Metal-Matrix Composites for Space Applications
金属基复合材料的主要特点
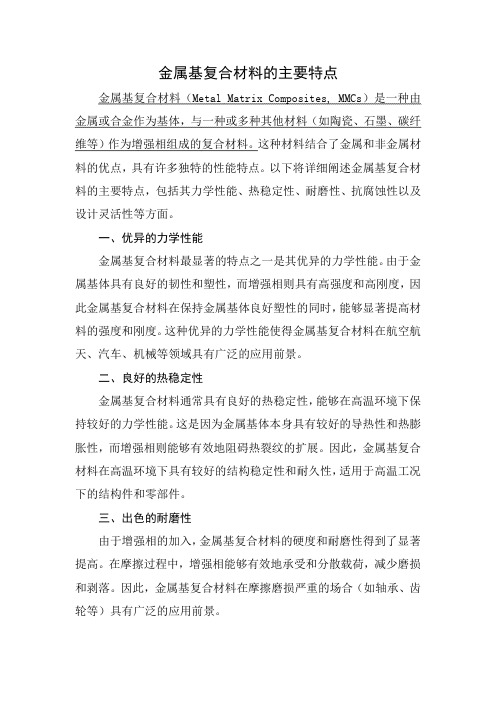
金属基复合材料的主要特点金属基复合材料(Metal Matrix Composites, MMCs)是一种由金属或合金作为基体,与一种或多种其他材料(如陶瓷、石墨、碳纤维等)作为增强相组成的复合材料。
这种材料结合了金属和非金属材料的优点,具有许多独特的性能特点。
以下将详细阐述金属基复合材料的主要特点,包括其力学性能、热稳定性、耐磨性、抗腐蚀性以及设计灵活性等方面。
一、优异的力学性能金属基复合材料最显著的特点之一是其优异的力学性能。
由于金属基体具有良好的韧性和塑性,而增强相则具有高强度和高刚度,因此金属基复合材料在保持金属基体良好塑性的同时,能够显著提高材料的强度和刚度。
这种优异的力学性能使得金属基复合材料在航空航天、汽车、机械等领域具有广泛的应用前景。
二、良好的热稳定性金属基复合材料通常具有良好的热稳定性,能够在高温环境下保持较好的力学性能。
这是因为金属基体本身具有较好的导热性和热膨胀性,而增强相则能够有效地阻碍热裂纹的扩展。
因此,金属基复合材料在高温环境下具有较好的结构稳定性和耐久性,适用于高温工况下的结构件和零部件。
三、出色的耐磨性由于增强相的加入,金属基复合材料的硬度和耐磨性得到了显著提高。
在摩擦过程中,增强相能够有效地承受和分散载荷,减少磨损和剥落。
因此,金属基复合材料在摩擦磨损严重的场合(如轴承、齿轮等)具有广泛的应用前景。
四、优异的抗腐蚀性金属基复合材料中的增强相通常具有较好的化学稳定性,能够有效地提高材料的抗腐蚀性能。
此外,通过合理的成分设计和表面处理,还可以进一步提高金属基复合材料的耐腐蚀性能。
这使得金属基复合材料在化工、海洋等腐蚀环境中具有广阔的应用前景。
五、设计灵活性高金属基复合材料的设计灵活性较高,可以通过调整基体和增强相的成分、含量和分布来实现对材料性能的定制和优化。
例如,通过改变增强相的种类、形状和取向,可以调整材料的强度和刚度;通过调整基体的成分和处理工艺,可以改善材料的塑性和韧性。
金属复合材料的优势和应用前景
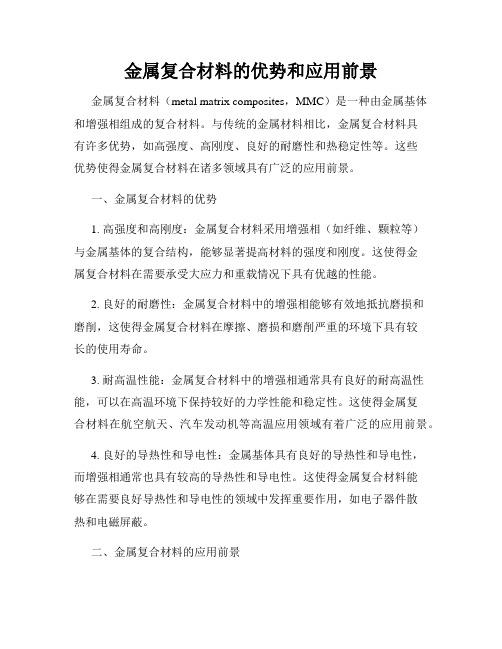
金属复合材料的优势和应用前景金属复合材料(metal matrix composites,MMC)是一种由金属基体和增强相组成的复合材料。
与传统的金属材料相比,金属复合材料具有许多优势,如高强度、高刚度、良好的耐磨性和热稳定性等。
这些优势使得金属复合材料在诸多领域具有广泛的应用前景。
一、金属复合材料的优势1. 高强度和高刚度:金属复合材料采用增强相(如纤维、颗粒等)与金属基体的复合结构,能够显著提高材料的强度和刚度。
这使得金属复合材料在需要承受大应力和重载情况下具有优越的性能。
2. 良好的耐磨性:金属复合材料中的增强相能够有效地抵抗磨损和磨削,这使得金属复合材料在摩擦、磨损和磨削严重的环境下具有较长的使用寿命。
3. 耐高温性能:金属复合材料中的增强相通常具有良好的耐高温性能,可以在高温环境下保持较好的力学性能和稳定性。
这使得金属复合材料在航空航天、汽车发动机等高温应用领域有着广泛的应用前景。
4. 良好的导热性和导电性:金属基体具有良好的导热性和导电性,而增强相通常也具有较高的导热性和导电性。
这使得金属复合材料能够在需要良好导热性和导电性的领域中发挥重要作用,如电子器件散热和电磁屏蔽。
二、金属复合材料的应用前景1. 航空航天领域:金属复合材料由于其高强度、高刚度和耐高温的特点,在航空航天领域具有广泛的应用前景。
例如,金属复合材料可以用于制造飞机结构件、发动机零部件和航天器热防护材料等。
2. 汽车工业:随着汽车行业对轻量化和节能环保要求的提升,金属复合材料作为一种重要的替代材料,其在汽车工业中的应用也越来越广泛。
金属复合材料可以应用于汽车发动机、底盘和车身结构等部件,以减轻整车重量、提高燃油效率和降低尾气排放。
3. 电子行业:金属复合材料具有良好的导热性和导电性,因此在电子行业中具有广泛的应用前景。
金属复合材料可以用于制造散热片、电磁屏蔽材料、半导体基底等,以提高电子器件的性能和稳定性。
4. 能源领域:金属复合材料的高强度、良好的耐高温性能和导热性,使其在能源领域具有潜在的应用前景。
新型包装材料
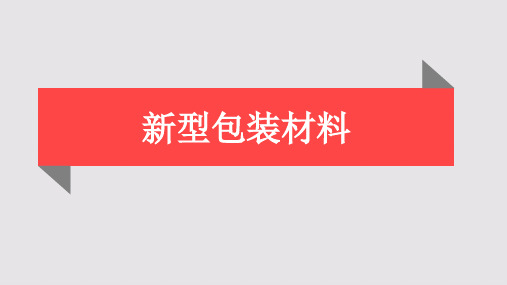
静电, 对包装物也会造成严重的后果。
纳米掺锑二氧化锡微粒具有良好的导电性, 浅色透明性, 良好的耐候性和稳定性 以及低的红外发射率等特性, 在包装材料中添加纳米掺锑二氧化锡微粒时, 涂层的导
电性能才明显改善, 可以消除的静电现象, 使得包装表面不再吸附灰尘, 可减少因摩擦
而导致的擦伤。
应用 功能(智能型纳米包装材料
纳米涂层包装材料具有色彩防伪、理化效应防伪等功能。
应用前景 Application prospect
纳米技术不仅可广泛应用于化纤、塑料、橡 胶、陶瓷、油漆、涂料、纸张等方面, 以改善产品 档,实现产品的某些特殊性能, 同时在无菌包装、 抗菌包装、除臭包装、高阻隔包装等方面也得以 广泛应用。 由于纳米技术是最近10年来新兴的综合科学 技术, 其研究内容涉及现代高新技术各领域多方。 但纳米包装材料的特殊性能, 在节省材料资源, 满 足特殊包装功能要求等方面具有广阔的前景。
但是, 采用紫外线照射法, 由于长期的紫外线照射会导致肉类食品因自动氧化而
变质, 而且还会破坏食品中纤维素和芳香化合物, 降低食品营养价值。 通过采用添加纳米TiO2 制成的塑料薄膜来包装食品, 既可以防止紫外线对食
品的破坏, 又可以使食品保持防腐保鲜。
应用 防静电功能的纳米包装材料
包装材料的质量直接或间接影响产品的质量, 包装不仅对包装物的外观、防潮、 隔热、保温等性能有影响, 而且包装材料和包装容器在运输途中很容易因摩擦而产生
应用 纳米涂层包装材料
纳米材料由于其表面和结构的特殊性, 具有一般材料难以获得的优异性能, 借 助于传统的涂层技术, 添加纳米材料, 可获得纳米复合体系涂层, 使得传统涂层功能改
性。例如, 以纳米硅基陶瓷制成的特种不污染耐磨透明涂料, 涂在玻璃、塑料等物体
金属基复合材料(MMC)
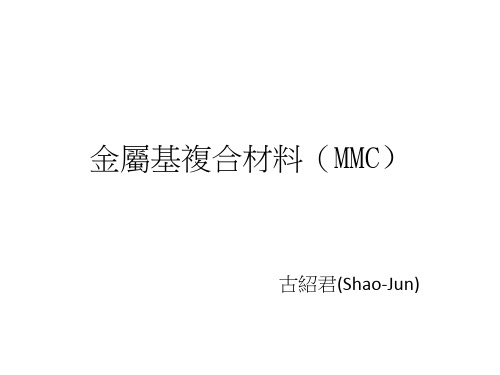
2. 液相複合法
– 該方法(特別是攪拌複合鑄造法)可以規模生產, 顆粒 金屬熔體 成本相對較低,但顆粒體積分數一般不超過 25%,成形過程與製品品質較難控制。
機械攪拌 3. 熔體噴霧與顆粒共噴射沉澱 擠壓鑄造法 壓力滲透法 混和澆鑄
– 壓力作用下通過噴嘴送入霧化器,在高速惰性 氣體射流的作用下,液態金屬被分散為細小的 液滴,形成“霧化錐”;同時通過一個或多個 胚料 複合材料錠材 噴嘴向“霧化錐”噴射入增強顆粒,使之與金 屬霧化液滴一齊在一基板(收集器)上沉積並 超塑 轧製 快速凝固形成顆粒增強金屬基複合材料。 擠出 鍛造 旋壓 機械加工
2. 藉外力強制克服結合不良之情形
1. 攪拌渦旋強迫顆粒納入鋁湯中 2. 採以加壓型式的鑄造方式成型
飛灰顆粒-鋁基(反應)
R. Q. Guo, P. K. Rohatgi, 1998, “Chemical Reactions between Aluminum and Fly Ash during Synthesis and Reheating of Al–Fly Ash Composite”, Metallurgical and Materials Transactions B, Vol. 29B, pp. 519-525.
鍵提供結合力。
• 物理結合
– 由兩相間原子中電子的交互作用的行為,即以凡得瓦力來結合。
• 擴散結合
– 某些複合體系的基體與增強體雖無界面反應,但可藉由原子間會 相互擴散作用。
• 機械結合
– 主要依靠補強材料粗糙表面的機械「錨固」力和基材的收縮力, 來抱緊補強材料產生摩擦力而結合。
一般情況,皆以化學結合為主,或者以兩種或兩種以上界面結合方式並存之的情況。
4. 靜電作用理論
金属复合材料的微观结构演化研究
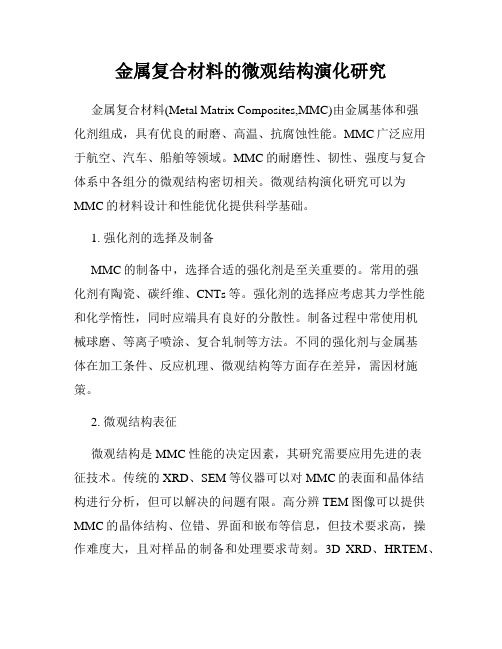
金属复合材料的微观结构演化研究金属复合材料(Metal Matrix Composites,MMC)由金属基体和强化剂组成,具有优良的耐磨、高温、抗腐蚀性能。
MMC广泛应用于航空、汽车、船舶等领域。
MMC的耐磨性、韧性、强度与复合体系中各组分的微观结构密切相关。
微观结构演化研究可以为MMC的材料设计和性能优化提供科学基础。
1. 强化剂的选择及制备MMC的制备中,选择合适的强化剂是至关重要的。
常用的强化剂有陶瓷、碳纤维、CNTs等。
强化剂的选择应考虑其力学性能和化学惰性,同时应端具有良好的分散性。
制备过程中常使用机械球磨、等离子喷涂、复合轧制等方法。
不同的强化剂与金属基体在加工条件、反应机理、微观结构等方面存在差异,需因材施策。
2. 微观结构表征微观结构是MMC性能的决定因素,其研究需要应用先进的表征技术。
传统的XRD、SEM等仪器可以对MMC的表面和晶体结构进行分析,但可以解决的问题有限。
高分辨TEM图像可以提供MMC的晶体结构、位错、界面和嵌布等信息,但技术要求高,操作难度大,且对样品的制备和处理要求苛刻。
3D XRD、HRTEM、STEM等仪器的应用可以获得MMC的本构行为和原位反应信息,可用于精确的材料建模和性能设计。
3. 微观结构演化机制强化剂与金属基体组成复合材料后,微观结构会因表面零件、位错和界面应力等因素发生变化,演变为一个新的能量最低的系统状态。
微观结构演化过程可以用分子动力学模拟和相场模型等方法进行数值模拟。
通过定量分析微观结构演化的机制,可以预测MMC的材料性能和寿命,并为MMC的制备和性能设计提供科学指导。
4. 微观结构对性能的影响MMC的力学性能与微观结构密切相关。
强化剂的尺寸、分布、形态等因素会影响MMC的力学性能。
界面和嵌布的存在可以增强MMC的强度和韧性,但同时也会削弱其疲劳性能。
在使用MMC进行加工和制造时,需对MMC微观结构的演化和性能的变化进行检测和修正。
总之,金属复合材料的微观结构演化研究是一个复杂而重要的研究领域。
金属基复合材料(Metalmatrixcomposites)
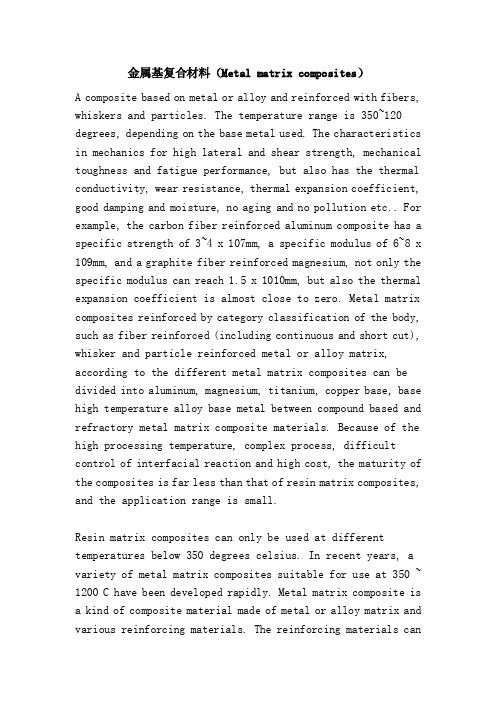
金属基复合材料(Metal matrix composites)A composite based on metal or alloy and reinforced with fibers, whiskers and particles. The temperature range is 350~120 degrees, depending on the base metal used. The characteristics in mechanics for high lateral and shear strength, mechanical toughness and fatigue performance, but also has the thermal conductivity, wear resistance, thermal expansion coefficient, good damping and moisture, no aging and no pollution etc.. For example, the carbon fiber reinforced aluminum composite has a specific strength of 3~4 x 107mm, a specific modulus of 6~8 x 109mm, and a graphite fiber reinforced magnesium, not only the specific modulus can reach 1.5 x 1010mm, but also the thermal expansion coefficient is almost close to zero. Metal matrix composites reinforced by category classification of the body, such as fiber reinforced (including continuous and short cut), whisker and particle reinforced metal or alloy matrix, according to the different metal matrix composites can be divided into aluminum, magnesium, titanium, copper base, base high temperature alloy base metal between compound based and refractory metal matrix composite materials. Because of the high processing temperature, complex process, difficult control of interfacial reaction and high cost, the maturity of the composites is far less than that of resin matrix composites, and the application range is small.Resin matrix composites can only be used at different temperatures below 350 degrees celsius. In recent years, a variety of metal matrix composites suitable for use at 350 ~ 1200 C have been developed rapidly. Metal matrix composite is a kind of composite material made of metal or alloy matrix and various reinforcing materials. The reinforcing materials canbe fibrous, granular and whisker like silicon carbide, boron, alumina and carbon fiber. In addition to metal aluminum and magnesium, metal matrix also develops non-ferrous metals such as titanium, copper, zinc, lead, beryllium, super alloy and intermetallic compound, and ferrous metal as metal matrix. In addition to the high strength and high modulus of resin matrix composites, metal matrix composites can resist high temperature, non burning, non moisture absorption, good thermal conductivity and radiation resistance. High temperature materials for impressiveness aerospace, surface material can be used as aircraft turbine engine and rocket engine hot and supersonic aircraft. At present, the development and perfection of metal matrix composites is the fastest development of SiC particle aluminum alloy. The proportion of the metal matrix composite is only 1/3 of the steel, 2/3 of the titanium alloy, similar to that of the aluminum alloy. Its strength is better than that of medium carbon steel, similar to titanium alloy but slightly higher than that of aluminum alloy. The wear resistance of the alloy is better than that of the titanium alloy and aluminum alloy. It has been used in automobile industry and machinery industry in small quantities. In 5~15 years of commercial applications are automobile parts, brake piston, connecting rod, robot parts, computer parts, sports equipment, etc.. The main problem of metal matrix composites is that the manufacturing process of metal composite is complex and expensive, and it can not be used in industrial scale production.Composite plate: refers to a metal plate on the cover of another metal plate, in order to achieve the effect of not reducing the use (corrosion resistance, mechanical strength, etc.) underthe premise of saving resources and reducing costs. Composite methods usually include explosive bonding, explosive rolling and rolling bonding. Composite materials can be divided into composite plate, composite pipe, composite rod and so on. Mainly used in anti-corrosion, pressure vessel manufacturing, electric power construction, petrochemical, pharmaceutical, light industry, automobile and other industries.Stainless steel clad plate is a composite plate steel made of carbon steel base and stainless steel cladding. Its main feature is the formation of a strong metallurgical bond between carbon steel and stainless steel. It can be processed by hot pressing, cold bending, cutting, welding and so on, and has good technological properties. The base material of stainless steel clad plate can be made of ordinary carbon steel and special steel, such as Q235B, 16MnR, 20R, etc.. Cladding materials can be made of stainless steels of various grades such as 304, 316L, 1Cr13 and duplex stainless steel. Material and thickness can be freely assembled to meet the needs of different users. Stainless steel clad plate has been widely used in petroleum, chemical industry, salt industry, water conservancy and electric power industry. As a resource saving product, the stainless steel clad plate can reduce the consumption of precious metal,Greatly reduce the project cost. Realize the perfect combination of low cost and high performance, and have good social benefits.Stainless steel clad plate is how to produce it? There are two methods for industrial production of stainless steel clad plate,explosive cladding and hot rolling. The production process of the explosive clad plate is that the stainless steel plate is overlapped on the carbon steel substrate, and the stainless steel plate and the carbon steel plate are separated by a cushion. The stainless steel plate is covered with explosive and explosive energy, which makes the stainless steel plate impact on the carbon steel substrate at high speed and produce high temperature and high pressure to realize the solid phase welding of the interface between the two materials. Ideally, the shear strength of the interface can reach 400 MPa per square millimeter. The hot rolling composite plate process is made of carbon steel substrate and stainless steel plate in the state of physical purity and rolled under high vacuum condition. In the process of rolling, two kinds of metal diffusion achieve complete metallurgical bonding. Of course, in order to improve the wettability of composite interface and improve the bonding strength, a series of technical measures should be taken in the physical and chemical treatment of the interface. The above two kinds of composite plate manufacturing methods are carried out GB/T-8165-1977 national standards. This standard is not equivalent to or higher than the same JISG36011990 Japanese standard, Japanese standard main technical indicators.How about the process characteristics of the blast clad and hot rolled composite plate? First, the explosive composite features: first, because the explosion is cold processing, so it can produce in addition to stainless steel composite plate, many kinds of metal composite plate, such as titanium, copper, aluminum and so on. Two, explosive composite can produce stainless steel composite plate, the total thickness can reach hundreds of millimeters thick stainless steel composite plate,such as some large base and tube plate, etc.. But it is not suitable for producing thinner composite steel plate with total thickness less than 10 mm. Three explosive composite use of explosive energy production, the environment will cause vibration, noise and soot pollution. However, the equipment investment is small, and there are hundreds of domestic explosive production plants. Due to the restrictions of weather and other process conditions, the explosive composite production efficiency is low. Besides, the characteristics of hot rolling compound technology are as follows: 1. Large medium plate mill and hot strip mill are used, so the production efficiency is high and the supply speed is fast. The product is large in size and free in thickness. Stainless steel coating thickness above 0.5mm can be produced. But the investment is large, so the manufacturers are less. Two, due to the limitation of steel rolling compression ratio, hot rolling production can not produce composite steel plate with thickness of more than 50mm, and it is not convenient to produce various small, round and other special shaped composite plate. Advantages of hot rolled composite plates of 6, 8 and 10 mm for thin gauge composite plates. Under the condition of hot continuous rolling, the composite bending plate can be produced, the production cost can be reduced, and more users' demands can be met. Three, in the current technical conditions, hot rolling process can not directly produce titanium, copper, aluminum and other non-ferrous metal composite plate. To sum up, the two different production processes have their own characteristics, exist and develop at the same time, to meet the diverse needs of different users. Explosive rolling is the combination of the two processes mentioned above, no more details.Stainless steel composite plate has the characteristics of various carbon steel and stainless steel, with its excellent performance price ratio for users, and has broad market prospects. But interestingly, from the beginning of 50s, after more than half a century of ups and downs of the development process, there are still many people do not know it. More people haven't used it. It should be said that the stainless steel composite plate market is far from mature, and still in the process of development. The exploration and efforts of scientific and technical workers to build a resource conserving society will never cease.Composite materials (Composite) is a kind of material which is made of one material as the matrix (Matrix) and the other as the reinforcing body (reinforcement). Various materials can complement each other in performance and produce synergistic effect, so that the comprehensive properties of composite materials are better than those of the original materials and meet various requirements. The matrix materials of composite materials are divided into two categories: metal and nonmetal. Aluminum, magnesium, copper, titanium and their alloys are commonly used in metal matrix.The non-metallic matrix mainly includes synthetic resin, rubber, ceramic, graphite, carbon and so on. The reinforcing materials mainly include glass fiber, carbon fiber, boron fiber, aramid fiber, silicon carbide fiber, asbestos fiber, whisker, wire and hard fine particles, etc..The history of composite materials can be traced back to ancient times. Since ancient times, the use of straw reinforced clayand reinforced concrete has been used for two centuries. In 1940s, due to the needs of the aviation industry, glass fiber reinforced plastics (commonly known as glass fiber reinforced plastics) were developed, and the name of composite material emerged. Since 50s, high strength and high modulus fibers such as carbon fiber, graphite fiber and boron fiber have been developed. Aramid fiber and silicon carbide fiber appeared in 70s. These high strength and high modulus fibers can be compounded with non metallic matrix such as synthetic resin, carbon, graphite, ceramic, rubber and other metal matrix, such as aluminum, magnesium, titanium, and so on, and form composite materials with different characteristics.The forming methods of composites are different according to the matrix materials. More molding method of resin matrix composites, a hand lay up molding injection molding, filament winding, molding, pultrusion, autoclave molding, molding, transfer molding, diaphragm reaction injection molding, molding, stamping and other soft expansion. The forming methods of metal matrix composites are divided into solid phase forming method and liquid phase forming method. The former is formed by applying pressure at lower than the melting point temperature of the matrix, including diffusion welding, powder metallurgy, hot rolling, hot drawing, hot isostatic pressing and explosive welding. The latter is the substrate melting, filling the reinforcement materials, including traditional casting, vacuum casting, vacuum counter pressure casting, extrusion casting and spray casting and forming method of ceramic matrix composite materials are mainly solid phase sintering, chemical vapor infiltration molding, molding and other chemical vapor deposition.The main application fields of composite materials are:Aerospace field. The composite material has good thermal stability, high specific strength and stiffness, can be used in the manufacture of aircraft wing and fuselage, satellite antenna and its supporting structure, solar wing and shell, large rocket engine shell, shell, space shuttle structure etc..Automobile industry. The composite material has the special characteristics of vibration damping, vibration damping and noise reduction, anti fatigue performance is good, easy to repair after injury, to facilitate the overall shape, it can be used in the manufacture of automobile, mechanical components, transmission shaft, engine frame and internal components.Chemical, textile and mechanical manufacturing. The material with good corrosion resistance of carbon fiber and resin matrix can be used for manufacturing chemical equipment, textile machines, paper machines, copiers, high-speed machine tools, precision instruments, etc..Medical field. Carbon fiber composite has excellent mechanical properties and does not absorb X ray characteristics. It can be used in the manufacture of medical X ray machine and orthopedic stent. Carbon fiber composites also have biological compatibility and blood compatibility, and have good stability in biological environment, and they are also used as biomedical materials. In addition, composites are also used to make sports devices and use as building materials.Metal matrix compositesMetal and ceramic composite material, belongs to particle reinforced composite material, also known as metal ceramicCemented carbideProperties and applications: high hardness, high wear resistance, high red hardness, high thermal stability and oxidation resistanceIt is suitable for all kinds of high speed cutting tools, wear parts at various high temperature, such as hot drawing die, etc.1. tungsten cobalt carbide - pressed and sintered from cobalt Co and tungsten carbide WCGrade: the content of YG+Co, such as YG3, YG6, YG8.Co, the higher the content, the better the toughnessPerformance characteristics - high hardness, high wear resistance, high red hardness, good toughnessPurpose: to make cutting tools for brittle materials such as cast iron, non-ferrous metals and non-metallic materials, such as: YG8 tools are suitable for rough cast iron, YG3 is suitable for finishing cast iron, YG6 is suitable for semi finishing cast iron2. tungsten titanium cobalt carbide - pressed and sintered from cobalt Co and tungsten carbide WC+TiCBrand: the percentage content of YT+TiC, such as YT5, YT15 and YYT30.TiC, is higher,The toughness is betterPerformance characteristics hardness and red hardness are higher than class YG, toughness, strength is slightly lower than class YGUse - cutting tools for making all kinds of steel, such as: YT5 tools suitable for rough machining steel, YT15 suitable for finishing steel, YT suitable for semi finishing steel3. tungsten titanium tantalum cobalt hard alloy - pressed and sintered from cobalt Co+WC+TiC+TaCBrands: YW, such as YW1 and YW2Performance characteristics - both YG, YT advantages, also known as general-purpose cemented carbide and universal cemented carbideApplication: cutting tools for refractory materials such as heat resistant steel and alloy。
碳纤维增强金属基复合材料翻译

Metal-Matrix Composites forSpace Applications------Suraj Rawal应用于航空航天的金属基复合材料航空航天研究开始初期,有机复合材料和金属基复合材料都应经通过特定的高刚度和接近为零的热膨胀系数开发为空间应用。
过去的30年里,在有机复合材料模型中,石墨环氧化合物已被应用于空间架元素、巴士板、天线、波参考线和抛物线反射体。
金属基复合材料具有耐高温、高导热能力、低的热膨胀系数和特定的高刚度和强度。
二十世纪八十年代末,这些潜在的优势让人们对金属基复合材料应用于主要空间系统抱有积极乐观的态度。
这篇文章的目的在于详细描述金属基复合材料应用于航空航天的历史、地位和前景。
简介空间中极端环境给研究材料的科学家提出了机遇和挑战。
在近地球轨道,航天器会遭遇到一些太空中自然产生的现象。
例如:真空、热辐射、氧原子、电离辐射和血浆。
还伴随有例如微流星体和人为产生的碎片等因素。
例如:国际空间站。
在其30年的研究期间,当其运行至或运行出地球的影子时,将经历从125℃到-125℃的175.000次热循环。
重返地球和火星的飞行器可能会遭遇到超过1500℃的高温情况。
因此,主体飞行器的任务要求轻量级的空间结构、高精度指向、范围内的动态稳定性和热扰动。
复合材料,其特定的高刚度和低的热膨胀系数为产生轻量和范围内相对稳定的结构提供了必要的特性。
因此说,有机基质和金属基复合材料已为空间应用开发。
尽管有成功的金属基复合材料成品如连续纤维增强硼铝、石墨铝和石墨镁。
出于对制造业的缓解和检查、扩大和成本问题的考虑,这一技术投入受到了限制。
有机复合材料继续成功地解决系统级问题。
这些问题是关于在热循环、辐射暴露和电磁干扰屏蔽中产生的裂纹。
金属基复合材料天生就抵触这些因素。
同时,非连续增强金属基复合材料如碳化硅系增强颗粒增强铝颗粒和铝玻璃钢复合材料被开发成为既为航空航天应用(例如电子包装),又为商业应用,具有成本效益。
考虑非均匀结构效应的金属材料剪切带

一、提名项目:考虑非均匀结构效应的金属材料剪切带二、提名意见:该项目以颗粒增强金属基复合材料和非晶合金为模型系统,突破经典的热塑剪切带理论框架,发展了位错机制依赖的应变梯度本构,揭示了蕴含的非均匀结构通过应变梯度效应对热塑剪切带形成具有强烈驱动作用;建立了包含多过程耦合与时空多尺度的剪切带新理论,澄清了非晶合金剪切带形成机制长期广泛的国际争议,得到了剪切带失稳判据、协同演化、特征厚度以及诱致断裂机理等一系列原创性成果。
该项目8篇代表性论文共被《Nature Materials》《Physical Review Letters〉、《Progress in Materials Science等SCI重要刊物他人引用393次,引用者包括国内外科学院或工程院院士、权威杂志主编、领域知名学者等。
项目研究成果系统揭示了材料内禀非均匀结构效应如何影响甚至颠覆热塑剪切带的传统认知,显著推动了剪切带理论的发展,在国际上产生了重要的学术影响。
提名该项目为国家自然科学二等奖。
三、项目简介剪切带是一类广泛存在的塑性变形局部化失稳现象。
本征上,具有特征厚度的剪切带是一种远离平衡态的动态耗散结构,其涌现与演化是材料内部多种速率依赖耗散过程高度非线性耦合控制的时空多尺度问题。
传统金属材料剪切带经百余年研究,逐渐形成了以热软化为主控机制的热塑剪切带理论,并获得了广泛的应用。
随着人们对高性能材料的不懈追求,众多内蕴微纳尺度非均匀结构的新型金属材料不断发展,其中代表性的有微米尺度颗粒增强的金属基复合材料和纳米尺度结构非均匀的非晶合金。
由于不考虑材料结构效应,经典热塑剪切带理论在描述这些新型金属材料的剪切带行为时,遇到了前所未有的挑战。
为此,该项目团队以颗粒增强金属基复合材料和非晶合金为模型材料,研究了材料内禀的非均匀结构效应如何影响甚至颠覆热塑剪切带的传统认知,显著推动了剪切带理论的发展,形成了具有鲜明特色的系统性的原创研究成果。
金属基复合材料概述
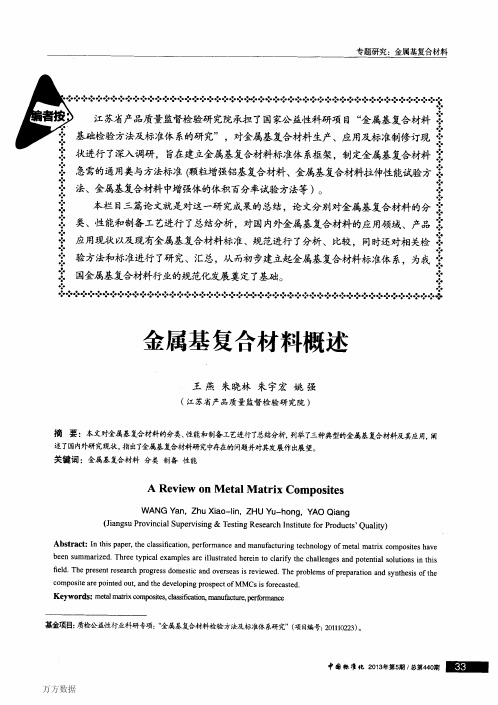
Si、Al—cu和Al—Fe等体系。增强体主要有Sic颗粒、 A1203颗粒、Bc4颗粒、TiC颗粒等。其中采用SiC颗 粒增强的铝基复合材料具有性能高、价格低、密度 小等优点,是目前应用最广泛的铝基复合材料,在 国外已经实现规模化生产。美国DwA公司用SiC颗 粒增强6092铝基复合材料代替铝合金制造F—16战 斗机的垂直尾翼,提高寿命17倍。Eurocopter公司已
按照基体和增强体的不同,金属基复合材料可 按照如下分类。 按基体材料分为:黑色金属基(钢、铁)、有色 金属基(铝基、锌基、镁基、铜基、钛基、镍基)、耐 热金属基、金属间化合物基复合材料等。目前铝基、 镁基、钛基复合材料发展较为成熟,已逐步应用于 航空航天、电子、汽车等工业领域。 按增强体分为:连续纤维增强金属基复合材料; 非连续增强金属基复合材料(颗粒、短纤维、晶须增 强金属基复合材料);混杂增强金属复合材料、层板金 属基复合材料;自生增强金属基复合材料(包括反应、 定向凝固、大变形等途径自生颗粒、晶须、纤维状增 强体)等。其中,自生复合材料的增强相在热力学上 是稳定的,界面结合强度高,而且增强体的尺寸和体 积分数可以通过工艺参数控制,是目前研究的热点。
Hale Waihona Puke 在金属基体中加入适量的高强度、高模量、低 密度的纤维、晶须及颗粒等增强体,可显著提高 复合材料的比强度和比模量。如:碳纤维密度只有
1.85咖m3,最高强度可达7,oooMPa,比铝合金强度
高出10倍以上。石墨纤维的最高模量可达900GPa, 比普通钢材要高4倍以上,而B纤维、Sic颗粒的密度
2金属基复合材料的分类
4典型的金属基复合材料
4.1铝基复合材料 铝及铝合金具有密度低、塑韧性好、导热导电 性较好等优点,但其熔点低、耐磨性差的缺陷限制 了其在更广范围和更高领域的应用。而铝基复合材 料通过增强相的加入使之具有高比强度、高比刚度、 耐磨性好、尺寸稳定性好以及易于加工等一系列优 良特性,在航空航天、汽车、电子等工业领域具有十 分广泛的应用前景。 铝基复合材料常用的基体有Al—M
复合材料英语词汇
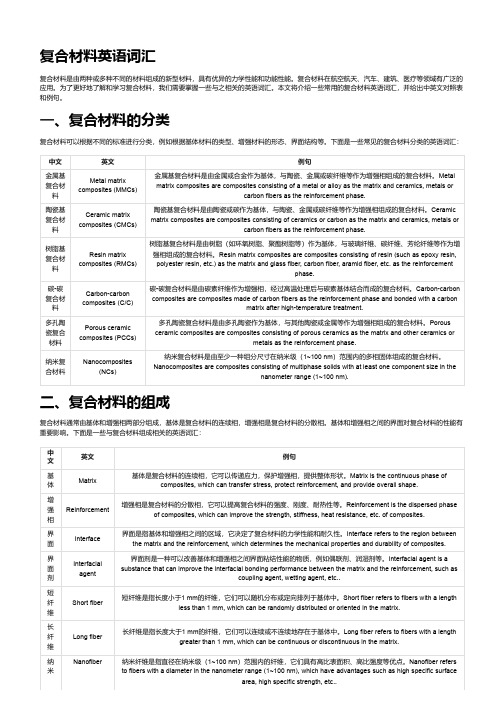
复合材料英语词汇复合材料是由两种或多种不同的材料组成的新型材料,具有优异的力学性能和功能性能。
复合材料在航空航天、汽车、建筑、医疗等领域有广泛的应用。
为了更好地了解和学习复合材料,我们需要掌握一些与之相关的英语词汇。
本文将介绍一些常用的复合材料英语词汇,并给出中英文对照表和例句。
一、复合材料的分类复合材料可以根据不同的标准进行分类,例如根据基体材料的类型、增强材料的形态、界面结构等。
下面是一些常见的复合材料分类的英语词汇:中文英文例句金属基复合材料Metal matrixcomposites (MMCs)金属基复合材料是由金属或合金作为基体,与陶瓷、金属或碳纤维等作为增强相组成的复合材料。
Metalmatrix composites are composites consisting of a metal or alloy as the matrix and ceramics, metals orcarbon fibers as the reinforcement phase.陶瓷基复合材料Ceramic matrixcomposites (CMCs)陶瓷基复合材料是由陶瓷或碳作为基体,与陶瓷、金属或碳纤维等作为增强相组成的复合材料。
Ceramicmatrix composites are composites consisting of ceramics or carbon as the matrix and ceramics, metals orcarbon fibers as the reinforcement phase.树脂基复合材料Resin matrixcomposites (RMCs)树脂基复合材料是由树脂(如环氧树脂、聚酯树脂等)作为基体,与玻璃纤维、碳纤维、芳纶纤维等作为增强相组成的复合材料。
Resin matrix composites are composites consisting of resin (such as epoxy resin,polyester resin, etc.) as the matrix and glass fiber, carbon fiber, aramid fiber, etc. as the reinforcementphase.碳-碳复合材料Carbon-carboncomposites (C/C)碳-碳复合材料是由碳素纤维作为增强相,经过高温处理后与碳素基体结合而成的复合材料。
金属基复合材料原位复合工艺
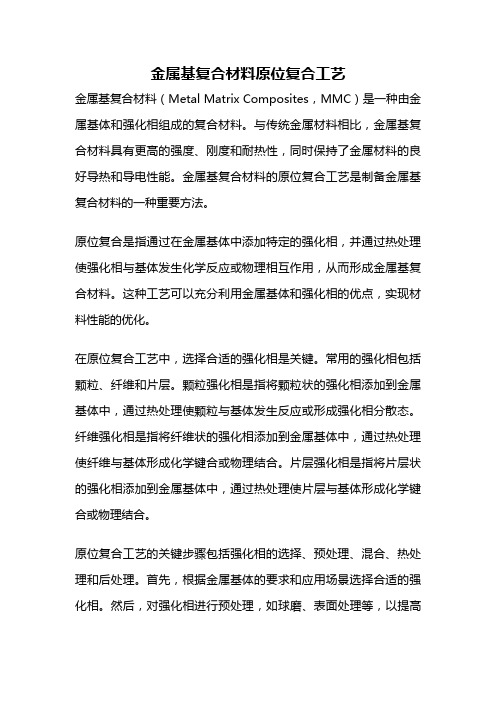
金属基复合材料原位复合工艺金属基复合材料(Metal Matrix Composites,MMC)是一种由金属基体和强化相组成的复合材料。
与传统金属材料相比,金属基复合材料具有更高的强度、刚度和耐热性,同时保持了金属材料的良好导热和导电性能。
金属基复合材料的原位复合工艺是制备金属基复合材料的一种重要方法。
原位复合是指通过在金属基体中添加特定的强化相,并通过热处理使强化相与基体发生化学反应或物理相互作用,从而形成金属基复合材料。
这种工艺可以充分利用金属基体和强化相的优点,实现材料性能的优化。
在原位复合工艺中,选择合适的强化相是关键。
常用的强化相包括颗粒、纤维和片层。
颗粒强化相是指将颗粒状的强化相添加到金属基体中,通过热处理使颗粒与基体发生反应或形成强化相分散态。
纤维强化相是指将纤维状的强化相添加到金属基体中,通过热处理使纤维与基体形成化学键合或物理结合。
片层强化相是指将片层状的强化相添加到金属基体中,通过热处理使片层与基体形成化学键合或物理结合。
原位复合工艺的关键步骤包括强化相的选择、预处理、混合、热处理和后处理。
首先,根据金属基体的要求和应用场景选择合适的强化相。
然后,对强化相进行预处理,如球磨、表面处理等,以提高其与金属基体的相容性。
接下来,将预处理后的强化相与金属基体进行混合,并形成均匀分散的体系。
混合过程中可采用机械混合、溶液共沉淀等方法。
混合后的体系经过热处理,使强化相与金属基体发生反应或结合。
最后,对原位复合材料进行后处理,如热处理、机械加工等,以获得所需的性能和形态。
金属基复合材料的原位复合工艺具有以下优点:首先,可以在金属基体中实现强化相的均匀分散,避免了强化相的聚集和团聚现象。
其次,原位复合工艺可以改善金属基体的力学性能,提高材料的强度、刚度和耐热性。
同时,金属基复合材料还保持了金属材料的导热和导电性能,具有良好的综合性能。
此外,原位复合工艺还可以实现对金属基体材料的改性,满足特定应用的需求。
金属基复合材料简介
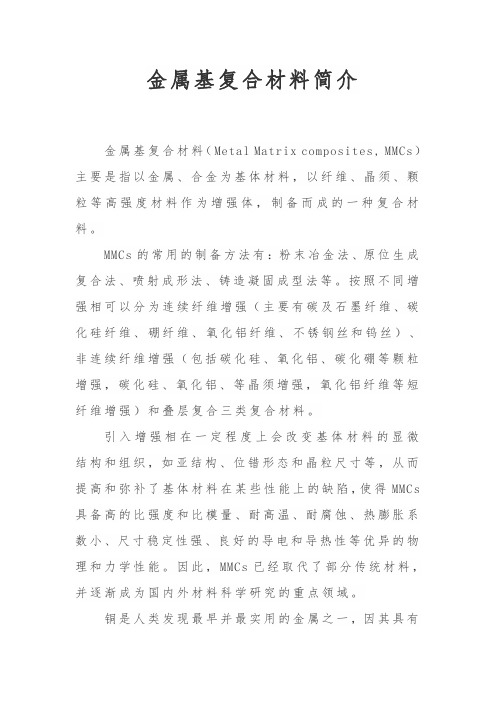
金属基复合材料简介金属基复合材料(Metal Matrix composites,MMCs)主要是指以金属、合金为基体材料,以纤维、晶须、颗粒等高强度材料作为增强体,制备而成的一种复合材料。
MMCs的常用的制备方法有:粉末冶金法、原位生成复合法、喷射成形法、铸造凝固成型法等。
按照不同增强相可以分为连续纤维增强(主要有碳及石墨纤维、碳化硅纤维、硼纤维、氧化铝纤维、不锈钢丝和钨丝)、非连续纤维增强(包括碳化硅、氧化铝、碳化硼等颗粒增强,碳化硅、氧化铝、等晶须增强,氧化铝纤维等短纤维增强)和叠层复合三类复合材料。
引入增强相在一定程度上会改变基体材料的显微结构和组织,如亚结构、位错形态和晶粒尺寸等,从而提高和弥补了基体材料在某些性能上的缺陷,使得MMCs 具备高的比强度和比模量、耐高温、耐腐蚀、热膨胀系数小、尺寸稳定性强、良好的导电和导热性等优异的物理和力学性能。
因此,MMCs已经取代了部分传统材料,并逐渐成为国内外材料科学研究的重点领域。
铜是人类发现最早并最实用的金属之一,因其具有优良的延展性,仅次于银的电导率,仅次于金银的热导率,一直以来备受重视。
但是,铜的力学性能(耐磨性、硬度、强度、抗蠕变性等)较差,限制了铜在工业和军事等领域的应用。
在众多MMCs中,铜基复合材料以其优异的导电、导热性能、耐腐蚀性以及良好的加工性而被广泛关注。
从二十世纪六十年代开始,铜基复合材料的相关研究逐渐开展,许多科学家在铜基体中加入了不同的增强体,发现该复合材料既保持了铜的优点,又弥补了铜力学性能上的不足。
时至今日,铜基复合材料的研究已经持续了几十年,形成了以颗粒增强铜基复合材料、纤维增强铜基复合材料、晶须增强铜基复合材料三大类别。
1、颗粒增强铜基复合材料颗粒增强铜基复合材料目的是将性能优异的颗粒均匀分散于铜基体,提高铜基复合材料的综合性能。
颗粒增强相产生的钉扎作用能够极大的阻碍位错的运动从而增强复合材料的强度,使铜基复合材料的力学性能、耐磨以及高温性能大幅提高。
金属基复合材料 书籍
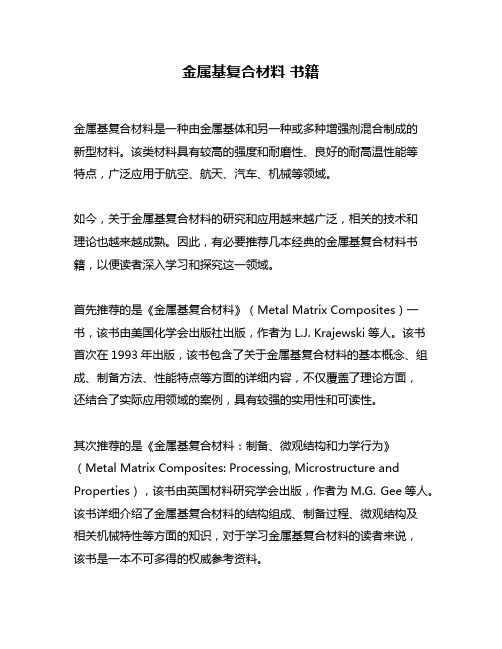
金属基复合材料书籍金属基复合材料是一种由金属基体和另一种或多种增强剂混合制成的新型材料。
该类材料具有较高的强度和耐磨性、良好的耐高温性能等特点,广泛应用于航空、航天、汽车、机械等领域。
如今,关于金属基复合材料的研究和应用越来越广泛,相关的技术和理论也越来越成熟。
因此,有必要推荐几本经典的金属基复合材料书籍,以便读者深入学习和探究这一领域。
首先推荐的是《金属基复合材料》(Metal Matrix Composites)一书,该书由美国化学会出版社出版,作者为L.J. Krajewski等人。
该书首次在1993年出版,该书包含了关于金属基复合材料的基本概念、组成、制备方法、性能特点等方面的详细内容,不仅覆盖了理论方面,还结合了实际应用领域的案例,具有较强的实用性和可读性。
其次推荐的是《金属基复合材料:制备、微观结构和力学行为》(Metal Matrix Composites: Processing, Microstructure and Properties),该书由英国材料研究学会出版,作者为M.G. Gee等人。
该书详细介绍了金属基复合材料的结构组成、制备过程、微观结构及相关机械特性等方面的知识,对于学习金属基复合材料的读者来说,该书是一本不可多得的权威参考资料。
最后推荐的是《金属基复合材料:材料科学、制备技术和应用》(Metal Matrix Composites: Materials Science, Manufacturing Techniques, and Applications),该书由CRC出版,作者为K. U. Kainer等人。
该书系统地介绍了金属基复合材料的制备工艺和应用前景,特别是替代传统材料、改进机械性能和提高可靠性等方面的优势,给读者提供了全面的知识体系以及未来的发展方向。
以上是我对金属基复合材料书籍的推荐,希望对学习金属基复合材料的读者有所帮助。
对于初学者来说,选择一本适合自己的书籍,系统地学习金属基复合材料的相关知识是很有必要的。
金属基复合材料在航空航天领域的应用

收稿日期:2007-05-11作者简介:吕一中(1961-),男,安徽理工大学机械制造专业毕业,中国矿业大学(北京)复合材料专业在读博士,研究员。
金属基复合材料在航空航天领域的应用吕一中1,3 崔岩2 曲敬信1(1.中国矿业大学(北京),北京100083;2.北京航空材料研究院,北京100095;3.北京工业职业技术学院,北京100042)摘 要:金属基复合材料已成为发达国家争夺高技术优势的热点之一,并作为先进复合材料将逐步取代部分传统的金属材料。
介绍了铝基复合材料在导弹、航天领域中的应用以及金属基复合材料在航空领域的其它应用。
关键词:金属基复合材料;导弹;航天;应用中图分类号:TF 121 文献标识码:B 文章编号:1671-6558(2007)03-01-04Application of Metal Matrix Composites to AerospaceL üY izhong 1,3 Cui Yan 2 Qu Jingxin 1(1.China University of Mining &Technology (Beijing ),Beijing 100083,China ;2.Beijing Institute of Aeronautical Materials ,Beijing 100095,China ;3.Beijing Polytechnic College ,Beijing 100042,China )Abstract :As one of the developed countries contention hotspots for the dominant position in high 2techs ,metal matrix composites will gradually substitute partial traditional metal materials as Advanced Composite.The appli 2cation of aluminium 2matrix composite to both missile and aerospace field and metal 2based composites to aerospace field is introduced.K ey w ords :metal matrix composites ;missile ;aerospace ;application 复合材料是二十世纪材料科学领域的重大突破之一,其中金属基复合材料是从20世纪60年代初发展起来的,具有高比强度、高比模量、耐磨、耐热、导电、导热、不吸潮、抗辐射、低热膨胀系数等优良性能,目前已成为发达国家争夺高技术优势的热点之一,并作为先进复合材料将逐步取代部分传统的金属材料而应用于航天航空、汽车工业、电子工业等领域。
- 1、下载文档前请自行甄别文档内容的完整性,平台不提供额外的编辑、内容补充、找答案等附加服务。
- 2、"仅部分预览"的文档,不可在线预览部分如存在完整性等问题,可反馈申请退款(可完整预览的文档不适用该条件!)。
- 3、如文档侵犯您的权益,请联系客服反馈,我们会尽快为您处理(人工客服工作时间:9:00-18:30)。
From the onset of the space era, both or-ganic-matrix and metal-matrix composites (MMCs), with high specific stiffness and near-zero coefficient of thermal expansion (CTE), have been developed for space appli-cations. Of the organic-matrix composites,graphite/epoxy (Gr/Ep) has been used in space for truss elements, bus panels, anten-nas, wave guides, and parabolic reflectors in the past 30 years. MMCs possess high-tem-perature capability, high thermal conductiv-ity, low CTE, and high specific stiffness and strength. Those potential benefits generated optimism for MMCs for critical space sys-tem applications in the late 1980s.1,2 The purpose of this article is to detail the history,status, and opportunities of MMCs for space applications.INTRODUCTIONThe extreme environment in space presents both a challenge and opportu-nity for material scientists. In the near-earth orbit, typical spacecraft encounter naturally occurring phenomena such as vacuum, thermal radiation, atomic oxy-gen, ionizing radiation, and plasma,along with factors such as micrometeor-Metal-Matrix Composites for Space ApplicationsSuraj RawalTable I. Material Properties of Unidrectional Metal-Matrix Composites forSpace Applications P100/6061 Al P100/AZ91C Mg Boron/AlProperties (0°) (0°)(0°)Volume Percent Reinforcement 42.24350Density, ρ (gm/cm 3) 2.5 1.97 2.7Poisson Ratio νxy 0.2950.30.23Specific Heat C p (J/kg-K)812795801LongitudinalYoung’s Modulus (x) (GPa)342.5323.8235Ultimate Tensile Strength (x) (MPa)905710.01100Thermal Conductivity K x (W/m-K)320.0189—CTE x (10–6/K*)–0.490.54 5.8TransverseYoung’s Modulus (y) (GPa)35.420.7138Ultimate Tensile Strength (y) (MPa)25.022.0110Thermal Conductivity K y (W/m-K)72.032.0—* Slope of a line joining extreme points (at –100°C and +100°C) of the thermal strain curve (first cycle).Figure 1. Mid-fuselage structure of Space Shuttle Orbiter showing boron-aluminum tubes. (Photo courtesy of U.S. Air Force/NASA).oids and human-made debris. For ex-ample, the International Space Station,during its 30-year life, will undergo about 175,000 thermal cycles from +125°C to –125°C as it moves in and out of the Earth’s shadow. Re-entry vehicles for Earth and Mars missions may encounter temperatures that exceed 1,500°C. Criti-cal spacecraft missions, therefore, de-mand lightweight space structures with high pointing accuracy and dimensional stability in the presence of dynamic andthermal disturbances. Composite mate-rials, with their high specific stiffness and low coefficient of thermal expan-sion (CTE), provide the necessary char-acteristics to produce lightweight and dimensionally stable structures. There-fore, both organic-matrix and metal-matrix composites (MMCs) have been developed for space applications.Despite the successful production of MMCs such as continuous-fiber rein-forced boron/aluminum (B/Al), graph-ite/aluminum (Gr/Al), and graphite/magnesium (Gr/Mg),3–7 the technology insertion was limited by the concerns related to ease of manufacturing and inspection, scale-up, and cost. Organic-matrix composites continued to success-fully address the system-level concerns related to microcracking during thermal cycling and radiation exposure, and elec-tromagnetic interference (EMI) shield-ing; MMCs are inherently resistant to those factors. Concurrently, discontinu-ously reinforced MMCs such as silicon-carbide particulate (p) reinforced alumi-num (SiC p /Al) and Gr p /Al composites were developed cost effectively both for aerospace applications (e.g., electronic packaging) and commercial applications.This paper describes the benefits, draw-backs, potential for the various MMCs in the U.S. space program.HISTORICAL PERSPECTIVE Historically, MMCs, such as steel-wire reinforced copper, were among the first continuous-fiber reinforced composites studied as a model system. Initial workEditor’s Note: A hypertext-enhanced version of this article can be found at /pubs/journals/JOM/0104/Rawal_0104.html.Table II. Material Properties of Discontinuously ReinforcedAluminum-Matrix CompositesGraphite AlProperty GA 7-230Al6092/SiC/17.5p Al/SiC/63pDensity, ρ (gm/cm3) 2.45 2.8 3.01Young’s Modulus (GPa)88.7100220Compressive Yield Strength (MPa)109.6406.5Tensile Ultimate Strength (MPa)76.8461.6253Compressive Ultimate Strength (MPa)202.6—CTE (x-y) (10-6/K) 6.5-9.516.47.9Thermal Conductivity (W/m-K) (x-y)190165175(z)150170Electrical Resistivity (µ-ohm-cm) 6.89—Figure 2. The P100/6061 Al high-gain antenna wave guides/boom for the Hubble Space Telescope (HST) shown (a) beforeintegration in the HST, and (b) on the HST as it is deployed inlow-earth orbit from the space shuttle orbiter.bain late 1960s was stimulated by the high-performance needs of the aerospace in-dustry. In these development efforts,performance, not cost, was the primary driver. Boron filament, the first high-strength, high-modulus reinforcement,was developed both for metal- and or-ganic-matrix composites. Because of the fiber-strength degradation and poor wettability in molten-aluminum alloys,the early carbon fibers could only be properly reinforced in organic-matrix composites. Therefore, the development of MMCs was primarily directed toward diffusion-bonding processing. At the same time, optimum (air stable) surface coatings were developed for boron and graphite fibers to facilitate wetting and inhibit reaction with aluminum or mag-nesium alloys during processing.METAL-MATRIX COMPOSITEPROCESSING Three processing methods have been primarily used to develop MMCs: high-pressure diffusion bonding, casting, and powder-metallurgy techniques. More specifically, the diffusion-bonding and casting methods have been used for con-tinuous-fiber reinforced MMCs. Discon-tinuously reinforced MMCs have been produced by powder metallurgy and pressure-assist casting processes. MMCs such as B/Al, Gr/Al, Gr/Mg, and Gr/Cu have been manufactured by diffu-sion bonding for prototype spacecraft components such as tubes, plates, and panels.PropertiesTable I lists the typical properties of a few continuous-fiber reinforced MMCs.Generally, measured properties of as-fabricated MMCs are consistent with the analytically predicted properties of each composite. The primary advantage of MMCs over counterpart organic-matrix composites is the maximum operating temperature. For example, B/Al offers useful mechanical properties up to 510°C,whereas an equivalent B/Ep composite is limited to about 190°C. In addition,MMCs such as Gr/Al, Gr/Mg, and Gr/Cu exhibit higher thermal conductivity because of the significant contribution from the metallic matrix.Table II lists the properties of discon-tinuously reinforced aluminum (DRA)composites for spacecraft and commer-cial applications. DRA is an isotropic MMC with specific mechanical proper-ties superior to conventional aerospace materials. For example, DWA Alumi-num Composites has produced MMCs using 6092 and 2009 matrix alloys for the best combination of strength, ductility,and fracture toughness, and 6063 matrix alloy to obtain high thermal conductiv-ity. Similarly, Metal Matrix Cast Com-posite (MMCC) Inc. has produced graph-ite particulate-reinforced aluminum composites for the optimum combina-tion of high specific thermal conductiv-ity and CTE.APPLICATIONSWhile the desire for high-precision,dimensionally stable spacecraft struc-tures has driven the development of MMCs, applications thus far have been limited by difficult fabrication processes.The first successful application of con-tinuous-fiber reinforced MMC has been the application of B/Al tubular struts used as the frame and rib truss members in the mid-fuselage section, and as the landing gear drag link of the Space Shuttle Orbiter (Figure 1). Several hun-dred B/Al tube assemblies with tita-nium collars and end fittings were pro-duced for each shuttle orbiter. In this application, the B/Al tubes provided 45% weight savings over the baselinealuminum design.The major application of Gr/Al composite is a high-gain antenna boom (Figure 2) for the Hubble Space Telescope made with diffusion-bonded sheet of P100 graphite fi-bers in 6061 Al. This boom (3.6 m long) offers the desired stiffness and low CTE to maintain the position of the antenna during space maneuvers.In addition, it provides the wave-guide function,with the MMC’s excel-lent electrical conductiv-ity enabling electrical-signal transmission be-tween the spacecraft and the antenna dish. Also contributing to its success in this function is the MMC’s high dimensional stability—the mate-rial maintains internal dimensional tol-erance of ±0.15 mm along the entire length. While the part currently in ser-vice is continuously reinforced with graphite fibers, replacement structures produced with less expensive DRA have been certified.Like the Gr/Al structural boom, a few MMCs have been designed to serve multiple purposes, such as structural,electrical, and thermal-control functions.For example, prototype Gr/Al compos-ites were developed as structural radia-tors to perform structural, thermal, and EMI-shielding functions.5 Also, Gr/Cu MMCs with high thermal conductivity were developed for high-temperature structural radiators.6 A DRA panel is used as a heat sink between two printed circuit boards to provide both thermalFigure 3. P100/AZ91C Gr/Mg tubes produced by the vacuum-assist casting process: (a) as-cast tubes, and (b) demonstration Gr/Mg truss structure.abFigure 4. Cast SiCp/Al attachment fittings: (a) multi-inlet fitting for a truss node, and (b) cast fitting brazed to a Gr/Al tube.baResearch Projects Agency and the U.S.Air Force, graphite/magnesium tubes for truss-structure applications have been successfully produced (jointly by Lockheed Martin Space Systems of Colo-rado and Fiber Materials of Maine) by the filament-winding vacuum-assisted casting process. Figure 3 shows a few of the cast Gr/Mg tubes (50 mm dia × 1.2 m long) that were produced to demonstrate the reproducibility and reliability of the fabrication method.Of the DRA composites, reinforce-ments of both particulate SiC p /Al and whisker (w) SiC w /Al were extensively characterized and evaluated during the 1980s. Potential applications included joints and attachment fittings for truss structures, longerons, electronic pack-ages, thermal planes, mechanism hous-ings, and bushings. Figure 4 shows a multi-inlet SiC p /Al truss node produced by a near net-shape casting process.Because of their combination of high thermal conductivity, tailorable CTE (to match the CTE of electronic materials such as gallium arsenide or alumina),and low density, DRA composites are especially advantageous for electronic packaging and thermal-management applications.8,9 Several SiC p /Al and Gr p /Al (Figure 5) electronic packages have been space-qualified and are now flown on communication satellites and Global Positioning System satellites. These com-ponents are not only significantly lighter than those produced from previous metal alloys, but they provide significant cost savings through net-shape manufactur-ing.9 DRA is also used for thermal man-agement of spacecraft power semicon-ductor modules in geosynchronous earth-orbit communication satellites,displacing Cu/W alloys with a much higher density and lower thermal con-ductivity, while generating a weight sav-ings of more than 80%. These modules are also used in a number of land-based systems, which accounts for an annual production near 1 million piece-parts.With these demonstrated benefits, ap-plication of DRA MMCs for electronicpackages will continue to flourish for space applications.STATUS AND FUTURE When continuous-fiber reinforced MMCs were no longer needed for the critical strategic defense system/mis-sions, the development of those MMCs for space applications came to an abrupt halt. Major improvements were still nec-essary, and manufacturing and assem-bly problems remained to be solved. In essence, continuous-fiber reinforced MMCs were not able to attain their full potential as an engineered material for spacecraft applications. During the same period, Gr/Ep, with its superior specific stiffness and strength in the uniaxially-aligned fiber orientation, became an es-tablished choice for tube structures in spacecraft trusses. Issues of environmen-tal stability in the space environment have been satisfactorily resolved.However, particle-reinforced metals provide very good specific strength and stiffness, isotropic properties, ease of manufacturing to near net shape, and excellent thermal and electrical proper-ties, and affordability, making discon-tinuous MMCs suitable for a wide range of space applications. The high struc-tural efficiency and isotropic properties of discontinuously reinforced metals pro-vide a good match with the required multiaxial loading for truss nodes, wherehigh loads are encountered. DRA is amanagement and protection against flex-ure and vibration, which could lead to premature failure of the components in the circuit board.In technology-development programs sponsored by the U.S. Defense AdvancedFigure 5. Discontinuously reinforced aluminum MMCs for elec-tronic packaging applications: (a) SiCp/Al electronic packagefor a remote power controller (photo courtesy of LockheedMartin Corporation), and (b) cast Gr p /Al components (photocourtesy of MMCC, Inc.).ba candidate for lightly-loaded trusses,while discontinuously reinforced Ti(DRTi) is more favorable for highly-loaded trusses. DRTi, now commerciallyavailable in both the United States and Japan, offers excellent values of absolute strength and stiffness as well as specific strength and stiffness.A wide range of additional applica-tions exist for discontinuously reinforced metals. Opportunities for thermal man-agement and electronic packaging in-clude radiator panels and battery sleeves,power semiconductor packages, micro-wave modules, black box enclosures,and printed circuit board heat sinks. For example, the DSCS-III, a military com-munication satellite, uses more than 23kg of Kovar for microwave packaging.Replacing this metal with Al/SiCp,which is used for thermal management in land-based systems, would save more than 13 kg of weight and provide a cost savings over Kovar components. Poten-tial satellite subsystem applications in-clude brackets and braces currently made from metals with lower specific strength and stiffness, semimono-coque plates and cylin-ders, fittings for organic-matrix composite tubes,hinges, gimbals, inertial wheel housings and electro-optical sub-systems.MMCs are routinely included as candidate materials for primary and secondary structural applications. However,simply having the best engineered material with extraordinary strength,stiffness, and environ-mental resistance is no guarantee of insertion.The availability and affordability of continu-ously reinforced MMCremains a significant bar-rier to insertion.Designers who often make the decision of ma-terial selection must be-come more familiar with the properties, commer-cial availability and life-cycle affordability of ex-isting discontinuously reinforced metals. Mate-rial performance must be integrated with innova-tive design and afford-able manufacturing methods to produce sys-tems and subsystemsthat provide tangiblebenefits. However, in the absence of system-pull and adequate resources,it is difficult to surmountthe technical and cost barriers.Recognizing that defense- and aero-space-driven materials need to turn to the commercial market place, Carlson 10cited four recurring principles that will shape the future of advanced materials such as organic-matrix and MMCs. These four principles included system solu-tions, economical manufacturing pro-cessing, diverse markets, and new tech-nologies. In terms of system solutions,the decision regarding designs, processesand materials must be made synergisti-cally to attain maximum benefit. No single mission or system application can sustain the cost of developing new mate-rials and processes. Thus, the use of DRA in diverse markets such as automotive,recreational, and aircraft industries has made DRA MMC affordable for space-craft applications such as electronic pack-aging. Building upon the success of DRA in electronic packaging and in structural applications in the automotive and aero-nautical fields, DRA is also being evalu-ated for truss end fittings, mechanism housings, and longerons.During the development of MMCs,significant advancements were made on the fundamental science and technology front, including a basic understanding of composite behavior, fiber-matrix in-terfaces, surface coatings, manufactur-ing processes, and thermal-mechanical processing of MMCs. Subsequently, the technology experience benefited the lat-ter development of high-temperature in-termetallic-matrix composites. (Research activities that will be required to sup-port more widespread use of MMCs for space applications have been discussed in Reference 9.)Lightweight, stiff, and strong Gr/Al and DRA MMCs will continue to be included in material trade studies for spacecraft components, as MMCs offer significant payoffs in terms of perfor-mance (e.g., high precision, survivable)for specific systems. For successful use in space applications, continuous MMCs must become more affordable, readily available, reliable/reproducible, and repairable, exhibiting equivalent or bet-ter properties than competing graphite/epoxy or metallic parts. Discontinuous metals, with their broad range of func-tional properties including high struc-tural efficiency and isotropic properties,offer the greatest potential for a wide range of space-system applications. A good understanding provided by years of research, and a strong industry based on applications in the automotive, recre-ation, aeronautical, and land-based com-munications markets, have estab-lished the foundation for cost-effective insertion of discontinuously reinforced metals in the space industry.References1. Jerry G. Baetz, “Metal Matrix Composites: Their Time Has Come,” Aerospace America (November 1998), pp. 14–16.2. W.S. Johnson, “Metal Matrix Composites: Their Time to Shine?,” ASTM Standardization News (October 1987), pp. 36–39.3. D.R. Tenny, G.F. Sykes, and D.E. Bowles, “Composite Materials for Space Structures,” Proc. Third European Symp.Spacecraft Materials in Space Environment, ESA SP-232(Noordwijk, Netherlands: European Space Agency, October 1985), pp. 9–21.4. M.E. Buck and R.J. Suplinskas, “Continuous Boron Fiber MMC’s,” Engineered Metal Handbook, Vol. 1 (Materials Park,OH: ASM, 1987), pp. 851–857.5. D.M. Goddard, P.D. Burke, and D.E. Kizer, “Continuous Graphite Fiber MMC’s,” Engineered Materials Handbook, Vol.1 (Materials Park, OH: ASM, 1987), p. 867.6. A.J. Juhasz and G.P. Peterson, Review of Advanced Radiator Technologies for Spacecraft Power Systems and Space Thermal Control, NASA TP-4555 (1994).7. S.P. Rawal and M.S. Misra, “Dimensional Stability of Cast Gr-Mg Composites,” 19th International SAMPE Conference (Covina, CA: SAMPE, October 1987), pp. 134–147.8. C. Thaw et al., “Metal Matrix Composites for Microwave Packaging Components,” Electronic Packaging and Production (August 1987), pp. 27–29.9. D.B. Miracle and B. Maruyama, “Metal Matrix Composites for Space Systems: Current Uses and Future Opportunities,”Proc. National Space and Missile Materials Symp., ed. M. Stropki (Dayton, OH: Anteon Corp., 2000).10. C.C. Carlson, “Polymer Composites: Adjusting the Com-mercial Marketplace,” JOM, 45 (8) (1993), pp. 56–57.Suraj P. Rawal is Manager of Advanced Structures and Materials and Thermal Control Group at Lockheed Mar-tin Space Systems–Astronautics Operations, Denver,CO.For more information, contact S.P. Rawal,Lockheed Martin Space Systems–Aeronautics Operations, Advanced Structures and Materials and Thermal Control Group, Denver, Colorado.。