Dynamics of chromophoric dissolved organic matter influenced by hydrological conditions in a large
英文简称资料
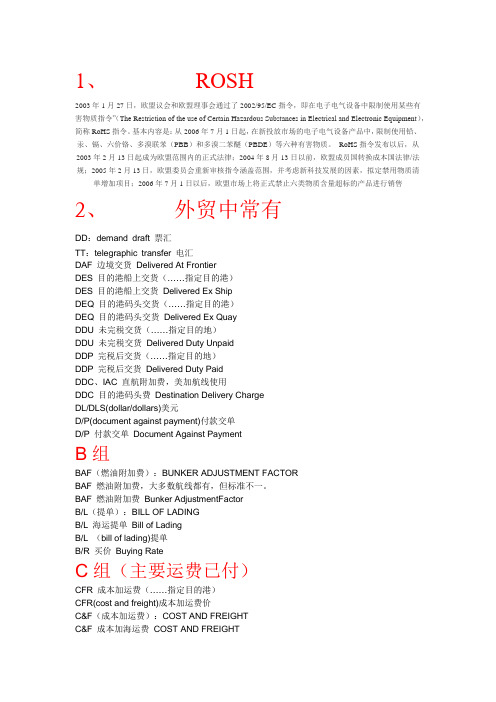
1、ROSH2003年1月27日,欧盟议会和欧盟理事会通过了2002/95/EC指令,即在电子电气设备中限制使用某些有害物质指令”(The Restriction of the use of Certain Hazardous Substances in Electrical and Electronic Equipment),简称RoHS指令。
基本内容是:从2006年7月1日起,在新投放市场的电子电气设备产品中,限制使用铅、汞、镉、六价铬、多溴联苯(PBB)和多溴二苯醚(PBDE)等六种有害物质。
RoHS指令发布以后,从2003年2月13日起成为欧盟范围内的正式法律;2004年8月13日以前,欧盟成员国转换成本国法律/法规;2005年2月13日,欧盟委员会重新审核指令涵盖范围,并考虑新科技发展的因素,拟定禁用物质清单增加项目;2006年7月1日以后,欧盟市场上将正式禁止六类物质含量超标的产品进行销售2、外贸中常有DD:demand draft 票汇TT:telegraphic transfer 电汇DAF 边境交货Delivered At FrontierDES 目的港船上交货(……指定目的港)DES 目的港船上交货Delivered Ex ShipDEQ 目的港码头交货(……指定目的港)DEQ 目的港码头交货Delivered Ex QuayDDU 未完税交货(……指定目的地)DDU 未完税交货Delivered Duty UnpaidDDP 完税后交货(……指定目的地)DDP 完税后交货Delivered Duty PaidDDC、IAC 直航附加费,美加航线使用DDC 目的港码头费Destination Delivery ChargeDL/DLS(dollar/dollars)美元D/P(document against payment)付款交单D/P 付款交单Document Against PaymentB组BAF(燃油附加费):BUNKER ADJUSTMENT FACTORBAF 燃油附加费,大多数航线都有,但标准不一。
有色可溶性有机物在线荧光技术在水质监测中的应用
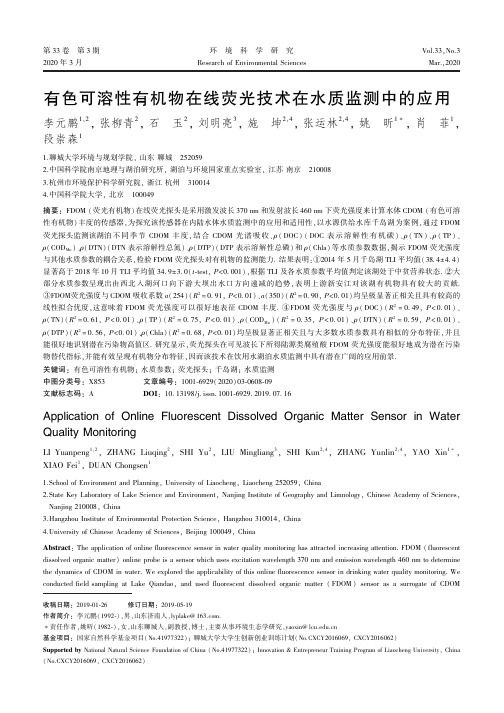
关键词: 有色可溶性有机物ꎻ 水质参数ꎻ 荧光探头ꎻ 千岛湖ꎻ 水质监测
中图分类号: X853 文章编号: 1001 ̄6929(2020)03 ̄0608 ̄09
文献标志码: A
DOI: 10 13198∕j issn 1001 ̄6929 2019 07 16
段崇森1
1.聊城大学环境与规划学院ꎬ 山东 聊城 252059
2.中国科学院南京地理与湖泊研究所ꎬ 湖泊与环境国家重点实验室ꎬ 江苏 南京 210008
3.杭州市环境保护科学研究院ꎬ 浙江 杭州 310014
4.中国科学院大学ꎬ 北京 100049
摘要: FDOM ( 荧光有机物) 在线荧光探头是采用激发波长 370 nm 和发射波长 460 nm 下荧光强度来计算水体 CDOM ( 有色可溶
460 nm of has broad application prospects in monitoring the water quality of drinking water lakes.
Keywords: chromophoric dissolved organic matter ( CDOM) ꎻ water quality parametersꎻ fluorescence sensorꎻ Lake Qiandaoꎻ water quality
0 91ꎬ P<0 01) and a(350) ( R2 = 0 90ꎬ P<0 01) . This indicated that the fluorescence intensity of FDOM could be a useful tracer for
水体中有色可溶性有机物的测量及其变化
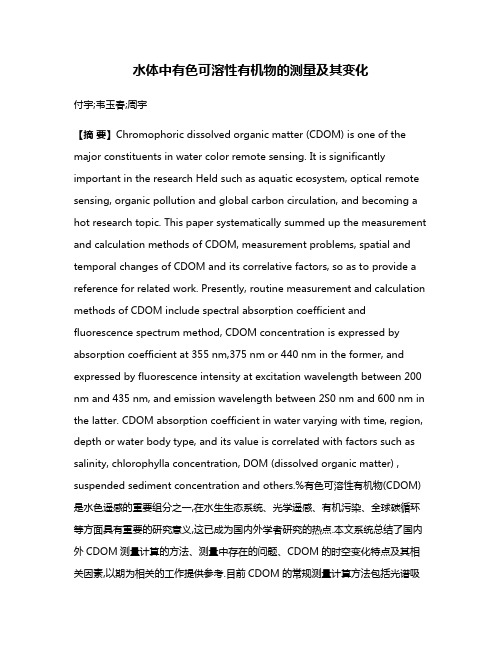
水体中有色可溶性有机物的测量及其变化付宇;韦玉春;周宇【摘要】Chromophoric dissolved organic matter (CDOM) is one of the major constituents in water color remote sensing. It is significantly important in the research Held such as aquatic ecosystem, optical remote sensing, organic pollution and global carbon circulation, and becoming a hot research topic. This paper systematically summed up the measurement and calculation methods of CDOM, measurement problems, spatial and temporal changes of CDOM and its correlative factors, so as to provide a reference for related work. Presently, routine measurement and calculation methods of CDOM include spectral absorption coefficient and fluorescence spectrum method, CDOM concentration is expressed by absorption coefficient at 355 nm,375 nm or 440 nm in the former, and expressed by fluorescence intensity at excitation wavelength between 200 nm and 435 nm, and emission wavelength between 2S0 nm and 600 nm in the latter. CDOM absorption coefficient in water varying with time, region, depth or water body type, and its value is correlated with factors such as salinity, chlorophylla concentration, DOM (dissolved organic matter) , suspended sediment concentration and others.%有色可溶性有机物(CDOM)是水色遥感的重要组分之一,在水生生态系统、光学遥感、有机污染、全球碳循环等方面具有重要的研究意义,这已成为国内外学者研究的热点.本文系统总结了国内外CDOM测量计算的方法、测量中存在的问题、CDOM的时空变化特点及其相关因素,以期为相关的工作提供参考.目前CDOM的常规测量计算方法包括光谱吸收系数和荧光光谱法两种,前者使用355 nm、375 nm、440 nm等波长处的吸收系数来表示CDOM的浓度,后者采用200 nm~435 nm激发波长和250 nm~600 nm发射波长的荧光强度来表示CDOM的浓度.CDOM吸收系数在不同时间段、不同区域、不同深度或不同类型水体中均存在差异,且其值大小与盐度、叶绿素a浓度、DOC、悬浮泥沙浓度等因素存在一定的相关性.【期刊名称】《南京师大学报(自然科学版)》【年(卷),期】2012(035)001【总页数】9页(P95-103)【关键词】有色可溶性有机物;吸光度;水色;碳循环【作者】付宇;韦玉春;周宇【作者单位】南京师范大学教育部虚拟地理环境重点实验室,江苏南京210046;南京师范大学教育部虚拟地理环境重点实验室,江苏南京210046;南京师范大学教育部虚拟地理环境重点实验室,江苏南京210046【正文语种】中文【中图分类】X832有色可溶性有机物最早称为黄色物质,黄色物质的研究开始于20世纪30年代,最初英文表示为Gelbstoff,原指海水中的粘质胶性溶解物.随着研究的深入,人们还发现自然水体中的黄色物质由可溶性有机物碎片组成.大部分学者认为黄色物质有两种来源:一种来源于陆地,主要是江河径流携带;另一种由海洋浮游植物有机体化学降解而形成.在近海,江河径流携带入海为主要来源,而在大洋则主要是由海洋有机物自身降解所产生的.因此海水中的黄色物质分为“海洋”和“陆地”两种起源[1].为了更准确地表达黄色物质的理化属性,目前国际上称其为“有色可溶性有机质”(Chromop horic dissolved organic matter,CDOM).CDOM是水体中重要的光衰减因子,也是生物光学模型构建的主要组成部分,其光学特性比较单一,主要表现为对光的吸收.CDOM浓度和光学特性显著地改变了水体水色和水下光场强度及光谱的分布[2],由于CDOM对光的衰减作用主要表现为吸收,且在紫外和蓝光波段随波长的增加呈指数下降[3];由于其在蓝光波段的吸收与叶绿素a吸收重叠[3],直接干扰了水色遥感,导致了水体中叶绿素a浓度估算偏高,因此CDOM也成为遥感专家关注的重点.1 研究意义CDOM作为水色遥感的重要组分之一,其吸收能力主要分布在紫外光及可见光的蓝光部分.由于其有效地吸收了紫外光波段,可以限制对生物有害的UVB辐射(280 nm~320 nm)穿透深度保护了水生生物,同时,CDOM吸收紫外辐射后容易发生光化学降解,释放碳、氮等元素参与营养盐的循环,是湖泊富营养化进程的重要驱动因子[4],而其在蓝光区的吸收作用,与浮游植物和非藻类颗粒物的吸收重叠,干扰了浮游植物生物量、初级生产力和悬浮物的定量遥感[5],而且降低了水中蓝光的能量及传输深度,一定程度上抑制了水体中叶绿素的光合作用.另外,CDOM还是一种荧光物质,在紫外短波光的激发下会发出长于吸收光波长的荧光.其含量与光学特性显著地改变了湖泊水色及水下光强和光谱分布[6],在水环境及水生生态系统中具有重要地位.因此,研究利用遥感方法提取大范围水体中CDOM含量对于湖泊水质和富营养化的遥感监测、水下光场结构改善等都非常重要.CDOM在海水中的化学性质比较稳定,是重要的水质参数之一.在以陆源黄色物质为主的近岸海域,黄色物质浓度可以作为海水污染程度的“指示剂”,黄色物质对海水中污染物质的形态、毒性、运移产生重要影响.在海陆相互作用研究中,陆源黄色物质可以作为陆地向海洋的化学物质输送的代表性参数之一[7].因而研究CDOM对有机污染物的迁移循环以及生态系统动力学过程有重要意义.CDOM吸收系数与溶解有机碳(DOC)浓度可以建立很好的区域关系,用以反演得出非光学活性的DOC.CDOM具有稳定的荧光特性,对于CDOM相对主导的海区,可以用某一激发波长对其进行高灵敏度荧光测定,再根据线性关系反演吸收系数,从而实现对海洋水色和叶绿素a浓度的遥感校正.另外,对近岸尤其对河口区,还可以通过建立CDOM吸收系数与盐度的相关关系,利用遥感间接获得盐度等物理参数[8].因此,研究其光谱吸收性质具有重要的实际意义.CDOM吸收紫外光后可产生能被浮游生物利用的低分子量含碳气体(如CO2),海水中的CDOM被推测是地球表面的最大碳源,研究CDOM的属性对海中碳含量的估算具有重大影响,因此对黄色物质的光研究是全球碳系统研究的重要组成部分,对储藏于大气层中CO2问题的研究具有重要意义[9].2 物质构成Kalle指出黄色物质由碳水化合物经麦拉德反应形成,即含有碱和氨基酸的溶液经化学反应受热形成黄色或棕色的类黑精终产物[10].随着研究的深入,Gagosian于1977年指出黄色物质可能由下列成分组成:氨基酸、糖、氨基糖和脂肪酸类,另外也可能含有类胡萝卜素、氯纶色素、碳水化合物和酚等[11].随着全球碳循环研究的进展,发现海水中碳的存在形式主要有颗粒有机碳(POC)和溶解有机碳(DOC)两种[1].在溶解有机碳中,在黄光波段吸收较小且呈黄色的那部分物质被称为黄色物质,此时将黄色物质的英文名称改为Yellow Substance.Carder K L研究发现,黄色物质是与悬浮物和浮游植物并列的海洋水色遥感的3个重要组分,直接影响和间接减少了浮游植物的光合作用,严重干扰了光学遥感确切估算海洋初级生产力和海洋悬浮泥沙含量[12].3 光谱特征对黄色物质的光吸收研究可追溯到20世纪30年代,Kalle、Jerlov等人进一步对黄色物质吸收光谱开展研究,发现黄色物质的吸收与吸收光波波长呈指数变化;Morel等[13]给出了黄色物质光吸收系数的指数表达式;1981年Bricaud得出CDOM光谱吸收曲线变化与非藻类颗粒物相似,在可见光区域随波长增加呈负指数规律递减[3];Babin M,Sasaki H等人研究表明,CDOM具有相对稳定的吸收光谱特征,可用指数衰减率来定量表示.黄色物质的光吸收特性表现为在紫外可见光区最高,到红外光谱区降为零[14].在我国,孔德星等通过对长江口海域黄色物质的研究发现,随着波长的增加,吸收系数基本呈指数衰减[15],这与朱建华等对东海的研究[16]、李猛等对厦门湾的研究[17]是一致的,也符合Bricaud的指数模型;但周虹丽等对青海湖水色要素吸收光谱的研究发现光谱在750 nm波段有强烈的吸收峰[18];黄昌春等对太湖水体的CDOM光谱吸收系数研究中发现,在275 nm附近存在一显著的肩[19],该肩值与类似研究[20,21]的报道结果一致;张运林等通过对云南高原湖泊群的黄色物质光谱吸收特性的研究发现,越到短波不同湖泊的黄色物质吸收曲线差异越大[22].4 常用测量计算方法目前对CDOM的研究方法有两大类:化学方法和物理方法.化学方法一般采用吸浮法,过程很复杂,而且只能获得CDOM的主要成分,限制了人们对它的研究,因此,CDOM的形成、成分和演化机理的研究逐渐从生化领域跨到物理领域.由于水体中CDOM含量很低,组成复杂,物理方法通常以水体光学性质为基础,以此获得CDOM有关指标,并根据其光吸收特点对水体中CDOM进行相对定量、定性研究.由于CDOM具有荧光特性,荧光光谱技术已成为开展CDOM研究的重要手段,被用于CDOM的定性定量及来源、分布及其变化规律的研究[23].4.1 光谱吸收系数4.1.1 测量计算由于黄色物质成分的复杂性,目前尚无法确定其浓度,常用的方法是用355 nm、375 nm、440 nm等波长处的吸收系数来表示CDOM的浓度[24],并以375 nm和380 nm处的吸收系数作为表征黄色物质浓度居多.吸收系数越大,对应的CDOM浓度就越高.CDOM吸收系数的测定相对比较简单、方法成熟.根据张运林[24]对太湖区域、孔徳星等对长江口海域的研究[15],总结得出目前一般采用0.7μm GF/F过滤掉水样中大的颗粒和浮游生物细胞,然后用经过酸和Milli-Q处理过的0.2μm的聚碳酸酯滤膜(10%HCl浸泡15min后,再用纯净水充分淋洗)对其进行过滤.过滤后的样品采用分光光度计测量吸光度.根据设计的光谱,对水样的光密度进行测量,可得到不同波长的光密度D(λ).式中:γ为比色皿(光程)长度,ODs(λ)为波长为λ时水样的光密度,ODb(λ)为波长为λ时空白的光密度[25-28].目前专家学者多用光吸收系数来作为表征黄色物质浓度的有效参数.尽管它并不是真正意义上以mg/L来表示的绝对浓度,但考虑到目前测量的可靠性和技术实现的可行性,认为吸收系数可以比较准确地表征海水中所含黄色物质的浓度[29].空白是校正分析方法、提高分析准确度的一种手段.而目前国际上对如何选取空白还没有一个统一的规范,归纳起来有以下2种做法:(1)直接用纯水作为空白,以此来消除纯水对所测光密度的贡献[30];(2)将经过过滤的纯水作为空白[31],从理论上讲这种做法可以更好地消除过滤器皿对样品测定结果的影响,因而更为科学[32].Green等认为由于淡水和海水折射率的不同,会引起基线的漂移,为了消除这种漂移,CDOM的光密度D(λ)需要经过校正.具体方法是将各个波长的光密度扣除700 nm~800 nm光密度的平均值,然后再由公式(1)将校正后的光密度D(λ)转换为CDOM的吸收系数a(λ)[33].Kowalczuk等[34]也认为样品的光密度应该首先进行校正,但作法略有不同,其扣除的是650 nm~700 nm光密度的平均值.Keith等[3]认为由公式(1)所得的吸收系数并非CDOM的真实吸收系数,该数值仍包含了透过滤膜的细颗粒物质和胶体的反射和散射的影响,利用方程(1)所得的吸收系数需要按照Bricaud等提出的方法进行校正:其中:a(λ)corr是在给定波长λ时,经过散射校正后的吸收系数.此外,对测量结果的影响还存在一些其他因素.吴永森对青岛近岸现场水样进行处理,分析发现与0.45μm的滤膜相比,采用0.22μm滤膜可以更有效地过滤水样中剩余粒子,所测得的黄色物质吸收系数能更有效地反映黄色物质本身的基本量值形态,其数据更为客观、可靠[29].测量时,比色皿宽度越宽,分光光度计检测出的信号越强,信噪比越低.在CDOM浓度较高的内陆,水体比色皿宽度在1 cm~5 cm均可,对于CDOM浓度非常低的大洋和高山深水湖泊水体,比色皿宽度一般要达到10 cm甚至更宽[35].4.1.2 指数吸收模型Bricaud采集不同水域的105个样品,进行了黄色物质光吸收测量,发现从紫外到可见光波段吸收系数与波长之间近似呈指数关系,可用(3)式来模拟[3]:式中,a(λ)是CDOM的吸收系数(m-1),λ是波长(nm),λ0是参照波长(nm),一般取440 nm,S是指数函数曲线斜率参数,是CDOM吸收光谱特性研究中最关注的量值之一,反映了黄色物质指数吸收的衰减程度[36].当无法测定CDOM吸收光谱时,可以测定特征波长440 nm处吸收系数,根据文献中报道的指数函数斜率S值,利用式(3)计算得到各波长的吸收系数.指数函数斜率S值大小反映了CDOM吸收系数随波长增加而递减的程度,其与CDOM浓度无关,但与CDOM组成及波段的选择有关.影响S值的因素主要有两个:一是选用的拟合波段,不同波长范围内的S值对CDOM的分子组成(或CDOM的分子中胡敏酸和富里酸)的敏感性具有一定的差异性,UVR的S值对CDOM的分子组成最为敏感,其次是UVA,再次为UVB,UVC最为不敏感[19].Stedmon等[30]对不同波段进行拟合分析发现,S值和拟合的精度随波长的增加而降低.另一个是CDOM的组成和分子大小.根据Carder的研究,黄色物质吸收光谱比例系数(S)的变化与黄色物质的成分有关,并指出海洋中灰黄酸的比例系数为0.019 nm-1,海洋中腐殖酸比例系数为0.011 nm-1[12].Keith和Yoder认为S值的大小也会反映黄色物质组成成分的比例.以灰黄酸为主要组分的黄色物质,其S值是腐殖酸占主要成分的黄色物质的两倍.Yacobi等的研究显示,CDOM分子越大、S值越小,与440 nm处的吸收系数a*(440)存在显著的负相关[37],而张运林在对长江中下游湖泊CDOM进行研究时也发现S值与a*(355)存在显著的负相关[38].早在1975年,Maul G A 等提出海水中 S值为15μm-1[39],之后 Bricaud发现 S值在10μm-1~20μm-1之间变化,平均值为14μm-1[3],这也是海洋水色遥感生物光学模式中使用的值.之后大多数研究者的结果基本上都落入Bricaud提出的范围内.随后Markager、Vincent和Stedmont等认为应在方程(3)中引入背景常数K,用K值来规避不是由有机物所造成的基线的抬高和降低[4]:通过上式对大量数据进行计算得到淡水湖泊中的S值在10μm-1~25μm-1之间,平均值为(17.7±0.8)μm-1,海水中S值在11μm-1~17.2μm-1间变化,平均值为(14.1 ±0.4)μm-1.目前模拟CDOM光谱吸收常用的3种指数模型如表1所示[22].表1 CDOM光谱吸收系数模型Table 1 Three spectra absorption coefficientmodels of CDOM模型描述函数表达式模型参数拟合方法模型1:吸收系数取自然对数线性拟合指数模型ln a CDOM(λ)=a1-S1λ a1,S1线性拟合模型2:非线性拟合指数模型a CDOM(λ)=a2 e s2(440-λ) a2,S2 非线性拟合模型3:增加背景项指数模型a CDOM(λ)=a3 e s3(440-λ)+K a3,S3,K 非线性拟合张运林等使用这3种指数模型对云南高原湖泊(碧沽天池、碧塔海、洱海、拉什海等34个湖泊)的CDOM进行模拟对比,发现增加背景项后的指数函数模型模拟的结果最好(R2最大、RMSE最小),而模型1的拟合结果是最差的[22].模型3相对于模型1光谱斜率S均值增加6.2%,这与其他人的研究结果一致.如Stedmon等对丹麦沿海水域的研究发现,模型3在300 nm~650 nm范围内S均值比模型1增加了13%[30].4.2 荧光光谱法4.2.1 测量方法CDOM的一个重要特征是吸收紫外光后可发射长于吸收光波长的荧光,所以也称为溶解荧光有机物(Fluorescent dissolved organic matter,简称FDOM),可用荧光法进行检测.荧光法具有操作简便、灵敏度高(10-9 g/mL~10-12 g/mL)、选择性好、特效检出等优点,且不需对目标物进行分离预处理,近年来已被广泛应用于天然水体中CDOM的检测和分析[41].三维荧光光谱(EEMS)可以作为激发光谱、发射光谱及同步荧光光谱进行分析,且其操作简便、灵敏度高、选择性好、特效检出等优点,已经被广泛地用于CDOM的定性和定量研究[42].测量时采用荧光分光光度计测定,激发和发射夹缝宽度为5nm,激发波长为200 nm~435 nm,间隔为5 nm,发射波长为250 nm~600 nm,以1 nm间隔得到荧光光谱,减去Milli-Q超纯水三维荧光光谱以校正水的拉曼散射[43].4.2.2 模型关系柳先平等研究发现,在浓度低于75mg/L时,CDOM水溶液荧光强度(λex/λem=320/410 nm)与浓度呈良好的线性关系,可用线性公式y=5.519x+18.898(r2=0.988 1)表示,在低浓度范围内,利用荧光强度与浓度间的线性关系对水体中的CDOM进行定量分析[23].夏达英研究发现,海水中黄色物质浓度范围在5×10-5 g/mL~1×10-9 g/mL时,黄色物质浓度与荧光强度之间具有良好的线性关系[44].季乃云等研究发现海洋可溶性有机物的荧光强度随着荧光溶解有机物含量的增加而线性增加,而受环境因子的影响较小[45].柳先平等研究发现,CDOM水溶液的发射光谱峰面积与超纯水的拉曼峰面积的比值与浓度呈良好的线性关系(r2=0.98 23),且能避免因在不同发射波长下取荧光强度值而导致对同一水样CDOM的定量结果的差异,从而更能真实地反映实际水样的CDOM量.当CDOM的浓度范围在0.17mg/L~1.36mg/L时,面积比值与浓度之间的关系可用线性公式y=3.145 7x+3.220 7(r2=0.999 7)来表示,用此关系式反算配制的CDOM标准溶液浓度,差值与真值之比在3% ~45%之间[23].当CDOM的浓度范围在1.36 mg/L~37.50mg/L时,面积比值与浓度之间的关系可用线性公式y=1.837x+5.046 6(r2=0.999 3)来表示,用此关系式反算配制的CDOM标准溶液浓度,差值与真值之比在6% ~3.0%之间[23].5 CDOM的时空变化规律5.1 同一区域、不同时间段CDOM吸收系数存在差异雷惠等对东海4个季节水体CDOM样品进行光谱测量和分析,发现CDOM吸收系数在短波段受陆源输入影响程度的不同,有明显的差异:春秋季高值区向外海扩展较广,而且秋季向南延伸明显远于春季,对应于冲淡水转向和东南沿岸流的季节变化.吸收系数值低值区与东海表层水团、台湾暖流以及上升流有一定的关系,需要进一步研究[8].郭卫东等通过对九龙江口CDOM的研究结果也确认了其吸收系数的季节变化,并认为是丰水期径流量增加导致入海陆源物质增加,使CDOM吸收系数值增大,因此建立CDOM吸收系数相关算法时需要考虑季节因素[41].就广为关注的太湖水体,黄昌春等认为8月份CDOM吸收系数的平均值最大,其次是11月,3月最小,且8、11月份的CDOM吸收系数最大值约为3月份最大值的4倍[19].乐成峰等认为太湖梅梁湾CDOM吸收系数夏季最大,春季最小,特别是在短波紫外波段的吸收系数具有明显的差异性,且夏季的CDOM吸收系数最大值约为春季最大值的10倍[46].张运林等在同一年份对梅梁湾、大太湖的研究后认为,夏季CDOM的吸收要明显高于冬季[5].由此可见,太湖水体CDOM的吸收系数大致变化规律是夏季最大、秋季次之、春季最小.然而,王鑫等对太湖北部湖区试验,代表夏、秋、冬、春4个月各采样点280 nm 处的吸收系数平均值进行比较发现,1月>4月>7月>10月,可以看出,水体中CDOM的吸收系数从冬季到次年秋季逐渐递减[6].原因可能与CDOM组成有关,其构成主要是腐殖酸、棕黄酸等.在冬季,生长1年的浮游植物和水生生物都已经死亡,其残体和腐烂产物使水体中的可溶解性有机物含量增加,而随着季节的转暖,浮游植物开始萌发,作为植物所需的营养物质,其生长必定会消耗掉一定的CDOM,从而使水体中的CDOM比例降低,而且夏季炽热的阳光下,至少表层的CDOM会发生明显的光化学降解,从而影响水体中CDOM的组成和吸收[6].5.2 同一水体、不同区域CDOM吸收系数存在差异雷惠等对东海水体CDOM样品进行光谱测量和分析,发现CDOM吸收系数有明显的区域性差异:近岸特别是杭州湾为吸收系数高值区域,反映出以长江冲淡水为主的陆源输入特性;向外海扩展受到海水的混合稀释作用愈显著,吸收系数值逐渐减小[8].王鑫等对太湖北部湖区试验发现,CDOM吸收系数在空间上表现为:竺山湾>梅梁湾>贡湖湾>大太湖,入湖河道附近的CDOM吸收系数高于其邻近水面[6].赵巧华等利用模糊聚类的方法分析了冬、夏季全太湖有色溶解有机质(CDOM)的吸收系数谱的时空演变特征,结果表明在冬夏两季中北部湾区的CDOM的浓度均大于其南部区域,且南部CDOM浓度的空间变化较北部平缓,冬季全太湖CDOM组成的空间变化较夏季更为均匀[20].张运林等对太湖梅梁湾研究发现,总体上呈现河口和湾内各点CDOM浓度一般还是要高于湾口各点.梅梁湾内CDOM浓度呈现从河口往湾内、湾口递减的趋势[38].Del Castillo在研究Caribbean海湾时发现类似现象,离河流入海口越近,DOC浓度越高,CDOM对光的吸收越强烈[47].张运林等发现草型湖区的东太湖CDOM浓度明显要小于藻型湖区的梅梁湾.在梅梁湾,CDOM吸收系数大致呈现从湾内向湾口递减的趋势,并且位于梁溪河和直湖港入湖口的采样点CDOM吸收系数明显要大于其他各点,受湖水稀释,到湾口逐渐降到最低值,体现了入湖河流带来大量CDOM[48].Frenette等对大型浅水湖泊SaintPierre湖的研究也显示在河流入湖区的CDOM浓度均明显高于湖体本身[49],而在许多海湾的CDOM的研究中这种分布趋势更为明显[50,51].段洪涛等对太湖沿岸水体研究发现,贡湖湾CDOM吸收系数a(440)明显高于太湖其他沿岸水域;同时,梅梁湾、贡湖湾、镇湖湾、光富湾等a(440)高于沿岸水体平均值,而南部3个湖湾皆低于平均值[52].陈晓玲等调查的长江中游地区3个湖泊CDOM发现,离湖岸较近站点的a(400)高于离湖岸较远的站点[53].刘明亮等研究发现太湖入河口区和开敞区CDOM吸收系数a(355)存在显著空间差异,河口区明显大于开敞区,a(355)最大值出现在大浦河口和竺山湾漕桥河口附近,最小值出现在东太湖和胥口湾[4].宋玲玲等测得淀山湖19个采样点的水样在355 nm波长处的吸收系数不高,平均吸收系数为8.84m-1,范围在6.95m-1~10.28m-1,且湖南区略高于湖北区[54].周虹丽等对青海湖研究发现,总体上黄色物质440 nm吸收系数由岸边向湖心递减,最小值出现在海心山东边的站点.在靠近海心山周围的湖区,黄色物质的吸收系数有增大的趋势,但量值小于湖边值[18].孔德星等对长江口海域进行测定得到长江口表层水CDOM的ag(440)变化范围为1.152m-1~8.751m-1,平均为4.928 2m-1,呈现从口门外向口门内增加的趋势[15].邢小罡等通过对渤海水域的研究发现,黄色物质的ag(440)的空间变化不大,平均值最大出现在渤海湾,为0.732 2m-1,辽东湾最小,为0.461 3m-1,莱州湾0.579 3m-1,秦皇岛外海0.5363m-1[55].说明渤海海域的黄色物质在整个夏季是比较稳定的.朱建华等测得春季黄海海区近岸站点的黄色物质的吸收系数大于离岸站点的吸收系数[56].李猛等测得厦门湾CDOM,九龙江口具有最高值,西海域和同安湾次之,东侧水道最低[17].总结来看,可发现规律:一般近河口较高.从湖岸到湖中间,从海岸到海洋深处减少.5.3 同一水体,不同深度CDOM吸收系数的差异朱建华等测得春季黄海海区表层的黄色物质440 nm的吸收系数在0.05m-1~0.1m-1之间,最大值吸收系数为0.18m-1,最小值吸收系数为0.03m-1.10m深水层的黄色物质440 nm的吸收系数在0.05 m-1左右,最大值为0.25m-1,最小值为0.015m-1.近岸站点的表层值大于10m层值的现象明显,离岸站点的表层值减小,接近10m层的吸收值[56].李猛等测得厦门湾表层水CDOM的a(355)变化范围为0.29m-1~3.80m-1,平均为0.94m-1;底层水 a(355)的变化范围为 0.30m-1~3.15m-1,平均为 0.64 m-1;表层高于底层,主要受水文和生物因素影响[17].5.4 不同类型水体中CDOM吸收系数的差异杨顶田等对长江中下游湖泊的巢湖和龙感湖作了一些对比性研究,发现藻型湖泊的CDOM浓度明显高于草型湖泊,这在太湖草、藻型湖区也得到进一步验证[57].张运林等发现草型湖区的东太湖CDOM浓度明显要小于藻型湖区的梅梁湾[58].陈晓玲等调查的长江中游地区3个湖泊CDOM在400 nm处吸收系数(0.37m-1~1.77m-1)[53]与同季节长江下游地区太湖(0.84m-1~3.2m -1)研究结果基本吻合[59],与国外学者对湖泊CDOM调查的结果(0.20 m-1~4.72 m-1)也较接近,但明显高于海岸带与海洋中的CDOM吸收系数[60-62].但从各站点的数据对比来看,CDOM吸收系数在洪湖最高、梁子湖最低.张运林等发现太湖CDOM吸收系数[58]与Frenette等人在河相的大型浅水湖泊SaintPierre湖的研究结果比较接近[49],此外与Morris等人在美国东北部及科罗拉多州、阿拉斯加州的湖泊调查结果一致[60],并且都落在Kirk报道的吸收系数范围之内[63],但明显高于沿岸带和海洋中的值[26,63].张运林等对云南高原湖泊CDOM研究发现,不同湖泊间差异非常明显,最高值为最低值的21.6倍[22].与世界上同海拔高度的阿尔卑斯湖泊相比[64],云南高原湖泊水体中CDOM浓度明显偏高,与太湖等长江中下游富营养浅水湖泊相比[37,40],云南高原湖泊CDOM 吸收系数要小得多.6 CDOM的相关因素分析在沿海带水体,盐度可以作为调查CDOM的保守性示踪物,许多研究显示CDOM吸收系数与盐度存在显著性负相关[34],如孔德星对长江口海域研究发现,a(440)随盐度增加而减小,且线性相关性明显(R2=0.995 2)[15].李猛等在厦门湾也发现了类似情况(R2=0.887 8,n=39)[17].Susanne Kratzer等在。
从轮叶党参中分离到的一种新的脱水素基因(英文文献)

BMBreports338BMB reports*Corresponding author. T el: 82-31-201-2688; Fax: 82-31-202-2687;E-mail: dcyang@khu.ac.krReceived 17 October 2007, Accepted 26 December 2007K eywords: Abiotic stress, Codonopsis lanceolata , Dehydrin (DHN), Semi-quantitative RT-PCRIsolation of a novel dehydrin gene from Codonopsis lanceolata and analysis of its response to abiotic stressesRama Krishna Pulla 1,2, Yu-Jin Kim 1, Myung Kyum Kim 1, Kalai Selvi Senthil 3, Jun-Gyo In 4 & Deok-Chun Yang 1,*1Korean Ginseng Center and Ginseng Genetic Resource Bank, Kyung Hee University, Seocheon-dong, Kiheung-gu Yongin, Kyunggi-do, South Korea, 2Kongunadu Arts and Science College, Coimbatore, Tamil Nadu, 641029, India. 3Avinashilingam University for Women, Coimbatore, 641043, India. 4Biopia Co., Ltd., Yongin, KoreaDehydrins (DHNs) compose a family of intrinsically unstructured proteins that have high water solubility and accumulate during late seed development at low temperature or in water-deficit conditions. They are believed to play a protective role in freez-ing and drought-tolerance in plants. A full-length cDNA encod-ing DHN (designated as ClDhn ) was isolated from an oriental medicinal plant Codonopsis lanceolata , which has been used widely in Asia for its anticancer and anti-inflammatory properties. The full-length cDNA of ClDhn was 813 bp and contained a 477 bp open reading frame (ORF) encoding a polypeptide of 159 amino acids. Deduced ClDhn protein had high similarities with other plant DHNs. RT-PCR analysis showed that different abiotic stresses such as salt, wounding, chilling and light, trig-gered a significant induction of ClDhn at different time points within 4-48 hrs post-treatment. This study revealed that ClDhn assisted C. lanceolata in becoming resistant to dehydration. [BMB reports 2008; 41(4): 338-343]INTRODUCTIONPlants have developed defensive strategies against various stresses that arise from frequent environmental fluctuations to which they are exposed. Drought and low temperatures are the most severe factors limiting plant growth and yield. More than 100 genes have been shown to be responsive to such conditions and they are believed to function either during the physiological protection of cells from water-deficiencies or temperature-changes or in the regulation of gene expression (1-3).DHNs are proteins that are known to accumulate in vegetative plant tissues under stress conditions, such as low temperature, drought, or salt-stress (2, 4-6). These proteins have been catego-rized as late embryogenesis abundant (LEA) proteins (7, 8).DHNs have been subdivided into five classes according to thepresence of highly conservative segments: YnSK 2, Kn, KnS, SKn and Y 2Kn. The K-segment (EKKIGIMDKIKEKLPG) is a conserved 15-mer lysine-rich sequence characteristic of DHNs, which may be present in one or several copies (5). The K-segment can form an amphiphathic α-helix structure that may interact with lipid components of bio-membranes and partially denatured proteins like chaperones (6, 9). The S-segment consists of contiguous ser-ine residues in the centre of the protein, which may be phosphorylated. They are involved in nuclear transport through their binding to nuclear localization signal peptides (6). The Y-segment with the consensus sequence DEYGNP, shares some similarities to the nucleotide-binding site of chaperones in plants and bacteria (5, 10). Another conserved domain contained in many DHNs is ϕ-segment (repeated Gly and polar amino acids), which interacts with and stabilizes membranes and macro-molecules, preventing structural damage and maintaining the activity of essential enzymes (11).DHNs have been found in the cytoplasm (12), nucleus (12, 13), mitochondria (14), vacuole (15), and chloroplasts (16). They are known to associate with membranes (17, 18), pro-teins (19) and excess salt ions (15, 20). Several DHN genes have been isolated and characterized from different species, including cor47, erd10 and erd14 from Arabidopsis thaliana ; Hsp90, BN59, BN115 and Bnerd10 from Brassica napus ; cor39 and wcs19 from Triticum aestivum (bread wheat); and cor25 from Brassica rapa subsp. Pekinensis (21). Many studies have reported a positive correlation between the accumulation of DHN transcripts or proteins and tolerance to freezing, drought, and salinity (12, 17, 22-24). Moreover, mod-ulation of transcripts by light has been reported for many DHN-encoding genes in drought- or cold-stressed plants (25-28). Although the biochemical functions and physiological roles of DHNs are still unclear, their sequence character-izations and expression patterns suggest that they may play a positive role in plant-response and adaptation to abiotic stress that leads to cellular dehydration. Indeed, many studies have indicated that transgenic plants with DHNs have a better stress-tolerance, recovery or re-growth after drought and freez-ing stress than that of the control (8, 29, 30).Thus far, there are no reports on isolation of the DHN gene from the oriental medicinal plant Codonopsis lanceolata . ThisCodonopsis lanceolata dehydrin geneRama Krishna Pulla, et al.339BMBreportsFig. 1. Nucleotide sequence and de-duced amino acid sequence of a ClDhn cDNA isolated from C. lanceolata . Num-bers on the left represent nucleotide positions. The deduced amino acid se-quence is shown in a single-letter code below the nucleotide sequence. The as-terisk denotes the translation stop signal.Amino acids in two double boxes repre-sent the Y-segment and amino acids in a single box the S-segment, respectively.The two underlined sequences represent the K-segments.plant belongs to the family of Campanulaceae (bellflower fam-ily), which contains many famous oriental medicinal plants such as Platycodon grandiflorum (Chinese bellflower or balloon flow-er), Codonopsis pilosula and Adenophora triphylla (nan sha shen). The roots of these plants have been used as herbal drugs to treat bronchitis, cough, spasm, macrophage-mediated immune responses and inflammation, and has also been administered as a tonic (31). C. lanceolata grows in North-eastern china, Korea, and far eastern Siberia. Despite their medicinal importance, little genomic study of this plant has been carried out. In this study, we characterized an Y 2SK 2 type DHN gene from C. lanceolata and analyzed its expression in response to various abiotic stresses.RESULTS AND DISCUSSIONIsolation and characterization of the full length cDNA of the ClDhn geneAs part of a genomic project to identify genes in the medicinal plant C. lanceolata , a cDNA library consisting of about 1,000 cDNAs was previously constructed. A cDNA encoding a dehy-drin (DHN), designated ClDhn was isolated and sequenced. The sequence data of ClDhn has been deposited in GenBank under accession number AB126059. As shown in Fig. 1, ClDhn is 813 bp in length and it has an open reading frame (ORF) of 477 bp nucleotide with an 87-nucleotide upstream sequence and a 248-nucleotide downstream sequence. The ORF of ClDhn starts at nucleotide position 88 and ends at position 565. ClDhn encodes a precursor protein of 159 amino acids resi-dues with no predicted signal peptide at the N-terminal. The calculated molecular mass of the protein is approximately 16.7kDa with a predicated isoelectric point of 6.87. In the deduced amino acid sequence of ClDhn protein, the total number of neg-atively charged residues (Asp +Glu) amounted to 21 while the total number of positively charged residues (Arg +Lys) was 20. In addition, transmembrane helix prediction (TMHMMv2.0) did not identify any transmembrane helices in the deduced protein, implying that the protein did not function in the membrane but might function within the cytosolic or nuclear compartment.Homology analysisA GenBank Blastp search revealed that ClDhn had the highest sequence homology to the carrot (Daucus carota ) DHN (BAD86644) with 51% identity and 61% similarity. ClDhn also shared homology with ginseng (Panax ginseng ) DHN5 (ABF48478, 50% identity and 60% similarity), wild potato (Solanum commersonii ) DHN (CAA75798, 50% identity and 58% similarity), robusta coffee (Coffea canephora ) DHN1α (ABC55670, 47% identity and 55% similarity), grape (Vitis vin-ifera ) DHN (ABN79618, 47% identity and 57% similarity), American beech (Fagus sylvatica ) DHN (CAE54590, 46% iden-tity and 56% similarity), tobacco (Nicotiana tabacum ) DHN (BAD13498, 45% identity and 56% similarity), sunflower (Helianthus annuus ) DHN (CAC80719, 45% identity and 52% similarity), and soybean (Glycine max ) DHN (AAB71225, 44% identity and 52% similarity). The DHNs showing the highest similarities were Y 2SK 2 type DHNs except grape (Vitis vinifera ) DHN (YSK 2 type) (32). Thus ClDhn might belong to Y 2SK 2 type DHNs based on the two Y-segments, one S-segment, and two K-segments present in its amino acid sequence. Phylogenetic analysis of ten of the plant DHNs were carried out using theCodonopsis lanceolata dehydrin gene Rama Krishna Pulla, et al.340BMB reportsFig. 2. A phylogenetic tree based on DHN amino acid sequence, showing the phylogenetic relationship between ClDhn and other plant DHNs . The tree was constructed using the Clustal X method (Neighbor-joining method) and a bar represents 0.1 substitutions peramino acid position.Fig. 3. Alignment of ClDhn with the most closely related DHNs from carrot (Daucus carota , BAD86644), ginseng (Panax ginseng DHN5, ABF48478), com-merson’s wild potato (Solanum commer-sonii , CAA75798), robusta coffee (Coffea canephora , ABC55670), grape (Vitis vin-ifera , ABN79618), American beech (Fagus sylvatica , CAE54590), tobacco (Nicotiana tabacum , BAD13498), sunflower (Helian-thus annuus , CAC80719) and soybean (Glycine max , AAB71225). Gaps are marked with dashes. The conserved ami-no acid residues are shaded and Y-, S-, and K-segments are shown.Clustal X program (Fig. 2). Fig. 3 is a sequence alignment result of ClDhn and other closely related DHNs .The differential expression of ClDhn in different organs of C . lanceolataThe expression patterns of ClDhn in different C . lanceolata or-gans were examined using RT-PCR analysis. Almost similar levels of ClDhn -mRNA expression were observed in leaves and roots, whereas ClDhn was expressed in slightly higher lev-els in the stems. (Data was not shown).Expression of ClDhn in response to various stressesExpression patterns of ClDhn under various conditions were ex-amined using RT-PCR analysis. Fig. 4A showed the accumu-lation of ClDhn -mRNA in response to 100 mM ABA in MS agar. ABA is a hormone secreted when environmental conditions be-come dry. Expression of ClDhn was induced and reached a maximum level after 12 hrs, and then gradually decreased. When plants are submitted to dehydration the endogenous con-tent of ABA increases, with ABA mediating the closure of the stomata. Several studies have identified ABA as a key hormone in the induction pathway of many inducible genes including DHN , in response to drought (33-36). 100 μM of ABA in sprayCodonopsis lanceolata dehydrin geneRama Krishna Pulla, et al.341BMBreportsFig. 4. RT-PCR analyses of the expressions of ClDhn gene in the leaves of C. lanceolata at various time points (h) post-treatment with various stresses: A, 100 mM ABA; B, 100 mM NaCl; C, wounding; D, chilling and E, light treatment. Actin was used as an internal control.induced DHN-levels in Brassica napus and increased its ex-pression up to 48 hrs after treatment with ABA (37). 100 μM of ABA in MS agar induced DHN -levels in rice and cause a max-imum expression level at 1 hr post-treatment (10).Fig. 4B shows the accumulation of ClDhn mRNA in re-sponse to salt stress (100 mM NaCl). ClDhn expression was in-duced at 4 hrs post-treatment and gradually increased until 48 hrs. In Brassica napus , 250 mM NaCl added in the nutrient medium induced DHN-expression and reached a maximum at 48 hrs post-treatment (37). The application of NaCl to soil brought on a progressive decrease of the pre-dawn leaf water potential, a decrease of stomatal-conductance and a growth- reduction. Osmotic potential increase during salt treatmentcould result from Na + or Cl −absorption and from the synthesis of compatible compounds (38).Under wounding stress, ClDhn gene transcription was in-duced at 4 hrs post-treatment and gradually increased until 48 hrs (Fig. 4C). Richard et al . (39) discussed that the cumulative effect of wounding on transcript accumulation could also be associated with greater water-loss through more open surfaces arising from the wounding treatment.Under cold treatment, increase of ClDhn transcripts was ob-served at 4 hrs post-treatment and gradually increased until 48 hrs (Fig. 4D). Induction of DHN by low temperatures has been observed in numerous plants (17, 38). Overexpression of citrus DHN improved the cold tolerance in tobacco (18). Overexpre-ssion of multiple DHN genes in Arabidopsis resulted in accu-mulation of the corresponding DHNs to levels similar or higher than in cold-acclimated wild-type plants (24). Another example showed that overexpression of the acidic DHN WCOR410 could improve freezing tolerance in transgenic strawberry leaves (29). Fig. 4E shows that ClDhn gene expression was induced bylight stress and increased continuously until 48 hrs post-treat-ment. Natali et al . (40) showed that the G-box (CACGTGGC), a motif found in the promoter region of many light regulated genes, was found in the DHN gene promoter of helianthus and that DHN was responsive to light stress (41).In conclusion, we isolated a new dehydrin gene (ClDhn ) from C. lanceolata and characterized its expression in response to various stresses. ClDhn was induced by various stresses related to wa-ter-deficiency (ABA, salt, wounding and cold) and was induced by light, similar to other DHN genes isolated from other plants.MATERIALS AND METHODSPlant materialsCodonopsis lanceolata were grown in vitro on MS medium supplemented with 3% sucrose and 0.8% agar under the 16 hrs light and 8 hrs dark period. Its growth was maintained by regular subculture every 4 weeks. Abiotic stress studies were carried out on plants that were subcultured for one month. To analyze gene expression in different organs, samples were col-lected from leaves, roots and stem of C. lanceolata plants.Sequence analysesThe full-length ClDhn gene was analyzed using the softwares BioEdit, Clustal X, Mega 3 and other databases listed below; NCBI (http://www.ncbi.nlm.nih), SOPMA (http://npsa-pbil.ibcp /npsaautomat.pl?page=npsopma.html).Stress assaysTo investigate the response of the ClDhn gene to various stress-es, the third leaves with petioles from C. lanceolata were used. For treatment with ABA (100 mM) and NaCl (100 mM), leaf samples were incubated in media containing each compound at 25o C for 48 hrs. For mechanical wounding stress, excised leaves were wounded with a needle puncher (42). Chilling stress was applied by exposing the leaves to a temperature of 4o C (43). To investigate the ClDhn gene-expressions in light, leaves were incubated under an electrical lamp with a light in-tensity of 24 mol m-2 s-1 for 48 hrs. All treatments were carried out on MS media with or without the treatment solution (ABA, NaCl). All treated plant materials were immediately frozen in liquid nitrogen and stored at -70o C until further analysis.Semi-quantitative RT-PCR analysisTotal RNA was extracted from various whole plant tissues (leaves, stem, roots) of C. lancolata using the Rneasy mini kit (Qiagen, Valencia, CA, USA). For RT-PCR (reverse tran-scriptase-PCR), 800 ng of total RNA was used as a template for reverse transcription using oligo (dT) primer (0.2 mM)(INTRON Biotechnology, Inc., South Korea) for 5 mins at 75oC. The reaction mixture was then incubated with AMV Reverse Transcriptase (10 U/μl) (INTRON Biotechology, Inc., SouthKorea) for 60 mins at 42oC. The reaction was inactivated byheating the mixture at 94oC for 5 mins. PCR was then per-Codonopsis lanceolata dehydrin gene Rama Krishna Pulla, et al.342BMB reportsformed using a 1 μl aliquot of the first stand cDNA in a final volume of 25 μl containing 5 pmol of specific primers for cod-ing of ClDhn gene (forward, 5'-AAA GAG AGA GAA AAT GGC AGG TTA C-3'; reverse, 5'-GGA GTA GTT GTT GAA GTT CTC TGC T-3') were used. As a control, the primers spe-cific to the C. lanceolata actin gene were used (forward, 5'-CAA GAA GAG CTA CGA GCT ACC CGA TGG-3'; reverse, 5'-CTC GGT GCT AGG GCA GTG ATC TCT TTG CT-3'). PCR was carried out using 1 μl of taq DNA polymerase (Solgent Co., South Korea) in a thermal cycler programmed as follows:an initial denaturation for 5 mins at 95oC, 30 amplification cy-cles [30 s at 95o C (denaturation), 30 s at 53o C (annealing), and90 s at 72oC (polymerization)], followed by a final elongation for 10 mins at 72o C. Actin was used as an internal control to normalize each sample for variations in the amount of RNA used.AcknowledgementsThis work was supported by the Korea Science and Engineering Foundation (KOSEF) grant funded by the Korea government (MOST) (No. R01-2006-000-11178-0).REFERENCES1.Bray, E. A. (2002) Classification of the genes differentially expressed during water-deficit stress in Arabidopsis thali-ana : An analysis using micro array and differential ex-pression data. Ann. Bot. 89, 803-811.2.Ingram, J. and Bartels, D. (1996) The molecular basis of dehydration tolerance in plants. Annu. Rev. Plant Physiol. Plant Mol. Biol. 47, 377-403.3.Kim, S. J., Jeong, D. H., An, G. and Kim, S. R. (2005) Characterization of a drought-responsive gene, OsTPS1, identified by the T-DNA gene-trap system in rice. J. Plant Biol. 48, 371-379.4.Allagulova, Ch. R., Gimalor, F. R., Shakirova, F. M. and Vakhitov, V. A. (2003) The plant dehydrins: Structure and putative functions. Biochemistry (Mosc .) 68, 945-951.5.Close, T. J. (1996) Dehydrins: emergence of a biochemical role of a family of plant dehydration proteins. Physiol. Plant. 97, 795-803.6.Close, T. J. (1997) Dehydrins: a commonalty in the re-sponse of plants to dehydration and low temperature. Physiol. Plant. 100, 291-296.7.Dure, L., Crouch, M., Harada, J., Ho, T-HD., Mundy, J. and Quatrano, R. (1989) Common amino acid sequence domains among the LEA proteins of higher plants. Plant. Mol. Biol. 12, 475-486.8.Hara, M., Terashima, S., Fukaya, T. and Kuboi, T. (2003) Enhancement of cold tolerance and inhibition of lipid per-oxidation by citrus dehydrin in transgenic tobacco. Planta. 217, 290-298.9.Ismail, A. M., Hall, A. E. and Close, T. J. (1999) Allelic variation of a dehydrin gene cosegregates with chilling tolerance during seedling emergence. Proc. Natl. Aca. Sci. U.S.A. 96, 13566-13570.10.Lee, S. C., Lee, M. Y., Kim, S. J., Jun, S. H., An, G. andKim, S. R. (2005) Characterization of an abiotic stress-in-ducible dehydrin gene OsDhn1 in rice (Oryza sativa L .). Mol. Cells. 19, 212-218.11.Svensson J, Ismail, A. M., Palva, E. T. and Close, T. J. (2002) Dehydrins. In: Storey KB, Storey JM (eds) Sensing, signaling and cell adaptation. Elsevier Science B. V., Amsterdam, 99, pp. 155-171.12.Houde, M., Daniel, C., Lachapelle, M., Allard, F., Laliberte, S. and Sarhan, F. (1995) Immunolocalization of freezing- tolerance-associated proteins in the cytoplasm and nucleo-plasm of wheat crown tissues. Plant J. 8, 583-593.13.Godoy, J. A., Lunar, R., Torres-Schumann, S., Moreno, J., Rodrigo, R. M. and Pintor-Toro, J. A. (1994) Expression tis-sue distribution and subcellular localization of dehydrin TAS14 in salt stressed tomato plants. Plant Mol. Biol. 26, 1921-1934.14.Borovskii, G. B., Stupnikova, I. V., Antipina, A. I., Vladimirova, S. V. and Voinikov, V. K. (2002) Accumulation of dehydrin-like proteins in the mitochondria of cereals in re-sponse to cold, freezing, drought and ABA treatment. BMC Plant Biol. [electronic resource] 2, 5.15.Heyen, B. J., Alsheikh, M. K., Smith, E. A., Torvik, C. F., Seals, D. F. and Randall, S. K. (2002) The calcium-binding activity of a vacuole-associated, dehydrin-like protein is regulated by phosphorylation. Plant Physiol. 130, 675-687. 16.Mueller, J. K., Heckathorn, S. A. and Fernando, D. (2003) Identification of a chloroplast dehydrin in leaves of ma-ture plants. Int. J. Plant Sci. 164, 535-542.17.Danyluk, J., Perron, A., Houde, M., Limin, A., Fowler, B., Benhamoun, N. and Sarhan, F. (1998) Accumulation of an acidic dehydrin in the vicinity of plasma membrane dur-ing cold acclimation of wheat. Plant Cell. 10, 623-638.18.Koag, M. C., Fenton, R. D., Wilkens, S. and Close, T. J. (2003) The binding of maize DHN1 to lipid vesicles. Gain of structure and lipid specificity. Plant Physiol. 131, 309-316. 19.Rinne, P. L. H., Kaikuranta, P. L. M., van der Plas, L. H. W. and van der Schoot, C. (1999) Dehydrins in cold-accli-mated apices of birch (Betula pubescens Ehrh.): production, localization and potential role in rescuing enzyme function during dehydration. Planta. 209, 377-388.20.Alsheikh, M. K., Heyen, B. J. and Randall, S. K. (2003) Ion binding properties of the dehydrin ERD14 are dependent upon phosphorylation. J. Biol. Chem. 278, 40882-40889. 21.Fan, Z. and Wang, X. (2006) Isolation and Characterization of a Novel Dehydrin Gene from Capsella bursa-pastoris. J. Molecular Biology 40, 52-60.22.Cheng, Z., Targolli, J., Huang, X. and Wu, R. (2002) Wheat LEA genes, PMA80 and PMA1959, enhance dehydration tolerance of transgenic rice (Oryza sativa L.). Mol. Breed. 10, 71-82.23.Ismail, A. M., Hall, A. E. and Close, T. J. (1999) Purification and partial characterization of a dehydrin involved in chill-ing tolerance during seedling emergence of cowpea. Plant Physiol. 120, 237-244.24.Puhakainen, T., Hess, M. W., Mäkelä, P., Svensson, J., Heino, P. and Palva, E. T. (2004) Over expression of mul-tiple dehydrin genes enhances tolerance to freezing stress in Arabidopsis. Plant Mol. Biol. 54, 743-753.25.Chauvin, L. P., Oude, M. and Sarhan, F. (1993) A leaf-spe-cific gene stimulated by light during wheat acclimation to low temperature. Plant Mol. Biol. 23, 255-265.Codonopsis lanceolata dehydrin geneRama Krishna Pulla, et al.343 BMB reports 26.Crosatti, C., Polverino, D. L. P., Bassi, R. and Cattivelli, L.(1999) The interaction between cold and light controls the expression of the cold-regulated barley gene cor14b and the accumulation of the corresponding protein. Plant Physiol. 119, 671-680.27.Ohno, R., Takumi, S. and Nakamura, C. (2003) Kinetics oftranscript and protein accumulation of a low-molecular weight wheat LEA D-11 dehydrin in response to low temperature. J. Plant Physiol. 160, 193-200.28.Panta, G. R., Rieger, M. W. and Rowland, L. J. (2001)Effect of cold and drought stress on blue berry dehydrin accumulation. J. Hort. Sci. Biotech. 76, 549-556.29.Houde, M., Dallaire, S., N’Dong, D. and Sarhan, F. (2004)Over expression of the acidic dehydrin WCOR410 im-proves freezing tolerance in transgenic strawberry leaves. Plant Biotech. J. 2, 381-388.30.Yin, Z., Pawlowicz, I., Bartoszewski, G., Malinowski, R.,Malepszy, S. and Rorat, T. (2004) Transcritional expression of a Solanum sogarandinum pGT Dhn10 gene fusion in cucumber, and its correlation with chilling tolerance in transgenic seedling. Cell Mol. Biol. Lett. 9, 891-902.31.Lee, K. T., Choi, J., Jung, W. T., Nam, J. H., Jung, H. J. andPark, H. J. (2002) Structure of a New Echinocystic Acid Bisdesmoside Isolated from Codonopsis lanceolata Roots and the Cytotoxic Activity of Prosapogenins. J. Agric. Food Chem. 50, 4190-4193.32.Xiao, H. and Nassuth, A. (2006) Stress- and development-induced expression of spliced and unspliced transcripts from two highly similar dehydrin1 genes in V. riparia and V . vinifera. Plant Cell Rep. 25, 968-977.33.Bray, E. A. (1997) Plant responses to water deficit. TrendsPlant Sci. 2, 48-54.34.Chandler, P. M. and Robertson, M. (1994) Gene expressionregulated by abscisic acid and its relation to stress tolerance. Annu. Rev. Plant Physiol. Plant Mol. Biol. 45, 113-141.35.Rabbani, M. A., Maruyama, K., Abe, H., Kyan, M. A.,Katsura, K., Yoshiwara, K., Shinozaki, K. and Yamaguchi- Shinozaki, K. (2003) Monitoring expression profiles of ricegenes under cold drought and high salinity and abscisic acid application using cDNA micro array and RNA gel-blot analyses. Plant Physio. 133, 1755-1767.36.Shinozaki, K. and Yamaguchi-Shinozaki, K. (1996) Mole-cular responses to drought and cold stress. Curr. Opin. Biotechnol. 7, 161-167.37.Deng, Z. X., Pang, Y. Z., Kong, W. W., Chen, Z. H., Wang, X. L., Liu, X. J., Pi, Y., Sun, X. F. and Tang, K. X. ( 2005) A novel ABA dependent dehydrin ERD10 gene from Brassica napus. DNA seq. 16, 28-35.38.Caruso, A., Morabito, D., Delmotte, F., Kahlem, G. and Carpin, S. (2002) Dehydrin induction during drought and osmotic stress in Populus. Plant Physiol. Biochem. 40, 1033-1042.39.Richard, S., Morency, M. J., Drevet, C., Jouanin, L. and S´eguin, A. (2000) Isolation and characterization of a de-hydrin gene from white spruce induced upon wounding, drought and cold stresses. Plant Mol. Biol. 43, 1-10.40.Natali., Giordani, T., Lercari, B., Maestrini, P., Cozza, R., Pangaro, T., Vernieri, P., Martinelli, F. and Cavallini. (2007) A. Light induces expression of a dehydrin-encoding gene during seedling de-etiolation in sunflower (Helianthus an-nuus L .). J. Plant Physiol. 164, 263-273.41.Menkens, A. E., Schindler, U. and Cashmore, A, R. (1995) The G-box: a ubiquitous regulatory DNA element in plants bound by the GBF family of bZIP proteins. Trends Biochem Sci. 20, 506-510.42.Huh, G. H., Lee, S. J., Bae, Y. S., Liu, J. R. and Kwak, S. S. (1997) Molecular cloning and characterization of cDNAs for anionic and neutral peroxidases from suspension cul-tured cells of sweet potato and their differential expression in response to stress. Mol. Gen. Genet. 255, 382-391.43.Wu, W., Pang, Y., Shen, G., Lu, J., Lin, J., Wang, J., Sun, X. and Tang, K. (2006) Molecular Cloning, Characterization and Expression of a Novel Trehalose-6-phosphate Synthase Homologue from Ginkgo biloba. J. Biochem. Mol. Biol. 39, 158-166.。
自然光和UV辐照下二级出水DOM及毒性的变化
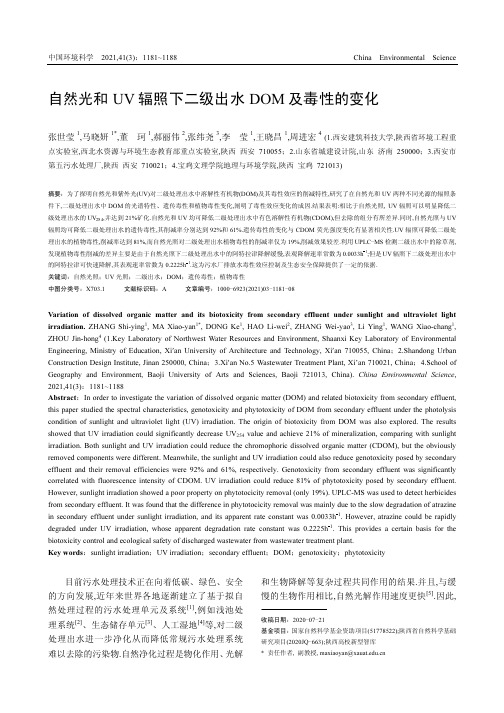
中国环境科学 2021,41(3):1181~1188 China Environmental Science 自然光和UV辐照下二级出水DOM及毒性的变化张世莹1,马晓妍1*,董珂1,郝丽伟2,张纬尧3,李莹1,王晓昌1,周进宏4(1.西安建筑科技大学,陕西省环境工程重点实验室,西北水资源与环境生态教育部重点实验室,陕西西安 710055;2.山东省城建设计院,山东济南 250000;3.西安市第五污水处理厂,陕西西安 710021;4.宝鸡文理学院地理与环境学院,陕西宝鸡 721013)摘要:为了探明自然光和紫外光(UV)对二级处理出水中溶解性有机物(DOM)及其毒性效应的削减特性,研究了在自然光和UV两种不同光源的辐照条件下,二级处理出水中DOM的光谱特性、遗传毒性和植物毒性变化,阐明了毒性效应变化的成因.结果表明:相比于自然光照, UV辐照可以明显降低二级处理出水的UV254,并达到21%矿化.自然光和UV均可降低二级处理出水中有色溶解性有机物(CDOM),但去除的组分有所差异.同时,自然光照与UV 辐照均可降低二级处理出水的遗传毒性,其削减率分别达到92%和61%.遗传毒性的变化与CDOM荧光强度变化有显著相关性.UV辐照可降低二级处理出水的植物毒性,削减率达到81%,而自然光照对二级处理出水植物毒性的削减率仅为19%,削减效果较差.利用UPLC-MS检测二级出水中的除草剂,发现植物毒性削减的差异主要是由于自然光照下二级处理出水中的阿特拉津降解缓慢,表观降解速率常数为0.0033h-1;但是UV辐照下二级处理出水中的阿特拉津可快速降解,其表观速率常数为0.2225h-1.这为污水厂排放水毒性效应控制及生态安全保障提供了一定的依据.关键词:自然光照;UV光照;二级出水;DOM;遗传毒性;植物毒性中图分类号:X703.1 文献标识码:A 文章编号:1000-6923(2021)03-1181-08Variation of dissolved organic matter and its biotoxicity from secondary effluent under sunlight and ultraviolet light irradiation. ZHANG Shi-ying1, M A Xiao-yan1*, DONG Ke1, HAO Li-wei2, ZHANG Wei-yao3, Li Ying1, WANG Xiao-chang1, ZHOU Jin-hong4 (1.Key Laboratory of Northwest Water Resources and Environment, Shaanxi Key Laboratory of Environmental Engineering, M inistry of Education, Xi′an University of Architecture and Technology, Xi′an 710055, China;2.Shandong Urban Construction Design Institute, Jinan 250000, China;3.Xi′an No.5 Wastewater Treatment Plant, Xi’an 710021, China;4.School of Geography and Environment, Baoji University of Arts and Sciences, Baoji 721013, China).China Environmental Science, 2021,41(3):1181~1188Abstract:In order to investigate the variation of dissolved organic matter (DOM) and related biotoxicity from secondary effluent, this paper studied the spectral characteristics, genotoxicity and phytotoxicity of DOM from secondary effluent under the photolysis condition of sunlight and ultraviolet light (UV) irradiation. The origin of biotoxicity from DOM was also explored. The results showed that UV irradiation could significantly decrease UV254 value and achieve 21% of mineralization, comparing with sunlight irradiation. Both sunlight and UV irradiation could reduce the chromophoric dissolved organic matter (CDOM), but the obviously removed components were different. Meanwhile, the sunlight and UV irradiation could also reduce genotoxicity posed by secondary effluent and their removal efficiencies were 92% and 61%, respectively. Genotoxicity from secondary effluent was significantly correlated with fluorescence intensity of CDOM. UV irradiation could reduce 81% of phytotoxicity posed by secondary effluent. However, sunlight irradiation showed a poor property on phytotocicity removal (only 19%). UPLC-MS was used to detect herbicides from secondary effluent. It was found that the difference in phytotocicity removal was mainly due to the slow degradation of atrazine in secondary effluent under sunlight irradiation, and its apparent rate constant was 0.0033h-1. However, atrazine could be rapidly degraded under UV irradiation, whose apparent degradation rate constant was 0.2225h-1. This provides a certain basis for the biotoxicity control and ecological safety of discharged wastewater from wastewater treatment plant.Key words:sunlight irradiation;UV irradiation;secondary effluent;DOM;genotoxicity;phytotoxicity目前污水处理技术正在向着低碳、绿色、安全的方向发展,近年来世界各地逐渐建立了基于拟自然处理过程的污水处理单元及系统[1],例如浅池处理系统[2]、生态储存单元[3]、人工湿地[4]等,对二级处理出水进一步净化从而降低常规污水处理系统难以去除的污染物.自然净化过程是物化作用、光解和生物降解等复杂过程共同作用的结果.并且,与缓慢的生物作用相比,自然光解作用速度更快[5].因此,收稿日期:2020-07-21基金项目:国家自然科学基金资助项目(51778522);陕西省自然科学基础研究项目(2020JQ-663);陕西高校新型智库* 责任作者, 副教授,*******************.cn1182 中国环境科学 41卷自然光解过程更值得引起关注.污水厂二级出水含有残留的溶解性微生物代谢产物、天然有机物和痕量有机物[6]等溶解性有机物(DOM),其中小分子痕量有机污染物和大分子溶解性物质是污水毒性效应的主要来源[7-8].痕量有机污染物较难被传统的污水处理工艺降解[9],因而污水厂二级出水中仍含有较多内分泌干扰物、杀虫剂、药品和个人护理产品等痕量有机污染化合物[10],可引起光合抑制效应、内分泌干扰效应、DNA 损伤和突变等多种生物毒性效应[11-13].前期研究结果表明,二级处理出水仍具有遗传毒性和植物毒性[14-15].二级出水中残留的DOM也可与消毒剂产生消毒副产物,从而增加出水的遗传毒性[16].由于二级出水中大分子溶解性有机物组分复杂、结构各异,因而常采用荧光特性表征其变化.大量研究表明在自然净化过程中农药[17-18]、抗生素[19-23]、药物[24-26]等痕量有机污染物可通过自然光光解作用得以衰减.痕量有机物既可以通过自然光直接光解[27],也可以通过被自然光照下由污水中的有机物产生的水生活性氧(ROS)氧化从而间接光解[28].Niu等[29]指出自然光照对污水中的DOM有转化和降解效果.大量研究也表明UV辐照可以降解DOM中的腐殖质组分[30-32].因而,自然光照和UV辐照均能转化和降解水体中DOM,但是在不同光源下哪些毒性效应可通过光照过程降低以及具体效率如何的研究较少.因此,本研究对比了自然光照和UV辐照对二级处理出水水质、DOM荧光特性的改变以及对二级处理出水遗传毒性和植物毒性的削减特性,同时深入分析了毒性效应削减的成因.这对污水处理技术的改进和水环境生态安全具有重要意义.1 材料与方法1.1自然光照及UV辐照实验本研究中,污水取自西安市某污水处理厂二沉池出水.污水处理厂处理能力为2×105m3/d,收集的市政生活废水采用厌氧-缺氧-好氧生物处理及紫外线消毒处理工艺.二级出水水质指标如表1所示. 为了避免吸附截留痕量有机污染物,水样首先经0.8µm Whatman GF/C玻璃纤维膜(预先450℃灼烧5h)过滤以去除水中大颗粒固体悬浮物.自然光照实验在夏季楼顶一处采光良好的区域进行,平均温度为(30±3)℃.在5L的圆柱形烧杯中倒入水样,进行自然光照处理.在自然光照的第0,4,7,11h采集水样.在辐照时间内,利用照度计(中国北京师范大学广电仪器)测量自然光在297nm、365nm和400~1000nm波长处的光强,具体如表2所示.同时,将烧杯用锡箔纸裹住,与其他样品进行相同的处理,在自然光下辐照11h,作为黑暗对照.UV辐照实验是在批处理反应器上进行,每个反应器上放有1个有效体积为5L的圆柱形烧杯、磁力搅拌器和位于反应堆中心的16W浸入式低压汞灯,具体如图1所示.低压汞灯的套管为石英玻璃,波长为254nm.采用UV-C型辐射计(中国北京师范大学广电仪器),在254nm处测量辐照度为31.7W/m2.为了使反应器温度保持在(25±1),℃将反应器置于恒温设备中,在UV辐照处理的第0,0.5,1, 2, 4, 8h分别采集水样.在实验过程中设置黑暗对照组.将采集的水样分别取100mL水样进行理化指标测定,取2L水样进行固相萃取浓缩用于除草剂浓度的测定和生物毒性的测定.图1 UV辐照实验装置示意Fig.1 Schematic diagram of UV irradiation experiment device表1污水厂二级处理出水理化指标(mg/L)Table1 Physicochemical indexes of secondary treatment effluent from wastewater treatment plant (mg/L)指标 COD Mn BOD5 TN NH4+-N TP 二级出水 19.41±2.7 6.63±1.2 9.65±1.5 0.93±0.57 0.27±0.043期 张世莹等:自然光和UV 辐照下二级出水DOM 及毒性的变化 11831.2 理化指标的测定将不同时间采集的水样用紫外-可见分光光度计(中国)测定UV 254,用总有机碳分析仪(日本岛津)测定TOC.三维荧光光谱采用F -7000荧光分光光度计进行测定.激发波长(E x)范围为220~400nm,波长间隔5nm,发射波长(E m)范围为280~500nm,波长间隔2nm.将三维荧光光谱图按照Chen 等[33]的方法分成5个区域(表2),分别在相应波长范围内,对三维荧光光谱选i 区域进行体积积分,积分公式下:ex em ex em ()d d i I ϕλλλλ=∫∫ (1)式中:φi 为区域的荧光强度积分值;λex 为激发波长,nm;λem 为发射波长,nm; I (λex λem )为相对应的荧光强度.二级出水中有色溶解性有机质(CDOM)即采用φ定量表征.表2 各荧光区域积分范围Table 2 Integral range of each fluorescence region荧光区域 荧光强度积分范围 蛋白质I 类(Ⅰ区) λex =220~250nm;λem =280~330nm 蛋白质Ⅱ类(Ⅱ区) λex =220~250nm;λem =332~380nm 富里酸类(Ⅲ区) λex =220~250nm;λem =382~500nm 代谢产物类(Ⅳ区) λex =255~400nm;λem =280~380nm 腐殖酸类(Ⅴ区)λex =255~400nm;λem =382~500nm1.3 毒性效应检测1.3.1 样品预处理 固相萃取的操作步骤如下.首先依次用10mL 正己烷和二氯甲烷(1:1)混合物、10mL 甲醇、10mL 超纯水活化Oasis HLB(waters, 6mL,500mg),然后以5~10mL/min 速度上样.待上样完成后,用10mL 的超纯水清洗柱子,然后抽真空30min,以去除柱子里面的水分.分别用10mL 甲醇、10mL 正己烷和二氯甲烷(1:1)混合物洗脱柱子.在氮吹仪上40℃水浴加热条件下缓缓吹至尽干,并用2mL 甲醇溶解.将提取物等分成两部分,一部分用于除草剂的液相色谱-质谱的测量,另一部分在40℃水浴加热条件下缓缓吹至尽干,并用1% DMSO 溶解,用于生物毒性测试.1.3.2 umu 遗传毒性检测 用鼠伤寒沙门氏菌 Salmonella typhimurium TA1535/pSK1002菌株作为umu 遗传毒性检测的试验菌种,遗传毒性检测参照ISO13829遗传毒性检测方法[34].umu 实验阳性对照采用 4-硝基喹啉-1-氧化物(4-NQO),1%的DMSO 溶液作为空白.将样品用1% DMSO 溶液以0.5倍系数梯度稀释,形成不同的浓度梯度样品用于检测.以阳性对照4-NQO 的浓度(C)或者水样的浓缩倍数(N)为横坐标,以诱导比率 IR 为纵坐标,进行线性拟合,作剂量-效应曲线,并计算诱导率IR=1.5时样品的浓度,记为IR 1.5.1.3.3 植物毒性检测 实验采用的小球藻是从中国科学院野生生物种质库—淡水藻种库购买的蛋白核小球藻(FACHB -1227).植物毒性的检测是采用郑凯等[35]建立的基于叶绿素荧光成像技术的光合抑制毒性的检测方法.样品检测在96孔平底黑色聚苯乙烯微板(美国Corning 公司)上进行,样品按照0.5倍系数稀释成7个浓度梯度,每个浓度设置三个平行,每个样品设置两个板间平行.阳性对照为农药敌草隆(Diuron),空白对照为1% DMSO 溶液.将50µL 样品或对照暴露于处于对数期的300µL 小球藻液中,待在恒温光照振荡培养箱中培养2h 后采用大面积叶绿素荧光成像系统Maxi -Imaging - PAM(德国WALZ 公司)测定荧光量子产率Y (II)值,同时计算抑制率.以阳性对照敌草隆(Diuron)的浓度(C )或者水样的浓缩倍数(N )为横坐标,以量子产率Y (II) 为纵坐标,作剂量-效应曲线获得样品或者阳性对照的 EC 50(半数效应浓度)值.1.3.4 毒性效应检测的定量表征 为了便于不同样品间进行比较,将样品的浓度转换为等效毒性效应的阳性对照的浓度.样品的umu 遗传毒性和植物毒性的当量浓度分别用TEQ 4-NQO 和TEQ diuron 表示,计算方法如式(2)和式(3)所示. 1.54-NQO 1.54-NQO IR TEQ =IR 的样品的 (2)50diuron 50EC TEQ =EC 敌草隆的样品的 (3)1.4 除草剂浓度检测及潜力计算利用HPLC/MS 进行全扫描识别出二级处理水含有西玛津、阿特拉津、莠灭净、特丁津、敌草隆和扑草净6种除草剂,使用配备有Acquity BEH C18柱(100m×2.1m×1.7m)的AcquityUPLC -XEVO TQ MS(UPLC/MS,Waters,USA)定量分析水样中除草剂浓度.以单一化合物浓度为5µg/L 和50µg/L 考察回收率,分别为120.0%~ 124.4%,110.0%~113.0%,104.0%~111.8%, 102.0%~ 103.0%,104.0%~108.4%和104.0%~104.2%.流动相1184 中 国 环 境 科 学 41卷采用溶剂A(98:2水/甲醇+0.1%甲酸)和溶剂B(乙腈),在流速0.4mL/min 下,随着时间从0min 到0.25min,溶剂A 和溶剂B 分别保持90%和10%不变,从0.25min 到4min 时,溶剂A 降到2%,溶剂B 升到98%,从4min 到5min 时,溶剂A 和溶剂B 保持不变,从5min 到5.01min 时,溶剂A 又升到90%,溶剂B 又降到10%,从5.01min 到6min 时,溶剂A 和溶剂B 分别保持90%和10%不变.植物毒性主要受光合抑制潜力的影响,分别配制不同浓度梯度的除草剂溶液并进行植物毒性检测以获得每种除草剂的EC 50.值根据式(4),以敌草隆为单位换算,计算不同除草剂的抑制潜力.式(4)中RP x 为除草剂的抑制潜力,EC 50(diuron)为敌草隆的EC 50值,EC 50(i )为第i 种除草剂的EC 50值.5050EC (diuron)RP =EC ()x i (4)2 结果与讨论 2.1 理化指标的变化2.1.1 TOC 和UV 254 对二级处理出水分别进行了11h 的自然光照和8h 的UV 辐照(平均光强31.7W/m 2),每组光照设置了黑暗对照.如表3所示,在UV 辐照下,二级处理出水的TOC 和UV 254分别降低了21%和55%.在自然光照下,TOC 和UV 254值并没有明显降低.这说明UV 辐照对二级处理出水中有机物的矿化比自然光照有更好的效果. UV 254值和物质所含芳香环数量有关[36].UV 辐照下二级处理水UV 254值明显降低,说明UV 有助于削减DOM 分子的芳香性.这也与Zhang 等[37]的研究结论一致.2.1.2 有色溶解性有机质(CDOM)浓度变化 如图2所示.随着自然光照和UV 辐照时间的延长,各组分荧光强度积分值(φi )逐渐下降.在自然光照超过7h 之后,荧光强度基本保持不变,说明CDOM 的去除效果已稳定.在自然光照下,Ⅰ区至Ⅴ区的去除率分别为7.53%、27.30%、42.97%、22.66%和44.64%,CDOM 总去除率为38.70%.富里酸类(42.97%)和腐殖酸类(44.64%)在5种CDOM 组分中去除效果较好,优于蛋白类物质和代谢产物类物质(图2a).这和薛爽等[38]对天然太阳光辐射下水体中DOM 组分的光降解研究结果一致.自然光照中设置的黑暗对照组各组分荧光强度积分值在实验过程中基本保持不变.UV 辐照下,Ⅰ区至Ⅴ区的CDOM 去除率分别为49.34%、65.45%、44.48%、33.67%和43.87%,CDOM 总去除率为41.47%(图2b).UV 辐照设置的黑暗对照组水样各组分荧光强度积分值在实验过程中基本保持不变.由此可见,UV 辐照相对于自然光照对二级处理出水中蛋白质类去除效果较好,对于代谢产物类、富里酸类和腐殖酸类物质的去除差异不大.UV 辐照对二级处理出水CDOM 的总去除率略高于自然光照.结合TOC 的变化可知,两种光照对二级处理出水中CDOM 的矿化程度作用较低,可能主要是将DOM 转化为小分子有机物.Liu 等[39]的研究表明,在UV 辐照下CDOM 组分中类腐殖质组分比类蛋白组分更加容易产生光降解.本文在UV 辐照下类蛋白组分降解效果优于类腐殖质组分.这可能和DOM 电子基团和分子量差异有关[31]表3 自然光和UV 辐照下二级处理水TOC 和UV 变化 Table 3 TOC and UV changes under natural light and UVirradiation项目光照时间(h)光强/400~1000nm,(W/m 2)TOC(mg/L) UV 254(cm -1)黑暗 / 7.781 0.161 0 4.32 7.727 0.160 4 6.14 7.638 0.16 7 4.52 7.599 0.166 自然光照11 5.6×10-47.36 0.161 项目光照时间(h)光强/254nm, (W/m 2)TOC(mg/L) UV 254(cm -1)黑暗 / 7.621 0.1710 7.629 0.1720.5 6.845 0.1401 7.082 0.1362 6.842 0.122 4 6.449 0.111UV 辐照831.76.008 0.078时间(h)3期张世莹等:自然光和UV辐照下二级出水DOM及毒性的变化 1185(b)UV辐照时间(h)图2 自然光照和UV辐照下二级处理出水除草剂CDOM组分变化Fig.2 Changes of CDOM components in the secondary treated water herbicide under natural and UV light2.2 毒性效应分析(b)UV辐照图3 自然光照和UV辐照下二级处理出水遗传毒性和植物毒性变化Fig.3 Changes in genotoxicity and plant toxicity of secondary treated water under natural and UV light由图3可知,自然光照和UV辐照下,遗传毒性和植物毒性均随着光照时间的增加而逐渐降低.自然光照11h后,遗传毒性被显著削弱,植物毒性从7h 到11h基本没有变化,说明遗传毒性和植物毒性的削减效果已趋于稳定.UV辐照4h之后,遗传毒性和植物毒性基本上也不再削减.在自然光照下,随着辐照时间的增加,二级处理出水的遗传毒性由5.41µg/L降至0.36µg/L,削减率为93.3%.植物毒性经过11h光照由314.68ng/L降至261.12ng/L,削减率为17.0%.对比UV辐照,经过8h辐照,遗传毒性由3.20µg/L降至1.25µg/L,植物毒性由68.57ng/L降至13.23ng/L,遗传毒性和植物毒性削减率分别为60.9%和80.7%.Jia等[40]的研究发现,UV光解作用可使再生水中植物毒性降低52%,遗传毒性增加35%.植物毒性与本文研究结果一致,遗传毒性与本文研究结果相反,这可能与所施加的紫外灯类型,辐射强度,以及水体pH值等差异有关[41].自然光照与UV辐照设置的黑暗对照组中,其遗传毒性及植物毒性在两组光照时间内基本保持不变.2.3毒性效应变化成因分析2.3.1遗传毒性变化成因解析对二级处理出水CDOM荧光强度和遗传毒性相关性分析结果如表4所示,自然光照下,二级处理出水总荧光强度和遗传毒性有显著的相关性(r=0.993,P<0.01).UV辐照下, 总荧光强度和遗传毒性(r=0.848,P<0.05)也有显著的相关性.自然光照下Ⅲ区(r=0.988,P<0.05)、Ⅳ区(r=0.992,P<0.01)和Ⅴ区(r=0.995,P<0.01)CDOM组分和遗传毒性相关性显著,说明腐殖质和微生物代谢产物对遗传毒性贡献较大.UV辐照下,遗传毒性和I(r=0.886,P<0.05)、II(r=0.903,P<0.05)、III(r= 0.839,P<0.05)、IV(r=0.860,P<0.05)区物质均有显著相关性,这说明遗传毒性也有可能和类蛋白组分有关.DOM分子结构复杂,可以与多种物质结合,DOM 和遗传毒性的关系还需要进一步研究.2.3.2植物毒性变化成因解析已有研究表明水体中的植物毒性主要来源于除草剂,非除草剂类化合物对植物毒性的贡献可以忽略不计[42-43].本研究在二级处理出水中共检测到6种除草剂,其EC50值及RP x值如表5所示.在自然光照和UV辐照下,二级处理出水中除草剂浓度的变化如图4所示.在11h 自然光照下,6种除草剂总浓度由初始的15.2ng/L最终降至12.0ng/L,降解率为21.1%,在8h的UV辐照下,除草剂浓度由初始的11.5ng/L最终降为0,降解率为100%.显然UV辐照对除草剂的降解效果优于1186 中 国 环 境 科 学 41卷自然光照.自然光照与UV 辐照实验设置的黑暗对照组中,6种除草剂浓度基本保持不变.表4 自然光和UV 辐照下5个CDOM 组分和遗传毒性的相关系数Table 4 Correlation coefficient of 5CDOM components and genotoxicity under natural light and UV light光照遗传毒性Ⅰ区Ⅱ区Ⅲ区Ⅳ区Ⅴ区总荧光强度自然光照 0.625 0.947 0.988* 0.992** 0.995** 0.993**UV 辐照 TEQ 4-NQO0.886* 0.903* 0.839* 0.860* 0.811 0.848* 注: *为P <0.05,为**P <0.01.表5 除草剂光合抑制潜力及浓度(ng/L)的变化Table 5 Changes of photosynthetic inhibition potential and concentration (ng/L) of herbicides自然光辐照UV 辐照化合物 EC 50 (ng/L) RP x 黑暗 0h 4h 7h 11h黑暗0h 0.5h 1h 2h 4h 8h西玛津 1.75 7.08 / / / / / 0.1 0.2 / / / / / 阿特拉津 0.61 20.47 4.6 4.6 4.5 4.2 4.5 4.0 4.0 2.8 2.2 1.5 0.1 / 莠灭净 10.95 1.13 0.2 0.2 0.3 0.2 0.2 4.9 5 0.7 0.6 0.4 0.2 / 特丁津 4.59 2.70 0.6 0.6 0.4 0.4 0.3 0.3 0.3 0.2 0.2 0.2 0.2 / 敌草隆 12.39 1.00 9.0 9.1 7.9 7.4 6.5 / / / / / / /扑草净 10.66 1.16 0.7 0.7 0.6 0.6 0.5 2 2 0.6 0.5 0.6 / /总浓度//15.1 15.213.712.81211.311.54.3 3.5 2.7 0.5 0注:“/”为未获得数据.(b)UV 辐照图4 自然光照和UV 辐照下二级处理出水中各除草剂浓度变化Fig.4 Changes of herbicide concentrations in secondary treated water under natural light and UV light irradiation阿特拉津的RP x 为20.47(表5),为6种除草剂中的最大值,并且在二级处理出水中的浓度也较高.这说明三嗪类除草剂阿特拉津对二级处理出水的植物毒性贡献最大.如图4所示,阿特拉津在11h 自然光照下几乎没有降解(由4.6ng/L 降至4.5ng/L),而在8h 的UV 辐照下降解较完全(由4.0ng/L 降至0ng/L).阿特拉津浓度在自然光照与UV 辐照随时间变化如图5所示.从图5可以得出,阿特拉津在UV 光照下的表观降解速率常数为0.2225h -1,而在自然光照下,阿特拉津的表观降解速率常数为0.0033h -1,在黑暗条件下,阿特拉津和敌草隆基本上不发生降解.Yang 等[44]指出阿特拉津是一种难降解的污染物,但可以在254nm UV 照射过程中被降解.由此可见,自然光中的紫外线强度较低是自然光对阿特拉津降解效果较差从而导致二级处理出水植物毒性削减较少的原因.另外,Fan 等[45]的研究表明在UV 辐照下腐殖酸的存在可以提高阿特拉津的光降解效果,本研究中UV 辐照组初始水样腐殖酸组分(Ⅴ区)荧光强度高于自然光照组,这可能也是阿特拉津降解差异的原因.3期张世莹等:自然光和UV辐照下二级出水DOM及毒性的变化 1187图5 阿特拉津在自然光照和UV辐照下的降解动力学曲线Fig.5 Degradation kinetics of Atrazine under natural and UVlight irradiation3结论3.1UV辐照下,二级处理出水的TOC和UV254分别降低了21%和55%;但在自然光照下,TOC和UV254值并没有明显降低. UV辐照对二级处理出水的TOC和UV254的降低效果要高于自然光照.3.2UV辐照对蛋白质I类和II类的去除能力强于自然光,去除率分别为49.34% vs. 7.53%和65.45% vs. 27.30%.自然光和UV均对二级处理出水遗传毒性有较好的削减作用,其削减率分别为93.3%和60.9%,并且遗传毒性和CDOM荧光强度有显著相关性.3.3 UV辐照对植物毒性的削减效果优于自然光照,削减率分别为80.7% 和17.0%.阿特拉津对二级处理出水植物毒性贡献最大,并且UV较自然光对阿特拉津的降解作用显著,表观降解速率常数分别为0.2225h-1和0.0033h-1;对阿特拉津极弱的降解能力是自然光对植物毒性削减效果较差的主要原因. 参考文献:[1] GARCíA J, Rousseau D P L, MORATó J, et al. Contaminant removalprocesses in subsurface-flow constructed wetlands: A Review [J].Critical Reviews in Environmental Science and Technology, 2010, 40(7):561-661.[2] Bear S E, Nguyen M T, Jasper J T, et al. Removal of nutrients, traceorganic contaminants, and bacterial indicator organisms in a demonstration-scale unit process open-water treatment wetland.Ecological Engineering, 2017,109:76-83.[3] Ma X Y, Wang X C, Wang D, et al. Function of a landscape lake in thereduction of biotoxicity related to trace organic chemicals from reclaimed water [J]. Journal of Hazardous Materials, 2016,318:663-670.[4] 杨海燕,郭金鹏,卢少勇,等.植物收割对人工湿地去除多环芳烃的影响 [J]. 中国环境科学, 2016,36(5):1554-1560.Yang H Y, Guo J P, Lu S Y, et al. Effects of plants harvesting on polycyclic aromatic hydrocarbons removal of constructed wetland [J].China Environmental Science, 2016,36(5):1554-1560.[5] Hawker D W, Cumming J L, Neale P A,et al. A screening level fate modelof organic contaminants from advanced water treatment in a potable water supply reservoir [J]. Water Research, 2011,45(2):768-780.[6] 金鑫,金鹏康,孔茜,等.污水厂二级出水溶解性有机物臭氧化特性研究 [J]. 中国环境科学, 2015,35(10):2985-2990.Jin X, Jin P K, Kong X, et al. Ozonation characteristics of dissolved effluent organic matter from the secondary effluent of WWTP [J].China Environmental Science, 2015,35(10):2985-2990.[7] Tang J Y M, Escher B I. Realistic environmental mixtures ofmicropollutants in surface, drinking, and recycled water: H erbicides dominate the mixture toxicity toward algae [J]. Environmental Toxicology & Chemistry, 2014,33(6):1427-1436.[8] Ma X Y, Dong K , Tang L , et al. Investigation and assessment ofmicropollutants and associated biological effects in wastewater treatment processes [J]. Journal of Environmental Sciences, 2020,94: 119-127.[9] 徐倩茹,赵飞,夏娜娜,等.垃圾渗滤液与再生水生物毒性的体外检测方法应用 [J]. 中国环境科学, 2020,40(9):3887-3893.Xu Q R, Zhao F, Xia N N, et al. Application of in vitro assays in the biotoxicity detection of landfill leachate and reclaimed water [J].China Environmental Science, 2020,40(9):3887-3893.[10] Park K Y, Choi S Y, Lee S H, et al. Comparison of formation ofdisinfection by-products by chlorination and ozonation of wastewater effluents and their toxicity to Daphnia magna [J]. Environmental Pollution, 2016,215:314-321.[11] Wu Q Y, H u H Y, Zhao X, et al. Effect of chlorination on theestrogenic/antiestrogenic activities of biologically treated wastewater [J]. Environmental Science & Technology, 2009,43(13):4940-4945. [12] Zhang X, Zhao X, Zhang M, et al. Safety evaluation of an artificialgroundwater recharge system for reclaimed water reuse based on bioassays [J]. Desalination, 2011,281:185-189.[13] 瞿建宏,吴伟.除草剂生产废水经微生物降解前后的毒理效应 [J].中国环境科学, 2002,22(4):297-300.Qu J H, Wu W. Toxic effects of weedicide wastewater before and after biodegradation with the microorganisms [J]. 2002,22(4):297-300. [14] 袁宏林,郝丽伟,马晓妍,等.UV/TiO2光催化过程对二级处理出水多重生物效应的削减特性研究 [J]. 环境科学学报, 2019,39(8):2499-2507.Yuan H L, H ao L W, Ma X Y, et al. The reduction of multiple biological effects of secondary treatment effluent by UV/TiO2 photocatalysis [J]. Acta Scientiae Circumstantiae, 2019,39(8):2499-2507.[15] 戴迪楠,刘永军,马晓妍,等.污水处理与回用过程对生态毒性的削减和水质安全评价 [J]. 安全与环境学报, 2017,17(4):1442-1447.Dai D N, Liu Y J, Ma X Y, et al. Ecotoxicity reduction and the safety as- sessment during the wastewater treat-ment and reuse process [J].Journal of Safety and Environment, 2017,17(4):1442-1447.[16] Li Y, Zhang X R, Yang M T,et al. Three-step effluent chlorinationincreases disinfection efficiency and reduces DBP formation and toxicity [J]. Chemosphere, 2016,168:1302-1308.[17] 刘宝林.硝基苯和有机氯农药在天然水环境中自然衰减的模拟研究[D]. 长春:吉林大学, 2007.Liu B L. Research on nature attenuation of nitrobenzene and organochlorine pesticides in nature waters [D]. Changchun: Jilin University, 2007.1188 中国环境科学 41卷[18] 邰超,张少栋,阴永光,等.太阳光下水中2,4,6-三氯酚的光解机制研究 [J]. 中国环境科学, 2016,36(8):2380-2387.Tai C, Zhang S D, Yin G Y, et al. Studies on the photodecomposition mechanism of 2,4,6-trichlorophenol in water under sunlight irradiation [J]. China Environmental Science, 2016,36(8):2380-2387.[19] Abellán M N, Giménez J, Esplugas S. Photocatalytic degradation ofantibiotics: The case of sulfamethoxazole and trimethoprim [J].Catalysis Today, 2009,144(1):131-136.[20] Sirtori C, Agueera A, Gernjak W, et al. Effect of water-matrixcomposition on Trimethoprim solar photodegradation kinetics and pathways [J]. Water Research, 2010,44(9):2735-2744.[21] Diugosz M, Zmudzki P, Kwiecien A, et al. Photocatalytic degradationof sulfamethoxazole in aqueous solution using a floating TiO2-expanded perlite photocatalyst [J]. Journal of H azardous Materials, 2015,298(15):146-153.[22] 代志峰,邰超,张少栋.等.天然水体溶解性物质对5种抗生素光解的影响 [J]. 中国环境科学, 2018,38(6):2273-2282.Dai Z F, Tai C, Zhang S D, et al. Influence of dissolved substances in natural water on the photolysis of five antibiotics [J]. China Environmental Science, 2018,38(6):2273-2282.[23] 申霞,王彬,朱静平,等.不同源胡敏酸对磺胺嘧啶紫外光降解的影响及作用机制 [J]. 中国环境科学, 2019,39(12):5210-5218.Shen X, Wang B, Zhu J P, et al. Influence and mechanism of humic acid from different sources on ultraviolet photodegradation of sulfadiazine [J]. China Environmental Science, 2019,39(12):5210-5218.[24] Prasse C, Wenk J, Jasper J T, et al. Co-occurrence of photochemicaland microbiological transformation processes in open-water unit process wetlands [J]. Environ. Sci. Technol., 2015,49:14136-14145. [25] Jasper J T, Jones Z L, Sharp J O, et al. Biotransformation of traceorganic contaminants in open-water unit process treatment wetlands [J]. Environmental Science & Technology, 2014,48(9):5136-5144. [26] Lv X T, Zhang X, Du Y, et al. Solar light irradiation significantlyreduced cytotoxicity and disinfection byproducts in chlorinated reclaimed water [J]. Water Research, 2017,125:162-169.[27] Fatta-Kassinos D, Vasquez M I, Kummerer K. Transformationproducts of Pharmaceuticals in surface waters and wastewater formed during photolysis and advanced oxidation processes - degradation, elucidation of byproducts and assessment of their biological potency [J]. Chemosphere, 2011,85(5):693-709.[28] Du Y, Wu Q Y, Lv X T, et al. Exposure to solar light reducescytotoxicity of sewage effluents to mammalian cells: Roles of reactive oxygen and nitrogen species [J]. Water Research, 2018,143:570-578. [29] Niu X Z, H arir M, Schmitt-Kopplin P, et al. Sunlight-inducedphototransformation of transphilic and hydrophobic fractions of suwannee river dissolved organic matter [J]. Science of the Total Environment, 2019,694:1-8.[30] Du Y X, Zhang Y Y, Chen F Z,et al. Photochemical reactivities ofdissolved organic matter (DOM) in a sub-alpine lake revealed by EEM-PARAFAC: An insight into the fate of allochthonous DOM in alpine lakes affected by climate change [J]. Science of the Total Environment, 2016,568:216-225.[31] Gao Z C, Lin Y L, Xu B, et al. A comparison of dissolved organicmatter transformation in low pressure ultraviolet (LPUV) and ultraviolet light-emitting diode (UV-LED)/chlorine processes [J].Science of the Total Environment, 2020,702:1-12. [32] Ávila C, Bayona J M, Martín I, et al. Emerging organic contaminantremoval in a full-scale hybrid constructed wetland system for wastewater treatment and reuse [J]. Ecological Engineering, 2015,80: 108-116.[33] Chen W, Westerhoff P, Leenheer J A, et al. Fluorescenceexcitation-emission matrix regional integration to quantify spectra for dissolved organic matter [J]. Environmental Science & Technology, 2003,37(24):5701-5710.[34] 13829:2000, I. (2000) Water quality — Determination of thegenotoxicity of water and waste water using the umu-test.[35] 郑凯,马晓妍,郝丽伟,等.基于叶绿素荧光成像技术的植物毒性检测法的建立及在环境监测中的应用 [J]. 环境科学学报, 2019,39(3): 768-773.Zheng K, Ma X Y, Hao L W, et al. The establishment of algal toxicity detection method based on chlorophyll fluorescence imaging and its application in environmental monitoring [J]. Acta Scientiae Circumstantia, 2019,39(3):768-773.[36] Uyguner C S, Bekbolet M. A comparative study on the photocatalyticdegradation of humic substances of various origins [J]. Desalination, 2005,176(1-3):167-176.[37] Zhang P, Shao Y F, Xu X J, et al. Phototransformation of biochar-derived dissolved organic matter and the effects on photodegradation of imidacloprid in aqueous solution under ultraviolet light [J]. Science of the Total Environment, 2020,724:1-9.[38] 薛爽,王超,马溪平,等.天然太阳辐射作用下冰体中溶解性有机物组分的光降解 [J]. 环境科学学报, 2015,35(10):2098-3106.Xue S, Wang C, Ma X P, et al. Photodegradation of dissolved organic matter fractions in ice under natural solar radiation [J]. Acta Scientiae Circumstantiae, 2015,35(10):3098-3106.[39] Liu S H, Feng W Y, Song F H, et al. Photodegradation of algae andmacrophyte-derived dissolved organic matter: A multi-method assessment of DOM transformation [J]. Limnologica, 2019,77:1-8. [40] Jia A, Escher B I, Leusch F D L,et al. In vitro bioassays to evaluatecomplex chemical mixtures in recycled water [J]. Water Research, 2015,80:1-11.[41] Beltran F J, Aguinaco A, Garcia-Araya J F, et al. Ozone andphotocatalytic processes to remove the antibiotic sulfamethoxazole from water[J]. Water Research, 2008,42(14):3799-3808.[42] Tang J Y M, Escher B I. Realistic environmental mixtures ofmicropollutants in surface, drinking, and recycled water: herbicides dominate the mixture toxicity toward algae [J]. Environmental Toxicology & Chemistry, 2014,33(6):1427-1436.[43] Vermeirssen E L M, Hollender J, Bramaz N, et al. Linking toxicity inalgal and bacterial assays with chemical analysis in passive samplers deployed in 21treated sewage effluents [J]. Environmental Toxicology & Chemistry, 2010,29(11):2575-2582.[44] Yang L X, Yao G X, Huang S J. Enhanced degradation of atrazine inwater by VUV/UV/Fe process: role of the in situ generated H2O2 [J].Chemical Engineering Journal, 2020,388:1-8.[45] Fan X Z, Lu B, Aijun G. Dynamics of solar light photodegradationbehavior of atrazine on soil surface [J]. Journal of Hazardous Materials, 2005,117(1):75-79.作者简介:张世莹(1994-),女,山东烟台人,西安建筑科技大学硕士研究生,从事水质安全保障方面研究.。
生物活性导向分离海洋真菌Alternaria sp.MNP801次级代谢产物
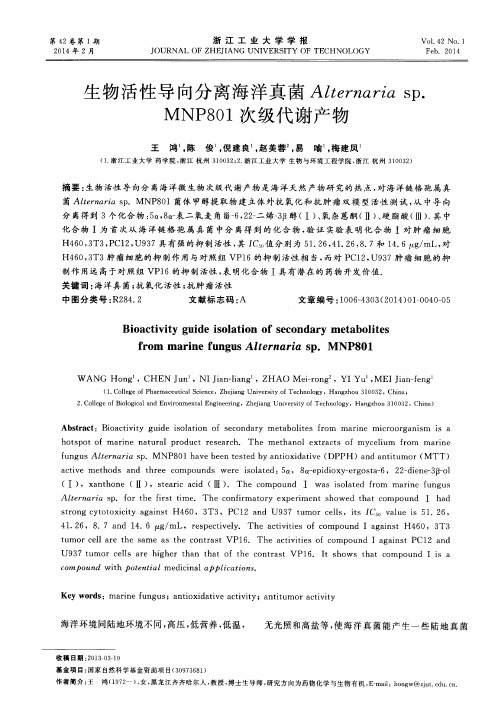
( 1 . 浙 江 工业 大 学 药 学 院 , 浙江 杭州 3 1 0 0 3 2 ; 2 . 浙江工业大学 生物与环境工程学院, 浙江 杭州 3 1 0 0 3 2 )
摘要: 生物 活性 导 向分 离海 洋微 生物 次级代 谢 产物是 海 洋天然 产物研 究 的热点 , 对 海洋链 格孢属 真
Ab s t r a c t :Bi o a c t i v i t y gu i d e i s ol a t i o n o f s e c o nd a r y me t a bo l i t e s f r om ma r i ne mi c r o or g a ni s m i s a ho t s po t o f ma r i ne n a t u r a l p r od u c t r e s e a r c h. Th e me t h a no l e xt r a c t s o f myc e l i u m f r o m ma r i ne
(工) ,x a n t h o n e (1 I) ,s t e a r i c a c i d (m ) . Th e c o mp o u n d 工 wa s i s o l a t e d f r o m ma r i n e f u n g u s
f u n g u s Al t e r n a r i a s p .M NP8 0 1 h a v e b e e n t e s t e d b y a n t i o x i d a t i v e( DP PH )a n d a n t i t u mo r( M TT)
f r o m ma r i n e f u ng u s Al t e r n ar i a s p. M NP8 0 1
基于三维荧光光谱—平行因子分析技术的蠡湖CDOM分布特征
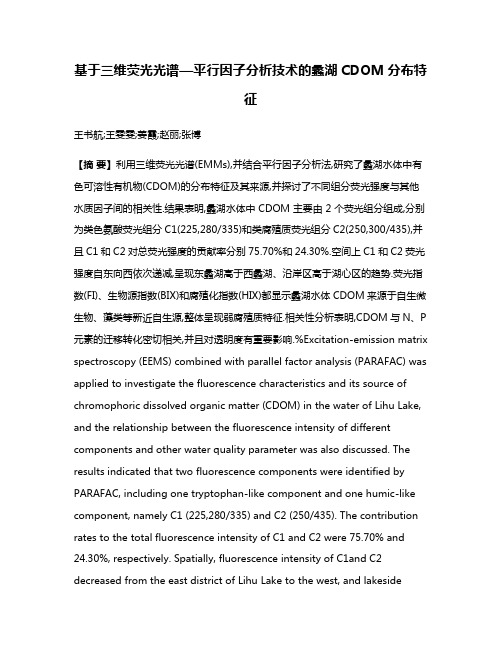
基于三维荧光光谱—平行因子分析技术的蠡湖CDOM分布特征王书航;王雯雯;姜霞;赵丽;张博【摘要】利用三维荧光光谱(EMMs),并结合平行因子分析法,研究了蠡湖水体中有色可溶性有机物(CDOM)的分布特征及其来源,并探讨了不同组分荧光强度与其他水质因子间的相关性.结果表明,蠡湖水体中 CDOM 主要由 2 个荧光组分组成,分别为类色氨酸荧光组分C1(225,280/335)和类腐殖质荧光组分C2(250,300/435),并且C1和C2对总荧光强度的贡献率分别75.70%和24.30%.空间上C1和C2荧光强度自东向西依次递减,呈现东蠡湖高于西蠡湖、沿岸区高于湖心区的趋势.荧光指数(FI)、生物源指数(BIX)和腐殖化指数(HIX)都显示蠡湖水体CDOM来源于自生微生物、藻类等新近自生源,整体呈现弱腐殖质特征.相关性分析表明,CDOM与N、P 元素的迁移转化密切相关,并且对透明度有重要影响.%Excitation-emission matrix spectroscopy (EEMS) combined with parallel factor analysis (PARAFAC) was applied to investigate the fluorescence characteristics and its source of chromophoric dissolved organic matter (CDOM) in the water of Lihu Lake, and the relationship between the fluorescence intensity of different components and other water quality parameter was also discussed. The results indicated that two fluorescence components were identified by PARAFAC, including one tryptophan-like component and one humic-like component, namely C1 (225,280/335) and C2 (250/435). The contribution rates to the total fluorescence intensity of C1 and C2 were 75.70% and 24.30%, respectively. Spatially, fluorescence intensity of C1and C2 decreased from the east district of Lihu Lake to the west, and lakesideareas was higher than lake center. Fluorescence index (FI), the index of recent autochthonous contribution (BIX) and humification index (HIX) show that CDOM in the water mainly derived from microbes, algae and other newly autochthonous sources, appeared weak humic characteristics overall. CDOM was closely related to the transformation and migration of nitrogen and phosophorus, and had important influence on transparency.【期刊名称】《中国环境科学》【年(卷),期】2016(036)002【总页数】8页(P517-524)【关键词】蠡湖;有色可溶性有机物;三维荧光光谱;平行因子分析【作者】王书航;王雯雯;姜霞;赵丽;张博【作者单位】中国环境科学研究院,环境基准与风险评估国家重点实验室,北京100012;中国环境科学研究院,环境基准与风险评估国家重点实验室,北京 100012;中国环境科学研究院,环境基准与风险评估国家重点实验室,北京 100012;中国环境科学研究院,环境基准与风险评估国家重点实验室,北京 100012;中国环境科学研究院,环境基准与风险评估国家重点实验室,北京 100012【正文语种】中文【中图分类】X524* 责任作者, 研究员,******************.cn有色可溶性有机物(CDOM)表征的是溶解性有机物中带发色团的那部分,主要由氨基酸、腐质酸、富里酸、芳烃聚合物以及一些人类活动产生的有机污染物等一系列物质组成[1-3].一方面,CDOM作为水体中光和有效辐射的重要吸收物质,对水体透明度和沉水植物的光补偿深度具有重大影响[4];另一方面,可以通过各种物理化学过程,与水体中的颗粒物以及污染物,如矿物颗粒、金属离子、有机污染物等相互作用,从而影响水体污染物的分布、迁移转化、生物可降解性以及生物毒性[5-8],同时CDOM含有丰富碳、氮、磷等湖泊生源要素,在藻类水华暴发过程中扮演了非常重要的角色[9-10],因而受到越来越多研究者的关注.对于受人类活动影响频繁的湖泊水体, CDOM的成分复杂且来源各异,目前很难对其有机成分进行全面分析.而作为较新兴的研究方法,三维荧光光谱法—平行因子分析法(EEMS-PARAFAC)在水体CDOM研究中的应用为广大水环境研究者提供了新的可能[11].Zhang等[12-14]对中国云贵高原区38个湖泊以及中营养湖泊天目湖进行了研究,识别出了腐殖质类组分和蛋白质类组分,认为其主要由流域物质以及湖泊中生物产生的内源物质构成;同时通过基于野外和实验室试验研究了藻类降解对太湖CDOM的贡献.Bai等[15]利用平行因子分析法分析后得到南黄海沿岸带和海域内CDOM荧光物质至少有4种组分:3种腐殖质类物质和1种蛋白质类物质.Singh等[16]利用EEMs-PARAFAC技术在墨西哥湾的巴拉塔里亚流域水体成功解析了CDOM的来源及组分.通过近年来水环境综合治理,蠡湖水环境恶化趋势得到有效遏制,总氮(TN)、总磷(TP)、高锰酸盐指数(CODMn)、叶绿素a(Chla)等反映湖泊富营养化状态的关键指标均显著下降,但代表感观指标的SD和ρ(SS)没有显著改善[18-19].因此,作为影响水体透明度的重要因素,CDOM的空间分布及其组分将是蠡湖水环境治理重点关注内容之一.本文尝试应用EEMS-PARAFAC技术对蠡湖有色可溶性有机物的分布特征、组成及其来源进行初步研究,为进一步揭示CDOM在蠡湖的环境行为特征以及对对水体富营养化的贡献提供基础资料.1.1 研究区域蠡湖位于太湖北部,东西长约6km,南北宽0.3~1.8km,面积约8.6km2,是一个既相对独立又与太湖相通的水体.为方便讨论,以蠡堤、宝界桥和蠡湖大桥为边界将蠡湖划分为4个区域(图1),其中,A区和B区为西蠡湖,已经开展了底泥疏浚和水生植被重建工程,水质较好;C区和D区为东蠡湖,实施了沿岸整治工程,建有长广溪湿地和金城湾公园,但底泥污染较为严重[17].1.2 样品的采集与处理与2015年4月5日在蠡湖4个湖区设置了37个采样点位,每个采样点分别采集表层(0.5m)水样,于预先处理过的棕色玻璃瓶瓶中,现场测试指标包括溶解氧(DO)、透明度(SD)等,同时记录采样点环境.水样放入保温箱中送回实验室,过玻璃纤维微孔膜(Whatman GF/F,450℃马氟炉中灼烧5h后使用)后扫描CDOM三维荧光光谱,同时测定Chla、CODMn、溶解性有机碳(DOC)、溶解性总氮(DTN)、溶解性总磷(DTP)和氨氮(NH4+-N)和硝酸盐氮(NO3--N).1.3 荧光光谱分析荧光光谱用采用日立F7000荧光分析仪进行分析,使用150W氙灯为激发光源,9PMT电压设为700V;激发波长(λEx)扫描范围为200~450nm,发射波长(λEm)扫描范围为250~600nm,激发波长和发射波长增量均设为5nm,狭缝宽度为5nm,扫描速度为1200nm/min.为了尽量降低荧光淬灭作用,对扫描的样品进行稀释,直至其在波长254nm处的紫外吸光度小于0.1.1.4 数据处理1.4.1 平行因子算法平行因子算法(PARAFAC)是基于三线性分解理论,采用交替最小二乘原理的迭代类型三维数阵分解算法,将一个由多个EEMs数据构成的三维阵列X分解为3个载荷矩阵后,对CDOM的三维荧光光谱进行解谱.将预处理好的数据,在Matlab12.0软件(美国Mathworks公司)中进行平行因子模型分析(PARAFAC),同时,采用裂半分析和残差分析检验PARAFAC模型的有效性,并确定最优的DOM组分数目[11].1.4.2 CDOM荧光光谱的空白校正为保证荧光光谱特性可比性,所得到的光谱均经过扣除超纯水空白后的矫正结果,以减少仪器条件和拉曼散射对荧光光谱的影响.通过扣除λEm<λEx+20nm的区域消除一级瑞利散射对EEMs的影响,通过扣λEm>2λEx-20nm的区域扣除二级瑞利散射对EEMs的影响,扣除部分用零替换.1.4.3 荧光强度和荧光组分占总组分比例的计算平行因子分析模型得出的是每种成分的相对荧光强度(scores),每种成分的荧光强度Ii按照以下公式计算[20]:Ii= Scorei× Exi( λmax) × Emi( λmax)式中:Scorei代表第i种成分的相对荧光强度;Exn(λmax)代表第n种成分激发负载的最大值;Emn(λmax)代表第n种成分发射负载的最大值.总荧光强度ITot和荧光组分占总组分比例Pi按照以下公式计算:1.4.4 统计分析两组数据显著性差异采用独立样本t检验,多组数据显著性差异采用单因素方差分析(one-way ANOVA).试验数据采用Excel2010、Origine9.0、suffer10.0以及SPSS19.0软件进行统计检验、绘图和分析.2.1 不同区域水质参数特征蠡湖不同区域采样点水质参数差异较大(表1).CODMn在3.31~8.12mg/L之间,平均为4.44mg/ L,C区和D区的含量较高.DOC浓度在9.16~ 22.35mg/L之间,平均为13.66mg/L,空间分布趋势与CODMn相似,两者呈显著正相关(P<0.01). DTN在C区最大,尤其是长广溪附近采样点位,都超过湖泊劣V类水质标准,可能是春季两侧绿化施肥随雨水进入蠡湖水体,导致蠡湖水体中氮,尤其是硝酸盐氮的含量明显升高.DTP在6.52~276.92µg/L之间,平均为29.13g/L,C区和D区的含量较高.SD空间分布趋势呈自西向东逐渐减小,在生态恢复较好的A区较高,平均值达到0.84m,D区最小,平均值仅为0.49m.Chl a含量在4.05~23.97mg/m3,平均为7.90mg/m3,C区显著高于其他区域;而DO在C区平均值较小,主要因为C的入湖河口较多,虽然大部分河口已经封堵,但河口水质明显差于湖体.2.2 水体CDOM的三维荧光光谱特征蠡湖各采样点CDOM的三维荧光光谱图类似,主要有3个峰,即:类蛋白T1峰(λEx/Em= 225~ 230nm/320~350nm)、类蛋白T2峰(λEx/Em=270~285nm/320~340nm)和类腐殖质A峰(λEx/Em=220~ 230nm/380~440nm),同时还能观测到微弱的C峰(λEx/Em= 280~310nm/380~440nm).一般认为A 峰和C峰反映的是外源输入的腐殖酸和富里酸形成的荧光峰值,而T1峰和T2峰则反映的是生物降解来源的色氨酸的荧光峰[1].各个点位荧光峰值出现的位置和强度的不尽相同,在A区、B区和D区类蛋白T1峰最为显著,而C区类腐殖质A峰最为显著,显示不同湖区部分水体采样点CDOM的来源不一样.蠡湖不同湖区部分水体采样点CDOM的三维荧光图谱如图2所示.利用PARAFAC模型对蠡湖水体CDOM的三维荧光光谱矩阵数据进行分析,主要解析出2个具有单一的最大发射波长CDOM荧光组分.2个荧光组分的最大激发/发射波长分布及主成分的三维荧光光谱见图3.从图3可以看出,组分1在225nm和280nm存在两个明显激发波长,最大发射波长为335± 10nm附近,反映的是生物降解的类色氨酸形成的荧光峰;组分2在250nm以及300nm处存在明显的激发波长,最大发生波长在435±10nm,反映的是腐殖酸和富里酸形成的荧光峰[1,12].2.3 水体CDOM各组分的荧光强度空间分布特征蠡湖水体中CDOM各组分空间分布特征明显.各采样点上覆水中代表类色氨酸的C1的荧光强度在7.41~21.01之间,平均为11.95;代表类富里酸的C2的荧光强度在2.53~8.07之间,平均为3.85.总体来看,C1和C2荧光强度自东向西依次递减,呈现东蠡湖高于西蠡湖、沿岸区高于湖心区的趋势(图4a、图4b).同时可以看出,C1的高值区主要集中在D区,而C2主要集中在C区的长广溪区域,说明C1和C2的来源可能不同.从各荧光组分占总组分比例的比例来看, C1占绝对优势,占总荧光强度的比例在52%~ 86%之间,平均为75.70%,其中在A区、B区、C区和D区的平均值分别为79.29%、79.00%、68.54%、75.70%.同时结合CDOM的三维荧光图谱可以明显看出,除长广溪区域个别点位外,蠡湖大部分湖区水体中CDOM主要以类蛋白质为主.3.1 CDOM来源的辨识CDOM的来源可以按陆源和生物来源进行区分,也可以按外源和内源进行区分.陆源由细菌和真菌降解流域土壤中高等动植物残体形成,多表现为类腐殖质峰占绝对优势;生物来源是指由水体中浮游生物、水生细菌、藻类等生物活动产生,多表现为类蛋白峰占绝对优势[13].荧光光谱特性是理想的表征天然水体中CDOM以及评估其来源的重要参数.荧光指数(FI)是在370nm激发波处,450nm与500nm发射波长下的荧光强度比值[21],后来修正为发射波长为470nm与520nm[22],可用来表征溶解有机质中腐殖质的来源,大于1.9说明主要来源于微生物代谢等过程,小于1.4说明陆源占主要贡献.本次研究中,采用I(370:470)/I(370:520)作为荧光指数,蠡湖各区域水体荧光指数范围为2.13~2.47,平均为2.22,说明各采样点生物来源作用大于陆源作用.生物源指数(BIX)是310nm激发波长下380nm发射波长处荧光强度与420~435nm区间最大荧光强度的比值,反映了新产生的DOM在整体DOM中所占的比例,生物源指数越高,说明新近自生源组分的比例越高.Huguet等[23]指出,BIX 在0.6~0.7之间时,具有较少的自生成分;BIX在0.7~0.8时具有中度新近自生源特征;在0.8~1.0之间时,具有较强自生源特征;大于1.0是为生物细菌活动产生.蠡湖水体BIX指数在0.89~1.13之间,平均为0.96,说明各采样点整体处于较强的自生源特征,尤其是D区,平均值达到1.0.腐殖化指数(HIX)用来表征有机质腐殖化的程度或成熟度,可以通过激发波长为254nm 时,发射波长从435~480nm的峰值面积比上300~345nm的荧光峰值面积计算.当HIX小于4 时,CDOM主要由生物活动产生,腐殖化程度较弱[24].本研究中,蠡湖水体HIX在1.00~2.60之间,平均为1.47,说明蠡湖的CDOM整体处于弱腐殖质特征.综上所述,3种荧光指数对CDOM来源表征上都具有良好的指示性,都显示出蠡湖水体CDOM来源于自生微生物、藻类的自生源.除了以上3个表征CDOM荧光特性的常用指标外,还能利用CDOM中类蛋白组分的荧光强度与类腐殖质组分的荧光强度的比值来判断水体中CDOM来源,一般认为其比值大于1说明自生源占主要优势[25-27].本次研究中,C1与C2的比值在1.08~5.94之间,平均为3.52,并且与FI、BIX和HIX等反映水体CDOM来源的指数显著相关,见图5.结合图5可以看出,rC1/C2与BIX、HIX呈极显著相关,而与FI呈负的显著相关,但相关性不明显,并且FI与BIX、HIX的相关性也不明显,说明在蠡湖这样的浅水湖泊中,用FI来指示CDOM的来源不太敏感.同时还可以看出,在图5(a)中有个明显的偏离点位,可能与其位于C区入湖河口处,周边有大片住宅有关,后期将做进一步研究. 2007年以后,无锡市政府根据蠡湖和太湖水质状况,对蠡湖与周边的一些重污染入湖河流实施闸控,保持蠡湖常年高水位,防止周边污水流入和渗入,因此,研究区的外源除了大气干湿沉降之外基本得到有效控制[19],本研究结果表明,蠡湖水体CDOM 主要来源于自生微生物、藻类的自生源,与蠡湖的现状相呼应.3.2 水体CDOM组分与其他水质参数的相关性蠡湖水体CDOM的两个荧光组分(C1和C2)之间没有明显相关性,说明水体中类蛋白质与类富里酸在组成和来源上不尽相同,结合C1和C2的空间分布特征可以看出,C1荧光强度较大的区域主要分布于D区以及C区较为开阔的区域,而C2荧光强度较大的区域主要集中在长广溪区域及入湖河口处,进一步说明,占绝对优势的C1主要来源于自生微生物、藻类的自生源,而占少量比重的C2可能来源于入湖河流及其两岸的陆源.两个荧光组分(C1和C2)都与DTN、NH4+-N、NO3--N、DTP、等营养盐呈显著正相关,说明CDOM与N、P元素的迁移转化密切相关.C1荧光强度与CODMn不呈显著正相关,而与DOC 呈极显著正相关,而C2、CTot都与CODMn和DOC呈极显著正相关,说明蠡湖水体中CDOM与DOC之间存在定量关系,可以相互替换;而C1荧光强度与CODMn不呈显著正相关,说明C1可能与CODMn没有氧化的部分有机物相关.藻类的降解是CDOM的重要来源.Zhang 等[14]野外试验和降解实验数据均显示:藻类的降解是富营养化浅水湖泊中CDOM的重要来源之一.而本研究中,CDOM的组分,尤其是占绝对优势的C1与Chla相关性并不明显,可能与本次采样主要发生在4月份,除个别区域外蠡湖的藻类生物量整体较小有关.两个荧光组分(C1和C2)的荧光强度及总荧光强度CTot都与SD呈显著负相关,说明春季CDOM对透明度有重要影响.水体CDOM组分与其他水质参数的相关性见表2.4.1 利用PARAFAC模型识别出蠡湖水体CDOM主要由2个荧光组分组成,分别为类色氨酸荧光组分C1(225, 280/335)和类腐殖质荧光组分C2(250,300/435),并且C1占绝对优势,占总荧光强度比例的平均值为75.70%.4.2 组分C1的荧光强度在7.41~21.01之间,平均为11.95; C2组分的荧光强度在2.53~8.07之间,平均为3.85.空间上,C1和C2荧光强度自东向西依次递减,呈现东蠡湖高于西蠡湖、沿岸区高于湖心区的趋势.4.3 荧光指数(FI)、生物源指数(BIX)和腐殖化指数(HIX)都显示蠡湖水体CDOM来源于自生微生物、藻类的自生源.相关性分析表明,两个荧光组分(C1和C2)都与DTN、氨氮、硝氮、DTP、DIP等营养盐呈显著正相关,说明CDOM与N、P元素的迁移转化密切相关,而与透明度呈显著负相关,说明春季CDOM对透明度有重要影响.【相关文献】[1] Baker A. Fluorescence properties of some farm wastes: implications for water quality monitoring [J]. Water Research, 2002,36(1):189-195.[2] Zhao J, Cao W, Wang G, et al. The variations in optical properties of CDOM throughout an algal bloom event [J]. Estuarine, Coastal and Shelf Science, 2009,82(2):225-232.[3] Kim T H, Waska H, Kwon E, et al. Production, degradation, and flux of dissolved organic matter in the subterranean estuary of a large tidal flat [J]. Marine Chemistry, 2012,142-144:1-10.[4] Kostoglidis A, Pattiaratchi C B, Hamilton D P. CDOM and its contribution to the underwater light climate of a shallow, microtidal estuary in south-western Australia [J]. Estuarine, Coastal and Shelf Science, 2005,63(4):469-477.[5] Yao X, Zhang, Y L, Zhu G W, et al. Resolving the variability of CDOM fluorescence to differentiate the sources and fate of DOM in Lake Taihu and its tributaries [J]. Chemosphere, 2011,82(2):145-55.[6] Ravichandran M. Interactions between mercury and dissolved organic matter-A review [J]. Chemosphere, 2004,55(3):319-331.[7] Zhou Y Q, Zhang Y L, Shi K, et al. Lake Taihu, a large, shallow and eutrophic aquatic ecosystem in China serves as a sink for chromophoric dissolved organic matter [J]. Journal of Great Lakes Research, 2015,41(2):597–606.[8] Mesfioui R, Abdulla H A, Hatcher P G. Photochemical alterations of natural and anthropogenic dissolved organic nitrogen in the York River [J]. Environmental Science and Technology, 2015, 49(1):159-167.[9] 殷燕,吴志旭,张运林,等.新安江水库夏季CDOM吸收光谱特征及来源分析 [J]. 中国环境科学, 2014,34(12):3207-3214.[10] 朱晓敏,黄清辉,李建华.咸水藻水华期溶解有机质光谱特征变化的模拟 [J]. 中国环境科学, 2009,29(1):68-72.[11] Stedmon C A, Bro R. Characterizing dissolved organic matter fluorescence with parallel factor analysis: a tutorial [J]. Limnology and Oceanography: Methods, 2008,6:572-579.[12] Zhang Y L, Zhang E L, Yin Y, et al. Charcteristic and sources of chromophoric dissolved organic matter in lakes of the Yungui Plateau, China, differcing in trophic state and altitude [J]. Limnology and Oceanorgraphy, 2010,55(6):2645-2659.[13] Zhang Y, Yin Y, Feng L, et al. Characterizing chromophoric dissolved organic matter inLake Tianmuhu and its catchment basin using excitation-emission matrix fluorescence and parallel factor analysis [J]. Water Research, 2011,45(16):5110-5122.[14] Zhang Y L, Dijk M A, Liu M L, et al. The contribution of phytoplankton degradation to chromophoric dissolved organic matter (CDOM) in eutrophic shallow lakes: Field and experimental evidence [J]. Water Research, 2009,43:4685-4697.[15] Bai Y, Su R, Shi X. Assessing the dynamics of chromophoric dissolved organic matter in the southern Yellow Sea by excitation–emission matrix fluorescence and parallel factor analysis (EEM-PARAFAC) [J]. Continental Shelf Research, 2014,88:103-116.[16] Singh S, D'Sa E J, Swenson E M. Chromophoric dissolved organic matter (CDOM) variability in Barataria Basin using excitation-emission matrix (EEM) fluorescence and parallel factor analysis (PARAFAC) [J]. Science of the Total Environment,2010,408(16):3211-22.[17] 张博,李永峰,姜霞,等.环境治理工程对蠡湖水体中磷空间分布的影响 [J]. 中国环境科学, 2013,33(7):1271-1279.[18] 姜霞,王书航,杨小飞,等.蠡湖水环境综合整治工程实施前后水质及水生态差异 [J]. 环境科学研究, 2014,27(6):595-601.[19] 王书航,王雯雯,姜霞,等.蠡湖水体氮、磷时空变化及差异性分析 [J]. 中国环境科学,2014,34(5):1268-1276.[20] 闫丽红,陈学君,苏荣国,等.2010年秋季长江口口外海域CDOM的三维荧光光谱-平行因子分析[J]. 环境科学, 2013,34(1):51-60.[21] McKnight D M, Boyer E W, Westerhoff P K, et al. Spectrofluorometric characterization of dissolved organic matter for indication of precursor organic material and aromaticity [J]. Limnology and Oceanography, 2001,46:38-48.[22] Copy R M, Mcknight D M. Fluorescence spectroscopy reveals ubiquitous presence of oxidized and reduced quinones in DOM [J]. Environmental Science and Technology, 2005,39(21):8142–8149.[23] Huguet A, Vacher L, Relexans S, et al. Properties of fluorescent dissolved organic matter in the Gironde Estuary [J]. Organic Geochemistry, 2009,40(6):706-719.[24] Zsolnay A, Baigar E, Jimenez M, et al. Differentiating with fluorescence spectroscopy the sources of dissolved organic matter in soils subjected to drying [J]. Chemosphere,1999,38(1):45-50.[25] Mopper K, Schultz, C A. Fluorescence as a possible tool for studying the nature and water column distribution of DOC components [J]. Marine Chemistry, 1993,41(1–3):229–238.[26] 刘明亮,张运林,秦伯强.太湖入湖河口和开敞区CDOM吸收和三维荧光特征 [J]. 湖泊科学, 2009,21(2):234-241.[27] 方芳,翟端端,郭劲松,等.三峡水库小江回水区溶解有机物的三维荧光光谱特征 [J]. 长江流域资源与环境, 2010,19(3): 323-328.。
生态修复后的昆承湖颗粒物和有色可溶性有机物吸收特性

生态修复后的昆承湖颗粒物和有色可溶性有机物吸收特性柳彩霞1,郭子祺1, 张宝钢1, 雷 霞1, 马茵驰21.中国科学院 遥感应用研究所 遥感科学国家重点实验室, 北京 100101;2.北京市农林科学院 北京市水产科学研究所, 北京 100068摘 要: 目前,海洋及国外内陆水体对水色三要素吸收谱的研究较多,但国内对于内陆水体的IOPs 研究区域还相对较少。
只有太湖、珠江口、巢湖、三峡等几个污染比较严重的水域有区域IOPs 的研究。
由于IOPs 具有季节性和区域性差异,为了提高二类水体水色遥感产品质量,需要更多不同区域IOPs 数据的观测和分析。
因此,本文以昆承湖水体的颗粒物和有色可溶性有机物(Chromophoric Dissolved Organic Matter, CDOM)的吸收特性为研究对象,为生态修复后的昆承湖生物光学反演算法提供数据基础,同时能从侧面定量描述自2006年以来,昆承湖水质逐渐转好的水下光场改善情况。
利用2010年4月23日在昆承湖采集的水体吸收系数数据,对总悬浮物颗粒物、藻类颗粒物、非藻类颗粒物和CDOM 的吸收特征进行了分析和研究。
结果表明,生态修复后的昆承湖水体:(1)除675nm 附近具有叶绿素吸收峰的红光波段外,非藻类颗粒物吸收系数大于藻类颗粒物吸收系数,总颗粒物吸收系数光谱分布与非藻类的吸收光谱类似,即吸收系数随波长的增大而减小;(2)非藻类颗粒物和CDOM 的吸收系数随波长的增大接近指数衰减规律且不同波段存在一定关系,(400)d a 和(440)d a 与总颗粒物浓度存在显著多项式关系;(3)多项式关系能较好的表达藻类颗粒物的单位吸收系数与叶绿素a 浓度之间的关系;(4)不同采样点的组分吸收系数对总吸收系数的贡献不同,有四种表现类型。
关键词: 昆承湖,生态修复,颗粒物,CDOM,吸收特性。
公民生态环境行为规范(试行)
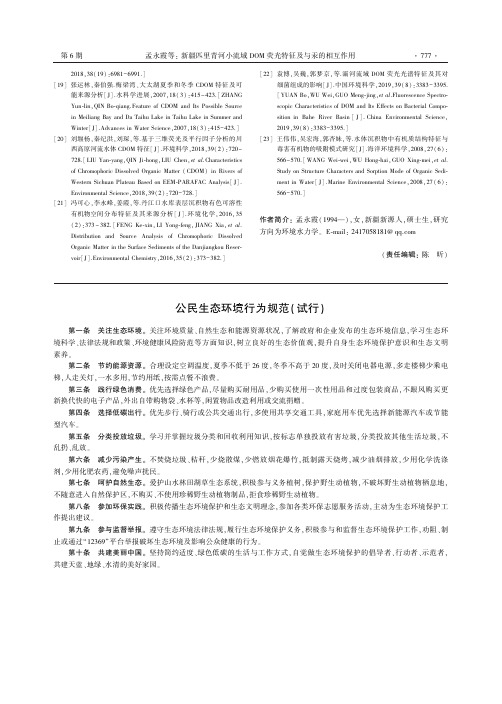
㊀第6期㊀孟永霞等:新疆匹里青河小流域DOM荧光特征及与汞的相互作用㊃777㊀㊃2018,38(19):6981-6991.][19]张运林,秦伯强.梅梁湾㊁大太湖夏季和冬季CDOM特征及可能来源分析[J].水科学进展,2007,18(3):415-423.[ZHANGYun⁃lin,QINBo⁃qiang.FeatureofCDOMandItsPossibleSourceinMeiliangBayandDaTaihuLakeinTaihuLakeinSummerandWinter[J].AdvancesinWaterScience,2007,18(3):415-423.][20]刘堰杨,秦纪洪,刘琛,等.基于三维荧光及平行因子分析的川西高原河流水体CDOM特征[J].环境科学,2018,39(2):720-728.[LIUYan⁃yang,QINJi⁃hong,LIUChen,etal.CharacteristicsofChromophoricDissolvedOrganicMatter(CDOM)inRiversofWesternSichuanPlateauBasedonEEM⁃PARAFACAnalysis[J].EnvironmentalScience,2018,39(2):720-728.][21]冯可心,李永峰,姜霞,等.丹江口水库表层沉积物有色可溶性有机物空间分布特征及其来源分析[J].环境化学,2016,35(2):373-382.[FENGKe⁃xin,LIYong⁃feng,JIANGXia,etal.DistributionandSourceAnalysisofChromophoricDissolvedOrganicMatterintheSurfaceSedimentsoftheDanjiangkouReser⁃voir[J].EnvironmentalChemistry,2016,35(2):373-382.][22]袁博,吴巍,郭梦京,等.灞河流域DOM荧光光谱特征及其对细菌组成的影响[J].中国环境科学,2019,39(8):3383-3395.[YUANBo,WUWei,GUOMeng⁃jing,etal.FluorescenceSpectro⁃scopicCharacteristicsofDOMandItsEffectsonBacterialCompo⁃sitioninBaheRiverBasin[J].ChinaEnvironmentalScience,2019,39(8):3383-3395.][23]王伟伟,吴宏海,郭杏妹,等.水体沉积物中有机质结构特征与毒害有机物的吸附模式研究[J].海洋环境科学,2008,27(6):566-570.[WANGWei⁃wei,WUHong⁃hai,GUOXing⁃mei,etal.StudyonStructureCharactersandSorptionModeofOrganicSedi⁃mentinWater[J].MarineEnvironmentalScience,2008,27(6):566-570.]作者简介:孟永霞(1994 ),女,新疆新源人,硕士生,研究方向为环境水力学㊂E⁃mail:2417058181@qq.com(责任编辑:陈㊀昕)公民生态环境行为规范(试行)第一条㊀关注生态环境㊂关注环境质量㊁自然生态和能源资源状况,了解政府和企业发布的生态环境信息,学习生态环境科学㊁法律法规和政策㊁环境健康风险防范等方面知识,树立良好的生态价值观,提升自身生态环境保护意识和生态文明素养㊂第二条㊀节约能源资源㊂合理设定空调温度,夏季不低于26度,冬季不高于20度,及时关闭电器电源,多走楼梯少乘电梯,人走关灯,一水多用,节约用纸,按需点餐不浪费㊂第三条㊀践行绿色消费㊂优先选择绿色产品,尽量购买耐用品,少购买使用一次性用品和过度包装商品,不跟风购买更新换代快的电子产品,外出自带购物袋㊁水杯等,闲置物品改造利用或交流捐赠㊂第四条㊀选择低碳出行㊂优先步行㊁骑行或公共交通出行,多使用共享交通工具,家庭用车优先选择新能源汽车或节能型汽车㊂第五条㊀分类投放垃圾㊂学习并掌握垃圾分类和回收利用知识,按标志单独投放有害垃圾,分类投放其他生活垃圾,不乱扔㊁乱放㊂第六条㊀减少污染产生㊂不焚烧垃圾㊁秸秆,少烧散煤,少燃放烟花爆竹,抵制露天烧烤,减少油烟排放,少用化学洗涤剂,少用化肥农药,避免噪声扰民㊂第七条㊀呵护自然生态㊂爱护山水林田湖草生态系统,积极参与义务植树,保护野生动植物,不破坏野生动植物栖息地,不随意进入自然保护区,不购买㊁不使用珍稀野生动植物制品,拒食珍稀野生动植物㊂第八条㊀参加环保实践㊂积极传播生态环境保护和生态文明理念,参加各类环保志愿服务活动,主动为生态环境保护工作提出建议㊂第九条㊀参与监督举报㊂遵守生态环境法律法规,履行生态环境保护义务,积极参与和监督生态环境保护工作,劝阻㊁制止或通过 12369 平台举报破坏生态环境及影响公众健康的行为㊂第十条㊀共建美丽中国㊂坚持简约适度㊁绿色低碳的生活与工作方式,自觉做生态环境保护的倡导者㊁行动者㊁示范者,共建天蓝㊁地绿㊁水清的美好家园㊂。
洱海沉积物有色可溶性有机物(CDOM)三维荧光空间分布特性及指示意义
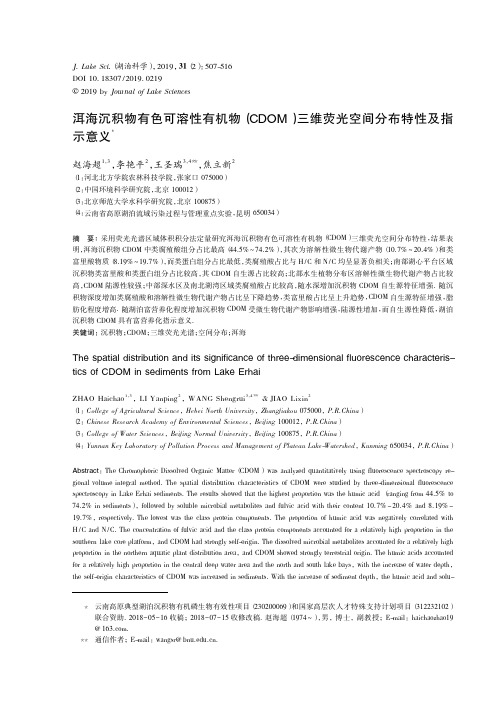
J.Lake Sci.(湖泊科学),2019,31(2):507-516DOI10.18307/2019.02192019by Journal of Lake Sciences洱海沉积物有色可溶性有机物(CDOM)三维荧光空间分布特性及指示意义*赵海超1,3,李艳平2,王圣瑞3,4**,焦立新2(1:河北北方学院农林科技学院,张家口075000)(2:中国环境科学研究院,北京100012)(3:北京师范大学水科学研究院,北京100875)(4:云南省高原湖泊流域污染过程与管理重点实验,昆明650034)摘要:采用荧光光谱区域体积积分法定量研究洱海沉积物有色可溶性有机物(CDOM)三维荧光空间分布特性,结果表明,洱海沉积物CDOM中类腐殖酸组分占比最高(44.5% 74.2%),其次为溶解性微生物代谢产物(10.7% 20.4%)和类富里酸物质(8.19% 19.7%),而类蛋白组分占比最低,类腐殖酸占比与H/C和N/C均呈显著负相关;南部湖心平台区域沉积物类富里酸和类蛋白组分占比较高,其CDOM自生源占比较高;北部水生植物分布区溶解性微生物代谢产物占比较高,CDOM陆源性较强;中部深水区及南北湖湾区域类腐殖酸占比较高,随水深增加沉积物CDOM自生源特征增强.随沉积物深度增加类腐殖酸和溶解性微生物代谢产物占比呈下降趋势,类富里酸占比呈上升趋势,CDOM自生源特征增强,脂肪化程度增高.随湖泊富营养化程度增加沉积物CDOM受微生物代谢产物影响增强,陆源性增加,而自生源性降低,湖泊沉积物CDOM具有富营养化指示意义.关键词:沉积物;CDOM;三维荧光光谱;空间分布;洱海The spatial distribution and its significance of three-dimensional fluorescence characteris-tics of CDOM in sediments from Lake ErhaiZHAO Haichao1,3,LI Yanping2,WANG Shengrui3,4**&JIAO Lixin2(1:College of Agricultural Science,Hebei North University,Zhangjiakou075000,P.R.China)(2:ChineseResearch Academy of Environmental Sciences,Beijing100012,P.R.China)(3:College of Water Sciences,Beijing Normal University,Beijing100875,P.R.China)(4:Yunnan Key Laboratory of Pollution Process and Management of Plateau Lake-Watershed,Kunming650034,P.R.China)Abstract:The Chromophoric Dissolved Organic Matter(CDOM)was analyzed quantitatively using fluorescence spectroscopy re-gional volume integral method.The spatial distribution characteristics of CDOM were studied by three-dimensional fluorescence spectroscopy in Lake Erhai sediments.The results showed that the highest proportion was the humic acid(ranging from44.5%to 74.2%in sediments),followed by soluble microbial metabolites and fulvic acid with their content10.7%-20.4%and8.19%-19.7%,respectively.The lowest was the class protein components.The proportion of humic acid was negatively correlated with H/C and N/C.The concentration of fulvic acid and the class protein components accounted for a relatively high proportion in the southern lake core platform,and CDOM had strongly self-origin.The dissolved microbial metabolites accounted for a relatively high proportion in the northern aquatic plant distribution area,and CDOM showed strongly terrestrial origin.The humic acids accounted for a relatively high proportion in the central deep water area and the north and south lake bays,with the increase of water depth,the self-origin characteristics of CDOM was increased in sediments.With the increase of sediment depth,the humic acid and solu-***云南高原典型湖泊沉积物有机磷生物有效性项目(230200069)和国家高层次人才特殊支持计划项目(312232102)联合资助.2018-05-16收稿;2018-07-15收修改稿.赵海超(1974 ),男,博士,副教授;E-mail:haichaozhao19 @163.com.通信作者;E-mail:wangsr@bnu.edu.cn.508J.Lake Sci.(湖泊科学),2019,31(2)ble microbial metabolites was decreased,the fulvic acid was increased.The self-generated source characteristics and the degree of fatification of CDOM were increased.As the lake eutrophication increased,the influence of microbial metabolites on the CDOM of sediments was increased,and its terrestrial sources was increased will its self-generated source was reduced.The CDOM of lake sediments had the indicating significance to eutrophication.Keywords:Sediments;chromophoric dissolved organic matter(CDOM);three-dimensional fluorescence spectroscopy;spatial distribution;Lake Erhai湖泊沉积物既是流域污染物输入的“汇”,又是上覆水体营养盐的“源”,记录了湖泊流域人类活动变化以及湖泊环境演化特征,是湖泊历史信息的重要来源[1].沉积物有色可溶性有机物(CDOM)是水体普遍存在的活跃有机组分[2],源于外源输入和湖泊自生两种途径.近年来国内外学者对其源解析、组分分级、赋存特征和转化行为等方面做了大量探索工作[3].CDOM是由腐殖质和非腐殖质构成的非均质混合物,其化学组成和结构复杂,可通过络合作用、光催化等方式与金属或有机污染物结合,进而影响水体的酸碱性、污染物迁移转化和生物有效性等,进而影响湖泊生态系统的稳定性[4-5].因此其在沉积物空间分布特征可反映湖泊营养物迁移转化规律[6],并记录湖泊及流域演变信息.荧光光谱技术(excitation-emission matrix fluorescence,EEM)具有灵敏度高、用量少和不破坏样品结构等优点[7],广泛用于研究湖泊和河流沉积物CDOM组成、来源和生物地球化学循环[3,5,8].区域一体化体积积分法(Fluorescence regional integration,FRI)能够对EEM进行定量分析,确定CDOM的配置和异质性[9],同时能定量区分EEM的细微变化,提高谱图的解析能力[10].Chen等[11]将EEM谱图分为5个区域,通过计算特定区域标准体积及其百分比确定对应区域特定结构荧光物质含量和相对比例.洱海作为典型高原湖泊,近年已处于“富营养化初期”[12]的关键转型期.随流域治理力度不断增加,外源污染输入逐渐减少,而沉积物内源释放对水质影响逐步加剧.作为沉积物有机质的活性组分,CDOM来源和结构是影响洱海沉积物内源释放的重要因子.本研究试图通过对洱海沉积物CDOM三维荧光光谱解析,阐明其沉积物CDOM组分空间分布特征,了解洱海沉积物的沉积及演变过程中CDOM组分变化规律及影响因素及沉积物CDOM组分变化对湖泊富营养化指示意义,以期进一步揭示洱海富营养化机制.1材料与方法1.1样品采集根据洱海水下地形及入湖负荷分布特征,于2014年8月在洱海采集14个表层样品(图1):北部(1# 5#点)、中部(6# 9#点)和南部(10# 14#点),同时在8#点(洱海最深处)和11#点(南部湖心平台)采集两根柱状样.表层沉积物采用彼得森采泥器采集,柱状样采用柱状采样器采集,柱长为50cm,现场按2cm分割装入样品袋,于4ħ保温箱内带回实验室,冷冻干燥,过100目筛后备用.1.2实验方法1.2.1沉积物CDOM荧光光谱分析取一定量的沉积物,按照1ʒ10(质量/体积)比例用1mol/L的KCl震荡提取(20ħ、200转/min、24h),离心后(5000转/min、15min)过0.45μm玻璃纤维滤膜后取上清液测定CDOM.CDOM三维荧光光谱采用Hitachi F-7000型荧光光谱分析仪测定.带通(Bandpass):激发波长(Ex)= 5nm,发射波长(Em)=5nm;扫描速度:2400nm/min.三维荧光光谱测定Ex为200 440nm,Em为250 600 nm,以Milli-Q超纯水做CK[13].1.2.2沉积物元素分析沉积物中C、N、H元素用元素分析仪(Vario ELⅢ,Elemeraor)进行测定.1.3数据分析采用Excel2007和SPSS17.0软件进行数据分析,三维荧光使用Matlab2007软件进行处理.2结果与分析2.1洱海表层沉积物CDOM荧光光谱特征运用荧光光谱FRI对洱海不同点位沉积物CDOM的EEM光谱进行定量分析.从图2可见,洱海沉积物)为络氨酸类蛋白质(Ex/Em=220 250/280 330 CDOM三维荧光光谱均可以分为5个区域:区域Ⅰ(PⅠ赵海超等:洱海沉积物有色可溶性有机物(CDOM)三维荧光空间分布特性及指示意义509图1洱海湖底地形及采样点位Fig.1Lake bottom terrain and sampling sites of Lake Erhainm),区域Ⅱ(PⅡ)为色氨酸类蛋白质(Ex/Em=220 250/330 380nm),区域Ⅲ(PⅢ)为类富里酸(Ex/Em=220 250/380 550nm),区域Ⅳ(PⅣ)为溶解性微生物代谢产物(Ex/Em=250 400/280 380nm),区域Ⅴ(PⅤ)为类腐殖酸(Ex/Em=250 400/380 550nm)[12].但不同点位的CDOM各荧光区域差异较大,北部2#和3#、中部的6#和南部13#点沉积物CDOM的PⅤ占比明显较高,南部10#和11#点沉积物CDOM的PⅠ和PⅡ占比明显较高.2.2洱海沉积物CDOM荧光组分空间分布特征洱海沉积物CDOM组分区域变化如图3所示,洱海沉积物CDOM的P(I,n)总体在1.0% 9.7%之间,平均值为3.7%,高值区分布在洱海污染物沉积区,如南部湖心平台区域(10#、11#点)和北部红山湾口区域(1#点);低值区主要分布在洱海有水生植物的浅水区,如南部湖泊出口区(14#点)和北部沙坪湾(2#点).CDOM 的P(Ⅱ,n)总体在2.3% 8.2%之间,平均值为4.7%,区域分布与P(I,n)基本相同.CDOM的P(Ⅲ,n)总体在8.2% 19.7%之间,平均值为15.0%,高值区主要分布在受污染物沉积影响较大的南部湖心平台区和北部狭口区(4#、5#点),低值区主要分布在受入湖河流冲击影响较大的北部沙坪湾口(3#点)和南部波罗江入湖口区(13#点).CDOM的P(Ⅳ,n)总体在10.7% 20.4%之间,平均值为16.8%,高值区分布在南部湖心平台区,中部510J.Lake Sci.(湖泊科学),2019,31(2)图2洱海不同点位表层沉积物CDOM三维荧光图Fig.2EEM spectra of CDOM in surface sediments of different sampling sites in Lake Erhai深水区(8#点)和北部红山湾口区,低值区分布在南部出水口区和沙坪湾.CDOM的P(Ⅴ,n)总体在44.5% 74.2%之间,平均值为59.7%,高值区分布在南部出湖口区和北部的沙坪湾,低值区分布在湖心平台.总体来看,洱海沉积物CDOM各组分占比大小顺序为类腐殖酸>溶解性微生物代谢产物>类富里酸>色氨酸类蛋白质>络氨酸类蛋白质;在北部水生植物分布区类腐殖酸占比较高,类蛋白质占比较低,受污染物沉积影响较大的南部湖心平台区域类蛋白质和类富里酸占比较高,中部深水区溶解性微生物代谢产物占比较高.洱海沉积物CDOM组分垂向变化如图4所示.可见洱海沉积物CDOM组分呈现类腐殖质和溶解性微生物代谢产物表层富集现象,随着深度的增加洱海沉积物CDOM类腐殖质和溶解性微生物代谢产物占比降低,类富里酸和类蛋白占比增高.洱海沉积物CDOM的P(I,n)随深度的增加呈波动式变化,表层高于亚表层,在28cm处出现峰值.洱海沉积物CDOM的P(Ⅱ,n)随着深度的增加呈上升趋势,6cm以下趋于稳定呈波动式变化,峰值位置与P(I,n)基本相同.洱海沉积物CDOM的P(Ⅲ,n)随深度的增加呈上升趋势,6cm以下趋于稳定呈波动式变化.洱海沉积物CDOM的P(Ⅳ,n)随深度的增加呈下降趋势,表层最高,6cm以下趋于稳定.洱海沉积物CDOM的P(Ⅴ,n)随深度的增加呈波动式下降趋势,4cm层最高.表明近年来洱海沉积物类富里酸和类蛋白占比降低,类腐殖质和溶解性微生物代谢产物增加,CDOM组分结构趋于复杂化.2.3洱海沉积物CDOM荧光参数时空变化特征洱海不同区域沉积物CDOM荧光参数变化如图5所示.荧光参数是揭示沉积物CDOM来源及降解特征的重要指标,能够在一定程度反映湖泊沉积物的来源及演化规律,进而反映湖泊营养状况.荧光指数(fluo-赵海超等:洱海沉积物有色可溶性有机物(CDOM)三维荧光空间分布特性及指示意义511图3洱海表层沉积物荧光组分空间分布Fig.3The spatial distribution of CDOM EEM components in surface sediments of Lake Erhairescence index,f450/500)是激发光波长370nm时,荧光发射光谱中450和500nm处荧光强度比值,可用来区别有机质的来源[14].Mcknight等[15]认为,f450/500>1.9表明CDOM主要来源于沉积物中微生物代谢产物,f450/500<1.4表明CDOM主要来源于外来输入和湖内营养盐的沉降.洱海表层沉积物中CDOM的f450/500在1.57 1.67之间,平均值为1.61.高值区主要分布在中部湖心区、南部湖心平台、南部罗时江入湖口、南部出湖口以及北部湖心深水区.因此洱海深水区及湖心平台沉积物中的CDOM主要来源于微生物代谢,西部和北部湖湾浅水区沉积物CDOM主要来源于外源输入.自生源指数(biological index,BIX)是激发波长254nm 下,发射波长在380和430nm处的荧光强度比值[16],亦可用来区别有机质的来源.Huguet等[16]研究表明,随着BIX的增加CDOM自生源特征增强,陆源特征降低.洱海表层沉积物BIX在0.59 1.13之间,平均值为0.76.BIX的高值区分布在中部湖心区,且随着水体深度变浅呈下降趋势.这表明随着水体深度的增加沉积物中CDOM自生源特征增强.湖心区沉积物CDOM主要是浮游植物和细菌的有机体降解产物,洱海西部及水生植物分布区陆源输入是CDOM的主要来源.洱海沉积物CDOM荧光指数垂向变化如图6所示.其中f450/500随着深度的增加呈波动式下降趋势,表层最高,4cm以下基本呈波动式变化.表明近年来洱海表层沉积物CDOM受微生物代谢产物影响增强.BIX随着深度的增加总体呈波动式上升趋势,0 10cm呈上升趋势,10cm以下呈波动式上升趋势.表明随着沉积物深度的增加CDOM自生源特征增强,根据洱海沉积物沉积速率(0.20cm/a)[17],近50年来洱海沉积物外源输入呈增加趋势,但近10年来随着洱海流域治理力度的增强,外源输入减弱,生物转化作用加强,CDOM 受微生物代谢影响增加.512J.Lake Sci.(湖泊科学),2019,31(2)图4洱海沉积物荧光组分垂向分布Fig.4The vertical distribution of CDOM EEM components in sediments of Lake Erhai3讨论3.1洱海沉积物CDOM影响因素湖泊沉积物CDOM含量及组分受多种因素影响,这些因素包括分子结构作用、有机和无机基质组分和沉积条件等[18].特别是H、C、N元素及其量比关系能够反映CDOM的结构特征.H/C比是反映有机物价键结构的重要参数,较小的H/C值表明有机质不饱和程度和芳香性较高,较大的H/C值则表明其脂肪化程度高[19].沉积物富里酸的芳香性比土壤富里酸要强,这可能与水体和沉积物中有水生植物以及微生物降解作用有关[20].研究表明随着枯落物碎屑N/C比的增加,细菌的生长效率大幅度升高(即被分配用来生长的碳部分增加)[21].从洱海沉积物CDOM各组分与元素间的相关性分析(表1)可以看出:沉积物CDOM的P (I,n)、P(Ⅱ,n)和P(Ⅲ,n)均与H/C呈显著正相关;P(Ⅴ,n)与H/C和N/C均呈显著负相关.P(Ⅱ,n)、P(Ⅲ,n)和P(Ⅳ,n)均与N/C呈显著正相关;P(Ⅱ,n)与H元素呈显著正相关.表明CDOM的P(I,n)、P(Ⅱ,n)和P(Ⅲ,n)组分不饱和程度较高,随着P(Ⅱ,n)、P(Ⅲ,n)和P(Ⅳ,n)组分的增加促进微生物的生长效率,随着P(Ⅴ,n)组分的增加细菌生长效率降低.可见湖心区和沉水植物分布区不饱和程度和芳香性较高,且随着沉积物深度的增加CDOM的脂肪化程度增高,不饱和程度和芳香性降低.南部湖心平台区、北部狭口区和西部沉积物CDOM受微生物活动影响较大,而深水区和水生植物分布区受微生物影响较少,且随着水体深度的增加微生物影响减弱,但近10年洱海沉积物微生物活力有所下降.赵海超等:洱海沉积物有色可溶性有机物(CDOM)三维荧光空间分布特性及指示意义513图5表层沉积物荧光指数的空间分布Fig.5Spatial distribution of EEM indexes of CDOM in surface sediments图6洱海沉积物荧光参数垂向分布Fig.6The vertical distribution of CDOM EEM parameter in sediments of Lake Erhai3.2洱海沉积物CDOM环境指示意义云贵高原是我国断陷湖泊聚集区域,由于流域经济和人类活动等的差异,这些湖泊富营养化程度差异较大,滇池为富营养化严重湖泊[22],程海呈现富营养化特征[23],洱海处于中营养向富营养转变的关键时期[24],泸沽湖为贫营养湖泊[25].洱海与李秋才[26]对滇池、程海和泸沽湖的研究结果对比分析如图7所示,贫营养湖泊沉积物CDOM类腐殖酸占比较低,且随着湖泊富营养化程度的增加,沉积物CDOM的f450/500呈上514J.Lake Sci.(湖泊科学),2019,31(2)升趋势,BIX呈下降趋势.洱海沉积物深水区、南部湖湾类腐殖酸占比较高,f450/500较大,表明该湖区富营养化程度较严重.此外,洱海沉积物随着深度的增加CDOM类腐殖酸占比和f450/500下降、BIX升高,表明近年来洱海富营养化加重.表1沉积物CDOM荧光组分与不同元素相关性分析Tab.1Correlations of EEM components of CDOM in the sediments and different parameters 相关系数P(I,n)P(Ⅱ,n)P(Ⅲ,n)P(Ⅳ,n)P(Ⅴ,n)H/C0.5423*0.6273*0.5227*0.3199-0.5567*N/C0.36610.5382*0.5632*0.5823*-0.5947*H/N-0.1207-0.1535-0.3189-0.35760.2936N0.11860.12730.25840.3612-0.2667C-0.3096-0.4665-0.3361-0.07060.3177H0.6141*0.38960.36810.1300-0.4079n=14;*为显著相关(P<0.05);**为极显著相关(P<0.01).图7云贵高原不同湖泊沉积物荧光参数[26]Fig.7EEM parameter of CDOM in sediments of different lakes in Yunnan-Guizhou Plateau4结论1)洱海沉积物CDOM以类腐殖酸为主,但不同区域沉积物CDOM各组分差异较大,在水生植物分布区类腐殖酸(P(Ⅴ,n))占比较高,类蛋白质(P(I,n)和P(Ⅱ,n))占比较低,南部湖心平台区类蛋白质和类富里酸(P)占比较高,深水区溶解性微生物代谢产物占比较高.深水区和北部湖湾区溶解性微生物代谢产物(Ⅲ,n)(P)占比较高.(Ⅳ,n)2)洱海沉积物CDOM随深度增加类蛋白占比呈上升趋势,类富里酸占比呈上升趋势,溶解性微生物占比呈下降趋势,类腐殖质占比呈波动式下降趋势.洱海沉积物呈现类腐殖质和溶解性微生物代谢产物表层富集现象.3)洱海沉积物CDOM在水生植物分布区陆源性较强,南部湖心平台区自生源性较强,洱海西部比东部陆源性强.随着水体深度的增加沉积物中CDOM自生源特征增强,湖心深水区主要是浮游植物和细菌的有机体降解产物.随着沉积物深度的增加CDOM自生源特征增强,陆源特征降低,受微生物代谢产物影响减弱.洱海沉积物随着深度的增加CDOM的脂肪化程度增高,不饱和程度和芳香性降低.4)富营养化湖泊DOM以生物源为主,中营养湖泊DOM以陆源为主,贫营养湖泊DOM以自生源为主,湖泊沉积物DOM具有富营养化指示意义,通过洱海沉积物CDOM组分特征可见近年来富营养化程度加重.5参考文献[1]Liu EF,Shen J,Liu XQ et al.Variation characteristics of heavy metals and nutrients in the core sediments of Taihu Lake赵海超等:洱海沉积物有色可溶性有机物(CDOM)三维荧光空间分布特性及指示意义515and their pollution history.Science in China:Series D,2006,49(1):82-91.[2]Kang S,Xing B.Humic acid fractionation upon sequential adsorption onto goethite.Langmuir,2008,24(6):2525-2531.[3]Fan CH,Chang M,Zhang YC.Spectral characteristics of dissolved organic matter(DOM)derived from water and sedi-ment in normal flow period of the intersection zone of Jing river and Wei river.Spectroscopy and Spectral Analysis,2016,36(9):2863-2869.[范春辉,常敏,张颖超.泾渭河交汇区域平水期水体和表层沉积物溶解性有机质的光谱性质.光谱学与光谱分析,2016,36(9):2863-2869.][4]Hosen JD,Mcdonough OT,Febria CM et al.Dissolved organic matter quality and bioavailability changes across an urbani-zation gradient in headwater streams.Environmental Science&Technology,2014,48(14):7817-7824.[5]Zhai TN,Huo SL,Zhang JT.Spectral characteristics of vertical distribution of dissolved organic matters in sediments.Chi-nese Journal of Environmental Engineering,2017,11(11):6196-6204.[翟天恩,霍守亮,张婧天.沉积物中溶解性有机质的垂向分布光谱特性.环境工程学报,2017,11(11):6196-6204.][6]Ziegelgruber KL,Chin YP,Zeng T et al.Sources and composition of sediment pore-water dissolved organic matter in prai-rie pothole lakes.Limnology and Oceanography,2013,58(3):1136-1146.[7]Li YP,Wang SR,Zhang L.Composition,source characteristic and indication of eutrophication of dissolved organic matter in the sediments of Erhai Lake.Environmental Earth Sciences,2015,74(5):3739-3751.[8]He XS,Xi BD,Wei ZM et al.Fluorescence excitation-emission matrix spectroscopy with regional integration analysis for characterizing composition and transformation of dissolved organic matter in landfill leachates.Journal of Hazardous Materi-als,2011,190:293-299.[9]He X,Xi B,Wei Z et al.Spectroscopic characterization of water extractable organic matter during composting of municipal solid waste.Chemosphere,2011,82(4):541-548.[10]Wu HY,Zhou ZY,Wang HT et al.Effect of spectra correction on the fluorescence characteristics of dissolved organic mat-ter.Spectroscopy and Spectral Analysis,2012,32(11):3044-3048.[吴华勇,周泽宇,王洪涛等.光谱校正对溶解有机物三维荧光光谱特征影响.光谱学与光谱分析,2012,32(11):3044-3048.][11]Chen W,Westerhoff P,Leenheer JA et al.Fluorescence excitation-emission matrix regional integration to quantify spectra for dissolved organic matter.Environmental Science Technology,2003,37(24):5701-5710.[12]Chen XH,Qian XY,Li XP et al.Long-term trend of eutrophication state of lake Erhai in1988-2013and analyses of its socio-economic drivers.J Lake Sci,2018,30(1):70-78.DOI:10.18307/2018.0107.[陈小华,钱晓雍,李小平等.洱海富营养化时间演变特征(1988-2013年)及社会经济驱动分析.湖泊科学,2018,30(1):70-78.][13]Wang SRed.Sediment-water interface process of lakes theories and methods.Beijing:Science Press,2014:108-111.[王圣瑞.湖泊沉积物—水界面过程基本理论与常用测定方法.北京:科学出版社,2014:108-111.][14]Wolfe AP,Kaushal SS,Fulton JRet al.Spectrofluorescence of sediment humic substances and historical changes of lacus-trine organic matter provenance in response to atmospheric nutrient enrichment.Environmental Science&Technology,2002,36:3217-3223.[15]Mcknight DM,Boyer EW,Westerhoff PK et al.Spectrofluorometric characterization of dissolved organic matter for indica-tion of precursor organic material and aromaticity.Limnology and Oceanography,2001,46(1):38-48.[16]Huguet A,Vacher L,Relexans S et al.Properties of fluorescent dissolved organic matter in the Gironde Estuary.Organic Geochemistry,2009,40:706-719.[17]Zhang ZK,WuRJ,Zhu YX et al.Lacustrine records of human activities in the catchment of Erhai Lake,Yunnan Prov-ince.Acta Geographica Sinica,2000,55(1):66-74.[张振克,吴瑞金,朱育新等.云南洱海流域人类活动的湖泊沉积记录分析.地理学报,2000,55(1):66-74.][18]Elizabeth JW,Elizabeth AC.Sources of sedimentary organic matter in the MississippiRiver and adjacent Gulf of Mexico as revealed by lipid biomarker andδ13C TOC analyses.Organic Geochemistry,2008,39:422-439.[19]Thurman EM.Organic geochemistry of natural waters.Boston,Dordrecht:Martinus Nijhoff/Dr Junk W Publishers,1985:497.[20]He MC,Shi YH,Lin CY.Characterization ofhumic acids extracted from the sediments of the various rivers and lakes in China.Journal of Environmental Sciences,2008,20:1294-1299.[21]Goldman JC,Caron DA,Dennett MR.Regulation of gross growth efficiency and ammonium regeneration in bacteria by substrate C:N ratio.Limnology and Oceanography,1987,32(6):1239-1252.516J.Lake Sci.(湖泊科学),2019,31(2)[22]Wang Q,Gao XQ,Xiao NW et al.Distribution pattern of submerged macrophytes and its influencing factors of water envi-ronment in Lake Dianchi.J Lake Sci,2018,30(1):157-170.DOI:10.18307/2018.0116.[王琦,高晓奇,肖能文等.滇池沉水植物的分布格局及其水环境影响因子识别.湖泊科学,2018,30(1):157-170.][23]Chen JY,Zhang EL.Sediment record of subfossil chironomid assemblages in the ecosystem evolution of Lake Chenghai.Ec-ological Science,2015,34(2):1-8.[陈静逸,张恩楼.云南程海湖泊生态系统演化的摇蚊亚化石沉积记录.生态科学,2015,34(2):1-8.][24]Zhao HC,Zhang L,Wang SRet al.Features and influencing factors of nitrogen and phosphorus diffusive fluxes at the sedi-ment-water interface of Erhai Lake.Environmental Science and PollutionResearch International,2018,25:1933-1942.[25]Wu H,Chang FQ,Zhang HC et al.Grain-size distribution patterns of the surface sediments and their influential factors in Lake Lugu.Acta Sedimentologica Sinica,2016,34(4):679-687.[吴汉,常凤琴,张虎才等.沪沽湖表层沉积物粒度空间分布特征及其影响因素.沉积学报,2016,34(4):679-687.][26]Li QC.The characteristic of dissolved organic nitrogen(DON)in lake sediments and their responses to drainagebasin[Dis-sertation].Guilin:Guilin University of Technology,2015:21-24.[李秋才.高原湖泊沉积物溶解性有机氮(DON)特征及其与流域间变化[学位论文].桂林:桂林理工大学,2015:21-24.]。
大汶河-东平湖CDOM的荧光特征及与营养物质的耦合关系
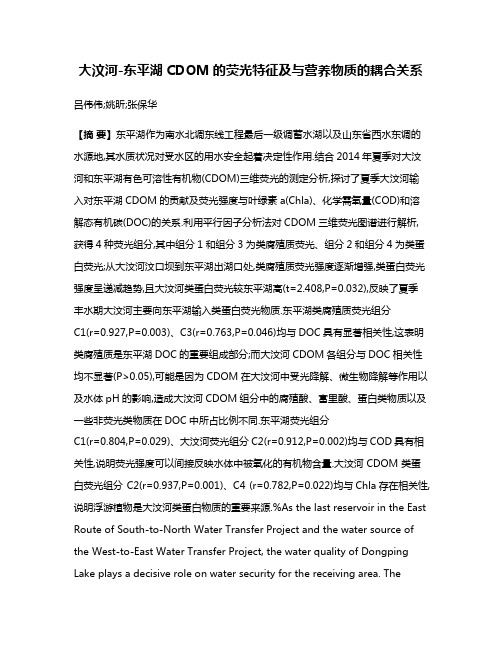
大汶河-东平湖CDOM的荧光特征及与营养物质的耦合关系吕伟伟;姚昕;张保华【摘要】东平湖作为南水北调东线工程最后一级调蓄水湖以及山东省西水东调的水源地,其水质状况对受水区的用水安全起着决定性作用.结合2014年夏季对大汶河和东平湖有色可溶性有机物(CDOM)三维荧光的测定分析,探讨了夏季大汶河输入对东平湖CDOM的贡献及荧光强度与叶绿素a(Chla)、化学需氧量(COD)和溶解态有机碳(DOC)的关系.利用平行因子分析法对CDOM三维荧光图谱进行解析,获得4种荧光组分,其中组分1和组分3为类腐殖质荧光、组分2和组分4为类蛋白荧光;从大汶河汶口坝到东平湖出湖口处,类腐殖质荧光强度逐渐增强,类蛋白荧光强度呈递减趋势,且大汶河类蛋白荧光较东平湖高(t=2.408,P=0.032),反映了夏季丰水期大汶河主要向东平湖输入类蛋白荧光物质.东平湖类腐殖质荧光组分C1(r=0.927,P=0.003)、C3(r=0.763,P=0.046)均与DOC具有显著相关性,这表明类腐殖质是东平湖DOC的重要组成部分;而大汶河CDOM各组分与DOC相关性均不显著(P>0.05),可能是因为CDOM在大汶河中受光降解、微生物降解等作用以及水体pH的影响,造成大汶河CDOM组分中的腐殖酸、富里酸、蛋白类物质以及一些非荧光类物质在DOC中所占比例不同.东平湖荧光组分C1(r=0.804,P=0.029)、大汶河荧光组分C2(r=0.912,P=0.002)均与COD具有相关性,说明荧光强度可以间接反映水体中被氧化的有机物含量.大汶河 CDOM 类蛋白荧光组分 C2(r=0.937,P=0.001)、C4 (r=0.782,P=0.022)均与Chla存在相关性,说明浮游植物是大汶河类蛋白物质的重要来源.%As the last reservoir in the East Route of South-to-North Water Transfer Project and the water source of the West-to-East Water Transfer Project, the water quality of Dongping Lake plays a decisive role on water security for the receiving area. Thethree-dimensional fluorescence of chromophoric dissolved organic matter (CDOM) in Dawen River and Dongping Lake were determined. Moreover, the CDOM influence of Dawen River on Dongping Lake and the correlations between fluorescence intensity and Chla, COD and DOC were studied. Four fluorescent components were obtained through Parallel Factor Analysis on the Three-dimensional Excitation-Emission Matrix Fluorescence Spectra, in which, C1 and C3 were humic-like fluorescence, and C2 and C4 were protein-like fluorescence. From Dawen River to Dongping Lake, the intensity of humic-like fluorescence increased and the intensity of protein-like fluorescence decreased. Furthermore, the intensity of protein-like fluorescence in Dawen River was higher than that in Dongping Lake (t=2.408, P=0.032), indicating that the protein-like fluorescence in Dongping Lake was mainly from Dawen River in summer. The humic-like components C1 (r=0.927, P=0.003) and C3 (r=0.763,P=0.046) of Dongping Lake were all significantly related with DOC, indicating that humic-like substances were the main components of DOC in Dongping Lake. However, the correlations between fluorescent components and DOC were not significant in Dawen River (P>0.05), probably because of the different proportions of humic acid, fulvic acid, protein-like matter of CDOM and some non-fluorescent substances in DOC caused by microbial degradation, light degradation and the effect of pH in the water. Significant correlations between C1 (r=0.804, P=0.029) of Dongping Lake, C2 (r=0.912, P=0.002) of Dawen River and COD were found, suggesting that CDOM could reflect the organic matter contentoxidated in the water. C2 (r=0.937, P=0.001) and C4 (r=0.782, P=0.022) of Dawen River were all significant related with Chla, indicating that phytoplankton was the main source of protein-like substances in Dawen River.【期刊名称】《生态环境学报》【年(卷),期】2018(027)003【总页数】8页(P565-572)【关键词】有色可溶性有机物;河流输入;三维荧光;平行因子分析法【作者】吕伟伟;姚昕;张保华【作者单位】聊城大学环境与规划学院,山东聊城 252000;聊城大学环境与规划学院,山东聊城 252000;中国科学院南京地理与湖泊研究所//湖泊与环境国家重点实验室,江苏南京 210008;聊城大学环境与规划学院,山东聊城 252000【正文语种】中文【中图分类】X12;X13有色可溶性有机物(Chromophoric Dissolved Organic Matter,CDOM)是水体中重要的有机碳库,占DOC的60%以上(段洪涛等,2009),在全球碳循环过程中起着重要的作用;并极大地影响光在水生环境中的传播等相关过程,对紫外辐射具有强烈的吸收作用,能够减少紫外线辐射对水生生态系统的影响(Williamson et al.,1996;Laurion et al.,2000)。
消落带夏冬季土壤溶解有机质的组成特征及来源

消落带夏冬季土壤溶解有机质的组成特征及来源郝蓉;徐召玉;沈祠福;伍玉鹏;宋艳暾;张金良【摘要】溶解性有机质(Dissolved organic matter,DOM)普遍存在于土壤、沉积物和水体中,在环境地球化学和全球碳循环中发挥重要作用.消落带独特的\"干湿交替\"使土壤有不同的季节特征,但有关不同季节土壤DOM的性质、组成及来源差异还未见报道.利用紫外-可见光谱、三维荧光光谱(Excitation-emission matrices,EEMs)与平行因子分析模型(Parallel factor analysis,PARAFAC)相结合的方法,分析了丹江口库区消落带表层土壤DOM在不同季节的组成、结构,并对其来源进行解析.结果表明,不同季节消落带土壤DOM的组成特征存在一定差异.冬季土壤的有色溶解性有机物(Chromophoric dissolved organic matter,CDOM)浓度、土壤DOM的芳香性、缩合度、腐殖化程度、所含疏水性组分都高于夏季土壤,但参与光漂白的活性要低于夏季土壤.荧光指数(FI)、腐殖化指数(HIX)和生物源指数(BIX)表明该区土壤DOM的来源在不同季节都有明显的自生源特征,且腐殖化程度和生物可利用性都不高,并未表现显著差异.该区土壤DOM存在两个主要的荧光峰:紫外光区类富里酸峰、可见光区类富里酸峰和类腐殖酸峰.研究提出消落带淹水期间产生的DOM对水环境有很大影响,以内源为主的库区在水质改善的过程中需加强对内源物质的管控.这些研究结果可为消落带的水体修复和污染物的迁移转化提供科学依据.【期刊名称】《生态环境学报》【年(卷),期】2019(028)006【总页数】7页(P1127-1133)【关键词】土壤溶解性有机质;季节变化;消落带;紫外-可见光谱;荧光光谱【作者】郝蓉;徐召玉;沈祠福;伍玉鹏;宋艳暾;张金良【作者单位】农业部产地环境污染防控重点实验室/天津市农业环境与农产品安全重点实验室,天津 300191;华中农业大学资源与环境学院/农业部长江中下游耕地保育重点实验室,湖北武汉 430070;华中农业大学资源与环境学院/农业部长江中下游耕地保育重点实验室,湖北武汉 430070;华中农业大学资源与环境学院/农业部长江中下游耕地保育重点实验室,湖北武汉 430070;华中农业大学资源与环境学院/农业部长江中下游耕地保育重点实验室,湖北武汉 430070;华中农业大学资源与环境学院/农业部长江中下游耕地保育重点实验室,湖北武汉 430070;天津市农业科学院,天津 300191【正文语种】中文【中图分类】X122溶解性有机质(DOM)广泛存在于陆地和水生生态系统中,是一类由腐殖酸、蛋白质、氨基酸及多糖等物质组成,化学结构复杂的有机物混合体。
环胶州湾河流入海口CDOM吸收光谱特征
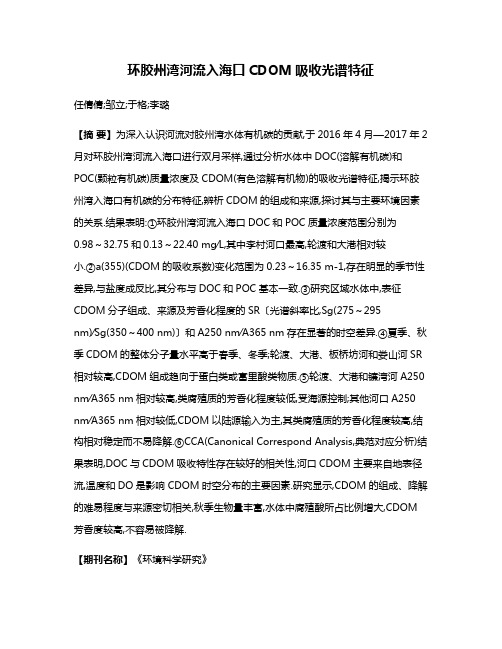
环胶州湾河流入海口CDOM吸收光谱特征任倩倩;邹立;于格;李璐【摘要】为深入认识河流对胶州湾水体有机碳的贡献,于2016年4月—2017年2月对环胶州湾河流入海口进行双月采样,通过分析水体中DOC(溶解有机碳)和POC(颗粒有机碳)质量浓度及CDOM(有色溶解有机物)的吸收光谱特征,揭示环胶州湾入海口有机碳的分布特征,辨析CDOM的组成和来源,探讨其与主要环境因素的关系.结果表明:①环胶州湾河流入海口DOC和POC质量浓度范围分别为0.98~32.75和0.13~22.40 mg∕L,其中李村河口最高,轮渡和大港相对较小.②a(355)(CDOM的吸收系数)变化范围为0.23~16.35 m-1,存在明显的季节性差异,与盐度成反比,其分布与DOC和POC基本一致.③研究区域水体中,表征CDOM分子组成、来源及芳香化程度的SR〔光谱斜率比,Sg(275~295nm)∕Sg(350~400 nm)〕和A250 nm∕A365 nm存在显著的时空差异.④夏季、秋季CDOM的整体分子量水平高于春季、冬季;轮渡、大港、板桥坊河和娄山河SR 相对较高,CDOM组成趋向于蛋白类或富里酸类物质.⑤轮渡、大港和镰湾河A250 nm∕A365 nm相对较高,类腐殖质的芳香化程度较低,受海源控制;其他河口A250 nm∕A365 nm相对较低,CDOM以陆源输入为主,其类腐殖质的芳香化程度较高,结构相对稳定而不易降解.⑥CCA(Canonical Correspond Analysis,典范对应分析)结果表明,DOC与CDOM吸收特性存在较好的相关性,河口CDOM主要来自地表径流,温度和DO是影响CDOM时空分布的主要因素.研究显示,CDOM的组成、降解的难易程度与来源密切相关,秋季生物量丰富,水体中腐殖酸所占比例增大,CDOM 芳香度较高,不容易被降解.【期刊名称】《环境科学研究》【年(卷),期】2018(031)008【总页数】10页(P1407-1416)【关键词】胶州湾;河流入海口;CDOM;吸收光谱特征;CCA【作者】任倩倩;邹立;于格;李璐【作者单位】中国海洋大学环境科学与工程学院,山东青岛 266100;中国海洋大学环境科学与工程学院,山东青岛 266100;中国海洋大学,海洋环境与生态教育部重点实验室,山东青岛 266100;中国海洋大学环境科学与工程学院,山东青岛 266100;中国海洋大学,海洋环境与生态教育部重点实验室,山东青岛 266100;中国海洋大学环境科学与工程学院,山东青岛 266100【正文语种】中文【中图分类】X522海洋中DOC (溶解有机碳)约占总有机碳的90%,CDOM(chromophoric dissolved organic matter, 有色溶解有机物)作为DOC循环的重要组成部分,约占海洋DOC的20%~70%[1]. CDOM是水体中一类含有腐殖酸、富里酸和芳烃聚合物等物质的可溶性有机物[2],具有光谱吸收性质[3],因其对黄色波段的光吸收最小而显黄色,又被称为“黄色物质”. CDOM特征光谱吸收不仅可以反演DOC含量[4-5],还可以示踪溶解有机物在海洋环境中的行为过程[6-8],另外,通过与重金属离子络合、吸附有机污染物,从而影响其他物质的存在形式和活性[6]. CDOM吸收紫外辐射而降低其对海洋生物的损害,在可见光范围的特征吸收与水体Chla含量相关[2,9],并且其光降解和化学降解过程使DOM分子量减小[10-11],为微生物提供食物供给,或者被浮游生物利用[4,10].水体CDOM特征吸收系数具有时空分布规律,一般说来夏季CDOM相对含量明显高于冬季[12]. 陆源径流输入是河口和近岸水域CDOM的主要来源[13-14],河口区域CDOM相对含量总体呈现由河到海逐渐降低的趋势[15]. 某些河口受流域影响较大,其特征光谱吸收与类腐殖质显著正相关,而与类蛋白之间无显著相关关系[16-17],进而表征区域有机污染程度和污染来源[9,18-19]. CDOM的迁移转化受多种因素影响,光降解作用引起CDOM的平均分子量减小[10],减小趋势随光照时间长而增加[11];底质沉积物再悬浮是近岸水体中CDOM重要来源,释放类腐殖质与类蛋白质荧光物质进入底层水体[20-21].胶州湾是位于黄海西岸的半封闭海湾,汇入胶州湾的河流有镰湾河、洋河和大沽河等10余条河流,属于区域季节性河流,汛期集中在7、8和9三个月,其中径流量较大的是大沽河和洋河,其流域功能以农业生产为主,而青岛市内的河流,如海泊河、李村河等受市区工业和生活行为的影响严重. 胶州湾东部海水中CDOM主要来自海泊河排放的废水,但由于较强的水动力和潮汐作用快速扩散,海泊河附近海区CDOM并没有明显的积累[22];大沽河水体中类腐殖质荧光强度较大,是胶州湾水体中类腐殖质荧光物质的重要来源[23]. 胶州湾水体CDOM主要由类腐殖质和类蛋白质两类物质组成,夏、秋季节的荧光强度明显高于冬、春季节[24],其中类腐殖质呈现由近岸海域向胶州湾中心逐渐减小,类蛋白质由胶州湾东北部的近岸海域向西南部逐渐减小[25].汇入胶州湾的河流有十几条,各河流的流域分布和季节变化,及其对胶州湾海域有机碳输入差异显著,其中任何一条河流对胶州湾的物质输送都不足以起到控制作用. 为深入探讨胶州湾溶解有机物的来源、组成,及其潜在的生态学意义,该研究通过研究环胶州河流入海口水体中CDOM的吸收光谱性质,认识其分子组成特征,辨析影响CDOM分布和组成的因素,进而阐述胶州湾CDOM来源、迁移转化及其潜在的生态学效应.1 调查区域和研究方法1.1 研究区域和样品采集图1 环胶州湾主要河流入海口采样点Fig.1 Study area and sampling sites at the estuaries of Jiaozhou Bay研究区域和采样点布设如图1所示,采样点设置于镰湾河、洋河、大沽河、白沙河、娄山河、板桥坊河、青岛市区的李村河和海泊河8个河流入海口处,以及青岛大港和团岛轮渡两处人文活动地,共计10个,编号依次为R1~R10. 于2016年4月—2017年2月进行现场调查和样品采集,每双月取样1次. 由于河流入海口的水深较浅,通常小于5 m,取表层水样,避光冷藏,经GF/F膜(Whatman,450 ℃灼烧)过滤,滤液用于DOC和CDOM分析,滤膜用于POC(颗粒有机碳)分析. 现场以多参数水质仪(HQ40d,哈希,美国)同步监测温度、盐度、pH和DO.1.2 POC和DOC质量浓度的测定POC膜样解冻后滴加10% HCl溶液除去膜上的无机碳,干燥后以锡杯包裹,采用元素分析仪(PerkinElmer 2400 series-Ⅱ,UCDavis,美国)进行测定,测量偏差小于0.3%. DOC水样解冻至室温,以总有机碳分析仪(TOC-L,日本岛津)高温催化氧化法测试DOC质量浓度[26],低碳水和标准海水(美国迈阿密大学Hansell实验室提供)作为仪器的空白和标准曲线,每个样品进样3~5次,分析误差小于3%. 图2 环胶州湾河流入海口水体中DOC和POC质量浓度变化Fig.2 Contents of DOC and POC at the estuaries of Jiaozhou Bay1.3 CDOM吸收光谱分析CDOM水样解冻至室温,装入1 cm石英比色皿,采用紫外-可见分光光度计(UV-2550,日本岛津)于190~800 nm波长下测定其吸光度,以Milli-Q水(电阻率为18.2 MΩ·cm)作为参照水样,采样间隔为1 nm. 每个波长下吸光度减去700 nm处的吸光度,以扣除由海水与参比Milli-Q水之间的折射率差异以及水样中细小颗粒物散射引起的基线漂移[27],从而校正其吸光度. 根据式(1)计算CDOM的吸收系数[4]:a(λ)=2.303×A(λ)/L(1)式中:a(λ)为波长λ处CDOM的光吸收系数,m-1;A(λ)为λ处校正后的吸光度;λ为波长,nm;L为比色皿长度,m.1.4 CDOM光谱斜率计算CDOM吸收特性主要表现为吸收系数随波长增加近似地呈指数衰减,根据式(2)对光谱斜率进行拟合[28-29]:a(λ)=a(λ0)×e[Sg×(λ0-λ)]+K(2)式中:Sg为吸收光谱曲线的光谱斜率,nm-1,它可以反映光吸收指数随波长增加的递减程度;λ0为参比波长,不同波段下Sg的拟合通常选用不同的参比波长,对275~295和 350~400 nm波段下的Sg进行拟合,其参比波长选用285和375 nm;K为背景参数,用来规避由非有机物引起的基线抬高或降低的现象.2 结果与讨论2.1 DOC和POC质量浓度变化环胶州湾河流入海口水体中DOC和POC质量浓度变化如图2所示. 从图2可以看出,DOC和POC质量浓度范围分别为0.98~32.75和0.13~22.40 mg/L,其平均值分别为5.04和1.73 mg/L. 4月轮渡(R1)和李村河(R4)、6月李村河(R4)和娄山河(R6)以及12月李村河(R4)和洋河(R9)水体中 DOC质量浓度较高,明显高于同期其他站位,且随时间变化明显,最高DOC质量浓度位于李村河(R4). 李村河(R4) POC质量浓度整体较高,其变化规律与DOC相似,随时间变化显著,POC最高质量浓度位于2016年4月,6月和12月次之,10月最低;洋河(R9)水体中POC质量浓度随时间变化呈上升趋势(10月除外),2017年2月最高;其余采样点POC质量浓度整体较低,且随时间变化较小. 人文活动地轮渡(R1)和大港(R2)水体中的DOC和POC较为接近,DOC和POC质量浓度变化范围分别为0.98~9.57和0.13~1.19 mg/L,其平均值分别为2.43和0.51 mg/L,与前人研究结果[30-31]相近. 海泊河(R3)水体中DOC质量浓度在2.33~5.19 mg/L之间,与文献报道结果[22]一致.总体来看,李村河(R4)水体中DOC和POC质量浓度均高于其他采样点,DOC约是POC的1~13倍,DOC是有机碳(OC)的主要贡献者,其中李村河(R4)水体DOC贡献率较低,仅占74%左右. 同时,环胶州湾河流入海口水体中DOC和POC质量浓度呈极显著相关(见图3,R=0.830 3, n=60, P<0.01),说明环胶州湾河流向海域输送的DOC和POC具有一致性. 从图3可以看出,2016年8月和10月DOC和POC质量浓度整体较低,与降雨量有关,因蓄水量增多而被稀释,DOC和POC质量浓度降低.2.2 CDOM的相对含量吸收系数常被用来量化有机物光吸收部分的相对含量[32-33],其吸收特性与荧光特性存在一定的线性关系[2,17];河口地区通常以a(355)(波长在355 nm处的吸收系数)表征CDOM的相对含量[27,34-35]. 环胶州湾河流入海口水体中a(355)变化情况如图4图3 环胶州湾河流入海口水体中DOC和 POC质量浓度的相关性分析Fig.3 Relationship between DOC and POC at the estuaries of Jiaozhou Bay所示. 从图4可以看出,a(355)变化范围为0.23~16.35 m-1,平均值为3.09 m-1,最高值位于李村河(R4),其中2016年4月、6月和12月高于其他月份,其a(355)分别为13.13、11.29和16.35 m-1;轮渡(R1)和大港(R2)位于胶州湾内,其a(355)均较低,其范围均在0.23~3.46 m-1之间. 结果表明李村河对胶州湾CDOM单位输送量最高,与其DOC和POC的监测结果基本一致.图4 环胶州湾河流入海口CDOM的吸收系数a(355)Fig.4 a(355) of CDOM at the estuaries of Jiaozhou Bay在整个调研时间里,2016年12月各采样点a(355)明显高于其他月份(R6除外),其浮动范围在3.45~16.35 m-1;2016年8月和10月各采样点a(355)较低,仅为2016年12月的1/5左右,且各采样点间a(355)相差较小,这可能与区域降水的稀释作用有关. 据青岛市水利局公告,青岛市2016年12月降水量最少,月平均降雨量仅为28.9 mm,而8月和10月月平均降水量分别为120.1和75.0 mm,河流蓄水量较高,对河流有机物质量浓度起到稀释作用. 相近水域a(355)的范围见表1. 该研究涉及8条小型河流和两处人文活动区,CDOM相对含量变化较大,整体水平与辽河口a(355)相当[22];其中海泊河口水体a(355)变化范围0.46~5.53 m-1,与前人的研究结果[15]基本一致,虽然海泊河入海口附近工厂废水大量排入,且受人为活动影响较大,海泊河污染相对严重,但其CDOM相对含量并不高,可能由于水动力和潮汐作用较强而快速扩散,CDOM相对含量迅速降低,导致海泊河河口(R3)无明显积累[22]. a(355)与盐度呈反比(R=-0.749 3, n=60, P<0.01),盐度低时a(355)较高,表明河口区CDOM相对含量总体呈现由河到海逐渐降低的趋势,河流输入是胶州湾CDOM的主要来源之一.表1 不同水域CDOM的a(355)变化范围Table 1 a(355) of CDOM in different estuaries水域a(355)∕m-1范围平均值数据来源长江口0.01~6.22—文献[36]海泊河附近海域1.81~3.512.39文献[22]海泊河入海口0.59~5.092.91文献[22]辽河口1.54~14.21—文献[15]太湖3.27~8.27—文献[37]海泊河河口0.46~5.532.69该研究胶州湾各河口0.23~16.353.09该研究CDOM是溶解有机物中的有色部分,DOC中有色部分的比例随地区和来源的不同而存在很大差异,当DOC中有色的部分的比例为常数或变化较小时,CDOM吸收系数与DOC质量浓度存在较好的相关性[3,8]. 研究区域a(355)与DOC质量浓度存在极显著的正相关(见图5,R=0.847 8, n=60, P<0.01),说明研究区域CDOM与DOC的产生和变化过程相关或相伴发生[2],即研究区域内CDOM相对含量分布在一定程度上可以反映DOC质量浓度分布,反之亦然. 该研究a(355)/DOC为0.05~1.87 L/(m·mg),平均值为0.63 L/(m·mg),长江口水体a(355)/DOC为0.06~1.42 L/(m·mg) [8],其比值略低于环胶州湾河流入海口;该研究海泊河口a(355)/DOC为0.80 L/(m·mg),已报道结果仅为0.24 L/(m·mg) [22]. 可见该研究样品中单位DOC中含有的CDOM较高,且二者呈显著相关,表明该研究来自河流输送的陆源DOC中含有较为丰富的CDOM. 如图5所示,2016年4月和12月李村河以及12月洋河水体中CDOM的a(355)与DOC的关系与其他结果相差较大,可能由于浮游植物自身降解或者光化学降解作用,使得该区域水体中小分子无色 DOC所占比例较大[2].图5 环胶州湾河流入海口水体中 DOC与a(355)的关系Fig.5 Relationship between DOC and a(355) at the estuaries of Jiaozhou Bay2.3 CDOM的分子组成特征Sg(275~295 nm)或SR〔光谱斜率比,Sg(275~295)/Sg(350~400 nm)〕能够反应CDOM的相对分子量大小,河口区SR的大小与CDOM分子量呈负相关,SR越大对应的平均分子量越小[16,38-39]. 研究水体中SR的范围为0.02~3.31,平均值为1.41(见图6). 整体来说,SR较高,说明环胶州湾河流入海口水体中CDOM分子量较小,其组成趋向于小分子的蛋白类物质或者富里酸类物质[39-40],其中又以轮渡(R1)、大港(R2)、板桥坊河(R5)和娄山河(R6)水体中SR相对较高,且随时间变化明显. 轮渡(R1)和大港(R2)以海水环境为主,其水体盐度相对较高,表现为显著的海源特征,CDOM以现场生物生产为主,同时高盐度海域更易发生光降解作用,使得该区域水体中分子量水平下降,综合表现为SR增高,因此轮渡(R1)和大港(R2)水体中蛋白类物质或富里酸类物质相对丰富,并且季节变化显著.与其他环胶州湾入海河流相比,板桥坊河和娄山河长度短,流域面积小,受邻近海域的影响显著,而呈现显著的现场生产有机物特征.图6 环胶州湾河流入海口CDOM的SR变化Fig.6 SR of CDOM at the estuaries of Jiaozhou Bay从随时间变化来看,研究区域中2016年6月、9月和10月SR较小,CDOM平均分子量较高,2月SR较大,CDOM平均分子量较低,4月和12月SR居中并且结果相近. SR结果说明,研究水域夏、秋季CDOM的整体平均分子量水平高于春、冬季,表现出一定程度的季节变化特征,这一结论与天目湖流域CDOM研究结果[41]一致;结合水体中荧光组分相对贡献的变化,说明环胶州湾河口CDOM的组成、来源和转化规律等都存在一定的季节性差异. 夏、秋季分子量较高,与夏、秋季气温高、河口生物种类或数量增多,尤其是浮游植物的数量增加有关[42]. CDOM的a(355)与SR之间的关系如图7所示. 从图7可以看出,约80%的SR介于0.8~1.8之间,CDOM相对含量较高时,SR相对集中,平均值约为1.5,说明环胶州湾河口总体CDOM分子组成相似,其来源相近. 该研究结果与长江口、九龙江口、天目湖流域及世界其他其他河口区研究结果基本一致(见表2).图7 不同采样点CDOM的a(355)与 SR之间的散点分布图Fig.7 Scatter diagram between a(355) and SR in different sites表2 不同河口区SRTable 2 SR of CDOM in different estuaries地区SR范围平均值数据来源Congo River0.81~1.37—文献[43]Delaware Estuary0.70~2.50—文献[39]长江口1.10~2.201.6文献[44]九龙江口0.88~1.40—文献[16]天目湖流域0.37~1.610.92±0.12文献[41]环胶州湾各河口0.02~3.311.41该研究2.4 人文活动对CDOM的影响汇入胶州湾河流中较大的海泊河、李村河、板桥坊河、大沽河和镰湾河,均受到不同程度的农业、工业和生活等人文活动影响;流域人文活动状况可以表征为CODMn和N、P营养盐质量浓度变化. 以DIN (溶解无机氮)、DIP (活性磷酸盐)和CODMn质量浓度与相对应河口区a(355)进行相关性分析,以其相关性强弱表征CDOM受人文活动影响程度高低. DIN、DIP和CODMn质量浓度与a(355)均呈显著性相关(见图8),a(355)与DIN、DIP和CODMn质量浓度相关性系数分别为 0.779 9、0.7420和 0.636 9(n=60, P<0.01),说明环胶州湾入海口CDOM的相对含量与流域人类活动密切相关,即人类活动加剧,是导致河口CDOM相对含量增加的重要因素.图8 环胶州湾河流入海口a(355)与DIN、 DIP和CODMn质量浓度的关系Fig.8 Relationships between a(355) of CDOM and DIN, DIP and CODMn at the estuaries of Jiaozhou Bay2.5 CDOM的芳香化程度图9 环胶州湾河流入海口A250 nm/A365 nmFig.9 A250 nm/A365 nm ratio at the estuaries of Jiaozhou BayA250 nm/A365 nm(水样在波长250和365 nm处吸光度的比值)与有机物的芳香性和腐殖质分子量的大小呈反比关系[45-47]. 环胶州湾河流入海口水体中CDOM的A250 nm/A365 nm分布情况如图9所示. 从图9可以看出,较高的A250 nm/A365 nm出现在2016年4月、8月和10月的轮渡(R1)、大港(R2)、海泊河(R3)和镰湾河(R10),且随时间变化明显,而其他河口A250 nm/A365 nm 无显著变化. 与不同区域CDOM的A250 nm/A365 nm相比(见表3),该研究河口环境差异较大,所以A250 nm/A365 nm变化范围较大. 轮渡(R1)、大港(R2)和镰湾河(R10)是码头所在地,水域CDOM组成受海源控制,CDOM组分中腐殖酸占比较少,类腐殖质芳香化程度低[40,49]. 其他河口A250 nm/A365 nm相对较小,尤其是李村河(R4),表明陆源输入的类腐殖酸所占比例较高[48],河口CDOM芳香度相对较高,其结构相对稳定,不易被降解[49].表3 不同区域CDOM的A250 nmA365 nmTable 3 A250 nmA365 nm ratiosof CDOM in different areas区域A250 nm∕A365 nm数据来源Lakes Savojarvi and Mekkojarvi(Finland)4.16~5.72文献[45]Lake Ria deAveiro(Portugal)5.17~8.26文献[46]Suwannee River(America)2.62~5.86文献[49]太湖5.16~8.17文献[12]辽河口4.76~8.95文献[15]胶州湾各河口3.67~27.00该研究2.6 理化因素对CDOM吸收特性的影响环胶州湾河流入海口环境复杂,诸多理化因素对CDOM的吸收特性共同影响,结合13项因子(温度、pH、DO、盐度、Chla、DOC、CODMn、POC、SS、NO2-、NO3-、NH4+、PO43-)数据应用CCA(Canonical Correspond Analysis,典范对应分析)对CDOM吸收特性相关参数〔a(355)、SR和A250 nm/A365 nm〕进行多元线性回归分析. 分析结果显示,CDOM吸收特性与环境因子的前两个排序轴的相关性系数较高,分别为0.89和0.54;对于CDOM吸收特性来说,第一、第二排序轴的相关性系数只有0.17,几乎相当于垂直;对于环境因子来说,第一、第二排序轴的相关性系数为0;结合第一、第二轴的贡献率来看,前两个排序轴的贡献率为72%. 以上结果表明CCA分析的排序结果是可信的,能够较好地反映环境因子对CDOM的吸收特性的影响.图10 环胶州湾河流入海口水体中CDOM 吸收特性与理化因素的典范对应分析Fig.10 CCA analysis on CDOM absorption and environmental factors at the estuaries of Jiaozhou Bay由图10可知,13个因子中,DOC质量浓度与CDOM吸收特性显著相关,pH与CDOM吸收特性相关性较弱. 环境因子与CDOM吸收特性的第一排序轴相关性大小依次为DOC>CODMn>NH4+>PO43->POC>SS>NO3->Chla>NO2-,均呈正相关关系;4种环境因子与CDOM吸收特性的第一排序轴呈负相关,其相关性大小依次为盐度>温度>DO>pH. 环境因子与CDOM吸收特性的第一排序轴的相关性系数远远高于第二排序轴,表明第一排序轴可以更好地解释CDOM吸收特性与环境因子之间的关系,且第一排序轴的贡献率高达68%. 整体说来,研究区域CDOM主要来自陆源输入和人文活动的产物,以及现场生物生产活动. 2016年6月、8月和10月CDOM吸收特性主要分布在第三象限,温度是其主要影响因素;2016年4月和2017年2月CDOM吸收特性大多聚集在第二象限,与盐度和DO关系密切;2016年12月CDOM吸收特性分布相对分散,可能受到多种理化因素的综合影响. 综上,温度和DO是影响CDOM时空分布的主要因素.3 结论a) DOC和POC质量浓度范围分别为0.98~32.75(平均值为5.04 mgL)和0.13~22.40 mgL(平均值为1.73 mgL),时空变化较大,2016年8月和10月质量浓度最低,轮渡(R1)和大港(R2)质量浓度较小.b) CDOM的a(355)变化范围为0.23~16.35 m-1(平均值为3.09 m-1),变化规律与DOC和POC相似,并与盐度呈负相关. a(355)DOC范围为0.05~1.87L(m·mg)〔平均值为0.63 L(m·mg)〕,与其他水域相比,单位DOC中含有更多的CDOM.c) SR变化范围为0.02~3.31(平均值为1.41),且相对集中,说明环胶州湾河口CDOM分子组成较为相似,来源相近;其中轮渡(R1)、大港(R2)、板桥坊河(R5)和娄山河(R6)水体中SR相对较高,其组成趋向于蛋白类或者富里酸类物质,主要与生物降解或者光降解有关;夏、秋季CDOM的整体分子量水平高于春、冬季,表现出一定程度的季节变化特征.d) 轮渡(R1)、大港(2)和镰湾(R10)河口A250 nmA365 nm较大,其为码头所在地,CDOM组成主要受海源控制,腐殖酸占比较少,类腐殖质芳香化程度低;其他河口A250 nmA365 nm相对较小,CDOM以陆源输入为主,大分子腐殖质质量分数相对较高,芳香度增加,结构相对稳定.e) CCA表明,CDOM吸收特性与DOC质量浓度关系密切,温度和DO质量浓度是影响CDOM时空分布的主要因素.参考文献(References):【相关文献】[1] COBLE P G.Marine optical biogeochemistry:the chemistry of ocean color[J].Chemical reviews,2007,107(2):402-418.[2] ROCHELLE-NEWALL E J,FISHER T R.Chromophoric dissolved organic matter and dissolved organic carbon in Chesapeake Bay[J].Marine Chemistry,2002,77(1):23-41. [3] FERRARI G M,DOWELL M D,GROSSI S,et al.Relationship between the optical properties of chromophoric dissolved organic matter and total concentration of dissolved organic carbon in the southern Baltic Sea Region[J].Marine Chemistry,1996,55(3/4):299-316. [4] KIRK J T O.Light and photosynthesis in aquatic ecosystems[M].England:Cambridge University Press,1994.[5] FERRARI G M.The relationship between chromophoric dissolved organic matter and dissolved organic carbon in the European Atlantic Coastal Area and in the West Mediterranean Sea (Gulf of Lions)[J].Marine Chemistry,2000,70(4):339-357.[6] CARDER K L,STEWARD R G,HARVEY G R,et al.Marine humic and fulvic acids:their effects on remote sensing of ocean chlorophyll[J].Limnology andoceanography,1989,34(1):68-81.[7] DE SOUZA SIERRA M M,DONARD O F X,LAMOTTE M.Spectral identification and behaviour of dissolved organic fluorescent material during estuarine mixing processes[J].Marine Chemistry,1997,58(1/2):51-58.[8] 陈欣,张霄宇,雷惠.长江口CDOM的光谱吸收特征以及DOC物源示踪意义[J].海洋环境科学,2012(5):625-630.CHEN Xin,ZHANG Xiaoyu,LEI Hui.Optical absorption properties of CDOM and tracingimplication of DOC in the Changjiang Estuary[J].Marine EnvironmentalScience,2012(5):625-630.[9] COBLE P G,DEL CASTILLO C E,AVRIL B,et al.Distribution and optical properties of CDOM in the Arabian Sea during the 1995 southwest Monsoon[J].Deep Sea Research Part II:Topical Studies in Oceanography,1998,45(1011):2195-2223.[10] KIEBER R J,ZHOU X,MOPPER K.Formation of carbonyl compounds from UV-induced photodegradation of humic substances in natural waters:fate of riverine carbon in the sea[J].Limnology and Oceanography,1990,35(7):1503-1515.[11] 王福利,郭卫东.秋季南海珠江口和北部湾溶解有机物的光降解[J].环境科学学报,2010,30(3):606-613.WANG Fuli,GUO Weidong.Photodegradation of DOM in the Pearl River Estuary and the Beibu Gulf of the South China sea in autumn[J].Acta ScientiaeCircumstantiae,2010,30(3):606-613.[12] 张运林,秦伯强.梅梁湾.大太湖夏季和冬季 CDOM 特征及可能来源分析[J].水科学进展,2007,18(3):415-423.ZHANG Yunlin,QIN Boqiang.Feature of CDOM and its possible source in Meiliang Bay and Da Taihu Lake in Taihu Lake in summer and winter[J].Advances in WaterScience,2007,18(3):415-423.[13] NELSON N B,SIEGEL D A,MICHAELS A F.Seasonal dynamics of colored dissolved material in the Sargasso Sea[J].Deep Sea Research Part Ⅰ:Oceanographic Research Papers,1998,45(6):931-957.[14] DEL CASTILLO C E,COBLEA P G,MORELL J M,et al.Analysis of the optical properties of the Orinoco River plume by absorption and fluorescence spectroscopy[J].Marine Chemistry,1999,66(1/2):35-51.[15] 杨阳,邹立,简慧敏.辽河口秋季有色溶解有机物的光谱特征研究[J].海洋环境科学,2017,36(1):94-100.YANG Yang,ZOU Li,JIAN Huimin.Spectral characteristics of chromophoric dissolved organic matter in Liaohe River estuary in autumn[J].Marine EnvironmentalScience,2017,36(1):94-100.[16] 郭卫东,黄建平,洪华生,等.河口区溶解有机物三维荧光光谱的平行因子分析及其示踪特性[J].环境科学,2010,31(6):1419-1427.GUO Weidong,HUANG Jianping,HONG Huasheng,et al.Resolving excitation emission matrix spectroscopy of estuarine CDOM with parallel factor analysis and its application in organic pollution monitoring[J].Environmental Science,2010,31(6):1419-1427.[17] VIGNUDELLI S,SANTINELLI C,MURRU E,et al.Distributions of dissolved organic carbon (DOC) and chromophoric dissolved organic matter (CDOM) in coastal waters of the northern Tyrrhenian Sea (Italy)[J].Estuarine,Coastal and Shelf Science,2004,60(1):133-149.[18] COBLE P G.Characterization of marine and terrestrial DOM in seawater using excitation-emission matrix spectroscopy[J].Marine Chemistry,1996,51(4):325-346. [19] 郭卫东,程远月,吴芳.海洋荧光溶解有机物研究进展[J].海洋通报,2007,26(6):98-106.GUO Weidong,CHENG Yuanyue,WU Fang.An overview of marine fluorescent dissolved organic matter[J].Journal of Marine Science Bulletin,2007,26(6):98-106.[20] 程远月,郭卫东,胡明辉.近岸沉积物再悬浮期间所释放溶解有机物的荧光特征[J].地球化学,2008,37(1):51-58.CHEN Yuanyue,GUO Weidong,HU Minghui.Fluorescence characteristics of dissolved organic matter released from estuarine sediments duringresuspension[J].Geochimica,2008,37(1):51-58.[21] BURDIGE D J,KLINE S W,CHEN W.Fluorescent dissolved organic matter in marine sediment pore waters[J].Marine Chemistry,2004,89(1):289-311.[22] 蒋凤华,杨黄浩,黎先春,等,胶州湾海水溶解有机物三维荧光特征研究[J].光谱学与光谱分析,2007,27(9):1765-1769.JIANG Fenghua,YANG Huanghao,LI Xianchun,et al.Excitation-emission-matrix spectral property of dissolved organic matter in seawater of Jiaozhou Bay,China[J].Spectroscopy and Spectral Analysis,2007,27(9):1765-1769.[23] 季乃云,赵卫红,王江涛,等.大沽河-胶州湾段溶解有机物类腐殖质荧光特征变化[J].环境科学,2006,27(6):1073-1077.JI Naiyun,ZHAO Weihong,WANG Jiangtao,et al.Change of humic-like fluorescence characteristics of dissolved organic matter from Dagu River to JiaozhouBay[J].Environmental Science,2006,27(6):1073-1077.[24] 鲁景亮.胶州湾溶解有机物荧光特征解析及水母暴发的潜在影响研究[D].青岛:中国科学院海洋研究所,2013.[25] 刘瑛,周倩倩,王江涛.秋季胶州湾有色溶解有机物荧光特性研究及其来源分析[J].中国海洋大学学报:自然科学版,2014 (6):60-66.LIU Ying,ZHOU Qianqian,WANG Jiangtao.Source and fluorescence characteristics of chromophoric dissolved organic matter in Jiaozhou Bay in autumn[J].Periodical of Ocean University of China,2014(6):60-66.[26] SHARP J H,CARLSON C A,PELTZER E T,et al.Final dissolved organic carbon broad community intercalibration and preliminary use of DOC reference materials[J].Marine Chemistry,2002,77(4):239-253.[27] GREEN S A,BLOUGH N V.Optical absorption and fluorescence properties of chromophoric dissolved organic matter in natural waters[J].Limnology and Oceanography,1994,39(8):1903-1916.[28] BROWN M.Transmission spectroscopy examinations of natural waters:C.Ultraviolet spectral characteristics of the transition from terrestrial humus to marine yellowsubstance[J].Estuarine and Coastal Marine Science,1977,5(3):309-317.[29] STEDMON C A,MARKAGER S,KAAS H.Optical properties and signatures of chromophoric dissolved organic matter (CDOM) in Danish coastalwaters[J].Estuarine,Coastal and Shelf Science,2000,51(2):267-278.[30] 李宁.长江口与胶州湾海水有机碳的分布,来源及与氮,磷的耦合关系[D].青岛:中国科学院海洋研究所,2006.[31] 梁成菊.青岛近海有机碳的分布特征及影响因素[D].青岛:中国海洋大学,2008.[32] PEACOCK M,BURDEN A,COOPER M,et al.Quantifying dissolved organic carbon concentrations in upland catchments using phenolic proxy measurements[J].Journal of Hydrology,2013,477(1):251-260.[33] OSBURN C L,BOYD T J,MONTGOMERY M T,et al.Optical proxies for terrestrial dissolved organic matter in estuaries and coastal waters[J].Frontiers in Marine Science,2016,2(127):1-15.[34] GUÉGUEN C,GUO L,TA NAKA N.Distributions and characteristics of colored dissolved organic matter in the western Arctic Ocean[J].Continental ShelfResearch,2005,25(10):1195-1207.[35] HOGE F E,VODACEK A,BLOUGH N V.Inherent optical properties of the ocean:retrieval of the absorption coefficient of chromophoric dissolved organic matter from fluorescence measurements[J].Limnology and Oceanography,1993,38(7):1394-1402.[36] GUO W,YANG L,ZHAI W,et al.Runoff-mediated seasonal oscillation in the dynamics of dissolved organic matter in different branches of a large bifurcated estuary:the Changjiang Estuary [J].Journal of Geophysical Research:Biogeosciences,2014,119(5):776-793.[37] 江俊武,李帅东,沈胤胤,等.夏季太湖CDOM光学特性空间差异及其来源解析[J].环境科学研究,2017,30(7):1020-1030.JIANG Junwu,LI Shuaidong,SHEN Yinyin,et al.Spatial differences of optical properties of CDOM and their source apportionment in Taihu Lake in summer[J].Research of Environmental Sciences,2017,30(7):1020-1030.[38] DEL VECCHIO R,BLOUGH N V.Spatial and seasonal distribution of chromophoric dissolved organic matter and dissolved organic carbon in the Middle AtlanticBight[J].Marine Chemistry,2004,89(1):169-187.[39] HELMS J R,STUBBINS A,RITCHIE J D,et al.Absorption spectral slopes and slope ratios as indicators of molecular weight,source,and photobleaching of chromophoric dissolved organic matter[J].Limnology and Oceanography,2008,53(3):955-969.[40] 李会杰.腐殖酸和富里酸的提取与表征研究[D].武汉:华中科技大学,2012.[41] 牛城,张运林,朱广伟,等.天目湖流域DOM和CDOM光学特性的对比[J].环境科学研究,2014,27(9):998-1007.。
长江口有色溶解有机物光谱特性及其示踪溶解有机碳研究
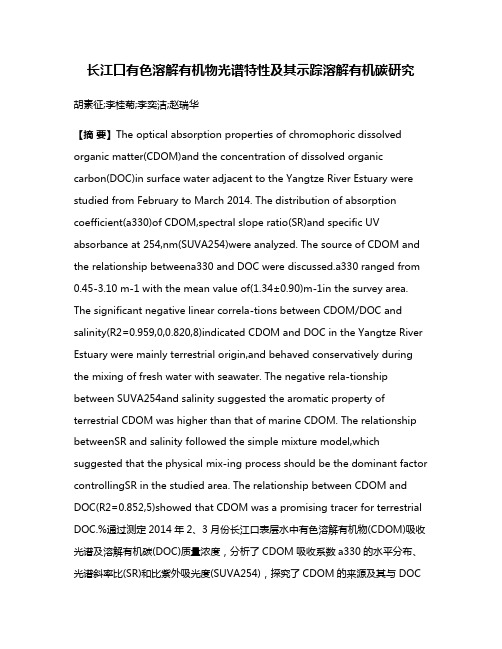
长江口有色溶解有机物光谱特性及其示踪溶解有机碳研究胡素征;李桂菊;李奕洁;赵瑞华【摘要】The optical absorption properties of chromophoric dissolved organic matter(CDOM)and the concentration of dissolved organic carbon(DOC)in surface water adjacent to the Yangtze River Estuary were studied from February to March 2014. The distribution of absorption coefficient(a330)of CDOM,spectral slope ratio(SR)and specific UV absorbance at 254,nm(SUVA254)were analyzed. The source of CDOM and the relationship betweena330 and DOC were discussed.a330 ranged from 0.45-3.10 m-1 with the mean value of(1.34±0.90)m-1in the survey area. The significant negative linear correla-tions between CDOM/DOC and salinity(R2=0.959,0,0.820,8)indicated CDOM and DOC in the Yangtze River Estuary were mainly terrestrial origin,and behaved conservatively during the mixing of fresh water with seawater. The negative rela-tionship between SUVA254and salinity suggested the aromatic property of terrestrial CDOM was higher than that of marine CDOM. The relationship betweenSR and salinity followed the simple mixture model,which suggested that the physical mix-ing process should be the dominant factor controllingSR in the studied area. The relationship between CDOM and DOC(R2=0.852,5)showed that CDOM was a promising tracer for terrestrial DOC.%通过测定2014年2、3月份长江口表层水中有色溶解有机物(CDOM)吸收光谱及溶解有机碳(DOC)质量浓度,分析了CDOM吸收系数a330的水平分布、光谱斜率比(SR)和比紫外吸光度(SUVA254),探究了CDOM的来源及其与 DOC的关系.研究表明:研究区域 a330范围为0.45~3.10,m-1,平均值为(1.34±0.90)m-1.CDOM 吸收光谱和 DOC质量浓度与盐度均呈负相关性(R2=0.959,0,0.820,8),表明二者的来源都是以陆源为主,且二者的河口混合行均表现为保守混合.由 SUVA254随盐度增大而减小得知,陆源 CDOM 相对于海源CDOM 的芳香性较强.SR与盐度关系符合保守混合模型,说明研究区域的 SR 主要受物理作用影响.a330与 DOC 质量浓度呈正相关性(R2=0.852,5),表明CDOM可以作为DOC的示踪物质.【期刊名称】《天津科技大学学报》【年(卷),期】2015(000)003【总页数】5页(P57-61)【关键词】长江口;有色溶解有机物;溶解有机碳;光谱特性;示踪物质【作者】胡素征;李桂菊;李奕洁;赵瑞华【作者单位】天津科技大学海洋科学与工程学院,天津 300457;天津科技大学海洋科学与工程学院,天津 300457;天津科技大学海洋科学与工程学院,天津300457;天津科技大学海洋科学与工程学院,天津 300457【正文语种】中文【中图分类】TQ050.4+21有色溶解有机物(chromophoric dissolved organic matter,CDOM)也称为黄色物质,是溶解有机物(dissolved organic matter,DOM)的重要组分[1–2].CDOM、浮游植物和非藻类的颗粒物质共同构成影响海洋水色的3 大重要组成成分,是海洋初级生产力光学遥感估算的重要参数[3].溶解有机碳(dissolved organic carbon,DOC)的海洋碳循环在整个海洋生态系统中起着重要的作用[4].研究水体中DOC 的质量浓度分布对分析了解某个区域的生物活动水平以及初级生产力有着重要意义. 长江是我国最长的河流,其平均入海径流量约9.28×1011,m3/a,占东海总径流量来源的84.4%,[5].长江口海区位于亚热带区域,属中纬度季风区,北面受苏北沿岸流的影响,东部有黑潮水与之交换,南面有台湾暖流上朔,西侧有长江、钱塘江等河流的输入,同时还受到上升流的影响,是最复杂的陆架海区之一[6].虽然许多学者[7–9]对不同水域的 CDOM 与DOC 进行了较多的研究,但是目前对长江口及邻近海域中CDOM 吸收系数与DOC 质量浓度的相关性研究及CDOM 光谱斜率比 SR和比紫外吸光度SUVA254(即a254/ρDOC)分布特征的研究较少.本研究通过测定CDOM 吸收光谱及DOC 质量浓度,分析了 CDOM 在表层海水中的水平分布及来源,SUVA254和SR的分布特征,并探究了CDOM 与DOC之间存在的关系,为河口区域遥感水质监测以及通过遥感水色估算DOC 质量浓度提供基础数据.1 材料与方法1.1 采样区域及样品预处理于2014 年2 月20 日—2014 年3 月10 日参加国家自然科学基金委员会“长江口调查共享航次”乘“润江1 号”科学考察船采取了32 个站位表层水样(图1).图1 采样站位Fig.1 Sampling stations样品采集后,立即用0.22,μm 的Millipore PES滤膜过滤,滤液转移至两个马弗炉焙烧(450,℃,4,h)过的100,mL 玻璃瓶中.其中一瓶用于CDOM 吸收光谱测定,另外一瓶加入1.2,mL 的2,mol/L HCl,用于DOC 质量浓度测定,所有样品冷藏保存带回陆地实验室进行测定分析.所用样品瓶在使用之前,均用MilliQ 水和海水样品充分润洗2~3 次,塑料瓶盖带有聚四氟乙烯衬垫,以尽量减小容器对样品的污染.1.2 研究方法CDOM 吸收光谱采用日本Shimadzu UV 2550型分光光度计测定,光谱测量范围为200~800 nm,每隔1 nm 读取1 次吸光度数据,石英比色皿光程为10 cm.以Milli-Q 水作为空白,对水样进行连续的扫描,得到各水样的光吸收曲线.根据式(1)计算CDOM 的吸收系数[10].式中:D(λ)为吸光度;r 为光程,m;a(λ)为波长λ处的吸收系数,m-1.为了去除仪器噪音、散射或样品与空白折射率差别所造成的影响,通常选用683~687 nm 吸收的平均值进行校正[11].CDOM 的光吸收特性表现为在紫外可见光区最高,到红外光谱区降为零,光谱吸收近似呈指数衰减的规律[12],可以用式(2)进行表示.式中:a(λ)为CDOM 的吸收系数,m-1;λ为波长,nm;λ0为参照波长,nm;S′为指数函数曲线斜率参数,nm-1,表示吸收系数随波长降低的程度.利用式(2),在275~295 nm(S′275-295)和350~400 nm(S′350-400)波段范围内,用Matlab 软件进行非线性拟合,得到光谱斜率参数.斜率比(SR)可以通过S′275-295和S′350-400的比值得到,用以表征CDOM 的来源[13].使用德国Elementar liqui Ⅱ 型总有机碳测定仪对DOC 进行测定,仪器每次进样3 mL,每个样品测定4 次取平均值,相对标准偏差小于2%,.测样前以Milli-Q 水作空白,用邻苯二甲酸氢钾标准液配制不同质量浓度标准溶液作为标准曲线,然后再进行测定.为验证仪器稳定性,测定10 个样品之后插入低碳水(Lot # 05-13,0.03 mg/L)和标准深海水(Lot #03-14,0.56 mg/L)标样,测定结果分别为(0.028±0.003)mg/L 和(0.54±0.01)mg/L.使用SBE-25 CTD 现场测得盐度剖面.2 结果与讨论2.1 长江口CDOM吸收系数a330的水平分布及来源研究区域 CDOM 的水平分布如图2 所示.a330范围0.45~3.10 m-1,平均值为(1.34±0.90)m-1,其大体趋势为近岸高远岸低.虽然河口C4、C5 站位处CDOM吸收系数a330受到最大浑浊带影响出现最大值3.10 m-1,但是整个研究区域的长江冲淡水锋面大体向南.冬季长江冲淡水向南流动与浙江沿岸水混合[5],研究区域的盐度水平分布(图3)更加清楚地看出长江冲淡水羽流向南的走向.图2 a330水平分布图Fig.2 Horizontal distribution of a330图3 盐度水平分布图Fig.3 Horizontal distribution of salinitya330与盐度(S)的关系如图4 所示.两者存在显著的相关性(R2=0.959,0,p<0.000,1,n=32),拟合方程为可以得出:CDOM 吸收系数a330从河端到海端逐渐降低,与盐度存在负相关性,说明物理混合作用是研究区域表层水CDOM 水平分布特性的主导因素.这与Bowers 等[14]研究发现盐度与CDOM 关系存在负相关性,李猛等[15]研究厦门湾九龙江河口区CDOM 吸收系数a355与盐度呈现显著负相关关系的结论一致.CDOM 与盐度较高的相关性表明CDOM的河口混合行为总体呈保守性.因此,陆源长江冲淡水为研究区域表层水中CDOM 的主要来源.SUVA254用于表征CDOM 中芳香性碳的含量[16].由图5 看出,随着盐度的升高CDOM 的芳香性呈逐渐降低趋势,说明陆源输入的CDOM 中含有较多的芳香性碳,远离河口受海水影响较大区域中CDOM 的芳香性碳较少.图4 a330与盐度的关系Fig.4 Relationship between a330and salinity图5 SUVA254与盐度关系Fig.5 Relationship between SUVA254and salinity2.2 SR与盐度和a330的关系光谱斜率比SR能够对CDOM 源进行区分,SR越高,海源CDOM 的成分越大[13].研究区域SR范围为1.00~2.18,随盐度增大呈指数增加的趋势(图6).在河水占主导控制作用时,SR主要表现为河流端元的性质,没有较大的变化;在海洋端元贡献较强的高盐度海区,SR有较大的增加.根据Stedmon 等[17]模拟方法拟合SR与盐度保守混合曲线.结果表明,研究区域SR与盐度的关系与保守混合曲线相吻合,说明在低盐区CDOM 以陆源为主,在高盐区CDOM 的源受陆源和海源共同控制.Xie 等[18]在研究北冰洋东南部的波弗特海域时发现指数函数可以很好地描述SR与CDOM 吸收系数的关系.研究区域表层水中SR与a330呈指数衰减关系(图7),相关系数R2 为0.834,7,两者关系为Helms 等[13]发现光漂白作用使得SR升高.由SR分别与盐度和a330具有较好的指数相关性可以看出,在研究区域中相对于物理过程的影响,光漂白对SR变化的作用较弱[18].这是由于2、3 月份光强较弱造成的光漂白效果不明显.图6 SR与盐度的关系Fig.6 Relationship between SRand salinity图7 SR与a330的关系Fig.7 Relationship between SRand a3302.3 CDOM对DOC示踪作用2.3.1 DOC 分布特征及河口混合行为研究区域表层水中DOC 质量浓度(以C 计,下同)为1.06~3.20 mg/L,平均值为(1.74±0.56)mg/L(图8).DOC 质量浓度大体趋势为近岸高远岸低,主要受长江冲淡水影响.图8 DOC质量浓度水平分布图Fig.8 Horizontal distribution of DOC concentrationsDOC 质量浓度与盐度的关系如图9 所示.研究区域表层水DOC 质量浓度和盐度总体呈现负相关关系(R2=0.820,8,p<0.000,1,n=32),两者关系为DOC 质量浓度与盐度之间存在良好的负相关关系,表明该区域溶解有机物浓度受控于保守性的稀释扩散机制,其输入量受控于陆源径流.表层水DOC河口混合为保守混合行为,说明DOC 混合主要是物理过程的影响,化学及微生物作用的影响较小.图9 DOC质量浓度与盐度的关系Fig.9 Relationship between DOC and salinity2.3.2 CDOM 吸收系数a330与DOC 质量浓度的关系通过上文研究结果发现 DOC 质量浓度和CDOM 吸收系数基本呈现一致的变化趋势.因此,希望利用CDOM 的光学特性快速准确地估算表层海水中的DOC 质量浓度.CDOM 吸收系数a330与DOC质量浓度的关系如图10 所示.结果表明二者存在较好的正相关关系(R2=0.852,5,p <0.000,1,n=32).两者之间的关系为由此表明在长江口及其邻近海域表层水中CDOM 可以作为DOC 的示踪物质.一些研究证明CDOM 吸收系数与DOC 质量浓度存在显著的正相关性[19-20].但是,由于不同流域与不同季节输水量有所不同,所以二者的具体关系仍需要更多的数据进行下一步完善.图10 a330与DOC质量浓度的关系Fig.10 Relationship between a330and DOC3 结论(1)研究区域表层水中CDOM 混合总体上为保守混合行为,陆源为CDOM 的主要来源,主要是长江淡水径流输入,长江冲淡水向南流动造成CDOM沿锋区分布.陆源CDOM 含有较多芳香性碳,随着海源不断地增加,CDOM 中芳香性碳逐渐减少.(2)SR分别与盐度和a330符合保守性混合模型,说明SR的分布受光漂白的影响较小,主要受长江冲淡水与海水混合过程物理因素的影响.(3)研究区域表层水中DOC 质量浓度分布随着盐度的升高总体呈下降趋势,DOC 混合大体表现为保守混合.CDOM 与 DOC 相关性较好:ρDOC=0.501,6a330+1.075,7(R2=0.855,1),CDOM 可以作为DOC 的示踪物质.参考文献:[1]施坤,李云梅,王桥,等.太湖、巢湖水体CDOM 吸收特性和组成的异同[J].环境科学,2010,31(5):1183–1191.[2]Hu C M,Lee Z P,Muller-Karger E,et al.Ocean color reveals phase shift between marine plants and yellow substance[J].IEEE Geoscience and Remote Sensing Letters,2006,3(2):262–266.[3]沈红,赵冬至,付云娜,等.黄色物质光学特性及遥感研究进展[J].遥感学报,2006,10(6):949–954.[4]石晓勇,李鸿妹,张传松,等.2006 年夏季黄、东海溶解有机碳的分布特征[J].海洋科学进展,2013,31(3):391–397.[5]吴稳.长江口水文环境信息与水下地形三维可视化应用研究[D].上海:华东师范大学,2010.[6]张述伟,王江涛,李宁,等.2010 年春季长江口邻近海区水体中溶解有机碳、氮的分布特征及其影响因素[J].海洋环境科学,2013,32(1):33–38.[7]Granakog M A,Macdonald R W,Mundy C J,et al.Distribution,characteristics and potential impacts of chromophoric dissolved organic matter(CDOM)in Hudson Strait and Hudson Bay,Canada[J].ContinentalShelf Research,2007,27(15):2032–2050.[8]王翔,杨红,孔德星,等.长江口外海水中有色溶解有机物(CDOM)的光吸收特性[J].台湾海峡,2010,29(4):518–524.[9]丁雁雁,张传松,石晓勇,等.春季黄渤海溶解有机碳的平面分布特征[J].环境科学,2012,33(1):37–41.[10]Keith D J,Yoder J A,Freeman S A.Spatial and temporal distribution of colored dissolved organic matter(CDOM)in Narragansett Bay,Rhode Island:Implications for phytoplankton in coastal waters[J].Estuarine,Coastal and Shelf Science,2002,55(5):705–717.[11]Babin M,Stramski D,Ferrari G M,et al.Variations in the light absorption coefficients of phytoplankton,nonalgal particles,and dissolved organic matter in coastal waters around Europe[J].Journal of Geophysical Research:Oceans,2003,108(C7):4-1–4-20.[12]Twardowski M S,Boss E,Sullivan J M,et al.Modeling the spectral shape of absorption by chromophoric dissolved organic matter[J].Marine Chemistry,2004,89(1):69–88.[13]Helms J R,Stubbins A,Ritchie J D,et a1.Absorption spectral slopes and slope ratios as indicators of molecular weight,source and photobleaching of chromophoric dissolved organic matter[J].Limnology and Oceanography,2009,54(3):955–969.[14]Bowers D G,Brett H L.The relationship between CDOM and salinity in estuaries:An analytical and graphical solution[J].Journal of Marine Systems,2008,73(1/2):1–7.[15]李猛,郭卫东,夏恩琴,等.厦门湾有色溶解有机物的光吸收特性研究[J].热带海洋学报,2006,25(1):9–14.[16]Weishaar J L,Aiken G R,Bergamaschi B A,et al.Evaluation of specific ultraviolet absorbance as an indicator of the chemical composition and reactivity of dissolved organic carbon [J].Environmental Science and Technology,2003,37(20):4702–4708.[17]Stedmon C A,Markager S.Behaviour of the optical properties of coloured dissolved organic matter under conservative mixing[J].Estuarine,Coastal and Shelf Science,2003,57(5/6):973–979.[18]Xie H,Bélanger S,Song G,et al.Photoproduction of ammonium in the southeastern Beaufort Sea and its biogeochemicalimplications[J].Biogeosciences,2012,9(8):3047–3061.[19]Del Vecchio R,Blough N V.Spatial and seasonal distribution of chromophoric dissolved organic matter and dissolved organic carbon in the Middle Atlantic Bight[J].Marine Chemistry,2004,89(1/2/3/4):169–187.[20]Rochelle-Newall E J,Fisher T R.Chromophoric dissolved organic matter and dissolved organic carbon in Chesapeake Bay[J].Marine Chemistry,2002,77(1):23–41.。
海洋荧光溶解有机物研究进展

第 26 卷第 1 期海洋通报V ol. 26,No.1 2007 年 02 月 JOURNAL OF MARINE SCIENCE BULLETIN Feb. 2007海洋荧光溶解有机物研究进展郭卫东,程远月,吴芳(厦门大学海洋学系,福建厦门 361005)摘要:海洋荧光溶解有机物(FDOM)是海洋有色溶解有机物中可产生荧光的组分,其理化性质对于海洋上层的水色遥感、光化学以及浮游植物的生产力和生态系统结构与功能等都有重要影响。
总结了 FDOM 的 3 种荧光光谱分析技术及其特点,重点对其两种主要成分(类腐殖质和类蛋白质荧光物质)的荧光特性、分布变化以及来源、分布变化及其去除等进行了全面细致的综述,阐述了研究 FDOM 的海洋学意义,并对今后有待深入研究的重点问题作了展望。
关键词:荧光溶解有机物;有色溶解有机物;类腐殖质;类蛋白质;荧光光谱中图分类号:P734 文献标识码:A 文章编号:1001-6932(2007)01-0098-0106天然海水中的荧光物质可分为两部分:由溶解有机物(DOM)产生的“溶解”荧光 [1]和由浮游植物光合色素产生的“颗粒”荧光 [2],前者被称为荧光溶解有机物 ( Fluorescent Dissolved Organic Matter, 简称FDOM )。
根据 FDOM 结构和性质的差异,可将其分为两类:类蛋白质 ( protein-like ) 和类腐殖质( humic-like ) 荧光物质 [3],类蛋白质荧光物质由具有芳香结构的氨基酸如色氨酸、酪氨酸等产生 [4],而类腐殖质荧光物质则由分子结构复杂的腐殖质类物质产生。
它们是海洋有色溶解有机物 ( chromophoric or colored dissolved organic matter,简称CDOM ) 吸收紫外可见光后可发射荧光的部分。
FDOM 是海洋 DOM 的主要成分之一,其地球化学过程是全球碳通量和海洋碳循环的重要而活跃的部分 [5]。
三维荧光光谱及平行因子分析法在CDOM研究中的应用_刘笑菡
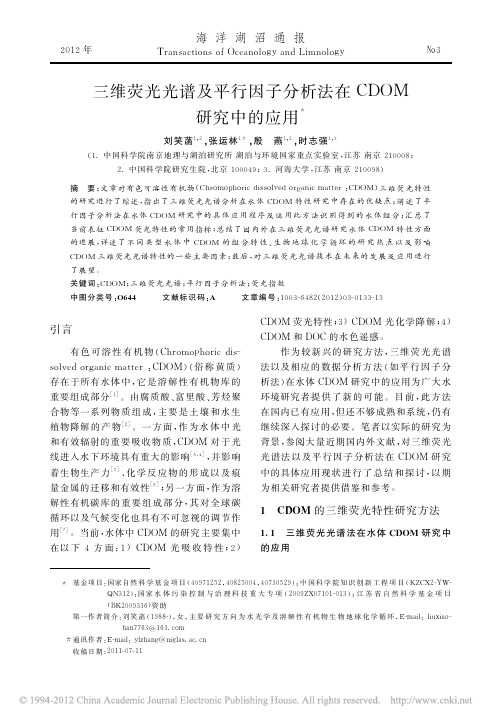
* 通 讯 作 者 :E-mail:ylzhang@niglas.ac.cn 收 稿 日 期 :2011-07-11
荧光光谱分析法已经广泛应用于分析水 体中 DOM 的 来 源,时 间 以 及 反 应 活 性。 然 而,DOM 中每种组分荧光光谱对 DOM 荧光
发射和吸收的仪器响应还没有一致的标准。
有研究者运用制造商提供的发射和吸收校正 文档,估 测 3 个 不 同 的 荧 光 计:Fluoromax-3 荧 光 计 (Horiba集 团Jobin Yvon 公 司 ),Cary Eclipse荧 光 计 (美 国 Varian 仪 器 公 司 ), LS50B 荧光计(Perkin Elmer仪器公司)对于 DOM 荧 光 光 谱 的 响 应。 研 究 结 果 显 示,尽 管样品在不同荧光计上的测定结果在校准前 差异显著,且 不 同 仪 器 生 产 商 提 供 的 仪 器 校 准因子有差别,但 是 经 校 准 后 同 一 样 品 测 定 的荧光谱图 的 重 叠 度 提 高。 因 而,根 据 同 一 资料 组 DOM 荧 光 数 据 趋 势 得 出 的 结 论,不 受所使用的仪器种类的影响 。 [15]
摘 要:文章对有色可溶性有机物(Chromophoric dissolved organic matter:CDOM)三 维 荧 光 特 性 的研究进行了综述,指出了三维荧光光谱分析在水 体 CDOM 特 性 研 究 中 存 在 的 优 缺 点;阐 述 了 平 行因子分析法在水体 CDOM 研究中 的 具 体 应 用 程 序 及 运 用 此 方 法 识 别 得 到 的 水 体 组 分;汇 总 了 当前表征 CDOM 荧光特性的常用指标;总结了国 内 外 在 三 维 荧 光 光 谱 研 究 水 体 CDOM 特 性 方 面 的进展,详述了 不 同 类 型 水 体 中 CDOM 的 组 分 特 性、生 物 地 球 化 学 循 环 的 研 究 热 点 以 及 影 响 CDOM 三维荧光光谱特性的一些主要因素;最后,对 三 维 荧 光 光 谱 技 术 在 未 来 的 发 展 及 应 用 进 行 了展望。 关 键 词 :CDOM;三 维 荧 光 光 谱 ;平 行 因 子 分 析 法 ;荧 光 指 数 中 图 分 类 号 :O644 文 献 标 识 码 :A 文 章 编 号 :1003-6482(2012)03-0133-13
- 1、下载文档前请自行甄别文档内容的完整性,平台不提供额外的编辑、内容补充、找答案等附加服务。
- 2、"仅部分预览"的文档,不可在线预览部分如存在完整性等问题,可反馈申请退款(可完整预览的文档不适用该条件!)。
- 3、如文档侵犯您的权益,请联系客服反馈,我们会尽快为您处理(人工客服工作时间:9:00-18:30)。
RESEARCH ARTICLEDynamics of chromophoric dissolved organic matter influenced by hydrological conditions in a large,shallow,and eutrophic lake in ChinaYongqiang Zhou 1,2&Yunlin Zhang 1&Kun Shi 1&Xiaohan Liu 1,2&Cheng Niu 1,2Received:4January 2015/Accepted:16April 2015/Published online:29April 2015#Springer-Verlag Berlin Heidelberg 2015Abstract High concentrations of chromophoric dissolved or-ganic matter (CDOM)are terrestrially derived from upstream tributaries to Lake Taihu,China,and are influenced by hydro-logical conditions of the upstream watershed.To investigate how the dynamics of CDOM in Lake Taihu are influenced by upstream inflow runoff,four sampling cruises,differing in hydrological conditions,were undertaken in the lake and its three major tributaries,rivers Yincun,Dapu,and Changdou.CDOM absorption,fluorescence spectroscopy,chemical oxy-gen demand (COD),and stable isotope δ13C and δ15N mea-surements were conducted to characterize the dynamics of CDOM.The mean absorption coefficient a (350)collected from the three river profiles (5.15±1.92m −1)was significantly higher than that of the lake (2.95±1.88m −1),indicating that the upstream rivers carried a substantial load of CDOM to the lake.This finding was substantiated by the exclusively terres-trial signal exhibited by the level of δ13C (−26.23±0.49‰)of CDOM samples collected from the rivers.Mean a (350)and COD in Lake Taihu were significantly higher in the wet sea-son than in the dry season (t test,p <0.0001),suggesting that the abundance of CDOM in the lake is strongly influenced by hydrological conditions of the watershed.Four components were identified by parallel factor analysis,including two protein-like components (C1and C2),a terrestrial humic-like component (C3),and a microbial humic-like (C4)com-ponent.The contribution percentage of the two humic-like components relative to the summed fluorescence intensity of the four components (C humic )increased significantly from the dry to the wet season.This seasonal difference in contribution further substantiated that an enhanced rainfall followed by an elevated inflow runoff in the lake watershed in the wet season may result in an increase in humic-like substances being discharged into the lake compared to that in the dry season.This finding was further supported by an elevated a (250)/a (365)of CDOM samples collected in the lake in the wet season than in the dry season.Significantly higher mean levels of C3and a (350)were recorded for CDOM samples collected from River Yincun than those from rivers Dapu and Changdou,differing in seasons,suggesting the significance of terrestrial CDOM input from River Yincun.Keywords Chromophoric dissolved organic matter(CDOM).Lake Taihu .Parallel factor (PARAFAC)analysis .Hydrological conditionsIntroductionFreshwater lakes serve as important drinking water sources in many places around the world (Williamson et al.2008;Williamson et al.2009);however,high concentration of chro-mophoric dissolved organic matter (CDOM)present in these water bodies is responsible for the formation of carcinogenic byproducts that can compromise the safety of the drinking water source (Zhang et al.2011a ;Duan et al.2014).CDOM is also responsible for unpleasant odor and taste during drink-ing water treatment processes (Baghoth et al.2011;Meng et al.2012).The translocation and transformation of CDOM in lakes and coastal waters are mediated by its structure andResponsible editor:Philippe Garrigues *Yunlin Zhangylzhang@1Taihu Laboratory for Lake Ecosystem Research,State KeyLaboratory of Lake Science and Environment,Nanjing Institute of Geography and Limnology,Chinese Academy of Sciences,73East Beijing Road,Nanjing 210008,People ’s Republic of China 2University of Chinese Academy of Sciences,Beijing 100049,ChinaEnviron Sci Pollut Res (2015)22:12992–13003DOI 10.1007/s11356-015-4556-xrelative abundance;thus,it can be beneficial to know the temporal–spatial dynamics of CDOM in these waters(Zhang et al.2011a).CDOM displays conservative mixing behavior in lakes and coastal waters,and its geochemical cycling pro-cesses are often coupled with river-borne pollutants (Yamashita et al.2008;Zhang et al.2011a;Hosen et al. 2014).Furthermore,CDOM can strongly absorb light in the blue part of visible region and can interfere with the remote sensing estimation of phytoplankton and nonalgal particle matter(Lee et al.2011;Hu et al.2012;Song et al.2013; Huang et al.2014).Thus,numerous studies have been dedi-cated to investigating factors that control the source,cycling, and fate of CDOM in lakes and coastal waters(Stedmon and Markager2005;Ishii and Boyer2012;Asmala et al.2013; Bianchi et al.2013;Guo et al.2014).In large,shallow,and eutrophic lakes,however,in addition to terrestrial origin,CDOM may also be produced and trans-formed through biological metabolism or released from lakebed sediment by wind-induced sediment resuspen-sion(Qin et al.2007).In inland and coastal waters, upstream tributaries carry substantial CDOM and dis-charge to downstream-linked water bodies(Stedmon and Markager2005;Zhang et al.2011a;Yang et al. 2013;Guo et al.2014).The presence of high CDOM concentration in coastal waters and bays derived from upstream rivers can result in eutrophication and algal blooms (Pearl1988).Precipitation may result in an elevated export of terrestrial CDOM fluorescence from upstream tributaries to downstream-linked waters(Stedmon and Markager2005; Zhang et al.2011a).How hydrological conditions may medi-ate CDOM dynamics in large,shallow,and highly eutrophic lakes remains to be elucidated.Lake Taihu,located in the Yangtze River Delta,has a water area of2338km2and a mean depth of1.9m,ranking it the third largest freshwater lake in China.Rapid economic devel-opment over the last three decades has resulted in high spatial heterogeneity in the lake,with its northern and northwestern regions dominated by algae and its southeastern region dom-inated by macrophytes(Zhu et al.2008).The lake has an average water level of∼3.3m above sea level,the lake basin has an area of36,900km2,and more than40million people live within the watershed.In the Lake Taihu basin,172rivers or channels connect to the lake(Qin et al.2007);most inflow tributaries are in the western and northwestern regions,and most outflow rivers are in the southern and southeastern sub-basins.Economic booms in nearby cities and towns since the 1980s have increased the amount of sewage being discharged into Lake Taihu(Qin et al.2010).In the large,shallow, and eutrophic lake,algal blooms caused by Microcystis spp. have occurred throughout summer since the last decade (Qin et al.2007).In the lake,in addition to in situ production of CDOM from the degradation of phytoplankton and aquatic macrophytes,CDOM export from upstream tributaries is the most important source to the whole CDOM pool of the lake(Zhang et al. 2011b).Previous results indicated that CDOM in lakes and coastal waters may be strongly influenced by hydrological conditions,especially inflow runoff,of upstream watersheds (Stedmon and Markager2005;Coble2007;Zhang et al. 2011a;Guo et al.2014).For example,a substantial increase in inflow runoff in the wet season may result in an elevated CDOM concentration of a downstream-linked lake as a result of high concentration CDOM input.Similarly,CDOM con-centration of a downstream-linked lake may drop as a result of a dilution effect by flushing low concentration CDOM waters from upstream tributaries.A recent study conducted in Lake Taihu found an elevated CDOM absorption coefficient in the wet season compared to the dry season(Zhang et al.2011b);however,the behaviors of different components of the overall CDOM dynamics remain unknown.Another study performed in the same region pro-vided a snapshot of the relative abundance of parallel factor (PARAFAC)analysis components(Yao et al.2011),but sea-sonal dynamics of CDOM mediated by hydrological condi-tions were lacking.The details of how hydrological condi-tions,including rainfall and inflow runoff,may alter the spa-tial and temporal dynamics of CDOM in the lake remain unknown.To address this knowledge gap,our objective was to char-acterize how the dynamics of CDOM in Lake Taihu may respond to different hydrological conditions,in-cluding rainfall and inflow runoff of the upstream wa-tershed.We used spectral absorption,chemical oxygen demand(COD),fluorescence spectroscopy,and stable isotopeδ13C andδ15N to characterize the dynamics of CDOM mediated by hydrological conditions in the lake. We assumed that the maximum fluorescence intensities(F max) of individual PARAFAC components are proportional to the concentrations of corresponding fluorescent fractions (Borisover et al.2009).Materials and methodsSample collectionFour sampling cruises were performed in Lake Taihu(Fig.1), one each in winter(February),spring(May),summer (August),and autumn(November)2011.Surface water sam-ples(0–0.5m)were taken from the lake and from profiles of its three major tributaries,rivers Changdou,Dapu,and Yincun (Fig.1).Thirty-two total sites were distributed evenly in Lake Taihu,composed of11sites along each of the three river profiles:sites1–5in the lake,site6at the three river mouths, and sites7–11upstream in the river(Fig.1).Note that thelocations of site Dapu 5and Yincun 4were the same as Taihu 10and Taihu 16,respectively (Fig.1).Thus,252total surface water samples were collected:Lake Taihu 128(32sites×4seasons)+Changdou 44(11sites×4seasons)+Dapu 40(10sites×4seasons)+Yincun 40(10sites×4seasons).These samples were grouped into two spa-tial categories,the lake group (samples collected from Lake Taihu)and the river group (samples collected from the three river profiles;Fig.1).The four cruises were divided into three seasonal groups according to the rainfall of the watershed and the water level of the lake (Fig.2):dry season (low water season),February+May (winter+spring);wet season (high water season),August (summer);and wet-to-dry transition season,November (autumn).Note that inflow runoff to the lake was relatively low in November compared to the rest of the year (Fig.2).Hydrological dataHydrological data,including the monthly rainfall of Lake Taihu Basin,monthly inflow runoff to the lake,and the corre-sponding outflow runoff,were provided by the Taihu Basin hydrological information service system (//tba/content/SWJ/sqyb/index.html ).Measurements of CDOM absorption and CODWater samples were held on ice while in the field and transported to the laboratory where they were filtered imme-diately.A small portion of water samples that cannot be fil-tered immediately in summer was stored at −20°C to prevent microbial degradation due to high air temperatures and then filtered within 2days.Our previous study showed that freez-ing the original water samples for 2days did not produce a marked change in the CDOM absorption (Zhang et al.2011a ).The filtered samples were stored in the dark at 4°C for CDOM optical and fluorescence measurements.CDOM optical and fluorescence measurements were finished within 3days,and all laboratory measurements were completed within 1week after field sampling.Samples were first filtered through precombusted 47-mm diameter Whatman GF/F filters (0.7μm porosity)and then through prerinsed 25-mm diameter Millipore membrane cel-lulose filters (0.22μm porosity)to determine CDOM absorp-tion coefficient and fluorescence excitation –emission matrices (EEMs).CDOM absorption spectra were obtained between 200and 800nm at 1-nm intervals using a Shimadzu UV-2450PC UV –vis recording spectrophotometer with matching 5-cm quartz li-Q water was used as reference.Absorbance measurements at each wavelength (λ)wereFig.1Locations of sampling sites in Lake Taihu and profiles of its three major tributaries:rivers Changdou,Dapu,andYincunFig.2a Variations of daily rainfall (in mm)of Lake Taihu Basin and water level (in m above sea level)of the lake in 2011gray bars denote sampling collection date.b Monthly variations of mean rainfall in the lake basin,and inflow runoff to the lake and corresponding outflow runoffbaseline-corrected by subtracting corresponding absorbance values at 700nm.Absorption coefficients were obtained using the following equation:a λðÞ¼2:303ÂD λðÞ=rð1Þwhere a (λ),D(λ),and r are CDOM absorption coefficient at wavelength λ,corrected optical density at wavelength λ,and cuvette path length (in m),respectively.In this study,the abundance of CDOM was expressed using a (350),which has been widely used in CDOM studies (Stedmon et al.2007;Osburn et al.2011).Spectral slope of CDOM was cal-culated by nonlinear fit over the 280–500nm range,using the following equation:a λðÞ¼a λ0ðÞÂe S λ0−λðÞ½ þkð2Þwhere a (λ)and a (λ0)are the corrected CDOM absorption coefficients at wavelength of λand reference wavelength λ0=440nm,respectively.S and k represent CDOM spectral slope and background parameter,respectively.The spectral slope ratio (S R )was defined as the ratio of spectral slope over wavelength range of 275–295nm to 350–400nm (i.e.,S 275–295/S 350–400).S R of <1and >1indicated CDOM of terrestrial and in situ autochthonous origins,respectively;solar exposure could result in a notable increase in S R of CDOM sample (Helms et al.2008).The ratio of CDOM absorption coeffi-cients at 250and 365nm,i.e.a (250)/a (365),was used to semi-quantitatively determine the relative molecular size of CDOM samples;the ratio increases with decreasing of CDOM molecular size (De Haan 1993;Helms et al.2008;Zhang et al.2013).Water samples filtered through 47-mm Whatman GF/F fil-ters were determined for COD concentration using a colori-metric method with potassium dichromate –sulfuric acid as reagent (Yao et al.2011).COD was only measured for sam-ples collected from the lake (n =128).CDOM fluorescence measurementCDOM EEMs were measured using a Hitachi F-7000fluores-cence spectrometer (Hitachi High-Technologies,Tokyo,Japan)with a 700-voltage xenon lamp.The excitation and emission spectra for scanning ranges spanned 200–450nm (every 5nm)and 250–600nm (every 1nm),respectively,with a 5-nm slit width for both excitation and emission.The EEMs were Raman-calibrated by subtracting Milli-Q water blanks.The spectra were corrected for instrumental response accord-ing to the procedure recommended in the Hitachi F-7000Instruction Manual,which comprised both excitation and emission calibration.Excitation was calibrated using Rhodamine B as standard and a single-sided frosted red filter in excitation scan mode,while emission was calibrated with adiffuser in synchronous scan mode.The subsequent excitation and emission spectra were internally corrected using Fluorescence Solutions 2.1software (internally installed in the instrument).Fluorescence intensity was calibrated in quinine sulfate units (QSU),where 1QSU represents the maximum fluores-cence intensity of 0.01mg/L of quinine in 1mol/L H 2SO 4at excitation=350nm and emission=450nm (Zhang et al.2010).Rayleigh scatter effects were removed by replacing the EEMs regions at emission wavelength ≤excitation wave-length+15nm,and at emission wavelength ≥2×excitation wavelength −20nm,with zeros.The inner-filter effect was calibrated according to the following equation (McKnight et al.2001):F cor ¼F obs Â10A Ex :þA Em :ðÞ=2ð3Þwhere F obs and F cor are fluorescence intensity before and after calibration,and A Ex.and A Em.are absorbance at corresponding excitation and emission wavelengths.PARAFAC modelingA total of 252EEMs were modeled using PARAFAC in DOMFluor Toolbox (Stedmon and Bro 2008),MATLAB 2012a software.The EEMs after inner-filter effect correction were further processed before PARAFAC modeling by delet-ing excitation wavelength from 200to 225nm and emission wavelength from 250to 300nm and 550to 600nm to elim-inate instrument measurement errors.All 252EEMs were split into a calibration half and a validation half and were run step-wise from three to seven components on both halves.Outputs of the PARAFAC model,such as sum of squares of residuals and explained variation of the model,indicated that a four-component model was sufficient to explain more than 99%of total EEM variables and was considered adequate to describe the EEMs dataset.Stable isotope δ13C and δ15N measurementsThree water samples (site 1,6,and 11)from each of the three river profiles were collected in the dry and wet seasons and stored at −20°C in the laboratory for stable isotope analysis after filtering through precombusted 47-mm diameter Whatman GF/F filters (0.7μm porosity).A few drops of HCl solution (1mol/L)were used to acidify samples to ap-proximately pH=2to remove the interferences of dissolved inorganic carbon.The samples were dried to constant weight under low temperature (55±5°C).The solid residue (i.e.,DOM)was stored in a desiccator and subsequently combusted using a Flash EA1112analyzer.CO 2and N 2gases were mea-sured using a Thermo FinniganMAT Delta plus dual-inlet con-tinuous flow isotope ratio mass spectrometer,with precisionof better than±0.1‰for both carbon and nitrogen.Stable isotope values for nitrogen and carbon are expressed asδ15N andδ13C,respectively.The ratios15N/14N and13C/12C were calibrated against high-purity N2gas and the Vienna Pee Dee Belemnite(VPDB)standard,respectively(Pruell et al.2006; Osburn and St-Jean2007;Hood et al.2009).The stable iso-tope values were calculated using the following equation:δA¼R sam=R sta−1ðÞÂ1000ð4ÞwhereδA is eitherδ15N orδ13C,R sam represents the isotope ratios of the samples collected(i.e.,13C/12C or15N/14N),and R sta represents the isotope ratios of the known isotopic standards.Statistical analysesStatistical analyses(mean value,standard deviation,t test,and linear regression)were performed using R-studio0.97.551 software.Linear fit,nonlinear fit,and the calculation of spec-tral slopes(S)of CDOM absorption spectra were operated using MATLAB2012a software.Significance level was re-ported as p<0.05in t test and correlation analysis.Results and discussionVariation of CDOM optical properties and CODunder different hydrological conditionsThe a(350)of all samples ranged from1.66to18.72m−1,with high levels of a(350)recorded in the Zhushan Bay and Meiliang Bay,especially the river mouths of Yincun and Dapu(Fig.3).COD concentrations ranged from2.45to 14.87mg/L(mean of4.54±1.69mg/L)and shared a similar distribution pattern with a(350),with high levels of COD found in Zhushan Bay and Meiliang Bay.The maximum of both a(350)and COD appeared during the wet season in TH6, located at the river mouth of another tributary,river Zhihu (Fig.1),a river in the city of Wuxi.This finding implied that CDOM in Lake Taihu,especially in Zhushan Bay and Meiliang Bay,was strongly influenced by upstream terrestrial input.The a(350)of all samples taken from the three river profiles,Changdou,Dapu,and Yincun(5.15±1.92m−1),were significantly higher than all samples from Lake Taihu(2.95±1.88m−1)(Table1),further verifying that a substantial amount of CDOM was carried and discharged into the lake by its tributaries.This was consistent with many studies conducted in other aquatic ecosystems that an increased precipitation and inflow runoff can result in an increased CDOM export to downstream-linked waters(Stedmon and Markager2005; Fellman et al.2009;Miller and McKnight2010;Yang et al. 2013).Seasonally,mean a(350)values of all the three river profiles were significantly higher than in the lake in the dry season,and only samples collected from River Yincun were significantly higher than in the lake in the wet season(Table1).Note that the mean a(350)of CDOM samples collected from River Yincun was significantly higher than that of the lake and rivers Changdou and Dapu,differing by seasons(Table1),indicating that a large quantity of CDOM was accumulated in Zhushan Bay.This was consistent with results that river mouths are considered as the sinks of particulate organic matter and sources of CDOM for inland and coastal waters(Shen et al.2012).In Lake Taihu,a significantly higher mean a(350)was found in the wet season than in the dry season(p<0.0001; Table1).Similarly,mean COD values shared a significantly higher level in the wet season(5.29±2.02mg/L)than in the dry season(4.27±0.95mg/L,t test,p<0.05),implying that vast amounts of CDOM were discharged and accumulated in the lake in the wet season compared to the dry season. Spatially,high levels of a(350)expanded from Zhushan Bay in the dry season to the northwestern half of the lake in the wet-to-dry transition season and further to the whole lake in the wet season(Fig.3),an additional indication that a substan-tial amount of CDOM was discharged into the northwestern regions of the lake in the wet season compared with that in the dry season.A notable decrease in the mean level of a(350)for samples collected from the three river profiles was recorded in the wet season compared to the dry seasons(Table1),proba-bly the result of a higher dilution effect caused by a substantial increase in inflow runoff of the three tributaries in the wet season compared with that in the dry season(Zhang et al. 2011a;Guo et al.2014).CDOM in Lake Taihu shared a significantly lower a(250)/ a(365)in the wet season than in the dry season(Fig.3; Table1),probably a result of terrestrial CDOM input. Previous studies indicated that terrestrial CDOM tends to have a higher molecular weight[lower level of a(250)/a(365)]com-pared with CDOM associated with in situ biological metabo-lisms(Helms et al.2008;Fichot and Benner2012).As further support,both CDOM quantity and quality in the lake were strongly influence by runoff-mediated terrestrial CDOM in-put.Lower a(250)/a(365)levels in CDOM samples collected from the three river profiles compared to the lake further in-dicated that substantial CDOM was discharged to the lake from upstream watershed(Helms et al.2008;Zhang et al. 2011a,b).Spatially,the area with high levels of a(250)/a(365) downsized from the whole lake in the dry season to the south-eastern half of the lake in the wet-to-dry transition season and further to Xukou Bay and Eastern Taihu Bay in the wet season (Fig.3).This systematic decrease exhibited an increased ter-restrial CDOM input from northwestern regions of the lake in the wet season compared to the dry season(Zhang et al. 2011b),substantiated by the significantly lower meana (250)/a (365)and S R of CDOM samples collected from the river Yincun compared to Lake Taihu in different seasons (Table 1).River Yincun carried high concentration of terres-trial CDOM and discharged into the lake,and this portion of terrestrial CDOM shares lower S R and a (250)/a (365)com-pared to CDOM produced internally from noncolored algal precursors in the lake (Fichot and Benner 2012;Yamashita et al.2013).PARAFAC componentsThe four components of PARAFAC included two autochtho-nous protein-like components (C1and C2),one terrestrial humic-like component C3,and one marine humic-like com-ponent C4(Fig.4).C1displayed three fluorescent peaks with excitation/emission maxima at ≤230nm (285,340nm)/361nm,similar to tryptophan-like fluorophore (peak T)(Coble 1996;Coble et al.1998).C2exhibited a tyrosine-like fluorophore,which was similar to peak B.These two compo-nents,C1and C2,together demonstrated the autochthonous properties contained in CDOM (Coble 1996;Coble et al.1998;Stedmon and Markager 2005;Zhang et al.2010).C3had similar excitation/emission maxima as peaks A (ultravio-let region)and C (visible region)and had characteristics of terrestrially derived humic-like material (Coble 1996;Coble et al.1998;Stedmon and Markager 2005;Zhang et al.2010).Fig.3CDOM absorptioncoefficient a (350)in the a dry,b wet-to-dry transition,and c wet season.Chemical oxygen demand (COD)concentration in the d dry,e wet-to-dry transition,and f wet seasonTable 1Optical properties a (350)(m −1),MS (arbitrary unit,AU)and S R (AU)of CDOM and fluorescence intensity of the four components (in QSU)identified by PARAFAC model of water samples taken from Lake Taihu and rivers Changdou (CD),Dapu (DP),and Yincun (YC)in the wet and dry season,and significance levels of difference between parameters using t test under these two hydrological conditions Parameters CDOM optical properties PARAFAC components SitesSeasons a (350)MSS RC1C2C3C4Lake TaihuDry 2.46±0.829.60±1.12 1.21±0.1037.95±16.6118.34±6.308.23±5.37 2.41±1.39Wet 4.22±3.207.46±1.24 1.37±0.1724.15±13.4034.16±19.5313.60±10.169.81±2.93p value<0.0001<0.0001<0.0001<0.0001<0.0001<0.0001<0.0001River CDDry 4.33±1.69 6.37±2.26 1.34±0.0529.90±7.1021.48±2.83 6.57±0.57 6.00±1.52Wet2.44±0.178.80±0.36 1.32±0.1411.62±11.8713.34±3.2810.55±0.517.88±1.99p value <0.0001<0.0001>0.05<0.0001<0.0001<0.0001<0.0001River DPDry 6.26±1.56 6.01±1.29 1.22±0.0856.69±18.3023.15±8.6217.50±3.47 6.50±1.17Wet 4.47±0.337.63±0.66 1.35±0.1623.64±7.9013.24±5.3517.07±3.829.63±2.48p value <0.0001<0.0001<0.0001<0.0001<0.0001>0.05<0.0001River YCDry 7.08±1.50 5.55±1.06 1.13±0.1180.18±31.8233.48±13.2633.75±9.20 5.29±10.46Wet 5.87±0.81 6.26±0.73 1.21±0.0329.18±8.089.87±4.1722.42±1.637.96±2.57p value<0.0001<0.0001<0.001<0.0001<0.0001<0.0001<0.001C4displayed fluorescence spectrum similar to peak M (Coble 1996;Coble et al.1998)and was considered microbially transformed products from terrestrially derived components (Stedmon and Markager 2005;Zhang et al.2010).Variations of CDOM fluorescence intensity under different hydrological conditionsAll the four components identified by PARAFAC were well validated by the split-half validation procedure (Fig.4).Mean fluorescence intensity (F max )and contributions of C1,C2,C3,and C4for all datasets were 45.05±32.66,24.03±13.20,16.52±12.75,and 8.81±10.46QSU and 47.71,25.46,17.50,and 9.33%,respectively (Table 1).In Lake Taihu,mean F max of C2,C3,and C4were significantly higher in the wet season than in the dry season;the opposite trend was recorded for C1,with high levels found in the dry season compared with the wet season (Table 1).Our results imply that the dynamics of CDOM composition in Lake Taihu is strongly influenced by hydrological conditions of the water-shed,and was consistent with observations in other aquatic ecosystems (Stedmon and Markager 2005;Fellman et al.2009;Yang et al.2013).Mean F max of protein-like C1and C2in the river profiles was significantly higher in the wet season than in the dry season (Table 1).Note that the mean F max of terrestrial humic-like C3was significantly higher in river Yincun than in the other two river profiles (Table 1),implying that river Yincun was responsible for the high level of CDOM in Zhushan Bay.Seasonally,mean F max of C3in river Yincun was significantly higher in the dry season than in the wet season,probably the result of the dilution effects of the inflowing rivers (Table 1).For the microbial humic-like C4,mean F max for samples collected from Lake Taihu and the three river profiles had significantly higher levels in the wet season than in the dry season (Table 1),most likely the result of the close association between the presence and abundance of microbial humic-like component with the allochthonous precursors (Yamashita et al.2011;Yao et al.2011).The microbial humic-like C4is often considered to be associated with microbial transformation from terrestrial humic-like sub-stances (Yamashita et al.2008;Yamashita and Tanoue 2009;Williams et al.2010).During the wet season,CDOM microbially transformed from algal substrate in the lake can also result in an elevated level of F max of the microbial humic-like component (Zhang et al.2009).Note that the mean F max of C3collected from the river Changdou profile was signifi-cantly higher in the wet season than in the dry season (Table 1).River Changdou (also referred to as River Tiaoxi)is the largest tributary of Lake Taihu,and its watershed land use is dominated by mountain forests (Qin et al.2007).An enhanced rainfall and followed by an elevated inflow runoff in the wet season can enhance flushing of soil organic matter (Asmala et al.2012;Kothawala et al.2014;Shafiquzzaman et al.2014)in the watershed.Previous results indicated that humic-like components are closely associated with terrestrial CDOM input,and their dy-namics are often strongly influenced by upstream hydrologi-cal conditions,especially inflow runoff (Stedmon and Markager 2005;Guo et al.2014).Thus,the contribution per-centage of humic-like components (C3and C4)with regard to the summed F max (C humic ,in %)of the four components was investigated to trace the dynamics of CDOM influenced by hydrological conditions in the lake watershed.C humic in Lake Taihu in the wet season (35.76±12.45%)was significantly higher than in the dry season (16.49±5.86%,t test,p <0.0001)(Table 1).Spatially,the area of high level C humic expanded from the river mouths of tributaries in western sub-basins in the dry season to the whole lake in the wet season (Fig.5),attributed to a higher inflow runoff in the wet season.An elevated inflow runoff from the upstream watershed in the wet season compared to the dry season resulted in an elevated F max of humic-like materials and an elevated C humic in the downstream-linked Lake Taihu,especially Zhushan Bay,in-fluenced by upstream river Yincun.In the present study,C3has exclusively terrestrial characteristics (Kowalczuk et al.2009;Osburn et al.2012;Andrew et al.2013),and C4wasFig.4Four robust components were identified using PARAFAC model.Highly overlapping excitation (left )and emission (right )spectra were estimated using split-half validation procedure,with red and green lines representing twoindependent halves dataset and the black line representing the complete dataset。