6.30(m,2H,PhCH]C H),6.39(d,J¼10.99Hz,1H, PhC H]CH),6.43(d,J¼10.99Hz,1H,PhC H]CH),7.12–7.29(m,20H, aryl H);13C NMR(CDCl3,100MHz)d13.3(s),13.7(s),29.4(s),29.5 (s),48.3(s),48.5(s),60.9(s),61.1(s),64.8(s),65.1(s),125.9 (s),125.9(s),126.6(s),126.7(s),127.1(s),127.1(s),127.5(s),127.5(s), 128.0(s),128.2(s),128.4(s),128.8(s),129.0(s),131.0(s),131.3(s), 136.2(s),136.3(s),139.7(s),139.9(s),167.1(s),167.4(s),200.9 (s),201.2(s);HRMS found m/z322.1574,calcd for C21H22O3:M, 322.1570.4.3.14.Ethyl2-acetyl-3,5-diphenylpent-4-ynoate(4ce)3bYield84%;R f0.50(hexane/EtOAc¼15:1);IR(neat)1719 (C]O),1746(C]O)cmÀ1;1H NMR(CDCl3,400MHz)d1.00(t, J¼7.06Hz,3H,COOCH2C H3),1.24(t,J¼7.06Hz,3H,COOCH2C H3), 1.97(s,3H,COCH3),2.39(s,3H,COCH3),3.95(q,J¼7.06Hz,2H, COOC H2CH3), 3.98(d,J¼10.69Hz,1H,C H COCH3), 4.04(d, J¼10.69Hz,1H,C H COCH3),4.22(q,J¼7.06Hz,2H,COOC H2CH3), 4.60(d,J¼10.69Hz,1H,CHPh),4.63(d,J¼10.69Hz,1H,CHPh), 7.20–7.42(m,20H,aryl H);13C NMR(CDCl3,100MHz)d13.8(s), 14.1(s),29.8(s),30.6(s),37.8(s),37.8(s),61.6(s),61.8(s),66.5 (s),66.8(s),84.1(s),84.7(s),88.2(s),88.5(s),122.8(s),123.1(s), 127.6(s),127.7(s),128.1(s),128.2(s),128.2(s),128.2(s),128.3 (s),128.6(s),128.7(s),131.6(s),138.2(s),138.3(s),166.8(s), 167.1(s),200.3(s),200.7(s);HRMS found m/z320.1413,calcd for C21H20O3:M,320.1415.4.3.15.Ethyl2-benzhydryl-3-oxopentanoate(4db)Yield89%;Mp87.8–88.1 C;R f0.38(hexane/CH2Cl2¼1:1);IR (KBr)1714(C]O),1747(C]O)cmÀ1;1H NMR(CDCl3,400MHz) d0.84(t,J¼7.25Hz,3H,COCH2C H3),0.97(t,J¼7.25Hz,3H, COOCH2C H3), 2.18–2.28(m,1H,COC H2CH3), 2.46–2.56(m,1H, COC H2CH3),3.90–4.01(m,2H,COOC H2CH3),4.56(d,J¼12.20Hz, 1H,CHPh),4.82(d,J¼12.20Hz,1H,C H COCH2CH3),7.11–7.32(m, 10H,aryl H);13C NMR(CDCl3,100MHz)d7.1(s),13.6(s),36.6(s), 50.7(s),61.2(s),64.0(s),126.6(s),126.7(s),127.5(s),127.7(s),128.4 (s),128.6(s),141.3(s),141.5(s),167.5(s),204.1(s);MS(EI)m/z292 (MÀH2O,19.3%).4.3.16.Ethyl2-benzhydryl-3-oxo-3-phenylpropanoate(4eb)11Yield97%;Mp137.0–137.5 C(lit.141.9–143.1 C);R f0.54(hex-ane/CH2Cl2¼1:1);IR(KBr)1682(C]O),1730(C]O)cmÀ1;1H NMR (CDCl3,400MHz)d0.93(t,J¼7.12Hz,3H,COOCH2C H3),3.85–3.99 (m,2H,COOC H2CH3),5.08(d,J¼11.83Hz,1H,CHCOPh),5.41(d, J¼11.83Hz,1H,CHPh),7.03–7.07(m,1H,arlyl H),7.12–7.30(m,7H, arlyl H),7.34–7.45(m,4H,arlyl H),7.53–7.57(m,1H,arlyl H),8.00–8.02(m,2H,arlyl H);13C NMR(CDCl3,100MHz)d13.7(s),50.9(s), 59.4(s),61.5(s),126.5(s),126.8(s),127.7(s),128.2(s),128.5(s), 128.6(s),128.6(s),128.7(s),133.5(s),136.6(s),141.7(s),167.7(s), 192.8(s);MS(EI)m/z340(MÀH2O,46.4%).4.4.Typical procedure for the recyclable Bro¨nsted acid1-catalyzed tandem direct benzylation,cyclization and dehydration of the alcohol3fA mixture of1-butyl-3-(3-sulfopropyl)-1H-imidazol-3-ium tri-fluoromethanesulfonate(1)(0.020g,0.050mmol),2-(hydroxy-(phenyl)methyl)phenol(3f)(0.199g,0.994mmol)and pentane-2,4-dione(2a)(0.503g,5.024mmol)in1-ethyl-3-methyl-1H-imidazol-3-ium trifluoromethanesulfonate(1ml)under argon was stirred at 100 C for3h.The mixture was then cooled to room temperature and extracted from the ionic liquid with a mixed solvent of Et2O/hexane (1:1)(30mlÂ3).After the solvent was removed under reduced pressure,the product was purified by column chromatography on silica gel with hexane/CH2Cl2(1:4)to give1-(2-methyl-4-phenyl-4H-chromen-3-yl)ethanone(6af)(0.204g,77%).4.4.1.1-(2-Methyl-4-phenyl-4H-chromen-3-yl)ethanone(6af)Yield77%;R f0.58(hexane/CH2Cl2¼1:4);IR(neat)1682 (C]O)cmÀ1;1H NMR(CDCl3,400MHz)d2.13(s,3H,COCH3),2.43 (s,3H,CCH3),4.99(s,1H,CHPh),6.92–6.99(m,2H,aryl H),7.06–7.14 (m,3H,aryl H),7.19–7.30(m,4H,aryl H);13C NMR(CDCl3,100MHz) d20.5(s),30.5(s),42.6(s),114.5(s),116.7(s),124.9(s),125.2(s), 127.2(s),127.9(s),128.0(s),129.3(s),129.3(s),146.2(s),149.4(s), 159.5(s),199.2(s);HRMS found m/z264.1147,calcd for C18H16O2: M,264.1151.4.4.2.(2,4-Diphenyl-4H-chromen-3-yl)(phenyl)methanone(6bf)Yield98%;Mp152.5–153.0 C;R f0.30(hexane/CH2Cl2¼2:1);IR (KBr)1643(C]O)cmÀ1;1H NMR(CDCl3,400MHz)d5.35(s,1H, CHPh),7.02–7.19(m,9H,aryl H),7.22–7.28(m,4H,aryl H),7.36–7.39 (m,2H,aryl H),7.43–7.51(m,4H,aryl H);13C NMR(CDCl3,100MHz) d43.9(s),114.4(s),116.5(s),124.6(s),126.7(s),127.6(s),127.8(s), 127.9(s),128.1(s),128.6(s),129.1(s),129.3(s),129.5(s),129.7(s), 131.7(s),133.3(s),138.4(s),145.2(s),150.3(s),155.3(s),197.2(s); HRMS found m/z388.1471,calcd for C28H20O2:M,388.1464.4.4.3.Ethyl2-methyl-4-phenyl-4H-chromene-3-carboxylate(6cf)9Yield84%;R f0.75(hexane/CH2Cl2¼1:4);IR(neat)1710 (C]O)cmÀ1;1H NMR(CDCl3,400MHz)d1.16(t,J¼7.12Hz,3H, COOCH2C H3),2.51(s,3H,CCH3),4.02–4.15(m,2H,COOC H2CH3),5.04(s,1H,CHPh),6.94–6.98(m,1H,aryl H),7.00–7.06(m,2H,arylH),7.09–7.15(m,2H,aryl H),7.20–7.24(m,4H,aryl H);13C NMR (CDCl3,100MHz)d13.6(s),19.0(s),41.0(s),59.6(s),105.6(s),115.7 (s),124.0(s),124.3(s),125.9(s),127.0(s),127.3(s),127.9(s),128.7 (s),146.2(s),148.8(s),159.5(s),166.6(s),HRMS found m/z 294.1265,calcd for C19H18O3:M,294.1256.4.4.4.Ethyl2-ethyl-4-phenyl-4H-chromene-3-carboxylate(6df)Yield80%;R f0.50(hexane/CH2Cl2¼1:1);IR(neat)1703 (C]O)cmÀ1;1H NMR(CDCl3,400MHz)d1.17(t,J¼7.30Hz,3H, CCH2C H3),1.29(t,J¼7.30Hz,3H,COOCH2C H3),2.84–3.00(m,2H, CC H2CH3),4.02–4.15(m,2H,COOC H2CH3),5.03(s,1H,CHPh),6.94–6.98(m,1H,aryl H),7.02–7.07(m,2H,aryl H),7.10–7.16(m,2H,arylH),7.21–7.23(m,4H,aryl H);13C NMR(CDCl3,100MHz)d11.9(s),K.Funabiki et al./Tetrahedron65(2009)7457–7463 7462。
众多高分文章引用的超敏小鼠总胆汁酸TBA检测试剂盒
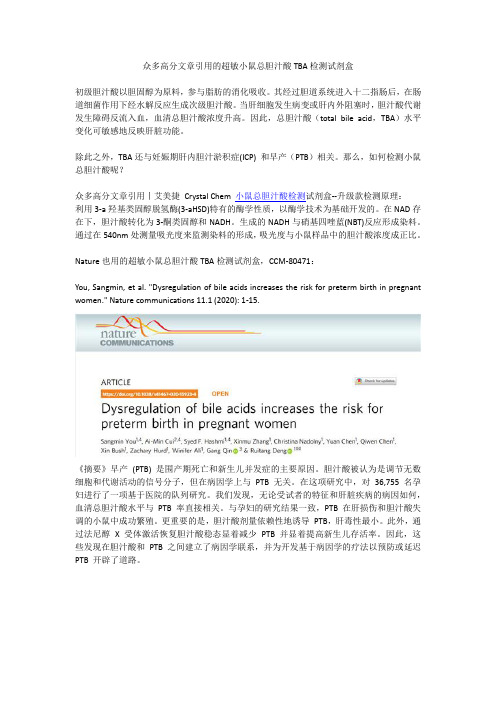
众多高分文章引用的超敏小鼠总胆汁酸TBA检测试剂盒初级胆汁酸以胆固醇为原料,参与脂肪的消化吸收。
其经过胆道系统进入十二指肠后,在肠道细菌作用下经水解反应生成次级胆汁酸。
当肝细胞发生病变或肝内外阻塞时,胆汁酸代谢发生障碍反流入血,血清总胆汁酸浓度升高。
因此,总胆汁酸(total bile acid,TBA)水平变化可敏感地反映肝脏功能。
除此之外,TBA还与妊娠期肝内胆汁淤积症(ICP) 和早产(PTB)相关。
那么,如何检测小鼠总胆汁酸呢?众多高分文章引用丨艾美捷Crystal Chem 小鼠总胆汁酸检测试剂盒--升级款检测原理:利用3-a羟基类固醇脱氢酶(3-aHSD)特有的酶学性质,以酶学技术为基础开发的。
在NAD存在下,胆汁酸转化为3-酮类固醇和NADH。
生成的NADH与硝基四唑蓝(NBT)反应形成染料。
通过在540nm处测量吸光度来监测染料的形成,吸光度与小鼠样品中的胆汁酸浓度成正比。
Nature也用的超敏小鼠总胆汁酸TBA检测试剂盒,CCM-80471:You, Sangmin, et al. "Dysregulation of bile acids increases the risk for preterm birth in pregnant women." Nature communications 11.1 (2020): 1-15.《摘要》早产(PTB) 是围产期死亡和新生儿并发症的主要原因。
胆汁酸被认为是调节无数细胞和代谢活动的信号分子,但在病因学上与PTB 无关。
在这项研究中,对36,755 名孕妇进行了一项基于医院的队列研究。
我们发现,无论受试者的特征和肝脏疾病的病因如何,血清总胆汁酸水平与PTB 率直接相关。
与孕妇的研究结果一致,PTB 在肝损伤和胆汁酸失调的小鼠中成功繁殖。
更重要的是,胆汁酸剂量依赖性地诱导PTB,肝毒性最小。
此外,通过法尼醇X 受体激活恢复胆汁酸稳态显着减少PTB 并显着提高新生儿存活率。
一株产吲哚乙酸菌的筛选、鉴定及培养条件优化

doi :10.3969/j.issn.2095⁃1736.2021.02.065一株产吲哚乙酸菌的筛选、鉴定及培养条件优化周铭典1,2,蔡冠竟1,宁静1,朱葛夫1(1.中国科学院城市环境研究所,厦门361021;2.中国科学院大学,北京100049)摘要通过平板涂布培养法和Salkowski 比色法对堆肥中具有产吲哚乙酸(IAA )能力的菌株进行初步筛选,并进一步利用分光光度法定量复筛其中具有高产IAA 能力的菌株。
试验共分离得到3株具有产IAA 能力的菌株,其中菌株29B 具有较强分泌IAA 的能力,培养96h 时IAA 产量达到54.73μg /mL 。
通过菌株形态观察、部分生理生化特性测定及16S rDNA 基因序列分析对菌株29B 进行分类鉴定,初步确定菌株29B 为停滞棒杆菌(Corynebacterium stationis )。
设计单因素试验以IAA 含量为评价指标,对菌株29B 产IAA 能力进行条件优化。
结果表明,菌株29B 最佳培养条件是初始pH 值为7.0,接种量(体积分数)为2%,35℃摇床培养108h 。
在此最优条件下,菌株29B 的IAA 产量可达138.87μg /mL ,比优化前提高153.74%。
研究结果为菌株29B 在农业生产中的应用提供了实验基础和理论依据。
关键词吲哚乙酸(IAA );筛选;条件优化;堆肥中图分类号Q939.9文献标识码A文章编号2095⁃1736(2021)02⁃0065⁃05Screening ,identification and culture condition optimization ofan indole-3-acetic acid producing bacterial strainZHOU Mingdian 1,2,C AI Guanjing 1,N ING Jing 1,Z HU Gefu 1(1.Institute of Urban Environment ,C hinese Academy of Sciences ,X iamen 361021,C hina ;2.University of Chinese Academy of Sciences ,B eijing 100049,C hina )Abstract The indole-3-acetic acid (IAA )-producing strains were preliminarily screened by using spread plate and Salkowski colori⁃metric method ,a nd quantitative analysis of spectrophotometry was used to rescreen the strain with high IAA producing capacity.Three IAA-producing strains were isolated from the compost sample ,a nd strain 29B showed the highest IAA productivity with the yield reached 54.73μg /mL in 96h of cultivation.Based on morphological observation ,p art of physio-biochemical characteristics and analysis of 16S rDNA gene sequences ,t he strain 29B was identified as Corynebacterium stationis .The single factor tests were de⁃signed to optimize the IAA production of strain 29B.Results showed that the optimum culture conditions were as follows :initial pH value ,7.0;i noculum amount (volume fraction ),2%;s haking culture at 35℃for 108h.Under the optimal culture conditions ,t he yield of IAA reached up to 138.87μg /mL ,w hich was increased by 153.74%compared with that before optimization.These results would provide an experimental and theoretical basis for the application of strain 29B in agricultural production.Key words indole-3-acetic acid (IAA );screening ;optimization ;compost吲哚乙酸(Indole-3-acetic acid ,I AA )是一种重要的植物生长激素,对植物的新陈代谢、生长发育都起着重要作用[1]。
单重态的氧──是敌是友?
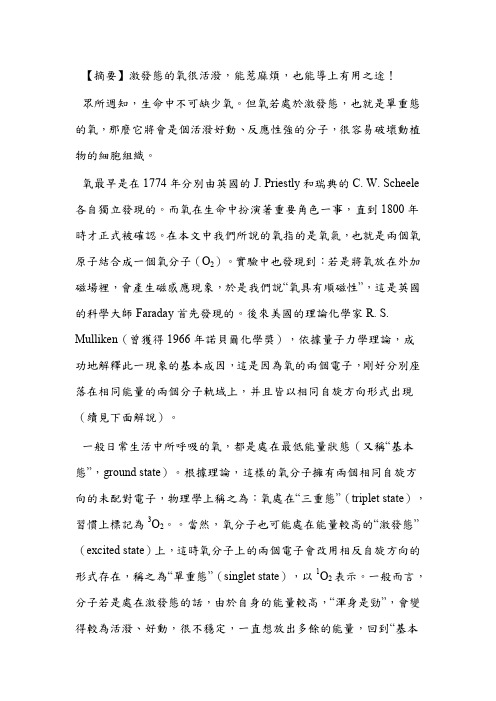
【摘要】激發態的氧很活潑,能惹麻煩,也能導上有用之途!眾所週知,生命中不可缺少氧。
但氧若處於激發態,也就是單重態的氧,那麼它將會是個活潑好動、反應性強的分子,很容易破壞動植物的細胞組織。
氧最早是在1774年分別由英國的J. Priestly和瑞典的C. W. Scheele 各自獨立發現的。
而氧在生命中扮演著重要角色一事,直到1800年時才正式被確認。
在本文中我們所說的氧指的是氧氣,也就是兩個氧原子結合成一個氧分子(O2)。
實驗中也發現到:若是將氧放在外加磁場裡,會產生磁感應現象,於是我們說“氧具有順磁性”,這是英國的科學大師Faraday首先發現的。
後來美國的理論化學家R. S. Mulliken(曾獲得1966年諾貝爾化學獎),依據量子力學理論,成功地解釋此一現象的基本成因,這是因為氧的兩個電子,剛好分別座落在相同能量的兩個分子軌域上,并且皆以相同自旋方向形式出現(續見下面解說)。
一般日常生活中所呼吸的氧,都是處在最低能量狀態(又稱“基本態”,ground state)。
根據理論,這樣的氧分子擁有兩個相同自旋方向的未配對電子,物理學上稱之為:氧處在“三重態”(triplet state),習慣上標記為3O2。
當然,氧分子也可能處在能量較高的“激發態”(excited state)上,這時氧分子上的兩個電子會改用相反自旋方向的形式存在,稱之為“單重態”(singlet state),以1O2表示。
一般而言,分子若是處在激發態的話,由於自身的能量較高,“渾身是勁”,會變得較為活潑、好動,很不穩定,一直想放出多餘的能量,回到“基本態”來。
而分子處於“激發態”時的存活時間,就稱之為“生命期”(life time)。
處在能量越高的激發態上,分子的“生命期”也就越短。
可推想而知,分子若是處於能量最低的“基本態”,那麼它的“生命期”將會是無限長,也就是非常穩定地存活著。
根據研究指出,氧分子的第一激發態和第二激發態皆屬於“單重態”,如圖一所示。
分子遗传学基因表达的调控

The structural genes of the loc operon are transcribed into a single
polycistronic mRNA ,which is traslated simulated simultaneously by several
ribosmes into the three enzmes encoded by the operon.
2.Lac操纵子表示是在Lac阻遏物负调控下进行。在没有诱导物 时,Lac阻遏物通常抑制Lac酶产生。
分子遗传学基因表达的调控
第22页
*在操纵基因中发生突变时,即使有活性阻遏物存在,Lac
酶照常合成。这种突变称为操纵基因组成型突变OC(
constitutive)。
15-6 b 分子遗传学基因表达的调控
第23页
单倍体和杂合二倍体操纵基因突变体Lac酶合成情况
基因型
O+Z+Y+ O+Z+Y+ / FO+Z—Y+ OC Z+Y+ O+Z+Y— / FOCZ+Y+ O+Z+Y+ / FOCZ—Y+ O+Z—Y+ / FOCZ+Y— IS O+Z+Y+ / FI+OC Z+Y+
β-半
None IPTG -+ -+
分子遗传学基因表达的调控
第15页
二、I 基因
控制Lac酶可诱导性区域。
I+ 细胞只能在有一个诱导物存在时才能正 常合成Lac酶;
I- 细胞不论诱导物存在是否都能合成Lac 酶,这种突变体称为组成型突变体( constitutive mutant)。
三七提取液对阿尔茨海默病模型小鼠的保护作用

ABSTRACT OBJECTIVE:To study protective effects of Panax notoginseng extracts on the senile dementia model mice. METHODS:Senile dementia model was established by intragastrical administration of aluminum gluconate. Model mice were divided into 5 groups,i.e. normal group,model group,huperzine A group and P. notoginseng high-dose and low-dose groups. They were given relevant medicine intragastrically once a day for 5 days a week for 3 months to establish senile dementia model. In step down test, ability of Passive elusion learning and memory of mice were observed,MDA content,SOD and acetylcholine shift enzyme (ChAT) activities were determined. RESULTS:Compared with model group,training times of P. notoginseng high-dose and low-dose groups reduced significantly(P<0.01 or P<0.05),and incubation period shortened significantly(P<0.01);MDA content of P. notoginseng high-dose and low-dose groups significantly reduced,SOD and ChAT activities enhanced significantly(P< 0.01).CONCLUSION:P. notoginseng can reduce MDA content,strengthen SOD and ChAT acitivities to relieve senile dementia. KEY WORDS Alzheimer’s disease;MDA;SOD;ChAT
中文翻译
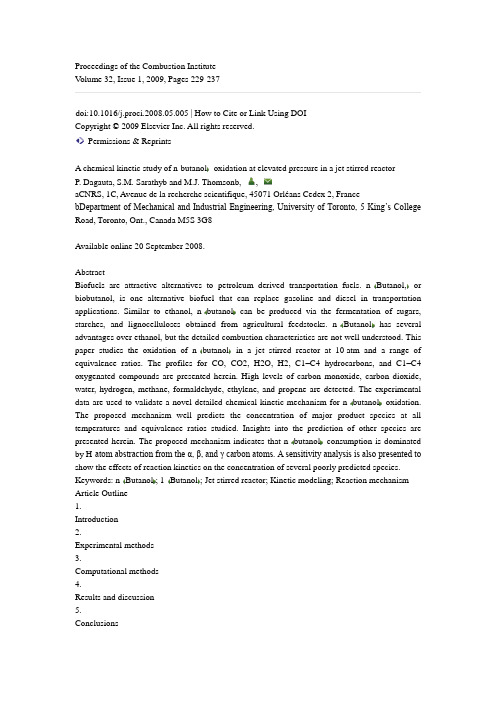
Proceedings of the Combustion InstituteV olume 32, Issue 1, 2009, Pages 229-237doi:10.1016/j.proci.2008.05.005 | How to Cite or Link Using DOICopyright © 2009 Elsevier Inc. All rights reserved.Permissions & ReprintsA chemical kinetic study of n-butanol oxidation at elevated pressure in a jet stirred reactorP. Dagauta, S.M. Sarathyb and M.J. Thomsonb, ,aCNRS, 1C, Avenue de la recherche scientifique, 45071 Orléans Cedex 2, France bDepartment of Mechanical and Industrial Engineering, University of Toronto, 5 King’s College Road, Toronto, Ont., Canada M5S 3G8Available online 20 September 2008.AbstractBiofuels are attractive alternatives to petroleum derived transportation fuels. n-Butanol,or biobutanol, is one alternative biofuel that can replace gasoline and diesel in transportation applications. Similar to ethanol, n-butanol can be produced via the fermentation of sugars, starches, and lignocelluloses obtained from agricultural feedstocks. n-Butanol has several advantages over ethanol, but the detailed combustion characteristics are not well understood. This paper studies the oxidation of n-butanol in a jet stirred reactor at 10 atm and a range of equivalence ratios. The profiles for CO, CO2, H2O, H2, C1–C4 hydrocarbons, and C1–C4 oxygenated compounds are presented herein. High levels of carbon monoxide, carbon dioxide, water, hydrogen, methane, formaldehyde, ethylene, and propene are detected. The experimental data are used to validate a novel detailed chemical kinetic mechanism for n-butanol oxidation. The proposed mechanism well predicts the concentration of major product species at all temperatures and equivalence ratios studied. Insights into the prediction of other species are presented herein. The proposed mechanism indicates that n-butanol consumption is dominated by H-atom abstraction from the α, β, and γ carbon atoms. A sensitivity analysis is also presented to show the effects of reaction kinetics on the concentration of several poorly predicted species. Keywords: n-Butanol; 1-Butanol; Jet stirred reactor; Kinetic modeling; Reaction mechanism Article Outline1.Introduction2.Experimental methods3.Computational methods4.Results and discussion5.ConclusionsAcknowledgementsAppendix A.Supplementary dataReferences1. IntroductionA potential biofuel for use in both gasoline and diesel engines is n-butanol.Historically, industrial scale production of n-butanol from biomass feedstocks was the second largest fermentation process, exceeded only by ethanol. However, its demise was brought about in the early 1960s when petroleum derived n-butanol became more economically feasible [1]. Recent advances in n-butanol production in the laboratory have spurred interest in commercial scale production of the n-butanol[2] and [3]. Recently, BP and Dupont announced that they would commercially produce n-butanol,which they call biobutanol, as a gasoline blending component for automotive fuels [4] and [5]. n-Butanol is produced via a fermentation process similar to that of ethanol, and therefore its feedstocks could include sugar beet, sugar cane, corn, wheat and also cellulosic biomass. n-Butanol has several advantages over ethanol including enhanced tolerance to water contamination allowing the use of existing distribution pipelines, the ability to blend at higher concentrations without retrofitting vehicles, and better fuel economy.Relatively few engine studies of n-butanol have been published. Yacoub et al. used gasoline blended with a range of C1–C5 alcohols (including n-butanol)to fuel a single-cylinder spark ignition (SI) engine [6]. They found that the n-butanol blends had less knock resistance than neat gasoline. The n-butanol blends also had reduced CO and hydrocarbon emissions but increased NOx emissions. This may be due to the n-butanol blends having a higher flame temperature and earlier spark timing. Of particular interest to the present study is that the primary oxygenated hydrocarbon emissions were n-butanol,formaldehyde and to a lesser extent, acetaldehyde. A study by Miller et al. successfully operated unmodified gasoline and diesel engines on blends containing 0–20% n-butanol in gasoline and 0–40% n-butanol in diesel fuel [7]. Another study successfully ran a compression ignition (CI) engine fueled with n-butanol and diesel fuel microemulsions [8].Predictive models provide a better understanding of the combustion performance and emissions characteristics of biofuel compositions and why they differ from petroleum derived materials. The development of an n-butanol model requires understanding of its fundamental pyrolysis and oxidation kinetics. However, few studies have examined the combustion chemistry of n-butanol, while none have developed a detailed chemical kinetic mechanism of the fuel. A 1959 study by Barnard examined the pyrolysis of n-butanol[9]. The experiments were carried out in a static reactor at temperatures between 579 and 629 °C. Barnard suggested that, in the absence of oxygen, n-butanol primarily reacts by the fission of the molecule at the C3H7–CH2OH bond. This produces formaldehyde, ethylene and a methyl radical, following the decomposition of the n-propyl radical. Barnard also conducted a similar study of t-butanol[10]. A study by Roberts measured the burning velocities of n-butanol using schlieren photographs of the flames [11], and found that the maximum burning velocity of n-butanol is similar to that of isopropyl alcohol and isopentyl alcohol. A recent study by McEnally and Pfefferle [12] measured the temperature and species in an atmospheric-pressure coflowing laminar nonpremixed flames. The fuels consisted of methane doped with one of the four isomers of butanol.They claimed that unimolecular dissociation was dominant, not H-atom abstraction. For n-butanol,this consisted of C–C fissionfollowed by β scission of the resulting radicals. In the case of n-butanol,complex fission involving four-center elimination of water was estimated to account for only 1% of n-butanol decomposition. The most important measured species included ethylene (C2H4) and propene (C3H6). More recently, Yang and co-workers [13] studied laminar premixed flames fuelled by one of four isomers of butanol(including n-butanol). Their results identify combustion intermediates in the butanol flames, but do not provide concentration profiles. The qualitative data provided lends support to the aforementioned dissociation mechanism proposed by McEnally and Pfefferle [12].In this paper, we report new experimental data obtained in a jet stirred reactor (JSR) for the oxidation of n-butanol at a pressure of 10 atm and a range of equivalence ratios (0.5–2.0) and temperatures (800–1150 K). In addition, a chemical kinetic model of n-butanol is developed using the JSR experiments as validation data. Both experimental and kinetic insights are offered below.2. Experimental methodsThe JSR experimental setup used in this study has been described earlier [14] and [15]. The JSR consists of a small sphere of 4 cm diameter (39 cm3) made of fused silica (to minimize wall catalytic reactions), equipped with four nozzles of 1 mm i.d. for the admission of the gases which achieve stirring. The reactants were diluted by high-purity nitrogen (<50 ppm O2, <1000 ppm Ar, <5 ppm H2) and mixed at the entrance of the injectors. A high degree of dilution (0.1% volume of fuel) was used, reducing temperature gradients and heat release in the JSR. High-purity oxygen (99.995% pure) was used in these experiments. All the gases were preheated before injection to minimize temperature gradients inside the reactor. A regulated heating wire of ca. 1.5 kW maintained the temperature of the reactor at the desired working temperature. The n-butanol was sonically degassed before use. A Shimadzu LC10 AD VP pump with an on-line degasser (Shimadzu DGU-20 A3) was used to deliver the fuel to an atomizer–vaporizer assembly maintained at 200 °C. Good thermal homogeneity along the vertical axis of the reactor (gradients of ca. 1 K/cm) was observed for each experiment by thermo-couple (0.1 mm Pt–Pt/Rh (10%) located inside a thin-wall silica tube) measurements. The reacting mixtures were probe sampled by means of a fused silica low pressure sonic probe. The samples were analyzed online by FT-IR and off-line after collection and storage in 1 L Pyrex bulbs. Off-line analysis was done using gas chromatographs equipped with capillary columns (DB-624 and Carboplot-P7), a TCD (thermal conductivity detector), and an FID (flame ionization detector).The experiments were performed at steady state, at a constant mean residence time of 0.7 s and a constant pressure of 10 atm. The reactants were continually flowing in the reactor while the temperature of the gases inside the JSR was increased stepwise. A good repeatability was observed in the experiments and reasonable good carbon balance of 100 ± 15% was achieved.3. Computational methodsThe kinetic modeling was performed using the PSR computer code [16] that computes species concentrations from the net rate of production of each species by chemical reactions and the difference between the input and output flow rates of the species. These rates are computed from the kinetic reaction mechanism and the rate constants of the elementary reactions calculated at the experimental temperature.The reaction mechanism used here is based on a previously proposed oxidation mechanism [17], [18] and [19] for C1–C4 chemistry. Additional reactions have been added to represent thebutanol mechanism and are listed in Table 1. The oxidation of n-butanol proceeds via unimolecular initiation and hydrogen abstraction reactions. The fuel radical species formed are consumed via unimolecular decomposition (β-scission) and biomolecular reactions. Isomerization of radical species is also included in the proposed model. Table 2 presents the structure of species produced during the oxidation of n-butanol.The rate expression for new reactions derives from tabulations for alkanes and alcohols [18] and [19]. This mechanism, including references and thermochemical data, is available as Supplementary material to this article. The rate constants for reverse reactions are computed from the corresponding forward rate constants and the appropriate equilibrium constants, calculated from thermochemistry [20] and [21].Table 1. Reactions representing the oxidation of n-butanolFull-size tableNote: X denotes a radical species (OH, H, CH3, O, HCO, HO2, CH2OH, CH3O, C2H5, C2H4, C4H7, aC3H5).View Within ArticleaC4H8OHbC4H8OHcC4H8OHdC4H8OHcC3H6OHaC3H6OHFull-size tableView Within Article4. Results and discussionMolecular species concentration profiles were measured by sonic probe sampling and GC and FT-IR analyses from the oxidation of n-butanol in a JSR: hydrogen (H2), water (H2O), carbon monoxide (CO), carbon dioxide (CO2), methane (CH4), acetylene (C2H2), ethylene (C2H4), ethane (C2H6), propene (C3H6), 1-butene (C4H8), acetaldehyde (CH3HCO), formaldehyde (CH2O), butyraldehyde (C3H7CHO), and n-butanol(C4H9OH). Figure 1 presents the experimental measurements and modeling results of n-butanol obtained at = 1.0. Theexperimental results (solid symbols) show that with increasing temperature, the n-butanol levels drop significantly between 800 and 900 K. This corresponds to a large increase in the concentrations of butyraldehyde, 1-butene, and propene, all of which are products of H abstraction pathways. The concentration of these compounds then quickly decreases as the temperature increases. Ethylene, ethane, acetaldehyde, and formaldehyde concentrations are also shown to increase between 800 and 900 K. However, as the temperature increases further, the concentrations of these species tends to diminish at a slower rate than the aforementioned species.Full-size image (63K)Fig. 1. Comparison of the experimental concentration profiles obtained from the oxidation of n-butanol in a JSR at = 1, P = 10 atm, τ = 0.7 s.View Within ArticleThe following oxygenated products were detected: butanal, ethyloxirane, propanal, 2-propenal, methyloxirane, oxirane, and acetaldehyde. The oxiranes, 2-propenal, and propanal are formed at low ppm levels, and therefore no concentration profiles are reported. Enols were not detected. A comparison with results obtained for ethanol in similar conditions and keeping the initial carbon content shows butanol oxidation produces less aldehydes overall. The maximum amount ofacetaldehyde production is reduced by ca. 70% when changing the fuel from ethanol to butanol. The model predictions (open symbols with line) for = 1.0 are also shown in Fig. 1. Reasonablygood agreement is obtained for all measured species. The major product species (i.e., CO, CO2, and H2O) are well predicted by the model. Methane, ethylene, hydrogen, and formaldehyde are also reasonably well predicted across the entire temperature range. The reactivity of n-butanol is well predicted between 800 and 950 K, but at greater temperatures the reactivity is overpredicted. Species concentrations of butyraldehyde, 1-butene, and acetaldehyde are well predicted until approximately 1000 K, above which they become underpredicted. The propene concentration is underpredicted across the entire temperature range, while ethane and acetylene concentrations are overpredicted across the entire temperature range.Figure 2 presents the experimental measurements and modeling results of n-butanol obtained at = 0.5. For the most part, the experimental results show a similar trend to that observed at= 1.0. The concentration of n-butanol is lower at = 0.5 than at = 1.0 due to the fact that agreater oxygen concentration exists in the oxygen–fuel mixture. The model better predicts the concentration of most species at = 0.5 than it does at = 1.0. 1-Butene, propene, butyraldehyde,carbon monoxide, carbon dioxide, methane, ethylene, acetaldehyde, formaldehyde, water, and hydrogen are well predicted across the entire temperature range. Similar to the case of = 1.0, thereactivity of n-butanol is overpredicted above 900 K. Again, the concentrations of acetylene and ethane are overpredicted across the entire temperature range.Full-size image (64K)Fig. 2. Comparison of the experimental concentration profiles obtained from the oxidation of n-butanol in a JSR at = 0.5, P = 10 atm, τ = 0.7 s.View Within ArticleFigure 3 presents the experimental measurements and modeling results of n-butanol obtained at = 2.0. Similar trends as those observed for other equivalence ratios are observed for theexperimental data at = 2.0. At = 2.0, the reactivity of n-butanol is well predicted across theentire temperature range, something which was not observed at other equivalence ratios In addition, there is good prediction of carbon monoxide, carbon dioxide, methane, ethylene, acetaldehyde, ethane, formaldehyde, water, and hydrogen. Qualitatively, the prediction of acetylene concentration is satisfactory. The butyraldehyde concentration is well predicted below 1000 K, while above 1000 K the model underpredicts the experimental data. The concentration of 1-butene is overpredicted above 900 K, while the concentration of propene is under underpredicted across the entire temperature range.Full-size image (63K)Fig. 3. Comparison of the experimental concentration profiles obtained from the oxidation of n-butanol in a JSR at = 2, P = 10 atm, τ = 0.7 s.View Within ArticleSome general trends are observed via analysis of the data across the three equivalence ratios. The model’s prediction of carbon monoxide, carbon dioxide, methane, ethylene, for maldehyde, water, and hydrogen concentrations is reasonably accurate across all equivalence ratios. The prediction of n-butanol,acetaldehyde, and acetylene concentrations tends to improve with increasing equivalence ratio. On the other hand, an increase in equivalence ratios results in poorer prediction of 1-butene, propene, butyraldehyde, and ethane concentrations.A reaction pathway analysis was performed at = 1.0 at T = 1000 K to determine the mostdominant pathways for n-butanol consumption. Figure 4 presents the results of the analysis in diagram format, wherein heavier weight arrows represent more dominant reaction pathways. According to the proposed model, n-butanol is consumed primarily via H-atom abstraction from the α, β, and γ carbon atoms, with each pathway accounting for approximately 22% of the total n-butanol consumption. The next most dominant pathway is H-atom abstraction from the hydroxyl group, which accounts for nearly 20% of n-butanol consumption. H-atom from the δ carbon atom accounts for nearly 14% while all the unimolecular decomposition pathways combined account for less than 0.5% of n-butanol consumption. Similarly, a reaction pathway analysis at T = 1200 K showed that unimolecular decomposition accounted for less than 4% of n-butanol consumption. Therefore, it is reasonable to conclude that n-butanol consumption in the JSR is dominated by H-atom abstraction.Full-size image (16K)Fig. 4. Reaction pathway diagram for n-butanol oxidation in the JSR at = 1, P = 10 atm,τ = 0.7 s, T = 1000 K.View Within ArticleThe pathways diagram in Fig. 4 indicates that the aC4H8OH radical primarily undergoes β-scission to form acetaldehyde and an ethyl radical (C2H5). The consumption of the bC4H8OH radical is also consumed primarily by β-scission to form a hydroxyl radical and 1-butene. The cC4H8OH radical primarily undergoes β-scission to form propene and a hydroxymethyl radical(CH2OH). The hydroxymethyl radical, which is also an intermediate in several n-butanol unimolecular decomposition pathways, undergoes β-scission to create formaldehyde. The C4H9O radical, which is formed primarily via H-atom abstraction from the n-butanol hydroxyl group, undergoes β-scission to form butyraldehyde. The least prominent n-butanol H-atom abstraction pathway leads to the formation of the dC4H8OH radical, which isomerizes to form the aC4H8OH radical. The n-butanol unimolecular dissociation reactions proceed to form radical species, which then undergo β-scission to form stable species such as acetylene, ethylene, and formaldehyde, and a number of radical species.Sensitivity analyses were conducted for n-butanol,propene, and acetylene as these compounds were not always well predicted by the model. n-Butanol was underpredicted above 900 K at both = 1.0 and = 0.5. Figure 5a displays the normalized sensitivity coefficients for the top 12reactions to which the n-butanol concentration is sensitive at T = 1050 K and all equivalence ratios. A positive sensitivity coefficient implies that an incre ase in the reaction’s forward rate will increase the n-butanol concentration at the specified temperature and equivalence ratio. At all equivalence ratios, the n-butanol concentration is very sensitive to the reaction producing OH radicals via the oxidation of H radicals. At = 0.5, the n-butanol concentration is mainlysensitive to elementary reactions between hydrogen and oxygen containing species. However, at = 1.0 and = 2.0, the n-butanol concentration is more sensitive to reactions involvinghydrocarbon radical species. This is because the pool of hydrocarbon radicals becomes more predominant as the fuel concentration in the oxygen–fuel mixture increases. Of all the n-butanol consumption reactions, the n-butanol concentration is most sensitive to those involving H-abstraction by OH radicals from the α and γ carbons.Full-size image (44K)Fig. 5. Sensitivity of n-butanol and propene to select reactions in the JSR at P = 10 atm, τ = 0.7 s.View Within ArticlePropene concentrations were not well predicted at = 1.0 and = 2.0. Figure 5b displays thenormalized sensitivity coefficients for the top 11 reactions to which the propene concentration is sensitive at T = 1000 K and all equivalence ratios. The propene concentration is sensitive to elementary reactions between hydrogen and oxygen containing species, as well as reactions involving small molecular weight hydrocarbon species. In addition, the propene concentration is sensitive to n-butanol consumption reactions involving H-abstraction from the α, β, and γ carbons.A sensitivity analysis on acetylene (not in figure) indicated the acetylene concentration is sensitive to reactions involving the C2H3 radical, and to elementary reactions between hydrogen and oxygen containing species. Adjusting the reaction rates of n-butanol consumption reactions hadlittle effect on the concentration of acetylene.5. ConclusionsNew experimental data for n-butanol oxidation in a JSR at 10 atm and equivalence ratios between 0.5 and 2.0 are compared to a novel chemical kinetic model for n-butanol oxidation. The most abundant measured product species were carbon monoxide, carbon dioxide, water, hydrogen, methane, formaldehyde, ethylene, and propene. Measured in lesser amounts were butyraldehyde, 1-butene, acetaldehyde, ethane, and acetylene. The model proposed herein provides good overall agreement with the experimental data obtained across various temperatures and equivalence ratios. It is shown that H-abstraction is the major pathway of n-butanol consumption in the JSR, while unimolecular decomposition is relatively insignificant. Further model validations are still needed; they are awaiting the availability of ongoing flame measurements.AcknowledgmentThis research acknowledges funding from NSERC.References[1] D.T. Jones and D.R. Woods, Microbiol. Rev. 50 (4) (1986), pp. 484–524. View Record in Scopus | Cited By in Scopus (326)[2] T.C. Ezeji, N. Qureshi and H.P. Blaschek, Curr. Opin. Biotechnol. 18 (2007), pp. 220–227.Article | PDF (327 K) | View Record in Scopus | Cited By in Scopus (79)[3] D. Ramey, S. Yang, Production of Butyric Acid and Butanol from Biomass, Report No. DE-F-G02-00ER86106. US Department of Energy, Morgantown, Washington, 2004.[4] G. Hess, Chem. Eng. News 84 (26) (2006), p. 9. Full Text via CrossRef | View Record in Scopus | Cited By in Scopus (7)[5] G. Hess, Chem. Eng. News 85 (27) (2007), p. 8. Full Text via CrossRef | View Record in Scopus | Cited By in Scopus (1)[6] Y. Yacoub, R. Bata and M. Gautam, Proc. Inst. Mech. Eng. 212 (1998), pp. 363–379. Full Text via CrossRef | View Record in Scopus | Cited By in Scopus (15)[7] G.L. Miller, J.L. Smith and J.P. Workman, Trans. ASAE 24 (1981), pp. 538–540.[8] T.K. Bhattacharya, S. Chatterjee and T.N. Mishra, Appl. Eng. Agric. 20 (3) (2004), pp. 253–257. View Record in Scopus | Cited By in Scopus (10)[9] J.A. Barnard, Trans. Faraday Soc. 53 (11) (1957), pp. 1423–1430. Full Text via CrossRef | View Record in Scopus | Cited By in Scopus (11)[10] J.A. Barnard, Trans. Faraday Soc. 55 (6) (1959), pp. 947–951. Full Text via CrossRef | View Record in Scopus | Cited By in Scopus (4)[11] A.F. Roberts, J. Imp. Coll. Chem. Eng. Soc. 12 (1959), pp. 58–73.[12] C.S. McEnally and L.D. Pfefferle, Proc. Combust. Inst. 30 (2005), pp. 1363–1370. Article |PDF (389 K) | View Record in Scopus | Cited By in Scopus (41)[13] B. Yang, P. Oswald and Y. Li et al., Combust. Flame 148 (2007), pp. 198–209. Article | PDF (1138 K) | View Record in Scopus | Cited By in Scopus (54)[14] P. Dagaut and M. Cathonnet, Prog. Energy Combust. Sci. 32 (2006), pp. 48–92. Article |PDF (1414 K) | View Record in Scopus | Cited By in Scopus (77)[15] P. Dagaut and S. Gail, J. Phys. Chem. A 111 (19) (2007), pp. 3992–4000. Full Text via CrossRef | View Record in Scopus | Cited By in Scopus (27)[16] P. Glarborg, R.J. Kee, J.F. Grcar, J.A. Miller, PSR: A FORTRAN Program for Modeling Well-Stirred Reactors, Report No. SAND86-8209, Sandia National Laboratories, Livermore, CA, 1986.[17] T. Le Cong, P. Dagaut, Kinetics of natural gas, natural gas/syngas mixtures oxidation and effect of burnt gas recirculation: experimental and detailed modeling, in: Proceedings of the ASME Turbo Expo 2007: Power for Land, Sea and Air, Montréal, Canada, May 14–17, 2007, GT2007-27146, pp. 1–9.[18] P. Dagaut, Phys. Chem. Chem. Phys. 4 (2002), pp. 2079–2094. Full Text via CrossRef | View Record in Scopus | Cited By in Scopus (100)[19] F. Battin-Leclerc, R. Bounaceur, G.M. Côme, et al., EXGAS-ALKANES, a software for the automatic generation of mechanisms for the oxidation of alkanes, 2004, CNRS-DCPR.[20] Y. Tan, P. Dagaut, M. Cathonnet and J.C. Boettner, Combust. Sci. Technol. 102 (1994), pp. 21–55. Full Text via CrossRef[21] C. Muller, V. Michel, G. Scacchi and G.M. Côme, J. Chim. Phys. Phys.-Chim. Biol. 92 (5) (1995), pp. 1154–1178.Appendix A. Supplementary dataDownload this File (85 K)Supplementary data. The proposed n-butanol chemical kinetic mechanism in CHEMKIN format View Within ArticleDownload this File (160 K)Supplementary data. The proposed n-butanol thermochemical data in CHEMKIN formatView Within ArticleDownload this File (515 K)Supplementary data. An MS Excel data file with all the experimental and model dataView Within ArticleCorresponding author. Fax: +1 416 978 7753.Proceedings of the Combustion InstituteV olume 32, Issue 1, 2009, Pages 229-237。
化学专业英语-马永祥-兰州大学

ContentsTHE ELEMENTS AND THE PERIODIC TABLE01. ......................................................- 3 -THE NONMETAL ELEMENTS02. ..................................................................................- 5 -GROUPS IB AND IIB ELEMENTS03. ............................................................................- 7 -GROUPS IIIB—VIIIB ELEMENTS04. ............................................................................- 9 -INTERHALOGEN AND NOBLE GAS COMPOUNDS05. ...........................................- 11 -06. ....................................- 13 -THE CLASSIFICATION OF INORGANIC COMPOUNDSTHE NOMENCLATURE OF INORGANIC COMPOUNDS07. ....................................- 15 -BRONSTED'S AND LEWIS' ACID-BASE CONCEPTS08. ..........................................- 19 -09. ..........................................................................- 22 -THE COORDINATION COMPLEXALKANES10. ..................................................................................................................- 25 -11. .............................................................................- 28 -UNSATURATED COMPOUNDSTHE NOMENCLATURE OF CYCLIC HYDROCARBONS12. ...................................- 30 -SUBSTITUTIVE NOMENCLATURE13. .......................................................................- 33 -14. .......................................................- 37 -THE COMPOUNDS CONTAINING OXYGENPREPARATION OF A CARBOXYLiC ACID BY THE GRIGNARD METHOD15. ..- 39 -THE STRUCTURES OF COVALENT COMPOUNDS16. ............................................- 41 -OXIDATION AND REDUCTION IN ORGANIC CHEMISTRY17. ............................- 44 -SYNTHESIS OF ALCOHOLS AND DESIGN OF ORGANIC SYNTHESIS18. ..........- 47 -ORGANOMETALLICS—METAL π COMPLEXES19. ................................................- 49 -THE ROLE OF PROTECTIVE GROUPS IN ORGANIC SYNTHESIS20. ...................- 52 -ELECTROPHILIC REACTIONS OF AROMATIC COMPOUNDS21. ........................- 54 -POLYMERS22. ................................................................................................................- 57 -ANALYTICAL CHEMISTRY AND PROBLEMS IN SOCIETY23. ............................- 61 -VOLUMETRIC ANALYSIS24. ......................................................................................- 63 -QUALITATIVE ORGANIC ANALYSIS25. ..................................................................- 65 -VAPOR-PHASE CHROMATOGRAPHY26. .................................................................- 67 -INFRARED SPECTROSCOPY27. ..................................................................................- 70 -NUCLEAR MAGNETIC RESONANCE (I)28. ..............................................................- 72 -NUCLEAR MAGNETIC RESONANCE(II)29. ..............................................................- 75 -A MAP OF PHYSICAL CHEMISTRY30. ......................................................................- 77 -THE CHEMICAL THERMODYNAMICS31. ................................................................- 79 -CHEMICAL EQUILIBRIUM AND KINETICS32. ........................................................- 82 -THE RATES OF CHEMICAL REACTIONS33. ............................................................- 85 -NATURE OF THE COLLOIDAL STATE34. .................................................................- 88 -ELECTROCHEMICAL CELLS35. .................................................................................- 90 -BOILING POINTS AND DISTILLATION36. ...............................................................- 93 -EXTRACTIVE AND AZEOTROPIC DISTILLATION37. ............................................- 96 -CRYSTALLIZATION38. ................................................................................................- 98 -39. ...................................................................................- 100 -MATERIAL ACCOUNTINGTHE LITERATURE MATRIX OF CHEMISTRY40. ...................................................- 102 -01. THE ELEMENTS AND THE PERIODIC TABLEThe number of protons in the nucleus of an atom is referred to as the atomic number, or proton number, Z. The number of electrons in an electrically neutral atom is also equal to the atomic number, Z. The total mass of an atom is determined very nearly by the total number of protons and neutrons in its nucleus. This total is called the mass number, A. The number of neutrons in an atom, the neutron number, is given by the quantity A-Z.The term element refers to, a pure substance with atoms all of a single kind. To the chemist the "kind" of atom is specified by its atomic number, since this is the property that determines its chemical behavior. At present all the atoms from Z = 1 to Z = 107 are known; there are 107 chemical elements. Each chemical element has been given a name and a distinctive symbol. For most elements the symbol is simply the abbreviated form of the English name consisting of one or two letters, for example:oxygen==O nitrogen ==N neon==Ne magnesium ==MgSome elements,which have been known for a long time,have symbols based on their Latin names, for example: iron==Fe(ferrum) copper==Cu(cuprum) lead==Pb(plumbum)A complete listing of the elements may be found in Table 1.Beginning in the late seventeenth century with the work of Robert Boyle, who proposed the presently accepted concept of an element, numerous investigations produced a considerable knowledge of the properties of elements and their compounds1. In 1869, D.Mendeleev and L. Meyer, working independently, proposed the periodic law. In modern form, the law states that the properties of the elements are periodic functions of their atomic numbers. In other words, when the elements are listed in order of increasing atomic number, elements having closely similar properties will fall at definite intervals along the list. Thus it is possible to arrange the list of elements in tabular form with elements having similar properties placed in vertical columns2. Such an arrangement is called a periodic Each horizontal row of elements constitutes a period. It should be noted that the lengths of the periods vary. There is a very short period containing only 2 elements, followed by two short periods of 8 elements each, and then two long periods of 18 elements each. The next period includes 32 elements, and the last period is apparently incomplete. With this arrangement, elements in the same vertical column have similar characteristics. These columns constitute the chemical families or groups. The groups headed by the members of the two 8-element periods are designated as main group elements, and the members of the other groups are called transition or inner transition elements.In the periodic table, a heavy stepped line divides the elements into metals and nonmetals. Elements to the left of this line (with the exception of hydrogen) are metals, while those to the right are nonmetals. This division is for convenience only; elements bordering the line—the metalloids-have properties characteristic of - both metals and nonmetals. It may be seen that most of the elements, including all the transition and inner transition elements, are metals.Except for hydrogen, a gas, the elements of group IA make up the alkali metal family. They are very reactive metals, and they are never found in the elemental state in nature. However, their compounds are widespread. All the members of the alkali metal family, form ions having a charge of 1+ only. In contrast, the elements of group IB —copper, silver, and gold—are comparatively inert. They are similar to the alkali metals in that they exist as 1+ ions in many of their compounds. However, as is characteristic of most transition elements, they form ions having other charges as well.The elements of group IIA are known as the alkaline earth metals. Their characteristic ionic charge is 2+. These metals, particularly the last two members of the group, are almost as reactive as the alkali metals. The group IIB elements—zinc, cadmium, and mercury are less reactive than are those of group II A5, but are more reactive than the neighboring elements of group IB. The characteristic charge on their ions is also 2+.With the exception of boron, group IIIA elements are also fairly reactive metals. Aluminum appears to be inert toward reaction with air, but this behavior stems from the fact that the metal forms a thin, invisible film of aluminum oxide on the surface, which protects the bulk of the metal from further oxidation. The metals of group IIIA form ions of 3+ charge. Group IIIB consists of the metals scandium, yttrium, lanthanum, and actinium.Group IVA consists of a nonmetal, carbon, two metalloids, silicon and germanium, and two metals, tin and lead. Each of these elements forms some compounds with formulas which indicate that four other atoms are present per group IVA atom, as, for example, carbon tetrachloride, GCl4. The group IVB metals —titanium, zirconium, and hafnium —also forms compounds in which each group IVB atom is combined with four other atoms; these compounds are nonelectrolytes when pure.The elements of group V A include three nonmetals — nitrogen, phosphorus, and arsenic—and two metals — antimony and bismuth. Although compounds with the formulas N2O5, PCl5, and AsCl5 exist, none of them is ionic. These elements do form compounds-nitrides, phosphides, and arsenides — in which ions having charges of minus three occur. The elements of group VB are all metals. These elements form such a variety of different compounds that their characteristics are not easily generalized.With the exception of polonium, the elements of group VIA are typical nonmetals. They are sometimes known, as the, chalcogens, from the Greek word meaning "ash formers". In their binary compounds with metals they exist as ions having a charge of 2-. The elements of group ⅦA are all nonmetals and are known as the halogens. from the Greek term meaning "salt formers.” They are the most reactive nonmetals and are capable of reacting with practically all the metals and with most nonmetals, including each other.The elements of groups ⅥB, ⅦB, and VIIIB are all metals. They form such a wide Variety of compounds that it is not practical at this point to present any examples as being typical of the behavior of the respective groups.The periodicity of chemical behavior is illustrated by the fact that. excluding the first period, each period begins with a very reactive metal. Successive element along the period show decreasing metallic character, eventually becoming nonmetals, and finally, in group ⅦA, a very reactive nonmetal is found. Each period ends with a member of the noble gas family.02. THE NONMETAL ELEMENTSWe noted earlier. that -nonmetals exhibit properties that are greatly different from those of the metals. As a rule, the nonmetals are poor conductors of electricity (graphitic carbon is an exception) and heat; they are brittle, are often intensely colored, and show an unusually wide range of melting and boiling points. Their molecular structures, usually involving ordinary covalent bonds, vary from the simple diatomic molecules of H2, Cl2, I2, and N2 to the giant molecules of diamond, silicon and boron.The nonmetals that are gases at room temperature are the low-molecular weight diatomic molecules and the noble gases that exert very small intermolecular forces. As the molecular weight increases, we encounter a liquid (Br2) and a solid (I2) whose vapor pressures also indicate small intermolecular forces. Certain properties of a few nonmetals are listed in Table 2.Table 2- Molecular Weights and Melting Points of Certain NonmetalsDiatomic Molecules MolecularWeightMelting Point°CColorH22-239.1'NoneN228-210NoneF238-223Pale yellowO232-218Pale blueCl271-102Yellow — greenBr2160-7.3Red — brownI2254113Gray—blackSimple diatomic molecules are not formed by the heavier members of Groups V and VI at ordinary conditions. This is in direct contrast to the first members of these groups, N2 and O2. The difference arises because of the lower stability of πbonds formed from p orbitals of the third and higher main energy levels as opposed to the second main energy level2. The larger atomic radii and more dense electron clouds of elements of the third period and higher do not allow good parallel overlap of p orbitals necessary for a strong πbond. This is a general phenomenon — strong π bonds are formed only between elements of the second period. Thus, elemental nitrogen and oxygen form stable molecules with both σand π bonds, but other members of their groups form more stable structures based on σbonds only at ordinary conditions. Note3 that Group VII elements form diatomic molecules, but πbonds are not required for saturation of valence.Sulfur exhibits allotropic forms. Solid sulfur exists in two crystalline forms and in an amorphous form. Rhombic sulfur is obtained by crystallization from a suitable solution, such as CS2, and it melts at 112°C. Monoclinic sulfur is formed by cooling melted sulfur and it melts at 119°C. Both forms of crystalline sulfur melt into S-gamma, which is composed of S8 molecules. The S8 molecules are puckered rings and survive heating to about 160°C. Above 160°C, the S8 rings break open, and some of these fragments combine with each other to form a highly viscous mixture of irregularly shaped coils. At a range of higher temperatures the liquid sulfur becomes so viscous that it will not pourfrom its container. The color also changes from straw yellow at sulfur's melting point to a deep reddish-brown as it becomes more viscous.As4 the boiling point of 444 °C is approached, the large-coiled molecules of sulfur are partially degraded and the liquid sulfur decreases in viscosity. If the hot liquid sulfur is quenched by pouring it into cold water, the amorphous form of sulfur is produced. The structure of amorphous sulfur consists of large-coiled helices with eight sulfur atoms to each turn of the helix; the overall nature of amorphous sulfur is described as3 rubbery because it stretches much like ordinary rubber. In a few hours the amorphous sulfur reverts to small rhombic crystals and its rubbery property disappears.Sulfur, an important raw material in industrial chemistry, occurs as the free element, as SO2 in volcanic regions, asH2S in mineral waters, and in a variety of sulfide ores such as iron pyrite FeS2, zinc blende ZnS, galena PbS and such, and in common formations of gypsum CaSO4 • 2H2O, anhydrite CaSO4, and barytes BaSO4 • 2H2O. Sulfur, in one form or another, is used in large quantities for making sulfuric acid, fertilizers, insecticides, and paper.Sulfur in the form of SO2 obtained in the roasting of sulfide ores is recovered and converted to sulfuric acid, although in previous years much of this SO2 was discarded through exceptionally tall smokestacks. Fortunately, it is now economically favorable to recover these gases, thus greatly reducing this type of atmospheric pollution. A typical roasting reaction involves the change:2 ZnS +3 O2—2 ZnO + 2 SO2Phosphorus, below 800℃ consists of tetratomic molecules, P4. Its molecular structure provides for a covalence of three, as may be expected from the three unpaired p electrons in its atomic structure, and each atom is attached to three others6. Instead of a strictly orthogonal orientation, with the three bonds 90° to each other, the bond angles are only 60°. This supposedly strained structure is stabilized by the mutual interaction of the four atoms (each atom is bonded to the other three), but it is chemically the most active form of phosphorus. This form of phosphorus, the white modification, is spontaneously combustible in air. When heated to 260°C it changes to red phosphorus, whose structure is obscure. Red phosphorus is stable in air but, like all forms of phosphorus, it should be handled carefully because of its tendency to migrate to the bones when ingested, resulting in serious physiological damage.Elemental carbon exists in one of two crystalline structures — diamond and graphite. The diamond structure, based on tetrahedral bonding of hybridized sp3orbitals, is encountered among Group IV elements. We may expect that as the bond length increases, the hardness of the diamond-type crystal decreases. Although the tetrahedral structure persists among the elements in this group — carbon, silicon, germanium, and gray tin — the interatomic distances increase from 1.54 A for carbon to 2.80 A for gray tin. Consequently .the bond strengths among the four elements range from very strong to quite weak. In fact, gray tin is so soft that it exists in the form of microcrystals or merely as a powder. Typical of the Group IV diamond-type crystalline elements, it is a nonconductor and shows other nonmetallic properties7.03. GROUPS IB AND IIB ELEMENTSPhysical properties of Group IB and IIBThese elements have a greater bulk use as metals than in compounds, and their physical properties vary widely.Gold is the most malleable and ductile of the metals. It can be hammered into sheets of 0.00001 inch in thickness; one gram of the metal can be drawn into a wire 1.8 mi in length1. Copper and silver are also metals that are mechanically easy to work. Zinc is a little brittle at ordinary temperatures, but may be rolled into sheets at between 120° to 150℃; it becomes brittle again about 200℃-The low-melting temperatures of zinc contribute to the preparation of zinc-coated iron .galvanized iron; clean iron sheet may be dipped into vats of liquid zinc in its preparation. A different procedure is to sprinkle or air blast zinc dust onto hot iron sheeting for a zinc melt and then coating.Cadmium has specific uses because of its low-melting temperature in a number of alloys. Cadmium rods are used in nuclear reactors because the metal is a good neutron absorber.Mercury vapor and its salts are poisonous, though the free metal may be taken internally under certain conditions. Because of its relatively low boiling point and hence volatile nature, free mercury should never be allowed to stand in an open container in the laboratory. Evidence shows that inhalation of its vapors is injurious.The metal alloys readily with most of the metals (except iron and platinum) to form amalgams, the name given to any alloy of mercury.Copper sulfate, or blue vitriol (CuSO4 • 5H2O) is the most important and widely used salt of copper. On heating, the salt slowly loses water to form first the trihydrate (CuSO4 • 3H z O), then the monohydrate (CuSO4 • H2O), and finally the white anhydrous salt. The anhydrous salt is often used to test for the presence of water in organic liquids. For example, some of the anhydrous copper salt added to alcohol (which contains water) will turn blue because of the hydration of the salt.Copper sulfate is used in electroplating. Fishermen dip their nets in copper sulfate solution to inhibit the growth of organisms that would rot the fabric. Paints specifically formulated for use on the bottoms of marine craft contain copper compounds to inhibit the growth of barnacles and other organisms.When dilute ammonium hydroxide is added" to a solution of copper (I) ions, a greenish precipitate of Cu(OH)2 or a basic copper(I) salt is formed. This dissolves as more ammonium hydroxide is added. The excess ammonia forms an ammoniated complex with the copper (I) ion of the composition, Cu(NH3)42+. This ion is only slightly dissociated; hence in an ammoniacal solution very few copper (I) ions are present. Insoluble copper compounds, execpt copper sulfide, are dissolved by ammonium hydroxids. The formation of the copper (I) ammonia ion is often used as a test for Cu2+ because of its deep, intense blue color.Copper (I) ferrocyanide [Cu2Fe(CN)6] is obtained as a reddish-brown precipitate on the addition of a soluble ferrocyanide to a solution of copper ( I )ions. The formation of this salt is also used as a test for the presence of copper (I) ions.Compounds of Silver and GoldSilver nitrate, sometimes called lunar caustic, is the most important salt of silver. It melts readily and may be cast into sticks for use in cauterizing wounds. The salt is prepared by dissolving silver in nitric acid and evaporating the solution.3Ag + 4HNO3—3AgNO3 + NO + 2H2OThe salt is the starting material for most of the compounds of silver, including the halides used in photography. It is readily reduced by organic reducing agents, with the formation of a black deposit of finely divided silver; this action is responsible for black spots left on the fingers from the handling of the salt. Indelible marking inks and pencils take advantage of this property of silver nitrate.The halides of silver, except the fluoride, are very insoluble compounds and may be precipitated by the addition of a solution of silver salt to a solution containing chloride, bromide, or iodide ions.The addition of a strong base to a solution of a silver salt precipitates brown silver oxide (Ag2G). One might expect the hydroxide of silver to precipitate, but it seems likely that silver hydroxide is very unstable and breaks down into the oxide and water — if, indeed, it is ever formed at all3. However, since a solution of silver oxide js definitely basic, there must be hydroxide ions present in solution.Ag2O + H2O = 2Ag+ + 2OH-Because of its inactivity, gold forms relatively few compounds. Two series of compounds are known — monovalent and trivalent. Monovalent (aurous) compounds resemble silver compounds (aurous chloride is water insoluble and light sensitive), while the higher valence (auric) compounds tend to form complexes. Gold is resistant to the action of most chemicals —air, oxygen, and water have no effect. The common acids do not attack the metal, but a mixture of hydrochloric and nitric acids (aqua regia) dissolves it to form gold( I ) chloride or chloroauric acid. The action is probably due to free chlorine present in the aqua regia.3HCl + HNO3----→ NOCl+Cl2 + 2H2O2Au + 3Cl2 ----→ 2AuCl3AuCl3+HCl----→ HAuCl4chloroauric acid (HAuCl4-H2O crystallizes from solution).Compounds of ZincZinc is fairly high in the activity series. It reacts readily with acids to produce hydrogen and displaces less active metals from their salts. 1 he action of acids on impure zinc is much more rapid than on pure zinc, since bubbles of hydrogen gas collect on the surface of pure zinc and slow down the action. If another metal is present as an impurity, the hydrogen is liberated from the surface of the contaminating metal rather than from the zinc. An electric couple to facilitate the action is probably Set up between the two metals.Zn + 2H+----→ Zn2+ + H2Zinc oxide (ZnO), the most widely used zinc compound, is a white powder at ordinary temperatures, but changes to yellow on heating. When cooled, it again becomes white. Zinc oxide is obtained by burning zinc in air, by heating the basic carbonate, or by roasting the sulfide. The principal use of ZnO is as a filler in rubber manufacture, particularly in automobile tires. As a body for paints it has the advantage over white lead of not darkening on exposure to an atmosphere containing hydrogen sulfide. Its covering power, however, is inferior to that of white lead.04. GROUPS IIIB—VIIIB ELEMENTSGroup I-B includes the elements scandium, yttrium, lanthanum, and actinium1, and the two rare-earth series of fourteen elements each2 —the lanthanide and actinide series. The principal source of these elements is the high gravity river and beach sands built up by a water-sorting process during long periods of geologic time. Monazite sand, which contains a mixture of rare earth phosphates, and an yttrium silicate in a heavy sand are now commercial sources of a number of these scarce elements.Separation of the elements is a difficult chemical operation. The solubilities of their compounds are so nearly alike that a separation by fractional crystallization is laborious and time-consuming. In recent years, ion exchange resins in high columns have proved effective. When certain acids are allowed to flow down slowly through a column containing a resin to which ions of Group III B metals are adsorbed, ions are successively released from the resin3. The resulting solution is removed from the bottom of the column or tower in bands or sections. Successive sections will contain specific ions in the order of release by the resin. For example .lanthanum ion (La3+) is most tightly held to the resin and is the last to be extracted, lutetium ion (Lu3+) is less tightly held and appears in one of the first sections removed. If the solutions are recycled and the acid concentrations carefully controlled, very effective separations can be accomplished. Quantities of all the lanthanide series (except promethium, Pm, which does not exist in nature as a stable isotope) are produced for the chemical market.The predominant group oxidation number of the lanthanide series is +3, but some of the elements exhibit variable oxidation states. Cerium forms cerium( III )and cerium ( IV ) sulfates, Ce2 (SO4 )3 and Ce(SO4 )2, which are employed in certain oxidation-reduction titrations. Many rare earth compounds are colored and are paramagnetic, presumably as a result of unpaired electrons in the 4f orbitals.All actinide elements have unstable nuclei and exhibit radioactivity. Those with higher atomic numbers have been obtained only in trace amounts. Actinium (89 Ac), like lanthanum, is a regular Group IIIB element.Group IVB ElementsIn chemical properties these elements resemble silicon, but they become increasingly more metallic from titanium to hafnium. The predominant oxidation state is +4 and, as with silica (SiO2), the oxides of these elements occur naturally in small amounts. The formulas and mineral names of the oxides are TiO2, rutile; ZrO2, zirconia; HfO2, hafnia. Titanium is more abundant than is usually realized. It comprises about 0.44%of the earth's crust. It is over 5.0%in average composition of first analyzed moon rock. Zirconium and titanium oxides occur in small percentages in beach sands.Titanium and zirconium metals are prepared by heating their chlorides with magnesium metal. Both are particularly resistant to corrosion and have high melting points.Pure TiO2 is a very white substance which is taking the place of white lead in many paints. Three-fourths of the TiO2 is used in white paints, varnishes, and lacquers. It has the highest index of refraction (2.76) and the greatest hiding power of all the common white paint materials. TiO2 also is used in the paper, rubber, linoleum, leather, and textile industries.Group VB Elements: Vanadium, Niobium, and TantalumThese are transition elements of Group VB, with a predominant oxidation number of + 5. Their occurrence iscomparatively rare.These metals combine directly with oxygen, chlorine, and nitrogen to form oxides, chlorides, and nitrides, respectively. A small percentage of vanadium alloyed with steel gives a high tensile strength product which is very tough and resistant to shock and vibration. For this reason vanadium alloy steels are used in the manufacture ofhigh-speed tools and heavy machinery. Vanadium oxide is employed as a catalyst in the contact process of manufacturing sulfuric acid. Niobium is a very rare element, with limited use as an alloying element in stainless steel. Tantalum has a very high melting point (2850 C) and is resistant to corrosion by most acids and alkalies.Groups VIB and VIIB ElementsChromium, molybdenum, and tungsten are Group VIB elements. Manganese is the only chemically important element of Group VIIB. All these elements exhibit several oxidation states, acting as metallic elements in lower oxidation states and as nonmetallic elements in higher oxidation states. Both chromium and manganese are widely used in alloys, particularly in alloy steels.Group VIIIB MetalsGroup VIIIB contains the three triads of elements. These triads appear at the middle of long periods of elements in the periodic table, and are members of the transition series. The elements of any given horizontal triad have many similar properties, but there are marked differences between the properties of the triads, particularly between the first triad and the other two. Iron, cobalt, and nickel are much more active than members of the other two triads, and are also much more abundant in the earth's crust. Metals of the second and third triads, with many common properties, are usually grouped together and called the platinum metals.These elements all exhibit variable oxidation states and form numerous coordination compounds.CorrosionIron exposed to the action of moist air rusts rapidly, with the formation of a loose, crumbly deposit of the oxide. The oxide does not adhere to the surface of the metal, as does aluminum oxide and certain other metal oxides, but peelsoff .exposing a fresh surface of iron to the action of the air. As a result, a piece of iron will rust away completely in a relatively short time unless steps are taken to prevent the corrosion. The chemical steps in rusting are rather obscure, but it has been established that the rust is a hydrated oxide of iron, formed by the action of both oxygen and moisture, and is markedly speeded up by the presence of minute amounts of carbon dioxide5.Corrosion of iron is inhibited by coating it with numerous substances, such as paint, an aluminum powder gilt, tin, or organic tarry substances or by galvanizing iron with zinc. Alloying iron with metals such as nickel or chromium yields a less corrosive steel. "Cathodic protection" of iron for lessened corrosion is also practiced. For some pipelines and standpipes zinc or magnesium rods in the ground with a wire connecting them to an iron object have the following effect: with soil moisture acting as an electrolyte for a Fe — Zn couple the Fe is lessened in its tendency to become Fe2+. It acts as a cathode rather than an anode.。
欧洲药典7.5版
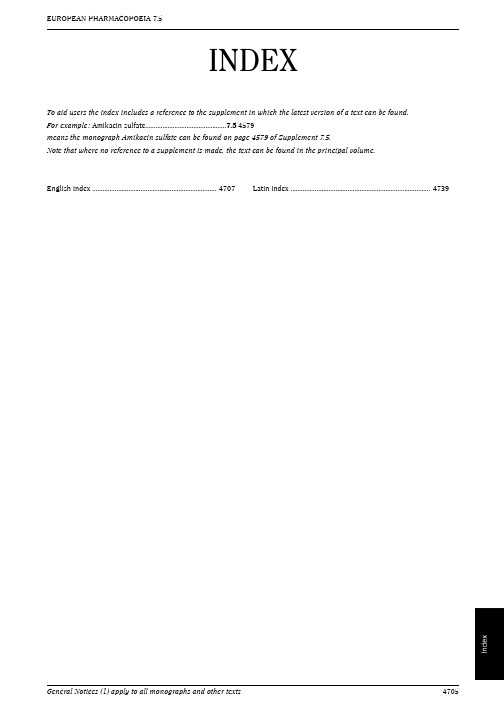
INDEX
To aid users the index includes a reference to the supplement in which the latest version of a text can be found. For example : Amikacin sulfate...............................................7.5-4579 means the monograph Amikacin sulfate can be found on page 4579 of Supplement 7.5. Note that where no reference to a supplement is made, the text can be found in the principal volume.
English index ........................................................................ 4707
Latin index ................................................................................. 4739
EUROPEAN PHARMACOPபைடு நூலகம்EIA 7.5
Index
Numerics 1. General notices ................................................................... 7.5-4453 2.1.1. Droppers...................
植物甾醇与三萜类皂苷生物合成基因调控的研究进展_刘强
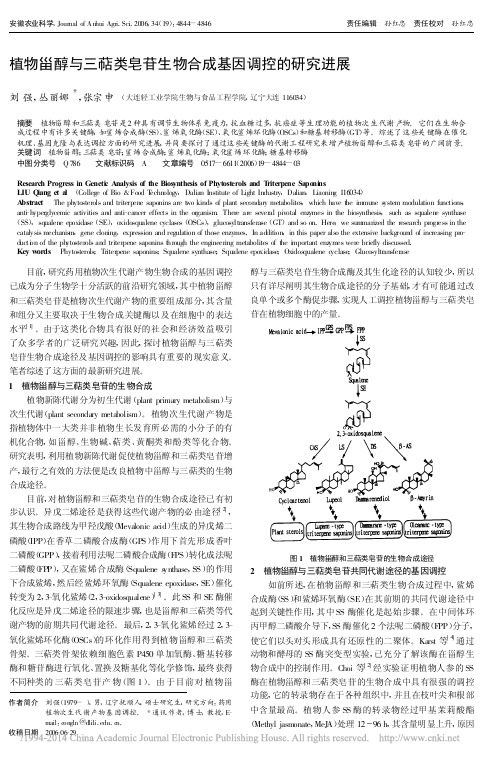
植物甾醇与三萜类皂苷生物合成基因调控的研究进展刘强,丛丽娜*,张宗申 (大连轻工业学院生物与食品工程学院,辽宁大连116034)摘要 植物甾醇和三萜类皂苷是2种具有调节生物体系免疫力,抗血糖过多,抗癌症等生理功能的植物次生代谢产物。
它们在生物合成过程中有许多关键酶,如鲨烯合成酶(S S )、鲨烯氧化酶(SE )、氧化鲨烯环化酶(OSCs )和糖基转移酶(GT )等。
综述了这些关键酶在催化机理、基因克隆与表达调控方面的研究进展,并简要探讨了通过这些关键酶的代谢工程研究来增产植物甾醇和三萜类皂苷的广阔前景。
关键词 植物甾醇;三萜类皂苷;鲨烯合成酶;鲨烯氧化酶;氧化鲨烯环化酶;糖基转移酶中图分类号 Q 786 文献标识码 A 文章编号 0517-6611(2006)19-4844-03Research Progress in Geneti c A nalysis o f the Bios ynthesis of Phytosterols a nd T riterpene Sa po ni ns LIU Q iang et a l (College of Bio &Food T echn ology ,Dalian Institute of Light In dustry ,Dalian ,Liaonin g 116034)A bstract The ph ytosterols an d triterp en e saponins are t wo kinds of plant second ary metabolites ,which h ave t he im mu ne s ystem modulation fu nctions ,anti -h yperglycemic activities an d anti -cancer effects in the organism .There are several pivotal enz ymes in the biosynthesis ,su ch as sq ualen e synthase (SS ),sq ualen e ep oxidase (SE ),oxidosqu alene cyclases (OSCs ),glucosyltransferase (GT )and so on .Here ,we su m marized th e research progress in the catalysis m echanis m ,gene clon ing ,exp ression and regulation of those enzymes .In ad dition ,in this paper als o the extensive backgroun d of increasing pro -d ucti on of the ph ytosterols an d triterpene saponins t hrough the engineering metabolites of t he i mportant enz ym es were briefly discussed .Key w ords Phytosterols ;Triterpene saponins ;Squ alene synthase ;Squ alene epoxidase ;Oxidos qualene c yclase ;Glucosyltran sferas e 目前,研究药用植物次生代谢产物生物合成的基因调控已成为分子生物学十分活跃的前沿研究领域,其中植物甾醇和三萜类皂苷是植物次生代谢产物的重要组成部分,其含量和组分又主要取决于生物合成关键酶以及在细胞中的表达水平[1]。
人类癌症中的磷脂酰肌醇3激酶(PI3K)通路突变
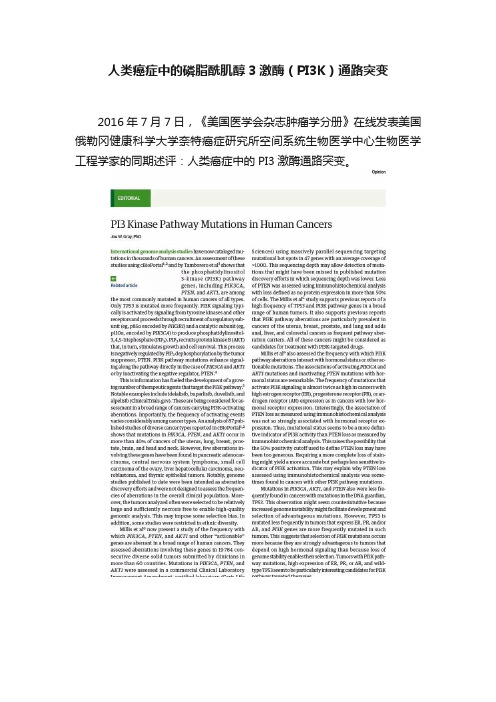
人类癌症中的磷脂酰肌醇3激酶(PI3K)通路突变2016年7月7日,《美国医学会杂志肿瘤学分册》在线发表美国俄勒冈健康科学大学奈特癌症研究所空间系统生物医学中心生物医学工程学家的同期述评:人类癌症中的PI3激酶通路突变。
JAMA Oncol. 2016 Jul 7. [Epub ahead of print]PI3 Kinase Pathway Mutations in Human Cancers. Joe W. Gray.Department of Biomedical Engineering, Center for Spatial Systems Biomedicine, Knight Cancer Institute, Oregon Health and Science University, Portland.International genome analysis studies have now cataloged mutations in thousands of human cancers. An assessment of these studies using cBioPortal [1,2] and by Tamborero et al [3] shows that the phosphatidylinositol 3-kinase (PI3K) pathway genes, including PIK3CA, PTEN, and AKT1, are among the most commonly mutated in human cancers of all types. Only TP53 is mutated more frequently. PI3K signaling typically is activated by signaling from tyrosine kinases and other receptors and proceeds through recruitment of a regulatory subunit (eg, p85α encoded by PIK3R1) and a cataly tic subunit (eg, p110α, encoded by PIK3CA) to produce phosphatidylinositol-3,4,5-trisphosphate (PIP3). PIP3 recruits protein kinase B (AKT) that, in turn, stimulates growth and cell survival. This process is negatively regulated by PIP3 dephosphorylation by the tumor suppressor, PTEN. PI3K pathway mutations enhance signaling along the pathway directly in the case of PIK3CA and AKT1 or by inactivating the negative regulator, PTEN. [4]This is information has fueled the development of a growing number of therapeutic agents that target the PI3K pathway. [5] Notable examples include idelalisib, buparlisib, duvelisib, and alpelisib (). These are being considered for assessment in a broad range of cancers carrying PI3K-activating aberrations. Importantly, the frequency of activating events varies considerably among cancer types. An analysis of 87 published studies of diverse cancer types reported in cBioPortal [1,2] shows that mutations in PIK3CA, PTEN, and AKT1 occur in more than 40% of cancers of the uterus, lung, breast, prostate,brain, and head and neck. However, few aberrations involving these genes have been found in pancreatic adenocarcinoma, central nervous system lymphoma, small cell carcinoma of the ovary, liver hepatocellular carcinoma, neuroblastoma, and thymic epithelial tumors. Notably, genome studies published to date were been intended as aberration discovery efforts and were not designed to assess the frequencies of aberrations in the overall clinical population. Moreover, the tumors analyzed often were selected to be relatively large and sufficiently necrosis free to enable high-quality genomic analysis. This may impose some selection bias. In addition, some studies were restricted in ethnic diversity.Millis et al [6] now present a study of the frequency with which PIK3CA, PTEN, and AKT1 and other "actionable" genes are aberrant in a broad range of human cancers. They assessed aberrations involving these genes in 19,784 consecutive diverse solid tumors submitted by clinicians in more than 60 countries. Mutations in PIK3CA, PTEN, and AKT1 were assessed in a commercial Clinical Laboratory Improvement Amendment-certified laboratory (Caris Life Sciences) using massively parallel sequencing targeting mutational hot spots in 47 genes with an average coverage of ×1000. This sequencing depth may allow detection of mutations that might have been missed in published mutation discovery efforts in which sequencing depth was lower. Loss of PTEN was assessed using immunohistochemical analysis with loss defined as no protein expression in more than 50% of cells. The Millis et al [6] study supports previous reports of a high frequency of TP53 and PI3K pathway genes in a broad range of human tumors. It also supports previous reports that PI3K pathway aberrations are particularly prevalent in cancers of theuterus, breast, prostate, and lung and adds anal, liver, and colorectal cancers as frequent pathway aberration carriers. All of these cancers might be considered as candidates for treatment with PI3K-targeted drugs.Millis et al [6] also assessed the frequency with which PI3K pathway aberrations interact with hormonal status or other actionable mutations. The associations of activating PIK3CA and AKT1 mutations and inactivating PTEN mutations with hormonal status are remarkable. The frequency of mutations that activate PI3K signaling is almost twice as high in cancers with high estrogen receptor (ER), progesterone receptor (PR), or androgen receptor (AR) expression as in cancers with low hormonal receptor expression. Interestingly, the association of PTEN loss as measured using immunohistochemical analysis was not so strongly associated with hormonal receptor expression. Thus, mutational status seems to be a more definitive indicator of PI3K activity than PTEN loss as measured by immunohistochemical analysis. This raises the possibility that the 50% positivity cutoff used to define PTEN loss may have been too generous. Requiring a more complete loss of staining might yield a more accurate but perhaps less sensitive indicator of PI3K activation. This may explain why PTEN loss assessed using immunohistochemical analysis was sometimes found in cancers with other PI3K pathway mutations.Mutations in PIK3CA, AKT1, and PTEN also were less frequently found in cancers with mutations in the DNA guardian, TP53. This observation might seem counterintuitive because increased genome instability might facilitate development and selection of advantageous mutations. However, TP53 is mutated less frequently in tumors that express ER, PR, and/or AR, and PI3Kgenes are more frequently mutated in such tumors. This suggests that selection of PI3K mutations occurs more because they are strongly advantageous to tumors that depend on high hormonal signaling than because loss of genome stability enables their selection. Tumors with PI3K pathway mutations, high expression of ER, PR, or AR, and wild-type TP53 seem to be particularly interesting candidates for PI3K pathway targeted therapies.Overall, Millis et al [6] have assessed the frequency of mutations in 47 genes, including PIK3CA, PTEN, and AKT1, in 19,784 consecutive diverse solid tumors from 60 countries using targeted massively parallel sequencing. They identify several cancer types in which PI3K pathway genes are present at high frequency that should be considered as candidates for PI3K pathway-targeted drugs. The use of immunohistochemical analysis to assess PTEN loss is novel, but additional work may be needed to define a scoring threshold that accurately identifies tumors in which PI3K signaling is aberrant. It is unfortunate that outcome information was not available for the Millis et al [6] study and that more PI3K pathway genes (eg, AKT2, PIK3R1) were not sequenced. Future studies might be designed to collect this information in order to further our understanding of the impact of PI3K pathway genes on cancer biology and treatment.REFERENCES1.Cerami E, Gao J, Dogrusoz U, et al. The cBio cancer genomics portal: an open platform for exploring multidimensional cancer genomics data. Cancer Discov. 2012;2(5):401-404.2.Gao J, Aksoy BA, Dogrusoz U, et al. Integrative analysis of complex cancer genomics and clinical profiles using the cBioPortal. Sci Signal. 2013;6(269):pl1.3.Tamborero D, Gonzalez-Perez A, Perez-Llamas C, et al. Comprehensive identification of mutational cancer driver genes across 12 tumor types. Sci Rep. 2013;3:2650.4.Yuan TL, Cantley LC. PI3K pathway alterations in cancer: variations on a theme. Oncogene. 2008;27(41):5497-5510.5.Wong KK, Engelman JA, Cantley LC. Targeting the PI3K signaling pathway in cancer. Curr Opin Genet Dev. 2010;20(1):87-90.lis SZ, Ikeda S, Reddy S, Gatalica Z, Kurzrock R. Landscape of phosphatidylinositol-3-kinase pathway alterations across 19 784 diverse solid tumors. JAMA Oncol. 2016 Jul7. DOI:10.1001/jamaoncol.2016.0891 [Epub ahead of print] DOI: 10.1001/jamaoncol.2016.0875。
马齿苋多糖对小鼠大脑神经细胞代谢损伤修复机制的研究

马齿苋多糖对小鼠大脑神经细胞代谢损伤修复机制的研究康洁【摘要】The method of the primary culture of mouse brain neurons was applied to discuss the protective regenerating mechanism of Portulaca oleracea polysaccharide (POP) on the metabolism damage of mouse brain neurons.. The cultured cells were treated with 20 μmol/L β-amyloid (Aβ), and with 20 μmol/L Aβ plus 10 mmol/L POP combined, respectively. The primarily-cultured cells were observed under an inverted phase contrast microscope. After being double-stained with PI-Hochest, the survival rate of these cells was analyzed. The P35 and P25 gene expression of the treated primarily-cultured cells were detected applying Western blotting.Then the primarily-cultured cells were transfected by pEGFP-tau-s3 and pcDNA-GSK-3β, and by pEGFP-tau-s3, pcDNA-GSK-3β plus POP respectively. 48 h after transfection, these cells were observed under fluorescence and the expression of GSK-3β was detected applying Western blotting. The results showed that the primarily-cultured cells treated with 20 μmol/L Aβ became wizened and some of them died under the inverted phase contrast microscope. According to survival analysis of PI-Hochest double-stained cells. the survival rate of the cells co-treated with Aβ and POP was significantly higher than that only t reated with Aβ. The Aβ treated cells exhibited P25 bands by Western blotting detection, which apparently were weaker in co-treated cells. Through Western blotting, it was found that the tau protein's mobility was higher in the cells co-transfected withpEGFP-tau-s3, pcDNA-GSK-3β plus POP than that transfected with pEGFP- tau-s3 and pcDNA-GSK-3β. Therefore, POP had a protective function of regenerating Aβ damage in the primarily-cultured cells of mouse brain, and it could also decrease the cell dissociation from P35 to P25 cells and the tau protein's phosphorylation by GSK-3β.%为探讨马齿苋多糖(Portulaca oleracea polysaccharide,POP)对小鼠大脑神经细胞代谢损伤的修复保护机制,采用原代培养小鼠大脑神经细胞的方法,分别用20 μmol/L的B淀粉样蛋白(β-amyloid,AB)处理原代培养细胞、20 μmol/L的Aβ加10 mmol/L的POP 共同处理原代培养细胞;对处理的原代细胞进行倒置相差显微镜观察与PI-Hochest 双染色成活分析.用Western bloRing检测处理后的原代细胞P35和P25条带的表达;pEGFP-tau-s3和pcDNA-GSK-3β转染原代培养细胞,pEGFP-tau-s3和pcD-NA-GSK-3β加POP共同转染原代培养细胞,48 h后荧光观察和Westem blotting检测GSK-3β的表达.结果显示,倒置相差显微镜下经20 μmol/L Aβ处理的原代培养细胞出现了皱缩,部分细胞死亡;经P1-Hochest双染色细胞成活分析,Aβ和POP共同处理的原代培养细胞成活率明显高于只用Aβ处理的原代培养细胞:Westem blotting检测到Aβ处理的细胞出现了P25条带.而Aβ加POP共同处理的细胞P25条带明显减弱:Westem blotting检测发现.pEGFP-tau-s3和pcDNA-GSK-3β与POP共转染的细胞中,其tau蛋白的迁移率高于只转染pEGFP-tau-s3和pcDNA-GSK-3β的细胞tau迁移率.所以POP对小鼠大脑原代培养细胞Aβ损伤有修复保护作用.并能减少P35到P25的裂解和GSK-3β对tau 的磷酸化.【期刊名称】《湖北农业科学》【年(卷),期】2011(050)002【总页数】5页(P353-357)【关键词】马齿苋多糖;小鼠;大脑神经细胞;β淀粉样蛋白;tau蛋白;修复机制【作者】康洁【作者单位】商丘师范学院生命科学系,河南商丘,476000【正文语种】中文【中图分类】O539;S865.1+3;Q954.67在早老性痴呆,即阿尔茨海默病(Alzheimer’s disease,AD)退行性疾病中,最显著的病理特征是神经细胞间隙出现大量的β淀粉样蛋白(β-amyloid,Aβ)沉积和神经细胞内出现大量神经原纤维缠结(Neurofibrillary tangles,NFTs),异常磷酸化的 tau 蛋白(一种微管相关的磷酸蛋白)是神经原纤维缠结中的主要组成部分[1]。
分子生物化学常用词汇之三
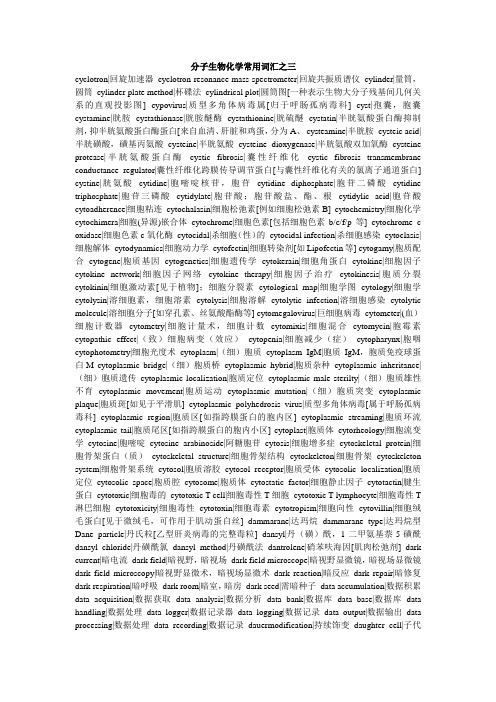
分子生物化学常用词汇之三cyclotron|回旋加速器cyclotron resonance mass spectrometer|回旋共振质谱仪cylinder|量筒,圆筒cylinder plate method|杯碟法cylindrical plot|圆筒图[一种表示生物大分子残基间几何关系的直观投影图] cypovirus|质型多角体病毒属[归于呼肠孤病毒科] cyst|孢囊,胞囊cystamine|胱胺cystathionase|胱胺醚酶cystathionine|胱硫醚cystatin|半胱氨酸蛋白酶抑制剂,抑半胱氨酸蛋白酶蛋白[来自血清、肝脏和鸡蛋,分为A、cysteamine|半胱胺cysteic acid|半胱磺酸,磺基丙氨酸cysteine|半胱氨酸cysteine dioxygenase|半胱氨酸双加氧酶cysteine protease|半胱氨酸蛋白酶cystic fibrosis|囊性纤维化cystic fibrosis transmembrane conductance regulator|囊性纤维化跨膜传导调节蛋白[与囊性纤维化有关的氯离子通道蛋白] cystine|胱氨酸cytidine|胞嘧啶核苷,胞苷cytidine diphosphate|胞苷二磷酸cytidine triphosphate|胞苷三磷酸cytidylate|胞苷酸;胞苷酸盐、酯、根cytidylic acid|胞苷酸cytoadherence|细胞粘连cytochalasin|细胞松弛素[例如细胞松弛素B] cytochemistry|细胞化学cytochimera|细胞(异源)嵌合体cytochrome|细胞色素[包括细胞色素b/c/f/p等] cytochrome c oxidase|细胞色素c氧化酶cytocidal|杀细胞(性)的cytocidal infection|杀细胞感染cytoclasis|细胞解体cytodynamics|细胞动力学cytofectin|细胞转染剂[如Lipofectin等] cytogamy|胞质配合cytogene|胞质基因cytogenetics|细胞遗传学cytokerain|细胞角蛋白cytokine|细胞因子cytokine network|细胞因子网络cytokine therapy|细胞因子治疗cytokinesis|胞质分裂cytokinin|细胞激动素[见于植物];细胞分裂素cytological map|细胞学图cytology|细胞学cytolysin|溶细胞素,细胞溶素cytolysis|细胞溶解cytolytic infection|溶细胞感染cytolytic molecule|溶细胞分子[如穿孔素、丝氨酸酯酶等] cytomegalovirus|巨细胞病毒cytometer|(血)细胞计数器cytometry|细胞计量术,细胞计数cytomixis|细胞混合cytomycin|胞霉素cytopathic effect|(致)细胞病变(效应)cytopenia|细胞减少(症)cytopharynx|胞咽cytophotometry|细胞光度术cytoplasm|(细)胞质cytoplasm IgM|胞质IgM,胞质免疫球蛋白M cytoplasmic bridge|(细)胞质桥cytoplasmic hybrid|胞质杂种cytoplasmic inheritance|(细)胞质遗传cytoplasmic localization|胞质定位cytoplasmic male sterilty|(细)胞质雄性不育cytoplasmic movement|胞质运动cytoplasmic mutation|(细)胞质突变cytoplasmic plaque|胞质斑[如见于平滑肌] cytoplasmic polyhedrosis virus|质型多角体病毒[属于呼肠孤病毒科] cytoplasmic region|胞质区[如指跨膜蛋白的胞内区] cytoplasmic streaming|胞质环流cytoplasmic tail|胞质尾区[如指跨膜蛋白的胞内小区] cytoplast|胞质体cytorheology|细胞流变学cytosine|胞嘧啶cytosine arabinoside|阿糖胞苷cytosis|细胞增多症cytoskeletal protein|细胞骨架蛋白(质)cytoskeletal structure|细胞骨架结构cytoskeleton|细胞骨架cytoskeleton system|细胞骨架系统cytosol|胞质溶胶cytosol receptor|胞质受体cytosolic localization|胞质定位cytosolic space|胞质腔cytosome|胞质体cytostatic factor|细胞静止因子cytotactin|腱生蛋白cytotoxic|细胞毒的cytotoxic T cell|细胞毒性T细胞cytotoxic T lymphocyte|细胞毒性T 淋巴细胞cytotoxicity|细胞毒性cytotoxin|细胞毒素cytotropism|细胞向性cytovillin|细胞绒毛蛋白[见于微绒毛,可作用于肌动蛋白丝] dammarane|达玛烷dammarane type|达玛烷型Dane particle|丹氏粒[乙型肝炎病毒的完整毒粒] dansyl|丹(磺)酰,1-二甲氨基萘-5-磺酰dansyl chloride|丹磺酰氯dansyl method|丹磺酰法dantrolene|硝苯呋海因[肌肉松弛剂] dark current|暗电流dark field|暗视野,暗视场dark field microscope|暗视野显微镜,暗视场显微镜dark field microscopy|暗视野显微术,暗视场显微术dark reaction|暗反应dark repair|暗修复dark respiration|暗呼吸dark room|暗室,暗房dark seed|需暗种子data accumulation|数据积累data acquisition|数据获取data analysis|数据分析data bank|数据库data base|数据库data handling|数据处理data logger|数据记录器data logging|数据记录data output|数据输出data processing|数据处理data recording|数据记录dauermodification|持续饰变daughter cell|子代细胞daughter chromatid|子染色单体daughter chromosome|子染色体daughter colony|子菌落[由原生菌落续发生长的小菌落] daunomycin|道诺霉素daunorubicin|道诺红菌素de novo sequencing|从头测序de novo synthesis|从头合成deactivation|去活化(作用),失活(作用),钝化deacylated tRNA|脱酰tRNA dead time|死时间dead volume|死体积deadenylation|脱腺苷化DEAE Sephacel|[商]DEAE-葡聚糖纤维素,二乙氨乙基葡聚糖纤维素dealkylation|脱烷基化deaminase|脱氨酶deamination|脱氨(基)death phase|死亡期[如见于细胞生长曲线] death point|死点deblocking|去封闭debranching enzyme|脱支酶,支链淀粉酶debris|碎片,残渣decahedron|十面体decane|癸烷decantation|倾析decanting|倾析decapacitation|去(获)能decarboxylase|脱羧酶decarboxylation|脱羧(作用)decay|原因不明腐败decay accelerating factor|衰变加速因子decay constant|衰变常数deceleration phase|减速期[如见于细胞生长曲线] dechlorination|脱氯作用deciduous leaf|落叶decline phase|[细胞生长曲线的]衰亡期decoagulant|抗凝剂decoding|译码,解码decomposer|分解者[可指具有分解动植物残体或其排泄物能力的微生物] decompression|降压,减压decondensation|解凝(聚)decontaminant|净化剂,去污剂decontaminating agent|净化剂,去污剂decontamination|净化,去污decorin|核心蛋白聚糖[一种基质蛋白聚糖,又称为PG-40] dedifferentiation|去分化,脱分化deep colony|深层菌落deep etching|深度蚀刻deep jet fermentor|深部喷注发酵罐deep refrigeration|深度冷冻deep shaft system|深井系统[如用于污水处理] defasciculation factor|解束因子[取自水蛭,可破坏神经束] defective|缺损的,缺陷的defective interfering|缺损干扰defective interfering particle|缺损干扰颗粒,干扰缺损颗粒defective interfering RNA|缺损干扰RNA defective interfering virus|缺损干扰病毒defective mutant|缺损突变体,缺陷突变型,缺陷突变株defective phage|缺损噬菌体,缺陷噬菌体defective virus|缺损病毒,缺陷病毒defense|防御,防卫defense peptide|防卫肽defense response|防御反应,防卫反应defensin|防卫素[动物细胞的内源性抗菌肽] deficiency|缺乏,缺损,缺陷deficient|缺少的,缺损的,缺陷的defined|确定的defined medium|确定成分培养基,已知成分培养液defintion|定义defoliating agent|脱叶剂defoliation|脱叶deformylase|去甲酰酶[见于原核细胞,作用于甲酰甲硫氨酸] degasser|脱气装置degassing|脱气,除气degeneracy|简并;简并性,简并度degenerate|简并的degenerate codon|简并密码子degenerate oligonucleotide|简并寡核苷酸degenerate primer|简并引物degenerate sequence|简并序列degeneration|退化,变性degenerin|退化蛋白[与某些感觉神经元的退化有关] deglycosylation|去糖基化degradable polymer|降解性高分子degradation|降解degranulation|脱(颗)粒(作用)degree of acidity|酸度degree of dominance|显性度degree of polymerization|聚合度degron|降解决定子[决定某一蛋白发生降解或部分降解的序列要素] deguelin|鱼藤素dehalogenation|脱卤(作用)dehardening|解除锻炼dehumidifier|除湿器dehydratase|脱水酶dehydrated medium|干燥培养基dehydration|脱水(作用)dehydroepiandrosterone|脱氢表雄酮dehydrogenase|脱氢酶dehydrogenation|脱氢(作用)dehydroluciferin|脱氢萤光素deionization|去离子(作用)deionized|去离子的deionized water|去离子水deionizing|去离子(处理)delayed early transcription|(延)迟早期转录[可特指病毒] delayed fluorescence|延迟荧光delayed heat|延迟热delayed hypersensitivity|延迟(型)超敏反应delayed ingeritance|延迟遗传delayed type hypersensitivity|迟发型超敏反应deletant|缺失体deletion|缺失deletion mapping|缺失定位,缺失作图deletion mutagenesis|缺失诱变deletion mutant|缺失突变体deletion mutantion|缺失突变deletional recombination|缺失重组delignification|脱木质化(作用)deliquescence|潮解delivery flask|分液瓶delocalized bond|离域键deltorphin|delta啡肽[见于叶泡蛙皮肤,对delta阿片样肽受体的亲和力和选择性很强] demasking|解蔽demethylation|脱甲基化demineralized water|脱矿质水demulsifier|破乳剂demyelination|脱髓鞘denaturant|变性剂denaturant gel|变性凝胶denaturation|变性(作用)denatured|变性的denatured DNA|变性DNA denatured protein|变性蛋白(质)denaturing gel|变性凝胶denaturing gel electrophoresis|变性凝胶电泳denaturing gradient polyacrylamide gel|变性梯度聚丙烯酰胺凝胶denaturing polyacrylamide gel|变性聚丙烯酰胺凝胶dendrite|树突dendritic cell|树状细胞dendrotoxin|树眼睛蛇毒素dengue virus|登革病毒denitrification|反硝化作用denitrifying bacteria|反硝化细菌denitrogen|排氮densimeter|密度计densitometer|光密度计densitometry|光密度(测定)法density|密度density gradient|密度梯度density gradient centrifugation|密度梯度离心density gradient electrophoresis|密度梯度电泳densovirus|浓核病毒dentritic cell|树枝状细胞Denver system|[染色体组型]丹佛体制deoxyadenosine|脱氧腺苷deoxyadenosine moniphosphate|脱氧腺苷(一磷)酸deoxyadenosine triphosphate|脱氧腺苷三磷酸deoxyadenylate|脱氧腺苷酸deoxyadenylic acid|脱氧腺苷酸deoxycholate|脱氧胆酸盐deoxycorticosterone|脱氧皮质酮deoxycytidine|脱氧胞苷deoxycytidine monophosphate|脱氧胞苷(一磷)酸deoxycytidine triphosphate|脱氧胞苷三磷酸deoxycytidylate|脱氧胞苷酸deoxycytidylic acid|脱氧胞苷酸deoxyformycin|脱氧型霉素deoxygenation|脱氧deoxyglucose|脱氧葡萄糖deoxyguanosine|脱氧鸟苷deoxyguanosine monophosphate|脱氧鸟苷(一磷)酸deoxyguanosine triphosphate|脱氧鸟苷三磷酸deoxyguanylate|脱氧鸟苷酸deoxyguanylic acid|脱氧鸟苷酸deoxyhemoglobin|脱氧血红蛋白,去氧血红蛋白deoxyinosine|脱氧肌苷,脱氧次黄苷deoxyinosine triphosphate|脱氧肌苷三磷酸,脱氧次黄苷三磷酸deoxynivalenol|脱氧萎镰菌醇deoxynucleoside|脱氧核苷deoxynucleoside methylphosphonate|脱氧核苷膦酸甲酯deoxynucleoside phosphoramidite|脱氧核苷亚磷酰胺deoxynucleotide|脱氧核苷酸deoxyriboaldolase|脱氧核糖醛缩酶deoxyribomutase|脱氧核糖变位酶deoxyribonuclease|脱氧核糖核酸酶,DNA酶[包括DNA酶I、DNA酶II等] deoxyribonucleic acid|脱氧核糖核酸deoxyribonucleoside|脱氧(核糖)核苷deoxyribonucleoside diphosphate|脱氧(核糖)核苷二磷酸deoxyribonucleoside hydrogenphosphonate|脱氧(核糖)核苷氢膦酸酯deoxyribonucleoside methylphosphonate|脱氧(核糖)核苷膦酸甲酯deoxyribonucleoside monophosphate|脱氧(核糖)核苷(一磷)酸deoxyribonucleoside phosphoramidite|脱氧(核糖)核苷亚磷酰胺deoxyribonucleoside triphosphate|脱氧(核糖)核苷三磷酸deoxyribonucleotide|脱氧(核糖)核苷酸deoxyribose|脱氧核糖deoxysugar|脱氧糖deoxythymidine|脱氧胸腺嘧啶核苷,脱氧胸苷deoxythymidine monophosphate|脱氧胸苷(一磷)酸deoxythymidine triphosphate|脱氧胸苷三磷酸deoxythymidylate|脱氧胸苷酸deoxythymidylic acid|脱氧胸苷酸deoxyuridine|脱氧尿苷deoxyuridine monophosphate|脱氧尿苷(一磷)酸deoxyuridylate|脱氧尿苷酸deoxyuridylic acid|脱氧尿苷酸depactin|[肌动蛋白]蚕食蛋白dependovirus|依赖病毒(属)[模式成员是腺伴随病毒] dephosphorylation|去磷酸化(作用)deplasmolysis|质壁分离复原depletion|耗竭,除尽depolarization|去极化,脱极化;解偏振(作用)depollution|去污染depolymerase|解聚酶depolymerization|解聚deposit|沉积;沉积物deposition|沉积(作用);沉积物depressed|脱阻抑的depression|脱阻抑;压抑deprivation|除去,丧失deprived|除去的,丧失的depropagation|负增长反应,逆增长反应deprotection|脱保护deprotonation|去质子化,脱质子化depurination|脱嘌呤(作用)derepressed|脱阻抑的derepression|脱阻抑derivative|衍生物derivatization|衍生化(作用)derivave spectrum|导数光谱dermatan sulfate|硫酸皮肤素dermatogen|表皮原dermatoglyphics|肤纹学dermatonecrotoxin|皮肤坏死毒素dermatophagoides|嗜皮螨属dermenkaphaline|皮脑啡肽dermis|真皮dermorphin|皮啡肽desalting|脱盐desaminase|脱氨酶desaturase|脱饱和酶,脱氢酶desaturation|脱饱和,去饱和descending development|下行展开(法)descending facilitatory system|下行易化系统[用于神经生物学] descending inhibition|下行抑制descending inhibitory system|下行抑制系统desensitization|脱敏(作用)desetope|(决定簇)选择位[抗原呈递中,II类主要组织相容性复合体与抗原相互作用的部位] desiccant|干燥剂desiccation|干燥(作用)desiccator|干燥器,保干器desiccator cabinet|干燥橱desmin|结蛋白desmocalmin|桥粒钙调(蛋白)结合蛋白,桥粒钙蛋白desmocollin|桥粒(芯)胶(粘)蛋白[见于桥粒芯的胶粘层,是桥粒芯蛋白的剪接变体] desmoglea|桥粒芯[桥粒的中央核心层] desmoglein|桥粒芯(糖)蛋白[一类跨膜蛋白,见于桥粒芯] desmolase|碳链酶desmoplakin|桥粒斑蛋白desmosine|锁链素[由四个赖氨酸侧链形成的交联体,见于弹性蛋白] desmosome|桥粒desmosome plaque|桥粒斑desmotubule|连丝微管desmoyokin|桥粒联结蛋白[见于桥粒斑] desolvation|去溶剂化desorption|解析(作用),脱附(作用)desorption ionization|解析电离destabilization|去稳定作用destabilizing agent|去稳定剂destaining|脱色destomycin|越霉素desulfurization|脱硫(作用)desynchronization|去同步化detachment|解析(作用),脱附(作用detection|检测,检出;观察detection limit|检出限detector|检测器detention|滞留detention time|滞留时间detergent|去污剂,除垢剂,洗涤剂deterioration|(生物)致劣,恶化determinant|决定簇;决定子[用于胚胎学];决定因素determination|测定determination limit|测定限detoxication|解毒detoxification|解毒detritus|碎屑;腐屑detritylation|脱(除)三苯甲基deuteromycetes|半知菌纲deutertion|氘化deutoplasm|滋养层,卵黄质developing solvent|展开剂developing tank|展开槽development folder|显影夹developmental biology|发育生物学developmental genetics|发育遗传学developmental malformation|发育畸形developmental phase|发育期developmental regulation|发育调节,发育调控developmental rhythm|发育节律developmental stage|发育阶段developmentally regulated expression|由发育(所)调节的表达devernalization|脱春化dexamethasone|地塞米松,9-alpha-氟-16-甲基脱氢皮质醇dextran|葡聚糖,右旋糖酐dextran bead|葡聚糖珠dextran sulfate|葡聚糖硫酸酯dextranase|葡聚糖酶dextrin|糊精dextroisomer|右旋异构体dextrorotary|右旋的dextrorotatory|右旋的dextrose|葡聚糖diabetes mellitus|糖尿病diacetoxyscirpenol|蛇形菌素diacylglycerol|二酰甘油diad|二分体,二联体diadric|雄异配(性)的diafiltration|渗滤[溶液通过滤器进行连续循环] diagnostic enzyme|诊断酶diagnostic kit|诊断试剂盒diagnostic procedure|诊断程序,诊断手续diagnostic reagent|诊断试剂diagnostics|诊断学diagonal|对角线;对角线的diagonal chromatography|对角线层析diagonal electrophoresis|对角线电泳diagynic|雌异配(性)的diakinesis|终变期dialkylglycine|二烷基甘氨酸dialkylglycine decarboxylase|二烷基甘氨酸脱羧酶diallel cross|双列杂交[用于进化遗传学] dialysate|透析物,透析液dialysis|透析dialysis apparatus|透析仪,透析装置dialysis bag|透析袋dialysis cultivation|透析培养dialysis fermentation|透析发酵dialysis membrane|透析膜dialysis tube|透析袋dialysis tubing|透析袋,透析管dialyzable|可透析的dialyzate|透析液dialyzator|透析仪diamagnetic compound|抗磁化合物diamagnetism|抗磁性diaminedichloroplatinum|二胺二氯铂diaminobenzidine|二氨基联苯胺diaminopimelate|二氨基庚二酸,二氨基庚二酸盐、酯、根diaminopimelic acid|二氨基庚二酸dianisidine|邻联茴香胺dianthovirus|香石竹病毒组[一组植物病毒,模式成员是香石竹环斑病毒] diapause hormone|滞育激素diapedesis|血细胞渗出[血细胞从血管内渗出] diaphragm|隔膜diarrhea|腹泻diastase|淀粉酶制剂diastereomer|非对映(异构)体diastereotopic|非对映异位的[在分子整体中,碳原子上互为非对映关系的原子、基团或面] diastole|心舒期diatomaceous earth|硅藻土diatrizoate|3,5-双(乙酰氨基)-2,4,6-三碘苯甲酸(盐)diauxie growth curve|双峰生长曲线diazo|重氮基diazo compound|重氮化合物diazoacridine|重氮吖啶diazobenzyloxymethyl paper|重氮苄氧甲基纸,DBM纸diazonorleucine|重氮基正亮氨酸diazophenylthio paper|重氮苯硫醚纸,DPT纸diazotization|重氮化diazouridine|重氮尿苷dibucaine|狄步卡因dicarboxyl cellulose|二羧基纤维素dicarboxylic acid|二羧酸dicarboxylicamino acid|二羧基氨基酸dicentric chromosome|双着丝染色体dichlorodimethylsilane|二氯二甲硅烷dichlorofluorescein|二氯荧光黄dichloromethane|二氯甲烷dichlorovos|敌敌畏dichogamy|雌雄(蕊)异熟dichroism|二色性Dick test|狄克试验[链球菌红斑毒素的皮肤试验] dicotyledons|双子叶植物dicoumarin|双羟香豆素,败坏翘摇素dictyosome|(分散)高尔基体dictyostelium|盘基网柄菌属,网柄菌属dicyclohexylcarbodiimide|二环己基碳二亚胺[常用缩合剂] didanosine|[商]2',3'-双脱氧肌苷didehydrothymidine|双脱氢胸苷dideoxy sequencing method|双脱氧测序法dideoxyadenosine triphosphate|双脱氧腺苷三磷酸dideoxycytidine|双脱氧胞苷dideoxycytidine triphosphate|双脱氧胞苷三磷酸dideoxyguanosine|双脱氧鸟苷dideoxyguanosine triphosphate|双脱氧鸟苷三磷酸dideoxyinosine|双脱氧肌苷dideoxyribonucleoside|双脱氧核苷dideoxyribonucleoside triphosphate|双脱氧核苷三磷酸dideoxythymidine triphosphate|双脱氧胸苷三磷酸dielectric constant|介电常数dielectric effect|介电效应dielectrometric titration|介电(常数)滴定(法)dielectrometry|介电(常数)滴定(法)dielectrophoresis|介电(电)泳diene|双烯diethyl pyrocarbonate|焦碳酸二乙酯diethyl sulfate|硫酸二乙酯diethylstilbestrol|乙酚,二乙基己烯雌酚difference spectrum|差光谱differential|(示)差的,鉴别的;微分;微分的differential analysis|示差分析differential centrifugation|差速离心differential detection|示差检测,鉴别检测differential expression|差异表达differential flotation centrifugation|差速浮式离心differential hybridization|示差杂交(法)differential medium|鉴别培养基differential operation|示差操作differential permeability|差别透性,选择透性differential precipitation|示差沉淀differential refractive index detector|示差折光率检测器differential scattering|差散射differential screening|示差筛选differential sedimentation|差速沉降differential sepctrophotometry|示差分光光度法differential species|区别种differential spectrum|(示)差光谱differential staining|鉴别染色(法)differential staining technique|鉴别染色技术[有时特指染色体显带技术] differential type detector|微分型检测仪differentiating solvent|鉴别剂,区分溶剂differentiation|分化differentiation antigen|分化抗原differentiation center|分化中心differentiation phase|分化时diffraction|衍射diffraction grating|衍射光栅diffraction symmetry|衍射对称性diffuser|扩散器;洗料器;[发酵罐]进气装置diffusion|扩散diffusion chamber|扩散盒,扩散小室diffusion coefficient|扩散系数diffusion controlled reaction|扩散控制(的)反应diffusion controlled termination|扩散控制的终止diffusional limitation|扩散限制diffusional resistance|扩散阻力diformazan|二甲digalactosyl diglyceride|双半乳糖甘油二酯digester|消化器,消化罐digestion|消化,(酶切)消化digestive enzyme|消化酶digital control|数字控制digital imaging microscope|数字成像显微镜digital imaging microscopy|数字成像显微术digitalis cardiac glycoside|毛地黄(类)强心苷digitalizer|数字化仪[用于计算机科学] digitonin|毛地黄皂苷digitoxigenin|毛地黄毒苷配基;beta-(丁烯酸内酯)-14-羟甾醇digitoxin|毛地黄毒苷diglyceride|甘油二酯digoxigenin|洋地黄毒苷,地高辛配基digoxin|异羟基洋地黄毒苷原,地高辛dihaploid|双单倍体dihedral angle|二面角,双面角dihydrobiopterin|二氢生物蝶呤dihydrochalcone|双氢查耳酮,二氢查耳酮dihydrofolate|二氢叶酸dihydrofolate reductase|二氢叶酸还原酶dihydrolipoamide|二氢硫辛酰胺dihydrolipoamide dehydrogenase|二氢硫辛酰胺脱氢酶dihydrolipoic acid|二氢硫辛酸dihydroorotase|二氢乳清酸酶dihydroorotate|二氢乳清酸dihydropteridine|二氢蝶啶dihydropteridine reductase|二氢蝶啶还原酶dihydropyridine|二氢吡啶dihydrotestosterone|双氢睾酮dihydrouracil|二氢尿嘧啶dihydrouracil arm|二氢尿嘧啶臂dihydrouracil loop|二氢尿嘧啶环dihydrouridine|二氢尿苷dihydroxyacetone phosphate|二氢丙酮磷酸dihydroxycholecalciferol|二羟胆钙化(固)醇dihydroxyphenylanaline|二羟苯丙氨酸,多巴dihydroxyphenylethylamine|二羟苯基乙胺,羟酪胺,多巴胺diisopropylfluorophosphate|二异丙基氟磷酸dikaryon|双核体diltiazem|硫氮酮diluent|稀释剂,稀释液dilution cloning|稀释克隆法[如用于获得细胞克隆株] dimensional electrophoresis|双向电泳dimer|二聚体dimerization|二聚化dimerization cofactor|二聚化辅因子dimethoxytrityl|二甲氧三苯甲基[在DNA合成中用作羟基保护剂] dimethyl sulfate|硫酸二甲酯dimethyl sulfoxide|二甲基亚砜dimethylallylpyrophosphate|二甲(基)烯丙基焦磷酸dimethylaminoazobenzene|二甲基氨基偶氮苯dimethylformamide|二甲基甲酰胺dimorphism|二态二氢,双态现象dinitro benzene|二硝基苯dinitrochlorobenzene|二硝基氯苯dinitrofluorobenzene|二硝基氟苯dinitrogen|双氮,分子氮dinitrogenase|固氮酶dinitrogenase reductase|固氮酶还原酶dinitrophenol|二硝基苯酚dinitrophenyl|二硝基苯基dinoflagellate|甲藻dinoxanthine|甲藻黄素dinucleotide frequency|二核苷酸频率dinucletide|二核苷酸diodeelectrode|二极管电极dioecism|雌雄异体,雌雄异株diosgenin|薯蓣皂苷配基,薯蓣皂苷元dioxide|二氧化物dioxygen|双氧dioxygenase|双加氧酶dipeptidase|二肽酶dipeptide|二肽diphenylamine blue|二苯胺蓝diphenyloxazole|二苯基唑diphosphate|二磷酸diphosphatidylglycerol|双磷脂酰甘油diphosphoglycerate|二磷酸甘油酸diphosphoglycerate shunt|二磷酸甘油酸支路diphosphoinositide|二磷酸肌醇磷脂,磷脂酰肌醇磷酸diphthamide|白喉酰胺diphthera toxin|白喉毒素dipicolinic acid|2,6-吡啶二羧酸diplobacillus|双杆菌diploblastic|双胚层的diplococcus|双球菌diplococcus pneumoniae|肺炎双球菌diploid|二倍体diploid cell line|二倍体细胞系diploidization|二倍化diploidy|二倍性diplonema|双线期diplotene stage|双线期dipolar aprotic solvent|偶极非质子溶剂dipolar protophilic solvent|偶极亲质子溶剂dipolar protophobic solvent|极疏质子溶剂dipole|偶极dipole molecule|偶极分子dipole moment|偶极矩dipyrromethane|联吡咯甲烷direct cross|正交direct duplication|同向重复direct insertion|同向插入direct repeat|同向重复(序列)direct selection|正选择[使用只有突变体或重组体能生长的条件进行选择] directed cloning|定向克隆directed mutagenesis|定向诱变directed perturbation|定向微扰directed sequencing|定向测序direction selectivity|方向选择性directional cloning|定向克隆disaccharide|二糖disassembly|解装配,分解disc electrophoresis|圆盘电泳disc gel electrophoresis|圆盘凝胶电泳disc membrane|圆盘膜discharge|放电;卸下discoidal cleavage|盘状卵裂discontinuous epitope|非连续表位discontinuous gradient|不连续梯度discontinuous replication|不连续复制discontinuous variation|不连续变异discontinuous zone electrophoresis|不连续区带电泳discrete|分立的,不连续的discriminant analysis|判别分析discrimination|辨别,判别disease association|疾病相关disease resistance|抗病性dish|平皿disinfectant|消毒剂disinfection|消毒disinfestation|灭虫disinhibition|去抑制disintegration|蜕变,衰变;去整合,解整合;分解,破碎disintegrator|粉碎器,粉碎机disjunction|分离disk centrifuge|圆盘(式)离心机[带有成叠的有孔圆盘,常用于溶液的澄清化处理] dislocation|脱位,转位,位错dismutase|岐化酶disome|二体,双体disordered state|无序状态disordered structure|无序结构dispase|分散酶,中性蛋白酶[用于分散组织培养中的动物细胞] dispenser|分液器dispermy|双精人卵dispersant|分散剂disperse medium|分散介质disperse phase|分散相disperse system|分散系统dispersion|分散;色散dispersion force|分散力;色散力dispersion spectrum|色散谱displaced loop|替代环displacement|顶替,替代,置换displacement analysis|顶替(分析)法displacement chromatography|顶替层析displacement electrophoresis|顶替电泳displacement loop|替代环,D 环[形如英文字母D] displacement reaction|置换反应disposable glove|一次性手套disposable microcentrifuge tube|一次性(使用的)微量离心管disposable tip|一次性(使用的)吸头disproportionation|岐化(反应)disrotatory|对旋disruption|破裂,破坏dissecting microscope|解剖显微镜dissection|解剖,剖分disseminated intravascular coagulation|弥漫性血管内凝血dissimilation|异化(作用)dissociation|解离,离解dissociation constant|解离常数dissolvability|溶(解)度,(可)溶(解)性dissolvant|溶剂distamycin|偏端霉素distance receptor|距离感受器distant hybirdization|远缘杂交distant hybrid|远缘杂种distorted peak|畸峰diterpene|双萜,二萜dithioerythritol|二硫赤藓糖醇dithiothreitol|二硫苏糖醇divergence|分散[用于神经系统];趋异divergent|趋异进化diverse ion effect|异离子效应diversity gene|D基因[为D区编码的基因] diversity region|多变区,D区[免疫球蛋白等分子重链的一个高变区] divinylbenzene|二乙烯苯dizygotic twins|二卵双生,异卵双生DNA adduct|DNA加合物DNA amplification in vitro|DNA体外扩增DNA amplification polymorphism|DNA扩增多态性DNA bending|DNA转折,DNA弯曲DNA blotting|DNA印迹(法)DNA catenation|DNA连环DNA circle|DNA环[指环状DNA] DNA cleavage|DNA裂解,DNA切割DNA cloning|DNA克隆(化)DNA jumping technique|DNA跳查技术DNA ladder|DNA梯[如大小不同的标准参照物的电泳谱] DNA nicking|DNA切口形成DNA pitch|DNA螺距DNA sizing|DNA大小筛分DNA sizing gene|DNA大小决定基因[如见于噬菌体,可决定所包装的DNA量] DNA typing|DNA 分型DNAase|DNA酶DNAase I footprinting|DNA酶足迹法docking|停靠docking protein|船坞蛋白,停靠蛋白[内质网上与信号识别颗粒相互作用从而使蛋白质继续翻译的蛋白] dodecahedron|十二面体dodecane|十二烷dodecapeptide motif|十二肽基序dodecyl|十二烷基dolichol|多萜醇,长醇domain|域,区域,结构域,功能域domain assmbly|结构域装配domain deletion|结构域删除domain substitute|结构域置换dominance|显性;优势(度)dominance variance|显性方差dominant|显性的,优势的dominant acting gene|显性开放基因dominant allele|显性等位基因dominant gene|显性基因dominant hemisphere|优势半球dominant interference|显性干涉dominant lethal|显性致死dominant mutation|显性突变dominant negative|显性阴性的,显性失活的dominant negative mutant|显性失活突变体dominant oncogenic|显性致癌的Donnan dialysis|唐南透析Donnan equilibrium|唐南平衡Donnan potential|唐南膜电势donor|供体,给体donor splicing site|剪接供体dopamine|多巴胺dosage compensation|计量补偿(效应)dot blot|斑点印迹,斑点印迹膜dot blotting|点渍法,斑点印迹(法)dot hybridization|斑点杂交dotting|打点,打点杂交double antibody method|双抗体法[免疫测定方法之一种] double balloon catheter|双气囊导管double bar|重棒眼,双棒眼,超棒眼[黑腹果蝇唾液腺染色体的X染色体上16A区段重复三次而出现的特殊表型] double beam mass spectrometer|双束质谱仪double beam spectrophotometer|双光束分光光度计double blind trial|双盲试验double bond migration|双键移位double coupling method|双偶联法,双偶合法double decomposition reaction|复分解double exchange|双交换double fertilization|双受精double focusing|双聚焦double focusing mass spectrometer|双聚焦质谱仪double helix|双螺旋double immunodiffusion|双向免疫扩散,免疫双扩散double innervation|双重神经支配double labeling|双重标记double minute chromosome|双微染色体[所携带基因得到扩增的成对额外小染色体] double recessive|双隐性double resonance|双共振doublet|双联体;双峰doubling time|倍增时间[培养物的生物质翻一番所需的时间] Dower resin|Dower 树脂[陶氏化学公司离子交换层析介质商品名] down promoter mutation|启动子减效突变downflow fixed bed|下流固定床doxorubicin|阿霉素drift|(遗传)漂变drilling mud|钻探泥浆drinking center|饮水中枢drop method|点滴法droplet countercurrent chromatography|液滴反流层析,液滴逆流层析dropping mercury electrode|滴汞电极Drosophila|果蝇属drug susceptibility|药物敏感性drug targeting|药物寻靶,药物导向Duchenne muscular dystrophy|Duchenne型肌营养不良,假肥大型肌营养不良duocrinin|促十二指肠液素duplex|双链体;双螺旋;二显性组合duplicon|重复子duramycin|耐久霉素dwarf colony|侏儒型菌落dwarf plant|矮化植物[由遗传因素决定不能长高];矮生植物[由认为措施或特殊环境决定不能长高] dyad|二分体,二联体dyad symmetry|二重对称dye exclusion test|染料排斥试验[用于检查细胞生活力] dynactin|动力蛋白激活蛋白dynamin|发动蛋白dynein|动力蛋白dynein arm|动力蛋白臂dynorphin|强啡肽dysbacteria|菌群失调dysbacteriosis|菌群失调dysentery bacillus|痢疾杆菌dysfunction|功能异常,机能障碍dysregulation|调节异常dystroglycan|(肌)营养不良(蛋白)聚糖[与肌)营养不良蛋白相关的蛋白聚糖] dystrophin|(肌)营养不良蛋白E rosette|E(玫瑰)花结[E表示红细胞erythrocyte] E rosette test|E(玫瑰)花结试验EA rossette|EA(玫瑰)花结[E表示红细胞erythrocyte,A表示抗体antibody] EAC rossette|EAC(玫瑰)花结[E表示红细胞erythrocyte,A表示抗体antibody,C表示补体complement] Eadie plot|Eadie图Eadie plotting|Eadie作图法[用于酶促反应动力学] early gene|早期基因[可特指病毒] early hypersensitivity|早发型超敏反应early phage|早期[有时特指病毒复制的早期] early promoter|早期启动子[有时特指病毒] early protein|早期蛋白[有时特指病毒] early transcription|早期转录[有时特指病毒] eburicoic acid|齿孔酸ecdysis|蜕皮ecdyson|蜕皮激素[见于昆虫等节肢动物] ecdyson response element|蜕皮激素效应元件ecdysteroid hormone|蜕皮类固醇激素[见于血吸虫] echinomycin|棘霉素echinonectin|海胆粘连蛋白echiststin|锯鳞(蝮蝰)血抑(环)肽[可抑制血小板凝集] ECHO virus|ECHO病毒,艾柯病毒eclipse period|[细胞生长的]隐蔽期eclipsed conformation|重叠构象eclosion hormone|蜕壳激素[见于昆虫] ecological isolation|生态隔离ecology|生态学ecosphere|生态圈ecotropic retrovirus|亲嗜性逆转录病毒[只能在原始宿主细胞引起产毒性感染] ecotype|生态型ecteinascidin|海鞘素ectendomycorrhiza|内外生菌根ectoderm|外胚层ectodesma|胞外连丝ectodesmata|(复)胞外连丝ectodomain|胞外(结构)域ectoenzyme|胞外酶ectomycorrhiza|外生菌根ectopic|异位的ectopic amplification|异位扩增[引物与非目标序列结合而引导的PCR扩增] ectopic expression|异位表达ectopic hormone|异位激素[不是由原内分泌腺所产生的激素] ectopic insertion|异位插入ectoplasm|外质ectoplast|外质体ectosarc|外质ectospore|外生孢子;(孢子)表壁ectotoxin|外毒素eddy diffusion|涡流扩散edeine|伊短菌素edema|水肿edge effect|边缘效应editing|编辑[如RNA编辑,核酸外切编辑等] editosome|编辑体[进行RNA编辑的场所] Edman chemistry|Edman化学(原理)Edman degradation|Edman 降解(法)[连续测定蛋白质N端氨基酸序列的经典方法] Edman stepwise degradation|Edman 分步降解(法)EF hand|EF手[由29个氨基酸组成的钙结合基序,含E、F两段螺旋,并形成螺旋-环-螺旋effective nuclear charge|有效核电荷effective population size|有效群体大小,有效种群大小effective quantum number|有效量子数effector|效应子,效应物,效应器,效应基因effector cell|效应细胞effector function|效应子功能effector molecule|效应分子effector site|效应物部位effector T cell|效应(性)T细胞efferent nerve|传出神经efficiency of plating|成斑效率efficient|高效的,有效的efflorescence|风化effluent|流出物,流出液efflux|外向通量;流(出)量;流出egg nucleus|卵核eglin|水蛭(蛋白酶)抑制剂[可抑制弹性蛋白酶、组织蛋白酶G 等] eicosane|二十碳烷eicosanoic acid|二十(烷)酸eicosanoid|类二十烷酸,类花生酸[指白细胞三烯,前列腺素、凝血烷等激素] eicosanol|二十碳醇eicosapentaenoic acid|十二碳五烯酸elaidic acid|反油酸,反-9-十八碳单烯酸elaioplast|油质体elastase|弹性蛋白酶elastatinal|弹性(蛋白)酶抑制剂elastic fiber|弹性纤维elastin|弹性蛋白elastoma|高弹性electric birefringence|电双折射electric conductance|电导electric conductivity|电导,电导率,电导性electric coupling|电偶合electric dehydration|电脱水(作用)electric double layer|电双层electric field|电场electric field flow fractionation|电场流分级(分离)electric impluse|电脉冲electric potential|电势,电位electric tape|绝缘胶布,绝缘胶带electrical stimulator|电刺激器electrical synapse|电突触electrical transmission|电传递electrically facilitated flow|电易化流动,电促流动electroblotting|电印迹electroblotting device|电印迹仪,电印迹装置electrocardiogram|心电图electrocatalysis|电催化electrochemical gradient|电化学梯度electrochemical proton gradient|质子电化学梯度electrochemical reaction|电化学反应electrochemical sensor|电化学传感器electrochemiluminescence|电化学发光electrode|电极electrode potential|电极电势electrodeionization|电去离子,电脱离子electrodialysis|电透析electroeluate|电洗脱物electroelution|电洗脱electroencephalogram|脑电图electroendoosmosis|电内渗electrofusion|电融合electrogenic ion pump|生电离子泵electrogenic pump|生电泵electrogenic sodium pump|生电钠泵electroimmunoassay|电免疫测定(法)electroimmunodiffusion|电免疫扩散electrokinetic separation technique|动电分离技术electrokinetic ultrafiltration analysis|动电超滤分析electroluminescence|电致发光electrolysis|电解electrolyte|电解质electrolyte solution|电解质溶液electrolytic cell|电解池electromagnetic radiation|电磁辐射electromorph|电泳异型酶[可通过电泳区分的同工酶] electromotive force|电动势electromyogram|肌电图electron acceptor|电子受体,电子纳体electron capture|电子俘获electron cloud|电子云electron collision|电子碰撞electron cryomicroscopy|冷冻电(子显微)镜术electron crystallography|电子晶体学electron density|电子密度electron diffraction|电子衍射electron donor|电子给体,电子供体electron flow|电子流,电子传递electron impact|电子碰撞electron impact desorption|电子碰撞解吸electron impact ion source|电子碰撞离子源electron ionization|电子电离electron microscope|电(子显微)镜electron microscopy|电(子显微)镜术electron multiplier|电子倍增器electron pair|电子对electron pair relay system|电子对中继系统electron pair repulsion|电子对互斥electron paramagnetic resonance|电子顺磁共振electron scattering|电子散射electron shell repulsion|电子层推斥electron shuttle|电子穿梭electron spectroscopy|电子能谱学electron spectroscopy for chemical analysis|光电子能谱(学),电子光谱(法)electron spin|电子自旋electron spin resonance|电子自旋共振electron spin resonance imaging|电子自旋共振成像electron stain|电子染色electron transfer|电子传递,电子转移electron transfer reaction|电子传递反应electron transfer system|电子传递系统electron transport|电子传递electron transport chain|电子传递链electron volt|电子伏特electronegativity|电负性electronic balance|电子天平electronic controlled incubator|电子控制培养箱electronic effect|电子效应electronic energy level|电子能级electronic quenching|电子猝灭electronic spectrum|电子光谱electroosmosis|电渗electroosmotic flow|电渗流electroosmotic mobility|电渗迁移率electrophile|亲电体electrophilic addition|亲电加成electrophilic reagent|亲电(子)试剂electrophilic rearrangement|亲电重排electrophilic substitution|亲电取代electrophobic reagent|疏电(子)试剂electrophoresis|电泳electrophoresis apparatus|电泳仪,电泳装置electrophoresis buffer|电泳缓冲液electrophoresis chamber|电泳槽electrophoresis pattern|电泳图谱electrophoresis tank|电泳槽electrophoretic analysis|电泳分析electrophoretic band|电泳条带electrophoretic buffer|电泳缓冲液electrophoretic force|电泳力electrophoretic light scattering|电泳光散射electrophoretic medium|电泳介质electrophoretic migration|电泳迁移electrophoretic mobility|电泳迁移率electrophoretic mobility shift assay|电泳迁移率变动分析electrophoretic property|电泳性质electrophoretic separation|电泳分离electrophoretic technique|电泳技术electrophoretic transfer|电泳转移(法)electrophoretically pure|电泳纯的electrophoretogram|电泳图(谱)electrophoretype|电泳型[根据病毒核酸的电泳谱而划分的型,如见于轮状病毒] electropolarization|电极化electropolarization chromatography|电极化层析electroporation|电穿孔(法)electroporation apparatus|电穿孔仪electrospray|电喷射electrospray ionization|电喷射离子化(作用)electrospray mass spectroscopy|电喷射质谱学electrostatic attraction|静电引力electrostatic effect|静电效应electrostatic field|静电场electrostatic interaction|静电作用electrostatic potential|静电势electrostatic repulsion|静电推斥electrotaxis|趋电性。
生物化学名词解释大全
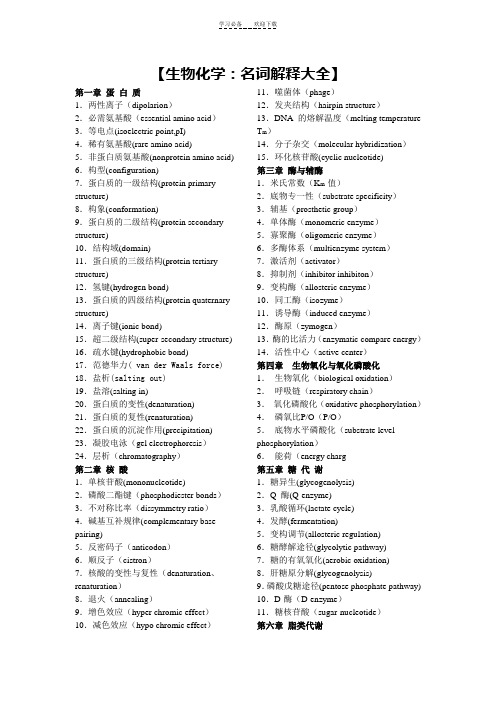
学习必备欢迎下载【生物化学:名词解释大全】第一章蛋白质1.两性离子(dipolarion)2.必需氨基酸(essential amino acid)3.等电点(isoelectric point,pI)4.稀有氨基酸(rare amino acid)5.非蛋白质氨基酸(nonprotein amino acid) 6.构型(configuration)7.蛋白质的一级结构(protein primary structure)8.构象(conformation)9.蛋白质的二级结构(protein secondary structure)10.结构域(domain)11.蛋白质的三级结构(protein tertiary structure)12.氢键(hydrogen bond)13.蛋白质的四级结构(protein quaternary structure)14.离子键(ionic bond)15.超二级结构(super-secondary structure) 16.疏水键(hydrophobic bond)17.范德华力( van der Waals force) 18.盐析(salting out)19.盐溶(salting in)20.蛋白质的变性(denaturation)21.蛋白质的复性(renaturation)22.蛋白质的沉淀作用(precipitation) 23.凝胶电泳(gel electrophoresis)24.层析(chromatography)第二章核酸1.单核苷酸(mononucleotide)2.磷酸二酯键(phosphodiester bonds)3.不对称比率(dissymmetry ratio)4.碱基互补规律(complementary base pairing)5.反密码子(anticodon)6.顺反子(cistron)7.核酸的变性与复性(denaturation、renaturation)8.退火(annealing)9.增色效应(hyper chromic effect)10.减色效应(hypo chromic effect)11.噬菌体(phage)12.发夹结构(hairpin structure)13.DNA 的熔解温度(melting temperature T m)14.分子杂交(molecular hybridization)15.环化核苷酸(cyclic nucleotide)第三章酶与辅酶1.米氏常数(K m 值)2.底物专一性(substrate specificity)3.辅基(prosthetic group)4.单体酶(monomeric enzyme)5.寡聚酶(oligomeric enzyme)6.多酶体系(multienzyme system)7.激活剂(activator)8.抑制剂(inhibitor inhibiton)9.变构酶(allosteric enzyme)10.同工酶(isozyme)11.诱导酶(induced enzyme)12.酶原(zymogen)13.酶的比活力(enzymatic compare energy)14.活性中心(active center)第四章生物氧化与氧化磷酸化1.生物氧化(biological oxidation)2.呼吸链(respiratory chain)3.氧化磷酸化(oxidative phosphorylation)4.磷氧比P/O(P/O)5.底物水平磷酸化(substrate level phosphorylation)6.能荷(energy charg第五章糖代谢1.糖异生(glycogenolysis)2.Q 酶(Q-enzyme)3.乳酸循环(lactate cycle)4.发酵(fermentation)5.变构调节(allosteric regulation)6.糖酵解途径(glycolytic pathway)7.糖的有氧氧化(aerobic oxidation)8.肝糖原分解(glycogenolysis)9.磷酸戊糖途径(pentose phosphate pathway) 10.D-酶(D-enzyme)11.糖核苷酸(sugar-nucleotide)第六章脂类代谢1.必需脂肪酸(essential fatty acid)2.脂肪酸的α-氧化(α- oxidation)3.脂肪酸的β-氧化(β- oxidation)4.脂肪酸的ω-氧化(ω- oxidation)5.乙醛酸循环(glyoxylate cycle)6.柠檬酸穿梭(citriate shuttle)7.乙酰CoA 羧化酶系(acetyl-CoA carnoxylase)8.脂肪酸合成酶系统(fatty acid synthase system)第八章含氮化合物代谢1.蛋白酶(Proteinase)2.肽酶(Peptidase)3.氮平衡(Nitrogen balance)4.生物固氮(Biological nitrogen fixation)5.硝酸还原作用(Nitrate reduction)6.氨的同化(Incorporation of ammonium ions into organic molecules)7.转氨作用(Transamination)8.尿素循环(Urea cycle)9.生糖氨基酸(Glucogenic amino acid)10.生酮氨基酸(Ketogenic amino acid)11.核酸酶(Nuclease)12.限制性核酸内切酶(Restriction endonuclease)13.氨基蝶呤(Aminopterin)14.一碳单位(One carbon unit)第九章核酸的生物合成1.半保留复制(semiconservative replication)2.不对称转录(asymmetric trancription)3.逆转录(reverse transcription)4.冈崎片段(Okazaki fragment)5.复制叉(replication fork)6.领头链(leading strand)7.随后链(lagging strand)8.有意义链(sense strand)9.光复活(photoreactivation)10.重组修复(recombination repair)11.内含子(intron)12.外显子(exon)13.基因载体(genonic vector)14.质粒(plasmid)第十一章代谢调节1.诱导酶(Inducible enzyme)2.标兵酶(Pacemaker enzyme)3.操纵子(Operon)4.衰减子(Attenuator)5.阻遏物(Repressor)6.辅阻遏物(Corepressor)7.降解物基因活化蛋白(Catabolic gene activator protein)8.腺苷酸环化酶(Adenylate cyclase)9.共价修饰(Covalent modification)10.级联系统(Cascade system)11.反馈抑制(Feedback inhibition)12.交叉调节(Cross regulation)13.前馈激活(Feedforward activation)14.钙调蛋白(Calmodulin)第十二章蛋白质的生物合成1.密码子(codon)2.反义密码子(synonymous codon) 3.反密码子(anticodon)4.变偶假说(wobble hypothesis)5.移码突变(frameshift mutant)6.氨基酸同功受体(isoacceptor)7.反义RNA(antisense RNA)8.信号肽(signal peptide)9.简并密码(degenerate code)10.核糖体(ribosome)11.多核糖体(poly some)12.氨酰基部位(aminoacyl site)13.肽酰基部位(peptidy site)14.肽基转移酶(peptidyl transferase) 15.氨酰- tRNA 合成酶(amino acy-tRNA synthetase)16.蛋白质折叠(protein folding)17.核蛋白体循环(polyribosome) 18.锌指(zine finger)19.亮氨酸拉链(leucine zipper)20.顺式作用元件(cis-acting element) 21.反式作用因子(trans-acting factor) 22.螺旋-环-螺旋(helix-loop-helix)第一章蛋白质1.两性离子:指在同一氨基酸分子上含有等量的正负两种电荷,又称兼性离子或偶极离子。
金属硫蛋白3对快速老化痴呆模型小鼠海马CA1区神经元细胞凋亡的阻碍
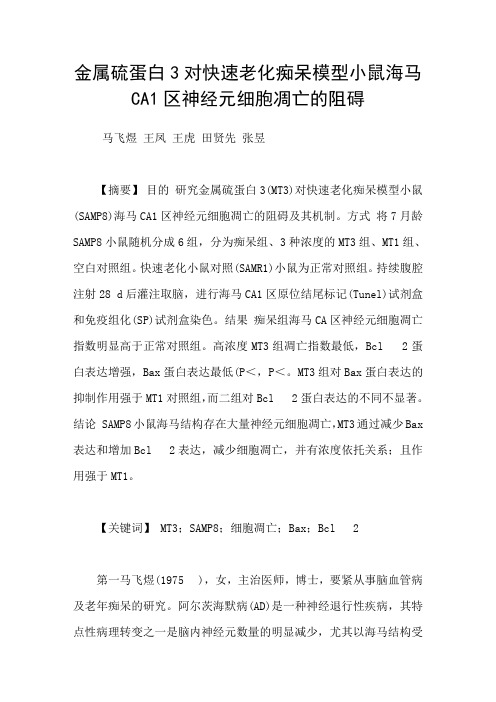
金属硫蛋白3对快速老化痴呆模型小鼠海马CA1区神经元细胞凋亡的阻碍马飞煜王凤王虎田贤先张昱【摘要】目的研究金属硫蛋白3(MT3)对快速老化痴呆模型小鼠(SAMP8)海马CA1区神经元细胞凋亡的阻碍及其机制。
方式将7月龄SAMP8小鼠随机分成6组,分为痴呆组、3种浓度的MT3组、MT1组、空白对照组。
快速老化小鼠对照(SAMR1)小鼠为正常对照组。
持续腹腔注射28 d后灌注取脑,进行海马CA1区原位结尾标记(Tunel)试剂盒和免疫组化(SP)试剂盒染色。
结果痴呆组海马CA区神经元细胞凋亡指数明显高于正常对照组。
高浓度MT3组凋亡指数最低,Bcl2蛋白表达增强,Bax蛋白表达最低(P<,P<。
MT3组对Bax蛋白表达的抑制作用强于MT1对照组,而二组对Bcl2蛋白表达的不同不显著。
结论 SAMP8小鼠海马结构存在大量神经元细胞凋亡,MT3通过减少Bax 表达和增加Bcl2表达,减少细胞凋亡,并有浓度依托关系;且作用强于MT1。
【关键词】 MT3;SAMP8;细胞凋亡;Bax;Bcl2第一马飞煜(1975),女,主治医师,博士,要紧从事脑血管病及老年痴呆的研究。
阿尔茨海默病(AD)是一种神经退行性疾病,其特点性病理转变之一是脑内神经元数量的明显减少,尤其以海马结构受累最为严峻。
细胞凋亡是AD神经元丢失及其他病理学特点形成的要紧缘故。
已有研究发觉身后的AD患者脑内存在大量凋亡神经元,并有凋亡信号分子凋亡增进基因(Bax)的高表达〔1〕,而在非痴呆老年人脑内几乎无表达。
1991年,Uchida等〔2〕第一次从人脑组织中分离出金属硫蛋白3(MT3),因其只在脑内特异表达,可能具有重要的神经生理和神经调剂功能。
临床报导AD患者脑内MT3含量明显减少〔3〕,这就引发了许多MT3与AD发病机制的研究。
快速老化痴呆模型小鼠(SAMP8)是日本Takeda等人精心培育,具有快速老化痴呆的特点,平均寿命个月〔4~6〕,是目前公认的比较理想的自然衰老痴呆模型。
- 1、下载文档前请自行甄别文档内容的完整性,平台不提供额外的编辑、内容补充、找答案等附加服务。
- 2、"仅部分预览"的文档,不可在线预览部分如存在完整性等问题,可反馈申请退款(可完整预览的文档不适用该条件!)。
- 3、如文档侵犯您的权益,请联系客服反馈,我们会尽快为您处理(人工客服工作时间:9:00-18:30)。
Abstract The/3-carotene absorption from the lowest triplet state was investigated over a wide spectral range. A new absorption transition in the nearIR region was found. The absorption band exhibited a 0--0transition at about 8100 cm- 1with a low intensity, lower by a factor of approximately 300 than the intensity of the known triplet-triplet absorption in the visible range. The vibronic structure of the absorption band was similar to that of the steady state/3-carotene absorption. The energy of the triplet state, resulting from this near-IR transition, was close to the fl-carotene S~ state energy. Correspondingly, the interconversion of the carotenoid should depend on the features of this triplet state. Moreover, this relatively low-lying triplet state could also take part in the quenching of molecular triplet states by carotenoids.
Keywords: Transient absorption; Phosphorescence; Energy transfer; Interconvesion; Retinoids; Carotenoids
1. Introduction
/3-Carotene is one of the most widespread natural dyes. Because of the important photoprotective and light-harvesting roles of/3-carotene and similar long-chain carotenoids [ 1,2], many investigations have been carried out for this group of polyenes. The lowest excited singlet state in polyenes has the same g symmetry as that of the ground state [ 3,4 ]. Therefore fluorescence from the $1 state of carotenoids is forbidden, which hinders the investigation of the lowest excited singlet state by the sensitive fluorescence technique. The allowed fluorescence from the high singlet levels of carotenoids is easier to detect. According to Refs. [5-7], the quantum yield of the /3-carotene $2 fluorescence has been determined to be approximately 10 -4 and the $1 lifetime is known to be approximately 10-1~ s. From the $2 fluorescence quantum yield and $2 radiation lifetime, the $2 lifetime was estimated to be approximately 10-13 s [6]. A recent direct measurement has shown that the fluorescence lifetime of the fl-carotene $2 state is approximately 200 fs in n-hexane [ 8]. While the singlet states of carotenoids can take part in lightharvesting processes [2], the triplet states play the main role in their photoprotective function. It is well known that/3carotene and other carotenoids are extremely efficient quenchers of molecular triplet states and singlet oxygen [9,10]. The mechanism of photoprotection is usually described by photophysical energy transfer from the metastable states of the energy donor to the low-lying triplet states of the carotenoid [ 11 ]. Unfortunately, the lowest triplet level
SSDI 1 0 1 0 - 6 0 3 0 ( 9 5 ) 0 4 0 7 7 - 3
112
S.M. Bachilo / Journal of Photochemistry and Photobiology A: Chemistry 91 (1995) 111-115
2. Experimental details 1.0 Crystalline all-trans-#-carotene (/3-carotene) was synthesized at the Vitamin Institute (Moscow). The sample purity was checked by different methods;/3-carotene contained less than 1% impurities, mainly substances with shorter polyene chains [6]. The compound was stored in the dark at a temperature below 0 °C and at a residual pressure of less than 10- 3 Torr. Anthracene was of scintillator grade; the solvents (toluene, n-hexane) were of spectroscopic grade purity. All measurements were carried out with fresh solutions. The spectral and kinetic study of the transient/3-carotene absorption was performed with the equipment described in more detail elsewhere [ 17,18]. Samples were excited with the doubled frequency of a ruby laser (347 nm). The pulse energy was usually in the 5-15 mJ range, the illuminated area in the sample was about 0.5 cm 2 and the pulse duration was 20 ns. A halogen filament lamp (microsecond timescale) and a flash high-pressure xenon lamp (submicrosecond range) were applied as probe lights. The intensity of the probe light was measured by a germanium photodiode with a grating monochromator. The spectral width of the monochromator slits was adjusted from 4 to 30 nm. The monochromator was complemented by red and IR cut-off filters. The photodiode had sufficient sensitivity in the 510-I 660 nm spectral range. A vacuum photodetector was used for the 300-700 nm range. Since all solvents with C - H bonds in their molecules absorb probe light at greater than 1650 nm, this wavelength is the near-IR limit for investigations of electronic absorption in organic solvents. The limit of the system time resolution was about 40 ns. An applied response time could be adjusted to obtain the best signal-to-noise ratio without noticeable distortion of the investigated kinetics. Kinetic traces were detected by oscilloscopes and, due to the high signal-to-noise ratio, we could use the measured kinetic traces without averaging. The noise values for a system response time of 0.5/zs corresponded to an optical density of transient absorption from 10 -5 to 10 -4. Transient absorption intensities (AA) were determined from the ratio of the transient signal (A U) to the signal detected without excitation (U), i.e. AA = In (A U~ U). The absorption spectra were measured point by point in steps of 10 or 20 nm. Since the /3-carotene interconversion yield is very low [ 19], the main measurements were performed using the triplet-triplet energy transfer from a donor to/3-carotene. The energy donor was anthracene, which can successfully be used to populate the #-carotene triplet state [20]. The concentrations of anthracene and #-carotene were chosen to provide an intense anthracene absorption at the excitation wavelength and sufficiently fast rates of triplet-triplet energy transfer from anthracene to B-carotene. The measurements were performed with deaerated solutions at a remaining air pressure of less than 10 -3 Torr. A similar detection system and anthracene as donor were used to examine the #-carotene phosphorescence and, in addition, the phosphorescence of 8,8'-apo-carotenal (Fluka). ,