核磁共振NMR高斯计的测绘原理
基于不同原理的高斯计
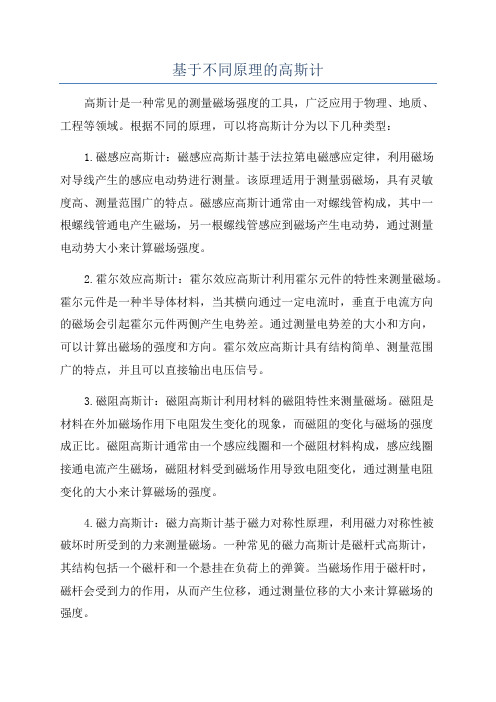
基于不同原理的高斯计高斯计是一种常见的测量磁场强度的工具,广泛应用于物理、地质、工程等领域。
根据不同的原理,可以将高斯计分为以下几种类型:1.磁感应高斯计:磁感应高斯计基于法拉第电磁感应定律,利用磁场对导线产生的感应电动势进行测量。
该原理适用于测量弱磁场,具有灵敏度高、测量范围广的特点。
磁感应高斯计通常由一对螺线管构成,其中一根螺线管通电产生磁场,另一根螺线管感应到磁场产生电动势,通过测量电动势大小来计算磁场强度。
2.霍尔效应高斯计:霍尔效应高斯计利用霍尔元件的特性来测量磁场。
霍尔元件是一种半导体材料,当其横向通过一定电流时,垂直于电流方向的磁场会引起霍尔元件两侧产生电势差。
通过测量电势差的大小和方向,可以计算出磁场的强度和方向。
霍尔效应高斯计具有结构简单、测量范围广的特点,并且可以直接输出电压信号。
3.磁阻高斯计:磁阻高斯计利用材料的磁阻特性来测量磁场。
磁阻是材料在外加磁场作用下电阻发生变化的现象,而磁阻的变化与磁场的强度成正比。
磁阻高斯计通常由一个感应线圈和一个磁阻材料构成,感应线圈接通电流产生磁场,磁阻材料受到磁场作用导致电阻变化,通过测量电阻变化的大小来计算磁场的强度。
4.磁力高斯计:磁力高斯计基于磁力对称性原理,利用磁力对称性被破坏时所受到的力来测量磁场。
一种常见的磁力高斯计是磁杆式高斯计,其结构包括一个磁杆和一个悬挂在负荷上的弹簧。
当磁场作用于磁杆时,磁杆会受到力的作用,从而产生位移,通过测量位移的大小来计算磁场的强度。
5.核磁共振高斯计:核磁共振高斯计利用核磁共振的原理来测量磁场。
核磁共振是指原子核在外加磁场作用下,能级的能量发生分裂的现象。
核磁共振高斯计通常由一个标准样品和一个射频脉冲场构成,通过测量标准样品的核磁共振频率来计算磁场的强度。
综上所述,高斯计根据不同的原理可以分为磁感应高斯计、霍尔效应高斯计、磁阻高斯计、磁力高斯计和核磁共振高斯计等多种类型。
这些高斯计可以根据实际应用场景的需求选择合适的测量原理和仪器,以获得准确可靠的磁场强度测量结果。
核磁共振波谱仪的原理

核磁共振波谱仪(Nuclear Magnetic Resonance Spectrometer,简称NMR)是一种用于研究核磁共振现象的仪器。
核磁共振波谱仪的工作原理涉及到核磁共振现象,这是一种基于原子核的磁性质的现象。
以下是核磁共振波谱仪的基本原理:
1. **磁共振现象:** 在外加磁场的作用下,原子核会表现出特定的磁性质。
当原子核受到外部磁场的作用时,其核自旋会在磁场方向上取向,分裂成不同能级。
这种取向的过程涉及到吸收或发射电磁辐射的能量,这就是核磁共振现象。
2. **射频脉冲:** 核磁共振波谱仪通过在样品中施加射频脉冲来激发样品中的原子核。
这个射频脉冲的频率通常与特定核的共振频率相匹配。
3. **共振频率测定:** 通过逐渐增加外部磁场的强度,使得样品中的原子核逐渐达到共振条件,从而测定原子核的共振频率。
4. **检测信号:** 在样品中的原子核共振时,它们会发出信号。
这个信号被检测器捕获,然后转化为核磁共振波谱。
5. **数据处理:** 捕获的信号经过处理,通过傅里叶变换等数学方法,将其转换为核磁共振谱图,其中包含了关于样品中不同原子核的信息。
核磁共振波谱仪广泛应用于化学、生物化学、医学等领域,用于分析化合物的结构、研究分子运动、探测生物体内的代谢过程等。
核磁共振测井的基本原理
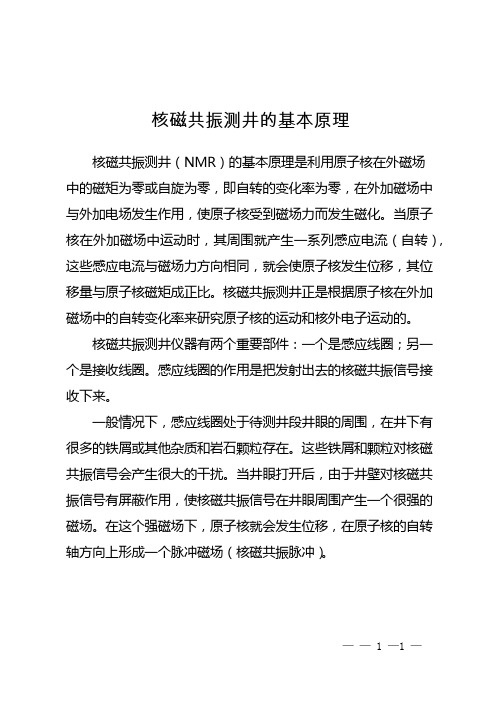
核磁共振测井的基本原理
核磁共振测井(NMR)的基本原理是利用原子核在外磁场
中的磁矩为零或自旋为零,即自转的变化率为零,在外加磁场中与外加电场发生作用,使原子核受到磁场力而发生磁化。
当原子核在外加磁场中运动时,其周围就产生一系列感应电流(自转),这些感应电流与磁场力方向相同,就会使原子核发生位移,其位移量与原子核磁矩成正比。
核磁共振测井正是根据原子核在外加磁场中的自转变化率来研究原子核的运动和核外电子运动的。
核磁共振测井仪器有两个重要部件:一个是感应线圈;另一个是接收线圈。
感应线圈的作用是把发射出去的核磁共振信号接收下来。
一般情况下,感应线圈处于待测井段井眼的周围,在井下有很多的铁屑或其他杂质和岩石颗粒存在。
这些铁屑和颗粒对核磁共振信号会产生很大的干扰。
当井眼打开后,由于井壁对核磁共振信号有屏蔽作用,使核磁共振信号在井眼周围产生一个很强的磁场。
在这个强磁场下,原子核就会发生位移,在原子核的自转轴方向上形成一个脉冲磁场(核磁共振脉冲)。
—— 1 —1 —。
高斯计工作原理
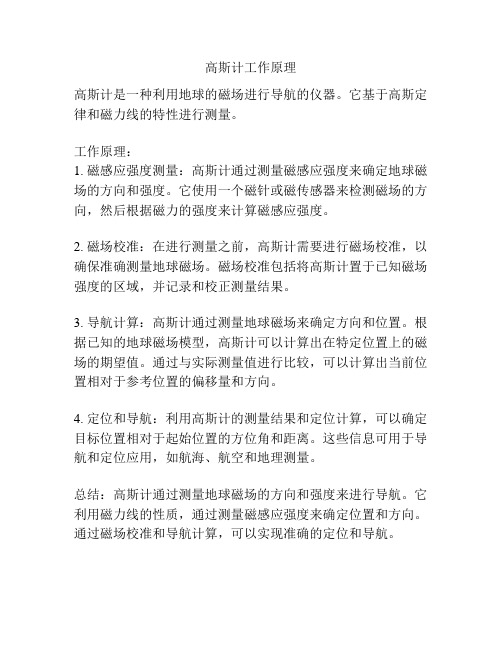
高斯计工作原理
高斯计是一种利用地球的磁场进行导航的仪器。
它基于高斯定律和磁力线的特性进行测量。
工作原理:
1. 磁感应强度测量:高斯计通过测量磁感应强度来确定地球磁场的方向和强度。
它使用一个磁针或磁传感器来检测磁场的方向,然后根据磁力的强度来计算磁感应强度。
2. 磁场校准:在进行测量之前,高斯计需要进行磁场校准,以确保准确测量地球磁场。
磁场校准包括将高斯计置于已知磁场强度的区域,并记录和校正测量结果。
3. 导航计算:高斯计通过测量地球磁场来确定方向和位置。
根据已知的地球磁场模型,高斯计可以计算出在特定位置上的磁场的期望值。
通过与实际测量值进行比较,可以计算出当前位置相对于参考位置的偏移量和方向。
4. 定位和导航:利用高斯计的测量结果和定位计算,可以确定目标位置相对于起始位置的方位角和距离。
这些信息可用于导航和定位应用,如航海、航空和地理测量。
总结:高斯计通过测量地球磁场的方向和强度来进行导航。
它利用磁力线的性质,通过测量磁感应强度来确定位置和方向。
通过磁场校准和导航计算,可以实现准确的定位和导航。
核磁共振光谱的原理

核磁共振光谱的原理核磁共振光谱,简称NMR,是一种分析化学中常用的非破坏性技术。
它基于原子或分子的核磁共振现象,通过测量核磁共振信号的强度和频率,得到样品中不同核的化学环境及其数量等信息。
核磁共振光谱的原理主要涉及核磁共振现象、核自旋、磁共振现象等。
本文将对核磁共振光谱的原理进行详细解析。
一、核磁共振现象核磁共振现象是物理学中的一种现象,它是原子核的原子核磁矩与外加磁场相互作用的结果。
在外加磁场的作用下,原子核会处于能量较低的基态或者激发态,不同核在磁场下的能量差异和跃迁导致核磁共振现象的出现。
核磁共振现象的存在可以通过核磁共振光谱的测量得到。
二、核自旋核自旋是核磁共振现象的基础,也是核磁共振光谱的理论基础之一。
核自旋指的是原子核的一个固有性质,表示核内部的质子和中子的自旋状态。
核自旋量子数用I表示,同一个原子中不同核的I值不同,常见的有I=1/2(例如氢(1H)核)、I=1(例如碳(13C)核)、I=3/2(例如氟(19F)核)等。
三、磁共振现象磁共振现象指的是原子核在外加静磁场和射频磁场作用下发生共振吸收和发射。
在核磁共振实验中,样品被置于静磁场中,使核在基态或者激发态中占据不同的能级,在此基础上加上一个与原子核自旋固有方向针对性的高频交变电磁场,当外加磁场(静磁场)强度满足磁场共振条件时,核能够吸收或发射一定频率的电磁波。
这就是核磁共振信号,信号强度和频率与样品中核的数量和周围化学环境有关。
四、核磁共振谱核磁共振谱是一种通过核磁共振光谱技术得到的谱图,它是通过测量样品中核吸收或发射的共振信号所得到的。
核磁共振谱是一种通常用于分析样品组成的技术,可以用以确定分子中不同原子核的数量和化学环境,因此被广泛应用于化学、生化、药物学等领域。
根据谱图形状和位置等特征,可以进一步分析样品的结构和组成等信息。
五、结论核磁共振光谱是一种利用原子核磁共振现象、核自旋、磁共振现象等原理得到的分析化学技术。
通过测量核磁共振信号的强度和频率等参数,可以得到样品中核的数量和化学环境等信息。
核磁共振成像原理
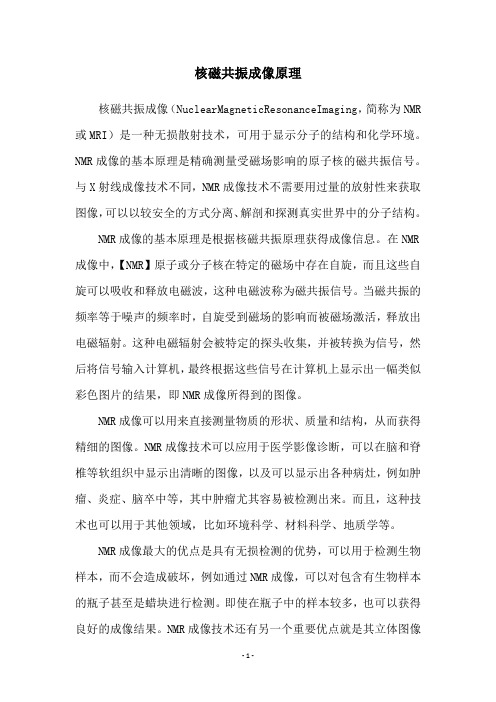
核磁共振成像原理核磁共振成像(NuclearMagneticResonanceImaging,简称为NMR 或MRI)是一种无损散射技术,可用于显示分子的结构和化学环境。
NMR成像的基本原理是精确测量受磁场影响的原子核的磁共振信号。
与X射线成像技术不同,NMR成像技术不需要用过量的放射性来获取图像,可以以较安全的方式分离、解剖和探测真实世界中的分子结构。
NMR成像的基本原理是根据核磁共振原理获得成像信息。
在NMR 成像中,【NMR】原子或分子核在特定的磁场中存在自旋,而且这些自旋可以吸收和释放电磁波,这种电磁波称为磁共振信号。
当磁共振的频率等于噪声的频率时,自旋受到磁场的影响而被磁场激活,释放出电磁辐射。
这种电磁辐射会被特定的探头收集,并被转换为信号,然后将信号输入计算机,最终根据这些信号在计算机上显示出一幅类似彩色图片的结果,即NMR成像所得到的图像。
NMR成像可以用来直接测量物质的形状、质量和结构,从而获得精细的图像。
NMR成像技术可以应用于医学影像诊断,可以在脑和脊椎等软组织中显示出清晰的图像,以及可以显示出各种病灶,例如肿瘤、炎症、脑卒中等,其中肿瘤尤其容易被检测出来。
而且,这种技术也可以用于其他领域,比如环境科学、材料科学、地质学等。
NMR成像最大的优点是具有无损检测的优势,可以用于检测生物样本,而不会造成破坏,例如通过NMR成像,可以对包含有生物样本的瓶子甚至是蜡块进行检测。
即使在瓶子中的样本较多,也可以获得良好的成像结果。
NMR成像技术还有另一个重要优点就是其立体图像处理功能,可以提供四维的动态反映,即可以捕获短暂的生物活动,以便更好的识别和显示活动的细节。
另外,NMR成像技术也特别适合对有机物、分子结构进行解析。
NMR成像技术有可以提供良好的结构解析效果,但由于其使用磁场,会对人体造成一定的影响,因此,有些情况下无法使用NMR成像技术,比如当患者体内金属器件,或者患者同时进行其他检查(比如X射线检查)时,要小心使用NMR成像技术。
高斯计 原理
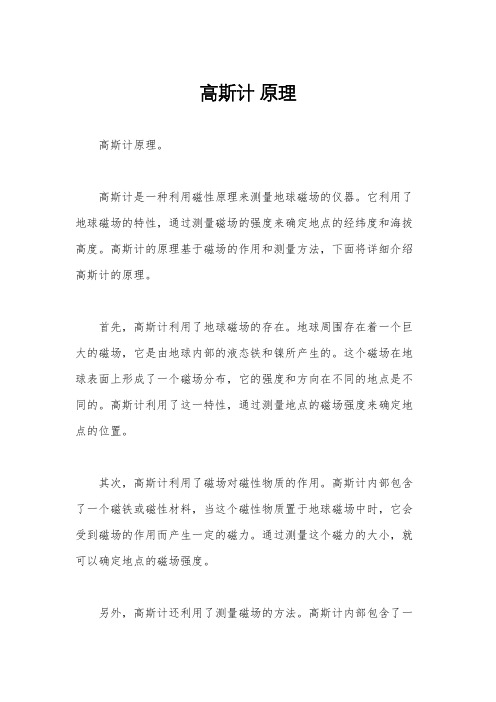
高斯计原理
高斯计原理。
高斯计是一种利用磁性原理来测量地球磁场的仪器。
它利用了地球磁场的特性,通过测量磁场的强度来确定地点的经纬度和海拔高度。
高斯计的原理基于磁场的作用和测量方法,下面将详细介绍高斯计的原理。
首先,高斯计利用了地球磁场的存在。
地球周围存在着一个巨大的磁场,它是由地球内部的液态铁和镍所产生的。
这个磁场在地球表面上形成了一个磁场分布,它的强度和方向在不同的地点是不同的。
高斯计利用了这一特性,通过测量地点的磁场强度来确定地点的位置。
其次,高斯计利用了磁场对磁性物质的作用。
高斯计内部包含了一个磁铁或磁性材料,当这个磁性物质置于地球磁场中时,它会受到磁场的作用而产生一定的磁力。
通过测量这个磁力的大小,就可以确定地点的磁场强度。
另外,高斯计还利用了测量磁场的方法。
高斯计内部包含了一
些测量磁场的装置,例如磁力计或霍尔元件。
这些装置可以测量磁性物质受到的磁力大小,从而确定地点的磁场强度。
通过以上原理,高斯计可以准确地测量地点的磁场强度,从而确定地点的经纬度和海拔高度。
高斯计在地质勘探、导航定位等领域有着广泛的应用,它为人们的生活和工作提供了便利。
总之,高斯计是一种利用磁性原理来测量地球磁场的仪器,它利用了地球磁场的存在、磁场对磁性物质的作用以及测量磁场的方法来确定地点的位置。
高斯计在各个领域有着广泛的应用,它为人们的生活和工作带来了诸多便利。
核磁共振(NMR)的原理和一些图谱分析的技巧
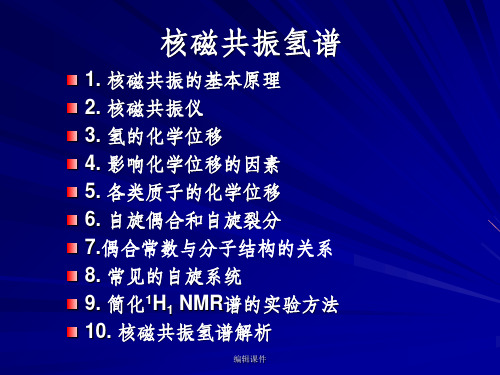
实际上多用后者。
对于1H 核,不同的频率对应的磁场强度:
射频(MHZ)
磁场强度(特斯拉)
60
1.4092
100
2.3500
200
4.7000
300
7.1000
500
11.7500
编辑课件
饱和与弛豫
饱和: 在外磁场作用下,1H 倾向于与外磁场相同取向的排 列。处于低能态的核数目多,由于能级差很小,只 占微弱的优势。
对称操作对称操作对称轴旋转对称轴旋转其他对称操作其他对称操作如对称面如对称面等位质子等位质子化学等价质子化学等价质子对映异位质子对映异位质子非手性环境为化学等价非手性环境为化学等价手性环境为化学不等价手性环境为化学不等价c2ch3clclch3hahbhahbcbrcl在非手性溶剂中化学等价
核磁共振氢谱
自旋核在B0场中的进动
当自旋核处在外磁场B0中时,除自旋外(自旋轴的方 向与 一致),还会绕B0进动,称Larmor进动,类似
于陀螺在重力场中的进动。
旋进轨道
自旋轴
自旋的质子
H 0 BO
编辑课件
回旋轴
B0
B0
核磁距 自旋轴
回旋轴
自旋轴 核磁距
I = 1/2
自旋核在BO场中的进动
编辑课件
I =1/2
编辑课件
化学键的各向异性,导致与其相连的氢核的化学位移 不同。
例如: CH3CH3 CH2=CH2 HC≡CH δ(ppm): 0.86 5.25 1.80
编辑课件
sp杂化碳原子上的质子:叁键碳
碳碳叁键:直线构型,π电子云呈
圆筒型分布,形成环电流,产生 的感应磁场与外加磁场方向相反。 H质子处于屏蔽区,屏蔽效应强, 共振信号移向高场, δ减小。 δ= 1.8~3 H-C≡C-H: 1.8
核磁共振(NMR)工作原理及基本操作
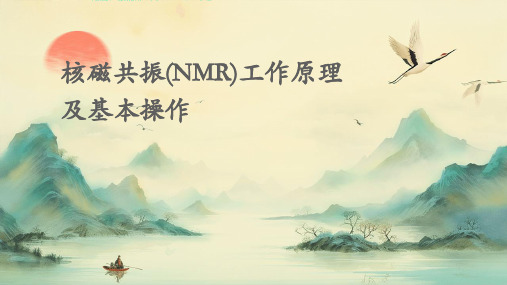
根据分析结果,对样品的组成和性质进行解释和推断。
06
NMR的未来发展与挑战
高场强与超导技术
总结词
高场强与超导技术是NMR领域的重要发 展方向,它们能够提高信号强度和分辨 率,从而更好地应用于复杂样品和生物 医学研究。
VS
详细描述
随着科学技术的不断发展,高场强核磁共 振技术已经成为一种重要的研究手段。高 场强NMR具有更高的磁场强度和分辨率 ,能够提供更精确的化学位移和更强的核 自旋磁化率,从而更好地解析分子结构和 动力学。超导技术是实现高场强NMR的 关键技术之一,它能够产生更强的磁场, 同时保持长时间的稳定性和可靠性。
NMR的定义和重要性
核磁共振(NMR)是一种基于原 子核磁性的物理检测方法,用 于研究物质的微观结构和动态
行为。
NMR技术具有非破坏性、高 灵敏度和高分辨率等优点, 广泛应用于化学、物理、生
物医学等领域。
NMR技术对于推动科学研究 和工业生产的发展具有重要意 义,是现代科技领域不可或缺
的重要工具之一。
样品准备
选择合适的样品容器,确保样品 纯净、无磁性杂质,并按照实验 要求进行样品制备。
实验步骤与参数设置
实验步骤 打开核磁共振谱仪,进行系统自检; 调整磁场强度和射频频率,确保与实验需求匹配;
实验步骤与参数设置
01
进行样品测量,记录数据;
02
分析数据,得出结论。
参数设置
03
实验步骤与参数设置
磁场强度
固态核磁技术
要点一
总结词
固态核磁技术是研究固体材料结构和性质的重要工具,具 有广泛的应用前景。
要点二
详细描述
固态核磁技术是利用核自旋磁矩进行研究的技术,它可以 提供关于物质结构和动态行为的详细信息。由于固态样品 在自然状态下处于非晶态或晶体状态,因此其结构和性质 与液态样品存在显著差异。通过固态核磁技术,可以深入 了解固体材料的晶体结构、化学键、电子结构等信息,对 于材料科学、化学、物理学等领域的研究具有重要意义。
核磁共振波普NMR的原理、用途、分析问题
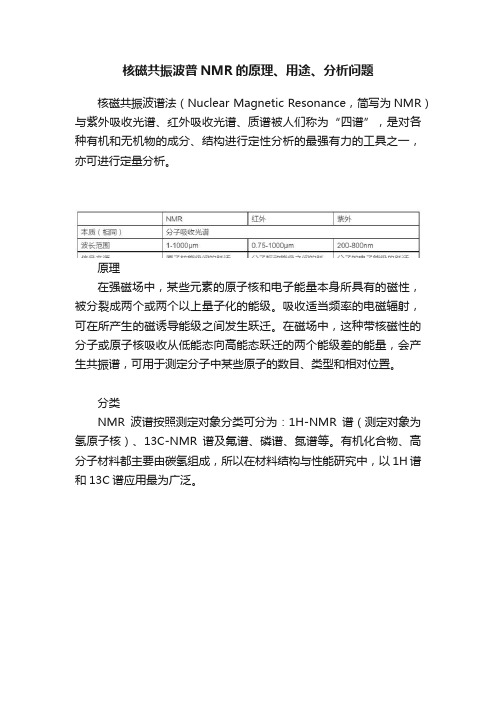
核磁共振波普NMR的原理、用途、分析问题核磁共振波谱法(Nuclear Magnetic Resonance,简写为NMR)与紫外吸收光谱、红外吸收光谱、质谱被人们称为“四谱”,是对各种有机和无机物的成分、结构进行定性分析的最强有力的工具之一,亦可进行定量分析。
原理在强磁场中,某些元素的原子核和电子能量本身所具有的磁性,被分裂成两个或两个以上量子化的能级。
吸收适当频率的电磁辐射,可在所产生的磁诱导能级之间发生跃迁。
在磁场中,这种带核磁性的分子或原子核吸收从低能态向高能态跃迁的两个能级差的能量,会产生共振谱,可用于测定分子中某些原子的数目、类型和相对位置。
分类NMR波谱按照测定对象分类可分为:1H-NMR谱(测定对象为氢原子核)、13C-NMR谱及氟谱、磷谱、氮谱等。
有机化合物、高分子材料都主要由碳氢组成,所以在材料结构与性能研究中,以1H谱和13C谱应用最为广泛。
用途除了运用在医学成像检查方面,在分析化学和有机分子的结构研究及材料表征中运用最多。
1、有机化合物结构鉴定一般根据化学位移鉴定基团;由耦合分裂峰数、偶合常数确定基团联结关系;根据各H峰积分面积定出各基团质子比。
核磁共振谱可用于化学动力学方面的研究,如分子内旋转,化学交换等,因为它们都影响核外化学环境的状况,从而谱图上都应有所反映。
2、高分子材料的NMR成像技术核磁共振成像技术已成功地用来探测材料内部的缺陷或损伤,研究挤塑或发泡材料,粘合剂作用,孔状材料中孔径分布等。
可以被用来改进加工条件,提高制品的质量。
3、多组分材料分析材料的组分比较多时,每种组分的 NMR 参数独立存在,研究聚合物之间的相容性,两个聚合物之间的相同性良好时,共混物的驰豫时间应为相同的,但相容性比较差时,则不同,利用固体NMR 技术测定聚合物共混物的驰豫时间,判定其相容性,了解材料的结构稳定性及性能优异性。
此外,在研究聚合物还用于研究聚合反应机理、高聚物序列结构、未知高分子的定性鉴别、机械及物理性能分析等等。
简述nmr的原理
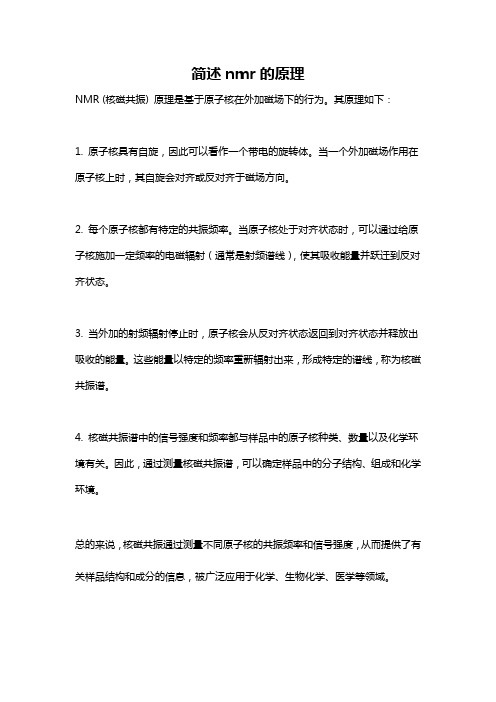
简述nmr的原理
NMR (核磁共振) 原理是基于原子核在外加磁场下的行为。
其原理如下:
1. 原子核具有自旋,因此可以看作一个带电的旋转体。
当一个外加磁场作用在原子核上时,其自旋会对齐或反对齐于磁场方向。
2. 每个原子核都有特定的共振频率。
当原子核处于对齐状态时,可以通过给原子核施加一定频率的电磁辐射(通常是射频谱线),使其吸收能量并跃迁到反对齐状态。
3. 当外加的射频辐射停止时,原子核会从反对齐状态返回到对齐状态并释放出吸收的能量。
这些能量以特定的频率重新辐射出来,形成特定的谱线,称为核磁共振谱。
4. 核磁共振谱中的信号强度和频率都与样品中的原子核种类、数量以及化学环境有关。
因此,通过测量核磁共振谱,可以确定样品中的分子结构、组成和化学环境。
总的来说,核磁共振通过测量不同原子核的共振频率和信号强度,从而提供了有关样品结构和成分的信息,被广泛应用于化学、生物化学、医学等领域。
NMR基本原理与仪器构造

NMR基本原理与仪器构造NMR (Nuclear Magnetic Resonance,核磁共振) 是一种通过测量和分析原子核在磁场中的行为来确定物质结构和性质的分析技术。
NMR的基本原理和仪器构造包括如下几个方面:一、基本原理:1. 核磁共振现象:核磁共振现象源于原子核具有自旋 (spin) 和带电性质,当原子核处于磁场中时,它们会产生一个旋磁比 (gyromagnetic ratio),并围绕磁场的方向进行旋转。
2.外加射频信号:为了激发核共振,需要将外加射频信号与核子的共振频率匹配,从而引起能级之间的跃迁。
二、仪器构造:1. 磁场系统:核磁共振仪的主要部件是磁场系统,它提供一个稳定的磁场,通常采用超导磁体。
磁场越强,谱线分辨率越高。
磁场的强度在核磁共振仪中以特斯拉 (Tesla,T) 为单位进行度量。
2.射频系统:射频系统包括射频发生器、射频放大器和射频探头。
射频发生器产生所需的射频信号,射频放大器将其放大到足够的强度,射频探头通过感应的方式将射频信号传递到样品中。
3.探测系统:探测系统主要由接收线圈、预放大器和检测器组成。
接收线圈感应出样品中由射频信号引起的信号,预放大器将其放大,检测器将信号转换为电信号进行处理和记录。
4.控制系统:核磁共振仪需要一个精确的控制系统来控制磁场强度和均匀性。
同时,控制系统也负责控制射频信号的频率和幅度,以及其他仪器参数的设置。
三、实验过程:1.样品制备:样品通常是液体状态或固体状态的溶液。
液体样品通常是通过溶解待测物质于溶剂中获得,固体样品则需要先进行特殊处理,如溶解或制备样品纤维。
2.样品放置:将样品放置在射频探头中的样品室内。
探头通常包含一个感应线圈和一个用于调整样品位置的样品保持装置。
3.实验参数设置:根据待测物质的性质和需求,设置磁场强度、射频信号频率、扫描次数等参数。
4. 数据采集:开始实验后,仪器将逐步扫描不同的频率,并记录回收信号的强度。
通过改变射频信号的频率,可以得到一系列的回波信号,称为自由感应衰减 (Free Induction Decay, FID)。
磁铁高斯计的测量方法-概述说明以及解释

磁铁高斯计的测量方法-概述说明以及解释1.引言1.1 概述磁铁高斯计是一种用于测量磁场强度的仪器,它可以精确地测量物体周围的磁场强度。
磁场在许多领域中都起着至关重要的作用,因此磁铁高斯计的使用方法和测量结果的可靠性显得尤为重要。
本文将介绍磁铁高斯计的原理和使用方法,并评估测量方法的可靠性和应用前景。
通过对磁铁高斯计的研究和实践应用,我们可以更好地理解磁场力学,并在工程和科学领域中应用它的优势。
磁铁高斯计的原理是基于霍尔效应和磁感应定律的。
当一个磁场与一个导体发生相对运动时,产生的感应电动势可以通过霍尔效应被测量出来。
磁铁高斯计使用霍尔效应传感器来测量感应电流大小,从而得出磁场的强度。
为了正确使用磁铁高斯计,需要首先对仪器进行校准。
校准的目的是确定传感器的灵敏度,并将其转换为磁通密度的单位,即高斯。
校准通常通过将磁铁高斯计置于已知磁场中进行实验来完成。
在使用磁铁高斯计进行测量时,需注意仪器的放置和周围环境的影响。
其他磁场、金属物体和电源等都可能对测量结果产生干扰。
因此,为了获得准确的测量结果,应在低磁场、无干扰的环境中进行测量,并采取合适的校正措施。
磁铁高斯计的测量方法在许多应用领域中具有广泛的用途。
例如,在物理学实验中,测量磁场强度可以帮助我们研究磁场的特性和相互作用。
在工业中,磁铁高斯计可用于检测电机、发电机和磁性材料的性能。
总之,磁铁高斯计是一种有效测量磁场强度的仪器,具有广泛的应用前景。
通过正确使用和校准磁铁高斯计,我们可以获取准确可靠的测量结果,并在各个领域中应用磁场力学的知识。
这对于推动科学研究和促进工业发展都具有重要意义。
1.2文章结构文章结构部分的内容如下:1.2 文章结构本文将按照以下几个部分展开对磁铁高斯计的测量方法进行介绍和分析:第一部分是引言,主要概述本文的背景和研究意义。
我们将介绍磁铁高斯计的基本原理和使用方法,并说明本文的目的。
通过阐述这些背景信息,读者能够更好地理解后续章节的内容。
磁共振校准高斯计的原理
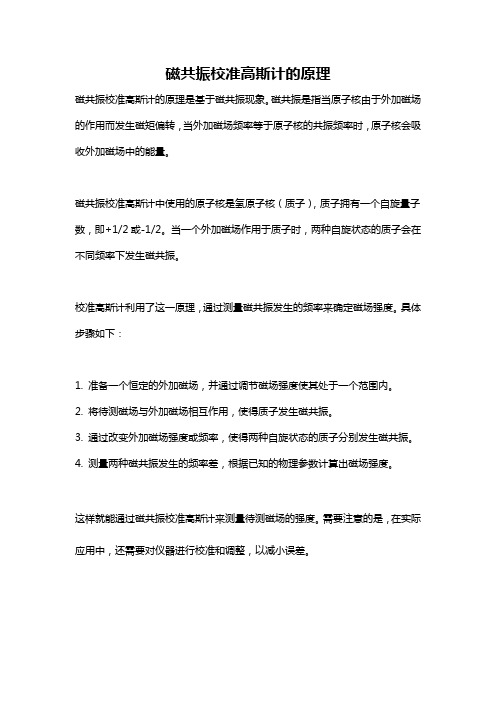
磁共振校准高斯计的原理
磁共振校准高斯计的原理是基于磁共振现象。
磁共振是指当原子核由于外加磁场的作用而发生磁矩偏转,当外加磁场频率等于原子核的共振频率时,原子核会吸收外加磁场中的能量。
磁共振校准高斯计中使用的原子核是氢原子核(质子),质子拥有一个自旋量子数,即+1/2或-1/2。
当一个外加磁场作用于质子时,两种自旋状态的质子会在不同频率下发生磁共振。
校准高斯计利用了这一原理,通过测量磁共振发生的频率来确定磁场强度。
具体步骤如下:
1. 准备一个恒定的外加磁场,并通过调节磁场强度使其处于一个范围内。
2. 将待测磁场与外加磁场相互作用,使得质子发生磁共振。
3. 通过改变外加磁场强度或频率,使得两种自旋状态的质子分别发生磁共振。
4. 测量两种磁共振发生的频率差,根据已知的物理参数计算出磁场强度。
这样就能通过磁共振校准高斯计来测量待测磁场的强度。
需要注意的是,在实际应用中,还需要对仪器进行校准和调整,以减小误差。
核磁共振谱分析知识点
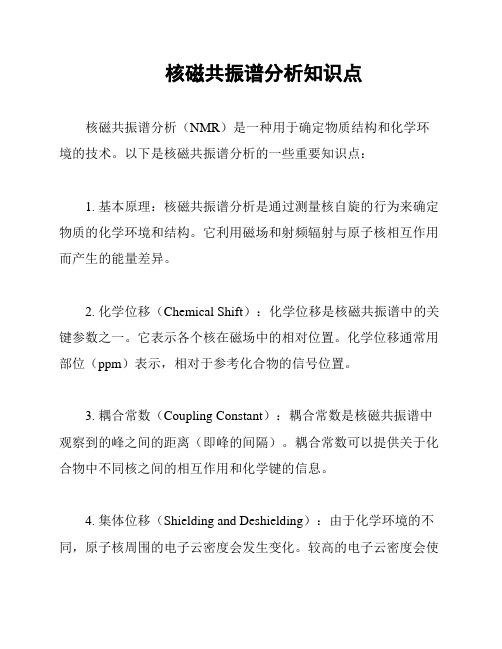
核磁共振谱分析知识点核磁共振谱分析(NMR)是一种用于确定物质结构和化学环境的技术。
以下是核磁共振谱分析的一些重要知识点:1. 基本原理:核磁共振谱分析是通过测量核自旋的行为来确定物质的化学环境和结构。
它利用磁场和射频辐射与原子核相互作用而产生的能量差异。
2. 化学位移(Chemical Shift):化学位移是核磁共振谱中的关键参数之一。
它表示各个核在磁场中的相对位置。
化学位移通常用部位(ppm)表示,相对于参考化合物的信号位置。
3. 耦合常数(Coupling Constant):耦合常数是核磁共振谱中观察到的峰之间的距离(即峰的间隔)。
耦合常数可以提供关于化合物中不同核之间的相互作用和化学键的信息。
4. 集体位移(Shielding and Deshielding):由于化学环境的不同,原子核周围的电子云密度会发生变化。
较高的电子云密度会使原子核受到屏蔽(shielding),而较低的密度会使原子核受到脱屏蔽(deshielding)。
这种集体位移会导致化学位移的变化。
5. 种子核(Spin-spin splitting):当一个核周围存在非等效的氢原子时,该核的信号会发生分裂。
分裂的数量和模式可以提供关于相邻氢原子的数量和位置的信息。
6. 核磁共振谱图的解读:核磁共振谱图可以提供关于化合物结构和化学环境的详细信息。
通过分析化学位移,耦合常数和分裂模式,可以确定化合物中不同原子的相对位置和化学键。
7. 应用领域:核磁共振谱分析在有机化学、药物研究、环境科学、材料科学等领域中得到广泛应用。
它可以帮助确定未知化合物的结构,鉴定有机合成产物,监测环境污染物等。
以上是核磁共振谱分析的一些重要知识点。
深入理解这些知识点可以帮助科学家和研究人员更好地利用核磁共振谱分析技术进行研究和分析。
核磁共振NMR高斯计的测绘原理

核磁共振NMR 高斯计的测绘原理By Philip Keller, Metrolab产品经理瑞士Metrolab公司是精密磁力计的全球市场领导者,在过去的30年中,已经赢得了大型物理实验室和磁共振成像的所有领先厂商的信赖。
磁共振磁强计已经成为世界各地的物理学家,工程师和技术人员日常工具。
最常见的应用包括研究,磁体的制造和测试,标准和校准。
北京华贺技术有限公司作为Metrolab在中国的专业代理与进口公司,全面支持其在中国的市场、销售和售后等服务。
1.NMR 高斯计技术With a resolution approaching 1 ppb (part per billion), Nuclear Magnetic Resonance (NMR) is the gold standard of magnetic measurement. Bellow picture illustrates the technical principle:Principles of NMR magnetic field strength measurement.A proton (hydrogen nucleus) has two possible spin states. In the absence of an external magnetic field, these states are degenerate, but as soon as we apply a field, the spinaligned state is lower energy than the unaligned state. The energy differential ΔE between the two states depends linearly on the magnetic field strength. This exact linear dependence, very nearly independent of all other external factors such as temperature, forms the basis for NMR teslameters.In an NMR teslameter, a coil is wrapped around a sample material rich in hydrogen nuclei – ordinary water works great. This is the "Nuclear" part of NMR. The sample is placed in the magnetic field to be measured ("Magnetic") and irradiated with an RF signal whose frequency gradually increases. When we hit the frequency that corresponds to ΔE, the sample suddenly absorbs energy ("Resonance"). For a field strength of 1 T, this resonance occurs at about 42.5 MHz; this is the gyromagneticratio (γ) for hydrogen nuclei.The resonance is extremely narrow, and so the frequency provides an extremely precise measure of the magnetic field strength.NMR has some overwhelming advantages over other measurement technologies:- extremely precise;- no drift; and- measures total field.That last point is perhaps not obvious. In fact, the protons align themselves with the magnetic field, and the resonance always occurs at B/γ, regardless of how we orient our coil. It turns out, however, that the best coupling between the RF field and the proton spins is achieved when the magnetic field is perpendicular to the coil's axis. In fact, NMR has a blind spot when the coil's axis is exactly aligned with the magnetic field.NMR also has substantial limitations:- Uniform field only: In a field gradient, one edge of the sample will resonate at one frequency, and the opposite edge at another. As the gradient increases, the resonance signal becomes broader and shallower, until it can no longer be detected; this limit typically lies in the range of several hundred to several thousand ppm/cm. In some situations, gradient correction coils can improve this situation.- DC / low-AC fields only: NMR is a relatively slow measurement method, making it unsuitable for measuring rapidly varying fields. Here, we have to distinguish search mode from measurement mode: scanning through a large frequency range to find the resonance requires the field to remain stable for seconds; but once the search is completed, we can track the field and achieve measurement rates of up to about 100 measurements per second.- Low fields require a large sample, ESR or pre-polarization: As the field and, consequently, the energy gap between the spin states tends towards zero, the populations of the two states tend to become equal, and the strength of the resonance diminishes. There are three possible remedies:●Use a very large sample to increase the number of spin-flips.●oPre-polarize the sample in a strong field to populate the spin-aligned state,and then remove this bias field during the measurement.●Use ESR, i.e. the spin of orbital electrons instead of protons, because thesehave a gyromagnetic ratio (γ) three orders of magnitude higher than protons.2.NMR 核磁共振测绘To build an NMR mapper, we need:- NMR probe or probe array. As we will see in the example, a probe array ramatically speeds up the acquisition and simplifies the jig.- Positioning jig.- NMR teslameter (the electronics).The output of an NMR mapper is straightforward:- total field B,- point-sampled,In fact, the size of the "points" are determined by the size of our NMR sample, which is typically a few millimetres in diameter.- very high precision.Well below 1 ppm, in the best case approaching 1 ppb.Computational post-processing depends very much on the application. The typical postprocessing for MRI applications is described in the following section.Close-up of an NMR probe array. Each probe – one of them is circled – is mounted on itsown circuit card. Such probe arrays typically contains 24 or 32 probes (maximum 96).3.NMR核磁共振测绘–案例The most widespread use of NMR mapping relates to the development, manufacturing and installation of MRI (Magnetic Resonance Imaging) magnets. These can be permanent, resistive, or superconducting magnets, with a typical field strength between 0.2 and 7 T.Technically, the preferred shape is a torroid, but for improved access to the patient, or for a less claustrophobic feel, C-shaped magnets are sometimes used. Most modern full-body systems from the major manufacturers use superconducting torroids, 1.5 or 3 T, as illustrated in Figure 12.Example of modern full-body MRI scanner (Philips Achieva).The homogeneity of the field in the imaging region must typically be guaranteed to within a few ppm. Since minor, unavoidable manufacturing variations cause inhomogeneities generally two orders of magnitude greater than that, the homogeneity must be adjusted in a process called shimming.The overall shimming procedure is to map the field, analyze the map, adjust the field with iron shims and/or shim-coil current adjustments, and to remap the field to verify the results.If necessary, this process is repeated until the specified homogeneity is achieved. The same process, and usually the same equipment, is used in R&D, manufacturing and installation. The measurement is extremely sensitive, and obscure effects such as the passing of an overhead crane or the return current of a nearby train can cause hugeerrors.NMR mapping jigs for torroid- and C-shaped MRI magnets, respectively.The probe arrays and jigs shown in Figure 11 and Figure 13 generate a map of thetotal field on the surface of a sphere. As long as there are no currents or magneticmaterial inside this sphere, and assuming the direction of the field is known, the fieldat every point inside the sphere can be calculated from Maxwell's equations. Generally we use an expansion in spherical harmonics to perform this computation.It is worth noting that the jigs shown are much simpler that in the previous examples. Fundamentally, there are two reasons for this:- Using a probe array reduces the required motion to a single dimension.- Partly because the field is so uniform, and partly because the NMR sample points are fairly large, the requirements on the positioning precision are much less –typically 0.1mm. Compare that to the 0.1 μm for the Hall mapper example!4.问答To summarize our observations from these different examples of magnetic mapping, here is a small check-list of key questions to ask if you want (rather, need) to build a mapper:- Use: R&D, production, field service?It makes a big difference if a system is to be used once in a while for R&D, or all the time in production, or packed up in a suitcase for field service.- Measurement: field components, total field, integral, gradient?Do you really need Bx, By, Bz, or do you really need B, or an integral or gradient of B?By choosing the appropriate measurement technology, you may be able to speed up the acquisition as well improve your precision.- Field: strength, uniformity, AC/DC, stability?The characteristics of your field also play an important role in the choice of measurement technology.- Precision: 10% or 10 ppm?…as does the precision required.- Positioning: access, range, precision, reproducibility?The design of your jig is as important as the choice of magnetic sensor. What sort of access do you have to the region to be measured? What positioning precision do you need? How reproducible must the positioning be?- Environment: vacuum, cryogenic?The environment changes dramatically depending on whether you're in a lab, on a production floor or in the field. In addition, magnetic systems are often combined with vacuums and cryogenic temperatures. Hall effect sensors, for example, don't work at liquid-helium temperatures.- Speed: cost, external error sources, human error?Making a fast mapper may not cost more; for example, we saw that the simplification of the jig may more than make up for the costs of a sensor array. In addition, the improved speed dramatically reduces external and human error.。
核磁共振法的基本原理是什么
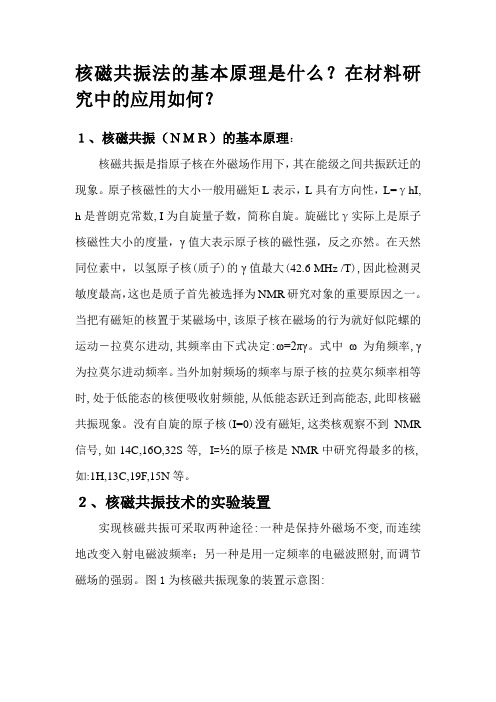
核磁共振法的基本原理是什么?在材料研究中的应用如何?1、核磁共振(NMR)的基本原理:核磁共振是指原子核在外磁场作用下,其在能级之间共振跃迁的现象。
原子核磁性的大小一般用磁矩L表示,L具有方向性,L=γhI, h是普朗克常数, I为自旋量子数,简称自旋。
旋磁比γ实际上是原子核磁性大小的度量,γ值大表示原子核的磁性强,反之亦然。
在天然同位素中,以氢原子核(质子)的γ值最大(42.6 MHz /T),因此检测灵敏度最高,这也是质子首先被选择为NMR研究对象的重要原因之一。
当把有磁矩的核置于某磁场中,该原子核在磁场的行为就好似陀螺的运动-拉莫尔进动,其频率由下式决定:ω=2πγ。
式中ω为角频率,γ为拉莫尔进动频率。
当外加射频场的频率与原子核的拉莫尔频率相等时,处于低能态的核便吸收射频能,从低能态跃迁到高能态,此即核磁共振现象。
没有自旋的原子核(I=0)没有磁矩,这类核观察不到NMR 信号,如14C,16O,32S等, I=½的原子核是NMR中研究得最多的核,如:1H,13C,19F,15N等。
2、核磁共振技术的实验装置实现核磁共振可采取两种途径:一种是保持外磁场不变,而连续地改变入射电磁波频率;另一种是用一定频率的电磁波照射,而调节磁场的强弱。
图1为核磁共振现象的装置示意图:采用调节入射电磁波频率的方法来达到核磁共振,样品装在小瓶中,并置于磁铁两极之间,瓶外绕有线圈,通有由射频振荡器输出的射频电流。
于是,由线圈向样品发射电磁波。
调制振荡器的作用是使射频电磁波的频率在样品共振频率附近连续变化,当频率正好与核磁共振频率吻合时,射频振荡器的输出就会出现一个吸收峰。
这可以在示波器上显示出来。
同时由频率计即刻读出这时的共振频率值。
核磁共振谱仪是专门用于观测核磁共振的仪器,主要由磁铁、探头和谱仪三大部分组成。
磁铁的功用是产生一个恒定的磁场;探头置于磁极之间,用于探测核磁共振信号;谱仪是将共振信号放大处理并显示和记录下来。
核磁共振检测仪工作原理

核磁共振检测仪工作原理
核磁共振检测仪(NMR)是一种常用于分析物质结构和性质的科学仪器。
它基于原子核在强磁场中的行为原理,以下是核磁共振检测仪的工作原理:
1. 磁场:核磁共振检测仪首先通过一个强大且稳定的静态磁场,通常是超导磁体或永磁体,产生一个均匀的磁场。
2. 原子核的自旋:在这个强磁场中,样品中的原子核会发生取向,具有自旋。
原子核的自旋可以类比为一个带有磁矩的微小磁针。
3. 射频脉冲:核磁共振检测仪还包括一个射频线圈,用于向样品中的原子核发送一系列特定频率的射频脉冲。
这些射频脉冲会使部分原子核从低能级跃迁到高能级。
4. 回复信号:当射频脉冲停止时,经过一段时间后,被激发的原子核会重新回到低能级。
这个过程称为弛豫。
在这个过程中,原子核会向周围的空间辐射出一个特定频率的电磁信号。
5. 探测:核磁共振检测仪的接收线圈会探测到这些回复信号,并将其转换为电信号。
6. 数据分析:通过对接收到的信号进行处理和分析,可以获得关于样品中原子核数量、化学环境以及相互作用等信息。
这些信息可以用于确定样品的化学结构和性质。
nmr计算高斯
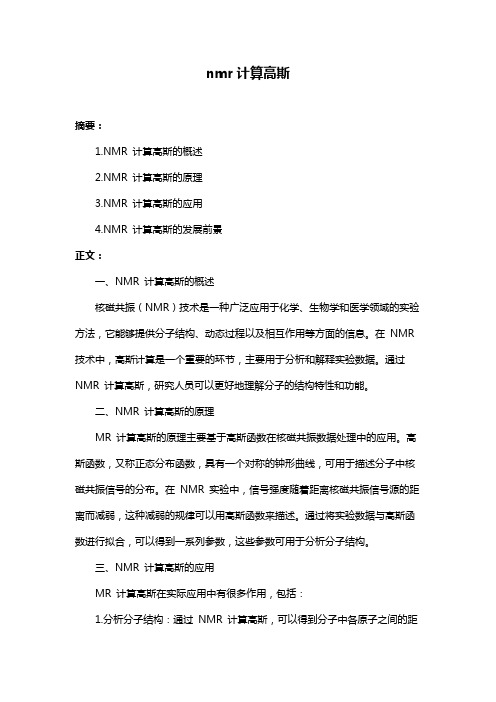
nmr计算高斯摘要:1.NMR 计算高斯的概述2.NMR 计算高斯的原理3.NMR 计算高斯的应用4.NMR 计算高斯的发展前景正文:一、NMR 计算高斯的概述核磁共振(NMR)技术是一种广泛应用于化学、生物学和医学领域的实验方法,它能够提供分子结构、动态过程以及相互作用等方面的信息。
在NMR 技术中,高斯计算是一个重要的环节,主要用于分析和解释实验数据。
通过NMR 计算高斯,研究人员可以更好地理解分子的结构特性和功能。
二、NMR 计算高斯的原理MR 计算高斯的原理主要基于高斯函数在核磁共振数据处理中的应用。
高斯函数,又称正态分布函数,具有一个对称的钟形曲线,可用于描述分子中核磁共振信号的分布。
在NMR 实验中,信号强度随着距离核磁共振信号源的距离而减弱,这种减弱的规律可以用高斯函数来描述。
通过将实验数据与高斯函数进行拟合,可以得到一系列参数,这些参数可用于分析分子结构。
三、NMR 计算高斯的应用MR 计算高斯在实际应用中有很多作用,包括:1.分析分子结构:通过NMR 计算高斯,可以得到分子中各原子之间的距离信息,从而推测分子的结构。
2.研究分子动态过程:NMR 计算高斯可以用于分析分子在溶液中的动态过程,如旋转、翻转等。
3.研究分子相互作用:NMR 计算高斯可以用于研究分子间的相互作用,如氢键、范德华力等。
4.确定分子构象:NMR 计算高斯可以帮助研究人员确定分子的构象,从而更好地理解其功能。
四、NMR 计算高斯的发展前景随着核磁共振技术的不断发展,NMR 计算高斯在生物学、化学和医学领域的应用将越来越广泛。
未来,随着计算能力的提升和算法的优化,NMR 计算高斯的精度和效率将进一步提高,为研究人员提供更加精确的分析结果。
- 1、下载文档前请自行甄别文档内容的完整性,平台不提供额外的编辑、内容补充、找答案等附加服务。
- 2、"仅部分预览"的文档,不可在线预览部分如存在完整性等问题,可反馈申请退款(可完整预览的文档不适用该条件!)。
- 3、如文档侵犯您的权益,请联系客服反馈,我们会尽快为您处理(人工客服工作时间:9:00-18:30)。
核磁共振NMR 高斯计的测绘原理By Philip Keller, Metrolab产品经理瑞士Metrolab公司是精密磁力计的全球市场领导者,在过去的30年中,已经赢得了大型物理实验室和磁共振成像的所有领先厂商的信赖。
磁共振磁强计已经成为世界各地的物理学家,工程师和技术人员日常工具。
最常见的应用包括研究,磁体的制造和测试,标准和校准。
北京华贺技术有限公司作为Metrolab在中国的专业代理与进口公司,全面支持其在中国的市场、销售和售后等服务。
1.NMR 高斯计技术With a resolution approaching 1 ppb (part per billion), Nuclear Magnetic Resonance (NMR) is the gold standard of magnetic measurement. Bellow picture illustrates the technical principle:Principles of NMR magnetic field strength measurement.A proton (hydrogen nucleus) has two possible spin states. In the absence of an external magnetic field, these states are degenerate, but as soon as we apply a field, the spinaligned state is lower energy than the unaligned state. The energy differential ΔE between the two states depends linearly on the magnetic field strength. This exact linear dependence, very nearly independent of all other external factors such as temperature, forms the basis for NMR teslameters.In an NMR teslameter, a coil is wrapped around a sample material rich in hydrogen nuclei – ordinary water works great. This is the "Nuclear" part of NMR. The sample is placed in the magnetic field to be measured ("Magnetic") and irradiated with an RF signal whose frequency gradually increases. When we hit the frequency that corresponds to ΔE, the sample suddenly absorbs energy ("Resonance"). For a field strength of 1 T, this resonance occurs at about 42.5 MHz; this is the gyromagneticratio (γ) for hydrogen nuclei.The resonance is extremely narrow, and so the frequency provides an extremely precise measure of the magnetic field strength.NMR has some overwhelming advantages over other measurement technologies:- extremely precise;- no drift; and- measures total field.That last point is perhaps not obvious. In fact, the protons align themselves with the magnetic field, and the resonance always occurs at B/γ, regardless of how we orient our coil. It turns out, however, that the best coupling between the RF field and the proton spins is achieved when the magnetic field is perpendicular to the coil's axis. In fact, NMR has a blind spot when the coil's axis is exactly aligned with the magnetic field.NMR also has substantial limitations:- Uniform field only: In a field gradient, one edge of the sample will resonate at one frequency, and the opposite edge at another. As the gradient increases, the resonance signal becomes broader and shallower, until it can no longer be detected; this limit typically lies in the range of several hundred to several thousand ppm/cm. In some situations, gradient correction coils can improve this situation.- DC / low-AC fields only: NMR is a relatively slow measurement method, making it unsuitable for measuring rapidly varying fields. Here, we have to distinguish search mode from measurement mode: scanning through a large frequency range to find the resonance requires the field to remain stable for seconds; but once the search is completed, we can track the field and achieve measurement rates of up to about 100 measurements per second.- Low fields require a large sample, ESR or pre-polarization: As the field and, consequently, the energy gap between the spin states tends towards zero, the populations of the two states tend to become equal, and the strength of the resonance diminishes. There are three possible remedies:●Use a very large sample to increase the number of spin-flips.●oPre-polarize the sample in a strong field to populate the spin-aligned state,and then remove this bias field during the measurement.●Use ESR, i.e. the spin of orbital electrons instead of protons, because thesehave a gyromagnetic ratio (γ) three orders of magnitude higher than protons.2.NMR 核磁共振测绘To build an NMR mapper, we need:- NMR probe or probe array. As we will see in the example, a probe array ramatically speeds up the acquisition and simplifies the jig.- Positioning jig.- NMR teslameter (the electronics).The output of an NMR mapper is straightforward:- total field B,- point-sampled,In fact, the size of the "points" are determined by the size of our NMR sample, which is typically a few millimetres in diameter.- very high precision.Well below 1 ppm, in the best case approaching 1 ppb.Computational post-processing depends very much on the application. The typical postprocessing for MRI applications is described in the following section.Close-up of an NMR probe array. Each probe – one of them is circled – is mounted on itsown circuit card. Such probe arrays typically contains 24 or 32 probes (maximum 96).3.NMR核磁共振测绘–案例The most widespread use of NMR mapping relates to the development, manufacturing and installation of MRI (Magnetic Resonance Imaging) magnets. These can be permanent, resistive, or superconducting magnets, with a typical field strength between 0.2 and 7 T.Technically, the preferred shape is a torroid, but for improved access to the patient, or for a less claustrophobic feel, C-shaped magnets are sometimes used. Most modern full-body systems from the major manufacturers use superconducting torroids, 1.5 or 3 T, as illustrated in Figure 12.Example of modern full-body MRI scanner (Philips Achieva).The homogeneity of the field in the imaging region must typically be guaranteed to within a few ppm. Since minor, unavoidable manufacturing variations cause inhomogeneities generally two orders of magnitude greater than that, the homogeneity must be adjusted in a process called shimming.The overall shimming procedure is to map the field, analyze the map, adjust the field with iron shims and/or shim-coil current adjustments, and to remap the field to verify the results.If necessary, this process is repeated until the specified homogeneity is achieved. The same process, and usually the same equipment, is used in R&D, manufacturing and installation. The measurement is extremely sensitive, and obscure effects such as the passing of an overhead crane or the return current of a nearby train can cause hugeerrors.NMR mapping jigs for torroid- and C-shaped MRI magnets, respectively.The probe arrays and jigs shown in Figure 11 and Figure 13 generate a map of thetotal field on the surface of a sphere. As long as there are no currents or magneticmaterial inside this sphere, and assuming the direction of the field is known, the fieldat every point inside the sphere can be calculated from Maxwell's equations. Generally we use an expansion in spherical harmonics to perform this computation.It is worth noting that the jigs shown are much simpler that in the previous examples. Fundamentally, there are two reasons for this:- Using a probe array reduces the required motion to a single dimension.- Partly because the field is so uniform, and partly because the NMR sample points are fairly large, the requirements on the positioning precision are much less –typically 0.1mm. Compare that to the 0.1 μm for the Hall mapper example!4.问答To summarize our observations from these different examples of magnetic mapping, here is a small check-list of key questions to ask if you want (rather, need) to build a mapper:- Use: R&D, production, field service?It makes a big difference if a system is to be used once in a while for R&D, or all the time in production, or packed up in a suitcase for field service.- Measurement: field components, total field, integral, gradient?Do you really need Bx, By, Bz, or do you really need B, or an integral or gradient of B?By choosing the appropriate measurement technology, you may be able to speed up the acquisition as well improve your precision.- Field: strength, uniformity, AC/DC, stability?The characteristics of your field also play an important role in the choice of measurement technology.- Precision: 10% or 10 ppm?…as does the precision required.- Positioning: access, range, precision, reproducibility?The design of your jig is as important as the choice of magnetic sensor. What sort of access do you have to the region to be measured? What positioning precision do you need? How reproducible must the positioning be?- Environment: vacuum, cryogenic?The environment changes dramatically depending on whether you're in a lab, on a production floor or in the field. In addition, magnetic systems are often combined with vacuums and cryogenic temperatures. Hall effect sensors, for example, don't work at liquid-helium temperatures.- Speed: cost, external error sources, human error?Making a fast mapper may not cost more; for example, we saw that the simplification of the jig may more than make up for the costs of a sensor array. In addition, the improved speed dramatically reduces external and human error.。