Dynamical quantum phase transitions in the dissipative Lipkin-Meshkov-Glick model and propo
第一届凝聚态物理会议

第一届凝聚态物理会议The 1st Conference on Condensed Matter Physics2015年7月15日- 17日清华大学目录01 会议概况02 组织委员会04 会议日程•总日程•大会报告•分会场报告•海报会场会议概况为了配合凝聚态物理在中国的迅速发展和国际地位的全面提升,进一步加强国内科研工作者在不同前沿领域的交流,推进国内和国际在凝聚态物理领域的相互交流和合作,为青年学生和研究人员学习和了解国际前沿进展创造更广泛的交流平台,拟定在过去已经成功举办了13届的“凝聚态理论与材料计算国际会议”系列会议的基础上,拓宽会议的主题,特别是加强凝聚态物理实验和理论的交流与融合,于2015年7月15日-17日在北京举办“第一届凝聚态物理会议”年会。
2015年第一届凝聚态物理会议是由清华大学物理系、中国科学院物理研究所、北京大学物理学院、量子物质科学协同创新中心联合主办。
这是国内首次在凝聚态物理方面举办的大型学术交流会。
本次会议是凝聚态理论与材料计算国际会议的延续和拓展,旨在增进国内外物理学者的学术交流,分享前沿科研成果,提高国内凝聚态物理的科研水平,扩大学术声誉。
第一届凝聚态物理会议将于2015年7月15日-17日在清华大学举行。
会议主题包括:拓扑量子态和多铁性、超导和多体物理、能源和低维物理、Quantum many-body theory and statistical physics、计算凝聚态物理、量子信息及其它与凝聚态物理的交叉领域等六个主题。
本次会议共设30个专题分会,将以大会特邀报告、分会特邀报告、口头报告和张贴海报等形式进行交流探讨。
组织委员会主办单位•清华大学物理系•中国科学院物理研究所•北京大学物理学院•量子物质科学协同创新中心顾问委员会:(按姓氏拼音序)崔田、杜瑞瑞、冯世平、龚新高、解士杰、李东海、李建新、林海青、李树深、陆卫、卢仲毅、吕力、沈保根、沈健、沈志勋、苏刚、王恩哥、王孝群、王玉鹏、向涛、薛其坤、张富春、张振宇组织委员会•清华大学物理系:陈曦、薛其坤•中科院物理研究所:胡江平、戴希、方忠、丁洪、周兴江、向涛•北京大学物理学院:谢心澄分会场负责人•拓扑量子态和多铁性:胡江平、陈曦、吕力、戴希、翁红明、寇谡鹏、吴从军•超导和多体物理:孙力玲、杨义峰、刘俊明、雒建林、袁辉球、李永庆、万歆、周毅•能源和低维物理:张振宇、李泓、陈弘、赵怀周、张远波•Quantum many-body theory and statistical physics:孟子扬、张广铭、郭文安、姚宏•计算凝聚态物理:姚裕贵、段文晖、龚新高、孟胜•量子信息及其它与凝聚态物理的交叉领域:范珩、田琳、翟荟、崔晓玲会议协调人•清华大学物理系:任俊(总协调人)•中国科学院物理研究所:齐建为、刘青梅•会务组:黄文艳、唐林、井小苏、周丹、骆洁、甘翠云、付德永、杨红、肖琳、胡文婷赞助单位•清华大学物理系•量子物质科学协同创新中心•中国科学院物理研究所•北京大学物理学院2015年第一届凝聚态物理会议分会场主题:A.拓扑量子态和多铁性A1拓扑半金属IA2拓扑半金属IIA3拓扑超导体和Majorana 费米子A4多铁性材料模拟与计算A5多铁性体系B.超导和多体物理B1铬基和锰基超导体B2极端条件下的超导行为B3铁基超导B4凝聚物质的激发态和动力学理论和实验B5重费米子物理C.能源和低维物理C1锂电池中的物理C2二维材料C3二维电子系统中的物理C4硅烯的最新进展C5热电中的新物理D.Quantum many-body theory and statistical physicsD1 Recent developments in strongly correlated quantum systems ID2 Recent developments in strongly correlated quantum systems IID3 Recent developments in strongly correlated quantum systems IIID4 Recent developments in strongly correlated quantum systems IVD5 Recent developments in strongly correlated quantum systems V注意事项:为了尊重外籍邀请报告人,如无特殊情况,D分会场报告请用英文。
自旋玻璃与消息传递算法Spin Glass and Message-Passing 概要
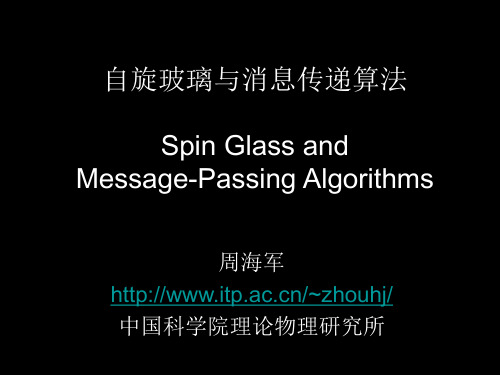
New vertex partially covered
New vertex always covered
39
Mean-field theory result is lower than experimental values for c > e=2.7183
2.7183
40
假定的相空间结构
41
14
distribution of equilibrium free-energies (contl.)
2
15
16
Which thermodynamic states contribute to the equilibrium properties? • If • If
Excited macrostates matter!
thevertexcoverproblem38alwaysuncoveredalwayscoveredunfrozenweigthartmannprl2000pre200139newvertexuncoverednewvertexpartiallycoverednewvertexalwayscovered40meanfieldtheoryresultislowerthanexperimentalvaluesforce271832718341假定的相空间结构42引入参数y43neighborsvertexiprobabilityvcsizeincreasereweightedprobabilityallunfrozenoralwayscoveredalwaysuncovered0atleastonealwaysuncoveredunfrozenoralwayscovered1444546同样的消息传递的算法可以用于解决神经网络?信息系统?满足性问题?
2022年自考专业(英语)英语科技文选考试真题及答案37

2022年自考专业(英语)英语科技文选考试真题及答案一、阅读理解题Directions: Read through the following passages. Choose the best answer and put the letter in the bracket. (20%)1、 (A) With the recent award of the Nobel Prize in physics, the spectacular work on Bose-Einstein condensation in a dilute gas of atoms has been honored. In such a Bose-Einstein condensate, close to temperatures of absolute zero, the atoms lose their individuality and a wave-like state of matter is created that can be compared in many ways to laser light. Based on such a Bose-Einstein condensate researchers in Munich together with a colleague from the ETH Zurich have now been able to reach a new state of matter in atomic physics. In order to reach this new phase for ultracold atoms, the scientists store a Bose-Einstein condensate in a three-dimensional lattice of microscopic light traps. By increasing the strength of the lattice, the researchers are able to dramatically alter the properties of the gas of atoms and can induce a quantum phase transition from the superfluid phase of a Bose-Einsteincondensate to a Mott insulator phase. In this new state of matter it should now be possible to investigate fundamental problems of solid-state physics, quantum optics and atomic physics. For a weak optical lattice the atoms form a superfluid phase of a Bose-Einstein condensate. In this phase, each atom is spread out over the entire lattice in a wave-like manner as predicted by quantum mechanics. The gas of atoms may then move freely through the lattice. For a strong optical lattice the researchers observe a transition to an insulating phase, with an exact number of atoms at each lattice site. Now the movement of the atoms through the lattice is blocked due to therepulsive interactions between them. Some physicists have been able to show that it is possible to reversibly cross the phase transition between these two states of matter. The transition is called a quantum phase transition because it is driven by quantum fluctuations and can take place even at temperatures of absolute zero. These quantum fluctuations are a direct consequence of Heisenberg’s uncertainty relation. Normally phase transitions are driven by thermal fluctuations, which are absent at zero temperature. With their experiment, the researchers in Munich have been able to enter a new phase in the physics of ultracold atoms. In the Mott insulator state theatoms can no longer be described by the highly successful theories for Bose-Einstein condensates. Now theories are required that take into account the dominating interactions between the atoms and which are far less understood. Here the Mott insulator state may help in solving fundamental questions of strongly correlated systems, which are the basis for our understanding of superconductivity. Furthermore, the Mott insulator state opens many exciting perspectives for precision matter-wave interferometry and quantum computing.What does the passage mainly discuss?A.Bose-Einstein condensation.B.Quantum phase transitions.C.The Mott insulator state.D.Optical lattices.2、What will the scientists possibly do by reaching the new state of matter in atomic physics?A.Store a Bose-Einstein condensate in three-dimensional lattice of microscopic light traps.B.Increase the strength of the lattice.C.Alter the properties of the gas of atoms.D.Examine fundamental problems of atomic physics.3、Which of the following is NOT mentioned in relation to aweak optical lattice?A.The atoms form a superfluid phase of a Bose-Einstein condensate.B.Each atom is spread out over the entire lattice.C.The gas of atoms may move freely through the lattice.D.The superfluid phase changes into an insulating phase.4、What can be said about the quantum phase transition?A.It can take place at temperatures of absolute zero.B.It cannot take place above the temperatures of absolute zero.C.It is driven by thermal fluctuations.D.It is driven by the repulsive interactions between atoms.5、The author implies all the following about the Mott insulator state EXCEPT that______.A.the theory of Bose-Einstein condensation can’t possibly account for the atoms in the Mott insulator stateB.not much is known about the dominating interactions between the atoms in the Mott insulator stateC.it offers new approaches to exact quantum computingD.it forms a superfluid phase of a Bose-Einstein condensate6、 (B) Gene therapy and gene-based drugs are two ways we would benefit from our growing mastery of genetic science. But therewill be others as well. Here is one of the remarkable therapies on the cutting edge of genetic research that could make their way into mainstream medicine in the c oming years. While it’s true that just about every cell in the body has the instructions to make a complete human, most of those instructions are inactivated, and with good reason: the last thing you want for your brain cells is to start churning out stomach acid or your nose to turn into a kidney. The only time cells truly have the potential to turn into any and all body parts is very early in a pregnancy, when so-called stem cells haven’t begun to specialize. Most diseases involve the death of healthy cells—brain cells in Alzheimer’s, cardiac cells in heart disease, pancreatic cells in diabetes, to name a few; if doctors could isolate stem cells, then direct their growth, they might be able to furnish patients with healthy replacement tissue. It was incredibly difficult, but last fall scientists at the University of Wisconsin managed to isolate stem cells and get them to grow into neural, gut, muscle and bone cells. The process still can’t be controlled, and may have unforeseen limitations; but if efforts to understand and master stem-cell development prove successful, doctors will have a therapeutic tool of incredible power. The same applies to cloning, whichis really just the other side of the coin; true cloning, as first shown, with the sheep Dolly two years ago, involves taking a developed cell and reactivating the genome within, resenting its developmental instructions to a pristine state. Once that happens, the rejuvenated cell can develop into a full-fledged animal, genetically identical to its parent. For agriculture, in which purely physical characteristics like milk production in a cow or low fat in a hog have real market value, biological carbon copies could become routine within a few years. This past year scientists have done for mice and cows what Ian Wilmut did for Dolly, and other creatures are bound to join the cloned menagerie in the coming year. Human cloning, on the other hand, may be technically feasible but legally and emotionally more difficult. Still, one day it will happen. The ability to reset body cells to a pristine, undeveloped state could give doctors exactly the same advantages they would get from stem cells: the potential to make healthy body tissues of all sorts. And thus to cure disease.That could prove to be a true “miracle cu re”.What is the passage mainly about?A.Tomorrow’s tissue factory.B.A terrific boon to medicine.C.Human cloning.D.Genetic research.7、 According to the passage, it can be inferred that which of the following reflects the author’s opinion?A.There will inevitably be human cloning in the coming year.B.The potential to make healthy body tissues is undoubtedly a boon to human beings.C.It is illegal to clone any kind of creatures in the world.D.It is legal to clone any kind of creatures in the world except human.8、Which of the following is NOT true according to the passage?A.Nearly every cell in the human brain has the instructions to make a complete human.B.It is impossible for a cell in your nose to turn into a kidney.C.It is possible to turn out healthy replacement tissues with isolated stem cells.D.There will certainly appear some new kind of cloned animal in the near future.9、All of the following are steps involved in true cloning EXCEPT_______.A.selecting a stem cellB.taking a developed cellC.reactivating the genome within the developed cellD.resetting the developmental instructions in the cell to its original state10、The word “rejuvenated” in para. 5 is closest in meaning to_______.A.rescuedB.reactivatedC.recalledD.regulated参考答案:【一、阅读理解题】1~5CDDAD6~10DBBA。
孙昌璞 - 中国科学院理论物理研究所
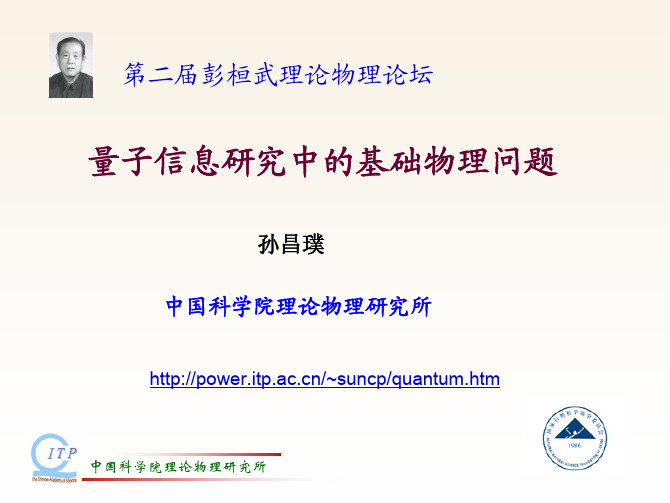
CEV
Controlled Evolution
̃, 1 1
1,0 ̃ 3 p 1,1 S , D |1, 0 〈1, 0| p S , D 1 , 1
̃ p 0,1 S ,D 0, 1
̃ , 1 p 0,0 |0, 0 〈0, 0|. 0 S ,D
中国科学院理论物理研究所
正功条件与热机效率
Measurement do not lead to entropy increase
,1 1, 0 ρ ( 2) = p 1 S , D | 1, 0〉〈1, 0 | + p S , D | 1, 1〉〈1, 1 | 0 ,1 0, 0 + pS , D | 0,1〉〈 0, 1 | + p S , D | 0, 0〉〈 0, 0 | .
2. 固态量子计算与关联系统演化的动力学敏感性
Quan, Song, Liu, Zanardi, and Sun, Decay of Loschmidt Echo Enhanced by Quantum Criticality, Phys. Rev. Lett. 96, 140604 (2006)
3. 量子信息启发的未来量子器件
中国科学院理论物理研究所
量子信息载体的物理实现
Ion Traps, Photons Liquid NMR
Nuclear Spins in Semiconductors
相干性
可规模化
易控制
Cooper-pair box ,SQUID, Single Juction
约瑟芬森结
2002-2003年JJ Q-比特的相干性得到极大改进
|e
|g
0 or 1
Quantum State
凝聚态物理相关诺贝尔奖Word版
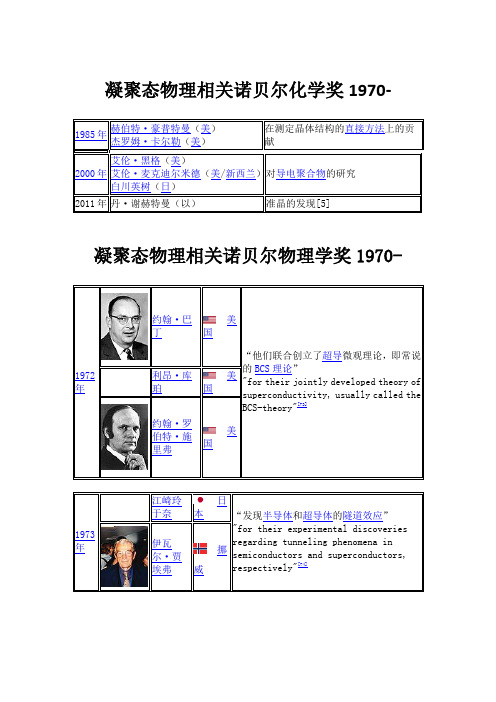
凝聚态物理相关诺贝尔化学奖1970-凝聚态物理相关诺贝尔物理学奖1970-约翰·巴丁美国美国约翰·罗伯特·施里弗美国日本“发现"for their experimental discoveriesregarding tunneling phenomena insemiconductors and superconductors,respectively"挪威英国“他理论上预测出通过隧道势垒的超电流的性质,特别是那些通常被称为象”"for his theoretical predictions of the properties of a supercurrent through a tunnel barrier, in particular those phenomena which are generally known as the Josephson effect"美国英国美国年彼得·列昂尼多维奇·卡皮苏联美国“对与相转变有关的临界现象理论的贡献”"for his theory for critical phenomena inconnection with phase transitions"克劳斯·冯·克利青德国“发现"for the discovery of thequantized Hall effect"德国德国瑞士约翰内斯·贝德诺尔茨德国卡尔·米勒瑞士法国美国美国美国若雷斯·阿尔费罗夫俄罗斯“发展了用于高速电子学和半导体异质结构"for developing semiconductorheterostructures used in high-speed-and optoelectronics"德国美国“在发明"for his part in the invention of theintegrated circuit"美国“在碱性原子稀薄气体的聚态质的早期基础性研究”"for the achievement of Bose-Einsteincondensation in dilute gases of alkaliatoms,of美国德国美国俄罗斯“对性贡献”"for pioneering contributions to thesuperfluids"俄罗斯英国美国法国德国英国美国[110]美国美国荷兰俄罗斯“在二维"for groundbreaking experiments regarding the two-dimensional material graphene"康斯坦丁·诺沃肖洛夫英国俄罗斯(注:可编辑下载,若有不当之处,请指正,谢谢!)。
berry phases in electronic structure theory pdf
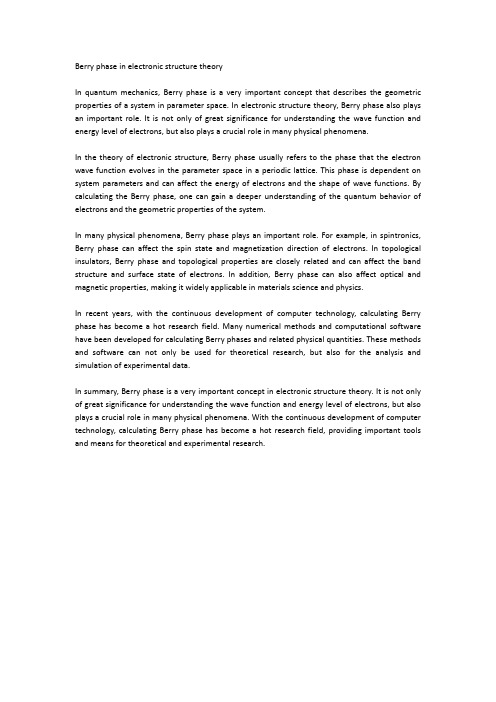
Berry phase in electronic structure theoryIn quantum mechanics,Berry phase is a very important concept that describes the geometric properties of a system in parameter space.In electronic structure theory,Berry phase also plays an important role.It is not only of great significance for understanding the wave function and energy level of electrons,but also plays a crucial role in many physical phenomena.In the theory of electronic structure,Berry phase usually refers to the phase that the electron wave function evolves in the parameter space in a periodic lattice.This phase is dependent on system parameters and can affect the energy of electrons and the shape of wave functions.By calculating the Berry phase,one can gain a deeper understanding of the quantum behavior of electrons and the geometric properties of the system.In many physical phenomena,Berry phase plays an important role.For example,in spintronics, Berry phase can affect the spin state and magnetization direction of electrons.In topological insulators,Berry phase and topological properties are closely related and can affect the band structure and surface state of electrons.In addition,Berry phase can also affect optical and magnetic properties,making it widely applicable in materials science and physics.In recent years,with the continuous development of computer technology,calculating Berry phase has become a hot research field.Many numerical methods and computational software have been developed for calculating Berry phases and related physical quantities.These methods and software can not only be used for theoretical research,but also for the analysis and simulation of experimental data.In summary,Berry phase is a very important concept in electronic structure theory.It is not only of great significance for understanding the wave function and energy level of electrons,but also plays a crucial role in many physical phenomena.With the continuous development of computer technology,calculating Berry phase has become a hot research field,providing important tools and means for theoretical and experimental research.。
分子动力学模拟碳纳米管中冰的相变
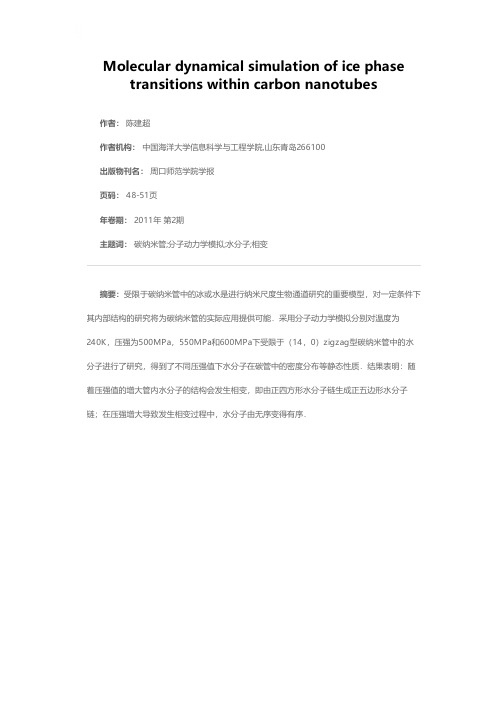
Molecular dynamical simulation of ice phase transitions within carbon nanotubes 作者: 陈建超
作者机构: 中国海洋大学信息科学与工程学院,山东青岛266100
出版物刊名: 周口师范学院学报
页码: 48-51页
年卷期: 2011年 第2期
主题词: 碳纳米管;分子动力学模拟;水分子;相变
摘要:受限于碳纳米管中的冰或水是进行纳米尺度生物通道研究的重要模型,对一定条件下其内部结构的研究将为碳纳米管的实际应用提供可能.采用分子动力学模拟分别对温度为
240K,压强为500MPa,550MPa和600MPa下受限于(14,0)zigzag型碳纳米管中的水分子进行了研究,得到了不同压强值下水分子在碳管中的密度分布等静态性质.结果表明:随着压强值的增大管内水分子的结构会发生相变,即由正四方形水分子链生成正五边形水分子链;在压强增大导致发生相变过程中,水分子由无序变得有序.。
物理学专业英语

华中师范大学物理学院物理学专业英语仅供内部学习参考!2014一、课程的任务和教学目的通过学习《物理学专业英语》,学生将掌握物理学领域使用频率较高的专业词汇和表达方法,进而具备基本的阅读理解物理学专业文献的能力。
通过分析《物理学专业英语》课程教材中的范文,学生还将从英语角度理解物理学中个学科的研究内容和主要思想,提高学生的专业英语能力和了解物理学研究前沿的能力。
培养专业英语阅读能力,了解科技英语的特点,提高专业外语的阅读质量和阅读速度;掌握一定量的本专业英文词汇,基本达到能够独立完成一般性本专业外文资料的阅读;达到一定的笔译水平。
要求译文通顺、准确和专业化。
要求译文通顺、准确和专业化。
二、课程内容课程内容包括以下章节:物理学、经典力学、热力学、电磁学、光学、原子物理、统计力学、量子力学和狭义相对论三、基本要求1.充分利用课内时间保证充足的阅读量(约1200~1500词/学时),要求正确理解原文。
2.泛读适量课外相关英文读物,要求基本理解原文主要内容。
3.掌握基本专业词汇(不少于200词)。
4.应具有流利阅读、翻译及赏析专业英语文献,并能简单地进行写作的能力。
四、参考书目录1 Physics 物理学 (1)Introduction to physics (1)Classical and modern physics (2)Research fields (4)V ocabulary (7)2 Classical mechanics 经典力学 (10)Introduction (10)Description of classical mechanics (10)Momentum and collisions (14)Angular momentum (15)V ocabulary (16)3 Thermodynamics 热力学 (18)Introduction (18)Laws of thermodynamics (21)System models (22)Thermodynamic processes (27)Scope of thermodynamics (29)V ocabulary (30)4 Electromagnetism 电磁学 (33)Introduction (33)Electrostatics (33)Magnetostatics (35)Electromagnetic induction (40)V ocabulary (43)5 Optics 光学 (45)Introduction (45)Geometrical optics (45)Physical optics (47)Polarization (50)V ocabulary (51)6 Atomic physics 原子物理 (52)Introduction (52)Electronic configuration (52)Excitation and ionization (56)V ocabulary (59)7 Statistical mechanics 统计力学 (60)Overview (60)Fundamentals (60)Statistical ensembles (63)V ocabulary (65)8 Quantum mechanics 量子力学 (67)Introduction (67)Mathematical formulations (68)Quantization (71)Wave-particle duality (72)Quantum entanglement (75)V ocabulary (77)9 Special relativity 狭义相对论 (79)Introduction (79)Relativity of simultaneity (80)Lorentz transformations (80)Time dilation and length contraction (81)Mass-energy equivalence (82)Relativistic energy-momentum relation (86)V ocabulary (89)正文标记说明:蓝色Arial字体(例如energy):已知的专业词汇蓝色Arial字体加下划线(例如electromagnetism):新学的专业词汇黑色Times New Roman字体加下划线(例如postulate):新学的普通词汇1 Physics 物理学1 Physics 物理学Introduction to physicsPhysics is a part of natural philosophy and a natural science that involves the study of matter and its motion through space and time, along with related concepts such as energy and force. More broadly, it is the general analysis of nature, conducted in order to understand how the universe behaves.Physics is one of the oldest academic disciplines, perhaps the oldest through its inclusion of astronomy. Over the last two millennia, physics was a part of natural philosophy along with chemistry, certain branches of mathematics, and biology, but during the Scientific Revolution in the 17th century, the natural sciences emerged as unique research programs in their own right. Physics intersects with many interdisciplinary areas of research, such as biophysics and quantum chemistry,and the boundaries of physics are not rigidly defined. New ideas in physics often explain the fundamental mechanisms of other sciences, while opening new avenues of research in areas such as mathematics and philosophy.Physics also makes significant contributions through advances in new technologies that arise from theoretical breakthroughs. For example, advances in the understanding of electromagnetism or nuclear physics led directly to the development of new products which have dramatically transformed modern-day society, such as television, computers, domestic appliances, and nuclear weapons; advances in thermodynamics led to the development of industrialization; and advances in mechanics inspired the development of calculus.Core theoriesThough physics deals with a wide variety of systems, certain theories are used by all physicists. Each of these theories were experimentally tested numerous times and found correct as an approximation of nature (within a certain domain of validity).For instance, the theory of classical mechanics accurately describes the motion of objects, provided they are much larger than atoms and moving at much less than the speed of light. These theories continue to be areas of active research, and a remarkable aspect of classical mechanics known as chaos was discovered in the 20th century, three centuries after the original formulation of classical mechanics by Isaac Newton (1642–1727) 【艾萨克·牛顿】.University PhysicsThese central theories are important tools for research into more specialized topics, and any physicist, regardless of his or her specialization, is expected to be literate in them. These include classical mechanics, quantum mechanics, thermodynamics and statistical mechanics, electromagnetism, and special relativity.Classical and modern physicsClassical mechanicsClassical physics includes the traditional branches and topics that were recognized and well-developed before the beginning of the 20th century—classical mechanics, acoustics, optics, thermodynamics, and electromagnetism.Classical mechanics is concerned with bodies acted on by forces and bodies in motion and may be divided into statics (study of the forces on a body or bodies at rest), kinematics (study of motion without regard to its causes), and dynamics (study of motion and the forces that affect it); mechanics may also be divided into solid mechanics and fluid mechanics (known together as continuum mechanics), the latter including such branches as hydrostatics, hydrodynamics, aerodynamics, and pneumatics.Acoustics is the study of how sound is produced, controlled, transmitted and received. Important modern branches of acoustics include ultrasonics, the study of sound waves of very high frequency beyond the range of human hearing; bioacoustics the physics of animal calls and hearing, and electroacoustics, the manipulation of audible sound waves using electronics.Optics, the study of light, is concerned not only with visible light but also with infrared and ultraviolet radiation, which exhibit all of the phenomena of visible light except visibility, e.g., reflection, refraction, interference, diffraction, dispersion, and polarization of light.Heat is a form of energy, the internal energy possessed by the particles of which a substance is composed; thermodynamics deals with the relationships between heat and other forms of energy.Electricity and magnetism have been studied as a single branch of physics since the intimate connection between them was discovered in the early 19th century; an electric current gives rise to a magnetic field and a changing magnetic field induces an electric current. Electrostatics deals with electric charges at rest, electrodynamics with moving charges, and magnetostatics with magnetic poles at rest.Modern PhysicsClassical physics is generally concerned with matter and energy on the normal scale of1 Physics 物理学observation, while much of modern physics is concerned with the behavior of matter and energy under extreme conditions or on the very large or very small scale.For example, atomic and nuclear physics studies matter on the smallest scale at which chemical elements can be identified.The physics of elementary particles is on an even smaller scale, as it is concerned with the most basic units of matter; this branch of physics is also known as high-energy physics because of the extremely high energies necessary to produce many types of particles in large particle accelerators. On this scale, ordinary, commonsense notions of space, time, matter, and energy are no longer valid.The two chief theories of modern physics present a different picture of the concepts of space, time, and matter from that presented by classical physics.Quantum theory is concerned with the discrete, rather than continuous, nature of many phenomena at the atomic and subatomic level, and with the complementary aspects of particles and waves in the description of such phenomena.The theory of relativity is concerned with the description of phenomena that take place in a frame of reference that is in motion with respect to an observer; the special theory of relativity is concerned with relative uniform motion in a straight line and the general theory of relativity with accelerated motion and its connection with gravitation.Both quantum theory and the theory of relativity find applications in all areas of modern physics.Difference between classical and modern physicsWhile physics aims to discover universal laws, its theories lie in explicit domains of applicability. Loosely speaking, the laws of classical physics accurately describe systems whose important length scales are greater than the atomic scale and whose motions are much slower than the speed of light. Outside of this domain, observations do not match their predictions.Albert Einstein【阿尔伯特·爱因斯坦】contributed the framework of special relativity, which replaced notions of absolute time and space with space-time and allowed an accurate description of systems whose components have speeds approaching the speed of light.Max Planck【普朗克】, Erwin Schrödinger【薛定谔】, and others introduced quantum mechanics, a probabilistic notion of particles and interactions that allowed an accurate description of atomic and subatomic scales.Later, quantum field theory unified quantum mechanics and special relativity.General relativity allowed for a dynamical, curved space-time, with which highly massiveUniversity Physicssystems and the large-scale structure of the universe can be well-described. General relativity has not yet been unified with the other fundamental descriptions; several candidate theories of quantum gravity are being developed.Research fieldsContemporary research in physics can be broadly divided into condensed matter physics; atomic, molecular, and optical physics; particle physics; astrophysics; geophysics and biophysics. Some physics departments also support research in Physics education.Since the 20th century, the individual fields of physics have become increasingly specialized, and today most physicists work in a single field for their entire careers. "Universalists" such as Albert Einstein (1879–1955) and Lev Landau (1908–1968)【列夫·朗道】, who worked in multiple fields of physics, are now very rare.Condensed matter physicsCondensed matter physics is the field of physics that deals with the macroscopic physical properties of matter. In particular, it is concerned with the "condensed" phases that appear whenever the number of particles in a system is extremely large and the interactions between them are strong.The most familiar examples of condensed phases are solids and liquids, which arise from the bonding by way of the electromagnetic force between atoms. More exotic condensed phases include the super-fluid and the Bose–Einstein condensate found in certain atomic systems at very low temperature, the superconducting phase exhibited by conduction electrons in certain materials,and the ferromagnetic and antiferromagnetic phases of spins on atomic lattices.Condensed matter physics is by far the largest field of contemporary physics.Historically, condensed matter physics grew out of solid-state physics, which is now considered one of its main subfields. The term condensed matter physics was apparently coined by Philip Anderson when he renamed his research group—previously solid-state theory—in 1967. In 1978, the Division of Solid State Physics of the American Physical Society was renamed as the Division of Condensed Matter Physics.Condensed matter physics has a large overlap with chemistry, materials science, nanotechnology and engineering.Atomic, molecular and optical physicsAtomic, molecular, and optical physics (AMO) is the study of matter–matter and light–matter interactions on the scale of single atoms and molecules.1 Physics 物理学The three areas are grouped together because of their interrelationships, the similarity of methods used, and the commonality of the energy scales that are relevant. All three areas include both classical, semi-classical and quantum treatments; they can treat their subject from a microscopic view (in contrast to a macroscopic view).Atomic physics studies the electron shells of atoms. Current research focuses on activities in quantum control, cooling and trapping of atoms and ions, low-temperature collision dynamics and the effects of electron correlation on structure and dynamics. Atomic physics is influenced by the nucleus (see, e.g., hyperfine splitting), but intra-nuclear phenomena such as fission and fusion are considered part of high-energy physics.Molecular physics focuses on multi-atomic structures and their internal and external interactions with matter and light.Optical physics is distinct from optics in that it tends to focus not on the control of classical light fields by macroscopic objects, but on the fundamental properties of optical fields and their interactions with matter in the microscopic realm.High-energy physics (particle physics) and nuclear physicsParticle physics is the study of the elementary constituents of matter and energy, and the interactions between them.In addition, particle physicists design and develop the high energy accelerators,detectors, and computer programs necessary for this research. The field is also called "high-energy physics" because many elementary particles do not occur naturally, but are created only during high-energy collisions of other particles.Currently, the interactions of elementary particles and fields are described by the Standard Model.●The model accounts for the 12 known particles of matter (quarks and leptons) thatinteract via the strong, weak, and electromagnetic fundamental forces.●Dynamics are described in terms of matter particles exchanging gauge bosons (gluons,W and Z bosons, and photons, respectively).●The Standard Model also predicts a particle known as the Higgs boson. In July 2012CERN, the European laboratory for particle physics, announced the detection of a particle consistent with the Higgs boson.Nuclear Physics is the field of physics that studies the constituents and interactions of atomic nuclei. The most commonly known applications of nuclear physics are nuclear power generation and nuclear weapons technology, but the research has provided application in many fields, including those in nuclear medicine and magnetic resonance imaging, ion implantation in materials engineering, and radiocarbon dating in geology and archaeology.University PhysicsAstrophysics and Physical CosmologyAstrophysics and astronomy are the application of the theories and methods of physics to the study of stellar structure, stellar evolution, the origin of the solar system, and related problems of cosmology. Because astrophysics is a broad subject, astrophysicists typically apply many disciplines of physics, including mechanics, electromagnetism, statistical mechanics, thermodynamics, quantum mechanics, relativity, nuclear and particle physics, and atomic and molecular physics.The discovery by Karl Jansky in 1931 that radio signals were emitted by celestial bodies initiated the science of radio astronomy. Most recently, the frontiers of astronomy have been expanded by space exploration. Perturbations and interference from the earth's atmosphere make space-based observations necessary for infrared, ultraviolet, gamma-ray, and X-ray astronomy.Physical cosmology is the study of the formation and evolution of the universe on its largest scales. Albert Einstein's theory of relativity plays a central role in all modern cosmological theories. In the early 20th century, Hubble's discovery that the universe was expanding, as shown by the Hubble diagram, prompted rival explanations known as the steady state universe and the Big Bang.The Big Bang was confirmed by the success of Big Bang nucleo-synthesis and the discovery of the cosmic microwave background in 1964. The Big Bang model rests on two theoretical pillars: Albert Einstein's general relativity and the cosmological principle (On a sufficiently large scale, the properties of the Universe are the same for all observers). Cosmologists have recently established the ΛCDM model (the standard model of Big Bang cosmology) of the evolution of the universe, which includes cosmic inflation, dark energy and dark matter.Current research frontiersIn condensed matter physics, an important unsolved theoretical problem is that of high-temperature superconductivity. Many condensed matter experiments are aiming to fabricate workable spintronics and quantum computers.In particle physics, the first pieces of experimental evidence for physics beyond the Standard Model have begun to appear. Foremost among these are indications that neutrinos have non-zero mass. These experimental results appear to have solved the long-standing solar neutrino problem, and the physics of massive neutrinos remains an area of active theoretical and experimental research. Particle accelerators have begun probing energy scales in the TeV range, in which experimentalists are hoping to find evidence for the super-symmetric particles, after discovery of the Higgs boson.Theoretical attempts to unify quantum mechanics and general relativity into a single theory1 Physics 物理学of quantum gravity, a program ongoing for over half a century, have not yet been decisively resolved. The current leading candidates are M-theory, superstring theory and loop quantum gravity.Many astronomical and cosmological phenomena have yet to be satisfactorily explained, including the existence of ultra-high energy cosmic rays, the baryon asymmetry, the acceleration of the universe and the anomalous rotation rates of galaxies.Although much progress has been made in high-energy, quantum, and astronomical physics, many everyday phenomena involving complexity, chaos, or turbulence are still poorly understood. Complex problems that seem like they could be solved by a clever application of dynamics and mechanics remain unsolved; examples include the formation of sand-piles, nodes in trickling water, the shape of water droplets, mechanisms of surface tension catastrophes, and self-sorting in shaken heterogeneous collections.These complex phenomena have received growing attention since the 1970s for several reasons, including the availability of modern mathematical methods and computers, which enabled complex systems to be modeled in new ways. Complex physics has become part of increasingly interdisciplinary research, as exemplified by the study of turbulence in aerodynamics and the observation of pattern formation in biological systems.Vocabulary★natural science 自然科学academic disciplines 学科astronomy 天文学in their own right 凭他们本身的实力intersects相交,交叉interdisciplinary交叉学科的,跨学科的★quantum 量子的theoretical breakthroughs 理论突破★electromagnetism 电磁学dramatically显著地★thermodynamics热力学★calculus微积分validity★classical mechanics 经典力学chaos 混沌literate 学者★quantum mechanics量子力学★thermodynamics and statistical mechanics热力学与统计物理★special relativity狭义相对论is concerned with 关注,讨论,考虑acoustics 声学★optics 光学statics静力学at rest 静息kinematics运动学★dynamics动力学ultrasonics超声学manipulation 操作,处理,使用University Physicsinfrared红外ultraviolet紫外radiation辐射reflection 反射refraction 折射★interference 干涉★diffraction 衍射dispersion散射★polarization 极化,偏振internal energy 内能Electricity电性Magnetism 磁性intimate 亲密的induces 诱导,感应scale尺度★elementary particles基本粒子★high-energy physics 高能物理particle accelerators 粒子加速器valid 有效的,正当的★discrete离散的continuous 连续的complementary 互补的★frame of reference 参照系★the special theory of relativity 狭义相对论★general theory of relativity 广义相对论gravitation 重力,万有引力explicit 详细的,清楚的★quantum field theory 量子场论★condensed matter physics凝聚态物理astrophysics天体物理geophysics地球物理Universalist博学多才者★Macroscopic宏观Exotic奇异的★Superconducting 超导Ferromagnetic铁磁质Antiferromagnetic 反铁磁质★Spin自旋Lattice 晶格,点阵,网格★Society社会,学会★microscopic微观的hyperfine splitting超精细分裂fission分裂,裂变fusion熔合,聚变constituents成分,组分accelerators加速器detectors 检测器★quarks夸克lepton 轻子gauge bosons规范玻色子gluons胶子★Higgs boson希格斯玻色子CERN欧洲核子研究中心★Magnetic Resonance Imaging磁共振成像,核磁共振ion implantation 离子注入radiocarbon dating放射性碳年代测定法geology地质学archaeology考古学stellar 恒星cosmology宇宙论celestial bodies 天体Hubble diagram 哈勃图Rival竞争的★Big Bang大爆炸nucleo-synthesis核聚合,核合成pillar支柱cosmological principle宇宙学原理ΛCDM modelΛ-冷暗物质模型cosmic inflation宇宙膨胀1 Physics 物理学fabricate制造,建造spintronics自旋电子元件,自旋电子学★neutrinos 中微子superstring 超弦baryon重子turbulence湍流,扰动,骚动catastrophes突变,灾变,灾难heterogeneous collections异质性集合pattern formation模式形成University Physics2 Classical mechanics 经典力学IntroductionIn physics, classical mechanics is one of the two major sub-fields of mechanics, which is concerned with the set of physical laws describing the motion of bodies under the action of a system of forces. The study of the motion of bodies is an ancient one, making classical mechanics one of the oldest and largest subjects in science, engineering and technology.Classical mechanics describes the motion of macroscopic objects, from projectiles to parts of machinery, as well as astronomical objects, such as spacecraft, planets, stars, and galaxies. Besides this, many specializations within the subject deal with gases, liquids, solids, and other specific sub-topics.Classical mechanics provides extremely accurate results as long as the domain of study is restricted to large objects and the speeds involved do not approach the speed of light. When the objects being dealt with become sufficiently small, it becomes necessary to introduce the other major sub-field of mechanics, quantum mechanics, which reconciles the macroscopic laws of physics with the atomic nature of matter and handles the wave–particle duality of atoms and molecules. In the case of high velocity objects approaching the speed of light, classical mechanics is enhanced by special relativity. General relativity unifies special relativity with Newton's law of universal gravitation, allowing physicists to handle gravitation at a deeper level.The initial stage in the development of classical mechanics is often referred to as Newtonian mechanics, and is associated with the physical concepts employed by and the mathematical methods invented by Newton himself, in parallel with Leibniz【莱布尼兹】, and others.Later, more abstract and general methods were developed, leading to reformulations of classical mechanics known as Lagrangian mechanics and Hamiltonian mechanics. These advances were largely made in the 18th and 19th centuries, and they extend substantially beyond Newton's work, particularly through their use of analytical mechanics. Ultimately, the mathematics developed for these were central to the creation of quantum mechanics.Description of classical mechanicsThe following introduces the basic concepts of classical mechanics. For simplicity, it often2 Classical mechanics 经典力学models real-world objects as point particles, objects with negligible size. The motion of a point particle is characterized by a small number of parameters: its position, mass, and the forces applied to it.In reality, the kind of objects that classical mechanics can describe always have a non-zero size. (The physics of very small particles, such as the electron, is more accurately described by quantum mechanics). Objects with non-zero size have more complicated behavior than hypothetical point particles, because of the additional degrees of freedom—for example, a baseball can spin while it is moving. However, the results for point particles can be used to study such objects by treating them as composite objects, made up of a large number of interacting point particles. The center of mass of a composite object behaves like a point particle.Classical mechanics uses common-sense notions of how matter and forces exist and interact. It assumes that matter and energy have definite, knowable attributes such as where an object is in space and its speed. It also assumes that objects may be directly influenced only by their immediate surroundings, known as the principle of locality.In quantum mechanics objects may have unknowable position or velocity, or instantaneously interact with other objects at a distance.Position and its derivativesThe position of a point particle is defined with respect to an arbitrary fixed reference point, O, in space, usually accompanied by a coordinate system, with the reference point located at the origin of the coordinate system. It is defined as the vector r from O to the particle.In general, the point particle need not be stationary relative to O, so r is a function of t, the time elapsed since an arbitrary initial time.In pre-Einstein relativity (known as Galilean relativity), time is considered an absolute, i.e., the time interval between any given pair of events is the same for all observers. In addition to relying on absolute time, classical mechanics assumes Euclidean geometry for the structure of space.Velocity and speedThe velocity, or the rate of change of position with time, is defined as the derivative of the position with respect to time. In classical mechanics, velocities are directly additive and subtractive as vector quantities; they must be dealt with using vector analysis.When both objects are moving in the same direction, the difference can be given in terms of speed only by ignoring direction.University PhysicsAccelerationThe acceleration , or rate of change of velocity, is the derivative of the velocity with respect to time (the second derivative of the position with respect to time).Acceleration can arise from a change with time of the magnitude of the velocity or of the direction of the velocity or both . If only the magnitude v of the velocity decreases, this is sometimes referred to as deceleration , but generally any change in the velocity with time, including deceleration, is simply referred to as acceleration.Inertial frames of referenceWhile the position and velocity and acceleration of a particle can be referred to any observer in any state of motion, classical mechanics assumes the existence of a special family of reference frames in terms of which the mechanical laws of nature take a comparatively simple form. These special reference frames are called inertial frames .An inertial frame is such that when an object without any force interactions (an idealized situation) is viewed from it, it appears either to be at rest or in a state of uniform motion in a straight line. This is the fundamental definition of an inertial frame. They are characterized by the requirement that all forces entering the observer's physical laws originate in identifiable sources (charges, gravitational bodies, and so forth).A non-inertial reference frame is one accelerating with respect to an inertial one, and in such a non-inertial frame a particle is subject to acceleration by fictitious forces that enter the equations of motion solely as a result of its accelerated motion, and do not originate in identifiable sources. These fictitious forces are in addition to the real forces recognized in an inertial frame.A key concept of inertial frames is the method for identifying them. For practical purposes, reference frames that are un-accelerated with respect to the distant stars are regarded as good approximations to inertial frames.Forces; Newton's second lawNewton was the first to mathematically express the relationship between force and momentum . Some physicists interpret Newton's second law of motion as a definition of force and mass, while others consider it a fundamental postulate, a law of nature. Either interpretation has the same mathematical consequences, historically known as "Newton's Second Law":a m t v m t p F ===d )(d d dThe quantity m v is called the (canonical ) momentum . The net force on a particle is thus equal to rate of change of momentum of the particle with time.So long as the force acting on a particle is known, Newton's second law is sufficient to。
凝聚态物理相关诺贝尔奖(可编辑修改word版)

凝聚态物理相关诺贝尔化学奖1970-1985 年赫伯特·豪普特曼(美)杰罗姆·卡尔勒(美)在测定晶体结构的直接方法上的贡献2000 年艾伦·黑格(美)艾伦·麦克迪尔米德(美/新西兰)白川英树(日)对导电聚合物的研究2011 年丹·谢赫特曼(以)准晶的发现[5]凝聚态物理相关诺贝尔物理学奖1970-1972年约翰·巴丁美国“他们联合创立了超导微观理论,即常说的BCS 理论”"for their jointly developed theory ofsuperconductivity, usually called theBCS-theory"[73]利昂·库珀美国约翰·罗伯特·施里弗国美1973年江崎玲于奈日本“发现半导体和超导体的隧道效应”"for their experimental discoveriesregarding tunneling phenomena insemiconductors and superconductors,respectively"[74]伊瓦尔·贾埃弗挪威国英 “他理论上预测出通过隧道势垒的超电流的性质,特别是那些通常被称为约瑟夫森效应 布赖 的现象” 恩·戴"for his theoretical predictions of the 维·约瑟 properties of a supercurrent through a 夫森 tunnel barrier, in particular those phenomena which are generally known as the Josephson effect"[74]1977 年菲利普·沃伦·安德森国美“对磁性和无序体系电子结构的基础性理论研究”"for their fundamental theoretical investigations of the electronicstructure of magnetic and disorderedsystems"[78]内维尔·莫特 国英约翰·凡扶累克 国美年彼得·列昂尼多维奇·卡皮查苏联“低温物理领域的基本发明和发现” "for his basic inventions and discoveries in the area of low- temperature physics"[79]1982 年肯尼斯·威尔逊美国“对与相转变有关的临界现象理论的贡献”"for his theory for critical phenomena in connection with phase transitions"[83]1985 年克劳斯·冯·克利青德国“发现量子霍尔效应”"for the discovery of thequantized Hall effect"[86]特·鲁斯卡德国“电子光学的基础工作和设计了第一台显微镜"for his fundamental work in electron optics,electron microscope"格尔德·宾宁德国“研制"for their design of the scanningtunneling microscope"海因里希·罗雷尔瑞士约翰内斯·贝德诺尔茨德国“在发现"for their important break-through the discovery of superconductivity ceramic materials"卡尔·米勒瑞士皮埃尔吉勒纳法国“发现研究简单系统中有序现象的方法可以被推广到比较复杂的物质形式,特别是推广到"forfor studying order phenomena in simple systems can be generalized to morecomplexliquid crystals and polymers"1996年戴维·李美国“发现了在氦-3 里的超流动性”"for their discovery ofsuperfluidity inhelium-3"[97]道格拉斯·奥谢罗夫美国罗伯特·理查森美国2000年若雷斯·阿尔费罗夫俄罗斯“发展了用于高速电子学和光电子学的半导体异质结构”"for developing semiconductorheterostructures used in high-speed-and optoelectronics"[101]赫伯特·克勒默德国杰克·基尔比美国“在发明集成电路中所做的贡献”"for his part in the invention of theintegrated circuit"[101]2001年埃里克·康奈尔国美“在碱性原子稀薄气体的玻色-爱因斯坦凝聚态方面取得的成就,以及凝聚态物质属性质的早期基础性研究”"for the achievement of Bose-Einsteincondensation in dilute gases of alkaliatoms, and for early fundamental studiesof the properties of the condensates"[102]卡尔·威曼国美沃尔夫冈·克特勒国德2003年阿列克谢·阿布里科索夫美国俄罗斯“对超导体和超流体理论做出的先驱性贡献”"for pioneering contributions tothe theory of superconductors andsuperfluids"[104]维塔利·金俄罗斯兹堡安东尼·莱格特英国美国2007 年艾尔伯·费尔法国“发现巨磁阻效应”"for the discovery of giant magnetoresistance"[108]彼得·格林贝格德国2009 年高锟英国 美国 [110] “在光学通信领域光在纤维中传输方面的突破性成就” "for groundbreaking achievementsconcerning the transmission of light infibers for optical communication"[111]威拉 德·博伊尔 美国“发明半导体成像器件电荷耦合器件” "for the invention of an imaging semiconductor circuit – the CCD sensor"[111]乔治·史密斯美国2010 年安德烈·海姆荷兰俄罗斯“在二维石墨烯材料的开创性实验”"for groundbreaking experimentsregarding the two-dimensionalmaterial graphene"[112]康斯坦丁·诺沃肖洛夫英国俄罗斯。
kurbatov方程
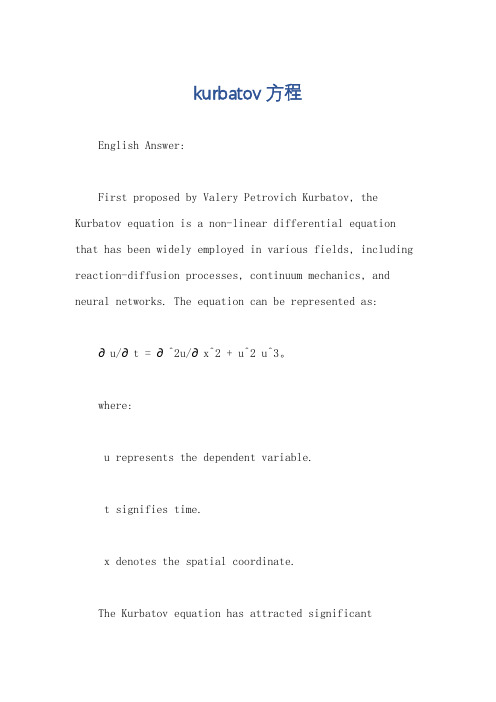
kurbatov方程English Answer:First proposed by Valery Petrovich Kurbatov, the Kurbatov equation is a non-linear differential equation that has been widely employed in various fields, including reaction-diffusion processes, continuum mechanics, and neural networks. The equation can be represented as:∂u/∂t = ∂^2u/∂x^2 + u^2 u^3。
where:u represents the dependent variable.t signifies time.x denotes the spatial coordinate.The Kurbatov equation has attracted significantattention due to its ability to exhibit a wide range of complex behaviors, including:Solitary wave solutions.Periodic and chaotic oscillations.Phase transitions.The solitons that emerge from the Kurbatov equation are self-reinforcing, localized waves that retain their form as they propagate. Periodic oscillations occur in certain parameter regimes, characterized by regular variations in the dependent variable over time. When the system becomes more complex, chaotic oscillations can arise, involving irregular and unpredictable fluctuations. Phase transitions refer to abrupt changes in the system's behavior, marked by shifts between different dynamical regimes.The Kurbatov equation has been used to model diverse phenomena, such as:Flame propagation.Chemical reactions.Pattern formation.Population dynamics.In the context of flame propagation, the Kurbatov equation describes the evolution of the flame front, capturing the interplay between heat transfer, chemical reactions, and fluid flow. It has also been applied to study chemical reactions, particularly those involving autocatalytic processes and oscillatory behavior. In pattern formation, the Kurbatov equation has been used to explain the emergence of complex spatiotemporal patterns in systems ranging from biological systems to chemical reactions. Furthermore, it has been employed in population dynamics to model the growth and interactions of biological populations.Chinese Answer:库尔巴托夫方程由瓦列里·彼得罗维奇·库尔巴托夫最先提出,是一个非线性偏微分方程,广泛应用于反应扩散过程、连续介质力学和神经网络等各个领域。
PACC国际物理学分类表

如何查PACC代码?PACC代码是《Physics Abstracts,Classification and Contents》的缩略。
PACC专业代码是英国科学文摘(INSPEC)用于论文分类的代码。
按照论文的内容将其分为十大类有0000,1000,……5000,……9000表示,例如:凝聚物质由6000及7000表示,其中6000内包括凝聚物质的结构、热学和力学性质,而7000内包括凝聚物质的电子结构、电学、磁学和光学性质。
再仔细分则由6100……6200等表示,例如6100表示液体和固体结构。
而X射线晶体结构测定及精确化技术表示固体结构的测定包含在6100中,而用6110M来表示。
所以要查出某一论文的PACC专业代码,应先确定该论文主要内容属于哪一大类,就在那一大类中找出其代码,其次再找出该论文包括的其它次要内容的代码。
国际物理学分类表PACC(Physics Abstracts, Classification andContents)0000 总论 GENERAL0100 通讯、教育、历史和哲学 communication,education,history,andphilosophy0110 通报、消息和组织活动announcements, news, and organizational activities0110C 通报、消息和颁奖announcements, news, and awards 0110F 会议、演讲和学会conferences, lectures, and institutes 0110H 物理学组织活动physics organizational activities 0130 物理学文献及出版物physics literature and publications0130B 讲稿的出版(进修学院,暑期学校等)publications of lectures (advanced institutes, summer schools, etc.)0130C 会议录 conferenceproceedings 0130E 专著和著作集 monographs,andcollections 0130K 手册和字典handbooks and dictionaries0130L 物理数据、表格汇编collections of physical data, tables0130N 教科书 textbooks0130Q 报告、学位论文、论文reports, dissertations, theses0130R 评论及教学参考论文,资源通讯reviews and tutorial papers, resource letters0130T 书目 bibliographies 0140 教育 education0140D 课程设置与评价course design and evaluation0140E 中小学科学science in elementary and secondary school0140G 课程设置,教学方法,策略和评价curricula, teaching methods, strategies, and evaluation0140J 教师培训 teachertraining0150 教具(包括设备和实验及教学用材料)educational aids(inc.equipment, experiments andteaching approaches to subjects)0150F 视听教具、电影audio and visual aids, films0150H 计算机在教学中的使用instructional computer use0150K 试验理论和技术testing theory and techniques0150M 示范教学的实验和设备demonstration experiments and apparatus 0150P 实验室实验和设备laboratory experiments and apparatus0150Q 实验室课程设置、组织和评价laboratory course design, organization, and evaluation0150T 建筑物和设备 buildingsandfacilities 0155 普通物理 generalphysics 0160 传记、历史和个人笔记biographical, historical, and personal notes 0165 科学史history of science0170 科学哲学 philosophyofscience 0175 科学与社会 scienceandsociety 0190 其他一般论题other topics of general interest0200 物理学中的数学方法mathematical methods in physics0210 代数、集合论和图论algebra, set theory, and graph theory0220 群论(量子力学中的代数方法见0365;基本粒子物理学中的对称见1130)group theory(for algebraic methods in quantummechanics, see 0365; for symmetries inelementary particle physics, see 1130)0230 函数论,分析function theory, analysis0240 几何学、微分几何学和拓扑学(0400相对论与引力)geometry, differential geometry, andtopology(0400 relativity and gravitation)0250 概率论、随机过程和统计学(0500统计物理学)probability theory, stochastic processes, andstatistics(0500 statistical physics)0260 数字近似及分析numerical approximation and analysis0270 计算技术(数据处理与计算见0650)computational techniques(for data handling and computation, see 0650)0290 物理学中数学方法的其它论题other topics in mathematical methods in physics0300 经典及量子物理学;力学与场classical and quantum physics; mechanics and fields0320 离散系统的经典力学:一般数学问题(离散系统的应用经典力学见4610;天体力学见9510)classical mechanics of discrete systems: generalmathematical aspects ( for applied classicalmechanics of discrete systems, see 4610; forcelestial mechanics, see 9510)0330 狭义相对论 specialrelativity0340 连续介质经典力学:一般数学问题classical mechanics of continuous media: general mathematical aspects0340D 弹性力学的数学理论(4620连续介质力学,4630固体力学)mathematical theory of elasticity(4620 continuummechanics, and 4630 mechanics of solids)0340G 流体动力学:一般数学问题(4700流体动力学)fluid dynamics; general mathematicalaspects(4700 fluid dynamics)0340K 波和波传播:一般数学问题(4630M机械波和弹性波;4320一般线性声学)waves and wave propagation; generalmathematical aspects(4630M mechanical andelastic waves, 4320 general linear acoustics)0350 经典场论 classicalfieldtheory0350D 麦克斯韦理论:一般数学问题(应用经典电动力学,见4100)Maxwell theory: general mathematical aspects(forapplied classical electrodynamics, see 4100)0350K 其它具体经典场论other special classical field theories0365 量子论;量子力学(0530量子统计力学;相对论性波动方程,见1110)quantum theory; quantum mechanics(0530quantum statistical mechanics;for relativisticwave equations, see 1110)0365B 基础、测量理论、其它理论foundations, theory of measurement, miscellaneous theories0365C 形式论 formalism 0365D 泛函分析方法functional analytical methods0365F 代数方法(0220群论;3115分子物理学中计算方法)algebraic methods(02 20 group theory; 3115calculation methods in molecular physics)0365G 波动方程解:边界态solutions of wave equations: bound state0365N 非相对论性散射理论 nonrelativisticscatteringtheory 0365S 半经典理论和应用semiclassical theories and applications0367 量子信息 Quantuminformation 0370 量子场论(1110场论) theory of quantized fields(1110 field theory)0380 散射的一般理论(1120 S-矩阵论;1180相对论性散射)general theory of scattering(1120 S-matrix theory,and 1180 relativistic scattering)0400 相对论与引力(狭义相对论,见0330;相对论性天体物理学,见9530; 相对论性宇宙学,见9880)relativity and gravitation(for special relarivity,see0330;for relativistic astrophysics,see 9530;forrelativistic cosmology,see 9880)0420 广义相对论(0240几何学和拓扑学)general relativity (0240 geometry and topology) 0420C 基本问题和普通形式论fundamental problems and general formalism0420F 典型的形式论、拉氏函数和变分原理canonical formalism, Lagrangians, and variationalprinciples0420J 方程解solutions to equations0420M 守恒定律和运动方程conservation laws and equations of motion 0430 引力波和辐射:理论gravitational waves and radiation: theory0440 连续介质;电磁及其它混合引力系统continuous media; electromagnetic and othermixed gravitational systems0450 统一场论及其它引力理论unified field theories and other theories of gravitation0455 引力替代理论alternative theories of gravitation0460 引力的量子论quantum theory of gravitation0465 超引力 supergravity0470 黑洞物理学(参见9760L 黑洞) physics of black holes (see also 9760L black holes)0480 广义相对论的实验检验及引力辐射观测experimental tests of general relativity andobservations of gravitational radiation0485 中程力(包括第五和第六力) intermediate range forces (inc.fifth and sixth forces)0490 相对论和引力的其它论题other topics in relativity and gravitation0500 统计物理学和热力学(0250概率论、随机过程和统计学)statistical physics and thermodynamics(0250probability thory,stochastic processes,andstatistics)0520 统计力学 statisticalmechanics 0520D 分子运动论 kinetictheory0520G 经典系综论classical ensemble theory0530 量子统计力学(6700量子流体;7100凝聚物质的电子态)quantum statistic al mechanics(6700 quantumfluids, and 7100 electron states in condensedmatter)0530C 量子系综论quantum ensemble theory0530F 费米子系统和电子气Fermion systems and electron gas 0530J 玻色子系统 Bosonsystems0530L 任意子和仲统计学(量子统计力学)anyons and parastatistics (quantum statistical mechanics)0540 涨落现象、随机过程和布朗运动fluctuation phenomena, random processes, and Brownian motion0545 混沌系统的理论和模型(流体系统中的混沌,见4752)theory and models of chaotic systems(for chaos inflowing systems,see 4752)0547 非线性动力学系统和分岔(流体系统中的分岔,见4752)nonlinear dynamical systems and bifurcations(bifurcations in flowing systems,see 4752)0550 点阵理论和统计学;伊辛问题(7510H伊辛模型)lattice theory and statistics; Ising problems(7510HIsing models)0555 分形(流体系统中的分形,见4752)fractals (fractals in flowing systems,see 4752) 0560 输运过程:理论 transportprocesses:theory 0565 自组织系统 Self-organizedsystems0570 热力学(4460热力学过程;6400状态方程,相平衡和相变;6500凝聚物质的热性质 ; 化学热力学,见8260)thermodynamics(4460 thermodynamic processes;6400 equation s of state, phase equilibria andphase transitions; 6500 thermal properties ofcondensed matter;for chemical thermodynamics,see 8260)0570C 热力学函数及状态方程thermodynamic functions and equations of state0570F 相变:一般问题phase transitions: general aspects 0570J 临界点现象critical point phenomena0570L 非平衡热力学、不可逆过程(3430势能表面;8200物理化学)nonequilibrium thermodynamics, irreversibleprocesses(3430 potential energy surfaces, 8200physical chemistry)0580 经济物理学 Econophysics0590 统计物理学和热力学的其它论题other topics in statistical physics and thermodynamics0600 测量科学、普通实验室技术及测试设备系统Measurement science, general laboratorytechniques, and instrumentation systems0620 基本度量学 metrology 0620D 测量与误差理论measurement and error theory0620F 单位 units 0620H 测量标准和校正measurement standards and calibration 0620J 基本常数测定determination of fundamental constants 0630 基本变量测量measurement of basic variables0630C 空间变量测量(包括空间延伸的所有变量如:直径、重量、厚度、位移、表面拓扑学、粒子尺寸、弥散系统区)spatial variables measurement(inc.measurementof all variables extending in space e.g. diameter,weight, thickness, displacement , surfacetopography, particle size, area of dispersesystems)0630E 质量与密度的测量mass and density measurement0630F 时间与频率的测量(天文学方面的,见9570)time and frequency measurement(for astronomicalaspects see 9570)0630G 速度、加速度和转动测量(流速测量,见4780)velocity, acceleration and rotationmeasurement(for flow velocity measurement see4780)0630L 基本电磁变量测量(0750电学仪器和技术)measurement of basic electromagneticvariables(0750 electrical instruments andtechniques)0630M 机械变量测量(包括弹性模量,力,冲击,应变,应力,力矩和振动)(压力测量,见0630N;声学变量测量,见4385D;固体力学测量,见4630R;粘度测量,见4780;材料试验,8170)measurement of mechanical variables(inc.elasticmoduli,force,shock ,strain,stress,torque,andvibration)(for pressure measurement,see0630N;for acoustic variables measurement,see4385D;for measurement in the mechanics ofsolids, see 4630R;for viscosity measurement,see4780;for materials testing,see 8170)0630N 压力测量(真空测量,见0730D;高压技术,见0735)pressure measurement(for vacuum measurement,see 0730D;for high-pressure techniques, see0735)0650 数据处理和计算(0270计算技术;2980核信息处理;光学数据处理,存贮及检索, 见423 0;地球物理数据采集和存贮,见9365)data handling and computation(0270computational techniques; 2980 nuclearinformation processing;for optical dataprocessing , storage and retrieval see 4230; forgeophysical data acquisition and storage see9365)0650D 数据搜集、处理、记录、数据显示(含数显技术)data gathering, processing, and recording, datadisplays (including digital techniques)0650M 计算装置与技术computing devices and techniques0660 实验室技术 laboratorytechniques 0660E 样品制备 samplepreparation0660J 高速技术(微秒到微微秒) high speed techniques (microsecond to picosecond)0660S 微检验装置、微定位器和切片机micromanipulators, micropositioners , and microtomes0660V 车间技术(焊接、机械加工、润滑作用和轴承等)workshop techniques ( welding, machining,lubrication, bearings, etc.)0660W 安全(2880辐射监测和防护;8760M辐射剂量测定法;8760P辐射防护)safety( 2880 radiation monitoring and protection,8760M radiation dosimetry, 8760P radiationprotection)0670 普通测试设备 generalinstrumentation 0670D 敏感元件和探测器sensing and detecting devices0670E 试验设备 testingequipment 0670H 显示、记录与指示器display, recording, and indicating instrument s0670M 换能器(电磁辐射换能器见0762;声换能器见4388;液流换能器见4780)transducers(for electromagnetic radiationtransducers see 0762; for acoustic transducers see4388; for flow transducers see 4780)0670T 伺服及控制装置servo and control devices0690 测量科学、普通实验室技术及测试设备系统中的其它论题other topics in measurement science, generallaboratory techniques and instrumentationsystems0700 物理学中普遍使用的专用测试设备与技术(各分支学科的专用测试设备与技术入各自的分支学科)specific instrumentation and techniques of generaluse in physics(within each subdiscipline forspecialized instrumentation and techniques)0710 机械仪器与测量方法(固体力学测量见4630R;材料试验见8170)mechanical instruments and measurementmethods(for measurement in the mechanics of solids, see 4630R; for materials testing, see 8170)0710C 微机械器件和系统(微光学器件和技术,见4283)micromechanical devices and systems (formicrooptical devices and technology,see 4283)0710F 隔振 vibrationisolation0710Y 其他机械仪器和技术(包括摆、陀螺仪、离心器)other mechanical instruments andtechniques(inc.pendulums,gyroscopes,centrifuges)0720 热仪器和技术(4450物质的热性质;4460热力学过程;热辐射的辐射度学和检测, 见 0760D和0762)thermal instruments and techniques(4450 thermalproperties of matter, 4460 thermodynamicprocesses;for radiometry and detection of thermalradiation see 0760D and 0762)0720D 温度测量 thermometry 0720F 量热学 calorimetry 0720H 加热炉 furnaces0720K 高温技术及测试设备;测高温术high temperature techniques and instrumentation; pyrometry0720M 低温实验法 cryogenics 0725 测湿法 hygrometry0730 真空产生与真空技术(包括低于1个大气压的压力;稀薄气体动力学入4745;8115 G 真空淀积)vacuum production and techniques(inc.pressuresbelow 1atmosphere; 4745 rarefied gas dynamics;8115G vacuum deposition)0730B 排空能力、除气、剩余气体evacuating power, degasification, residual gas 0730C 真空泵 vacuumpumps 0730D 真空计 vacuummeters 0730G 真空设备及试验方法vacuum apparatus and testing methods0730K 辅助设备、器件及材料auxiliary apparatus, hardware and materials0735 高压产生与技术(包括大于1个大气压的压力)high pressure production and techniques(inc.pressures above 1 atmosphere)0750 电学仪器及技术electrical instruments and techniques 0755 磁测量仪器及技术magnetic instruments and techniques0758 磁共振谱仪、辅助仪器和技术(6116N电子顺磁共振和核磁共振测定)magnetic resonance spectrometers, auxiliaryinstruments and techniques(6116N EPR and NMRdeterminations)0760 光学仪器和技术(辐射探测见0762;光谱学和光谱计见0765;全息术见4240;光源和标准见4272;光学透镜和反射系统见4278;光学器件、技术和应用见4280;光学试验和加工技术见4285;辐射谱仪和光谱技术见2930;辐射测量、检测和计数见2970)optical instruments and techniques(for radiationdetection, see 0762; for spectroscopy andspectrometers, see 0765; for holography, see4240; for optical sources and standards, see 4272;for optical lens and mirror systems, see 4278; foroptical devices , techniques and applications, see4280; for optical testing and workshop techniques,see 4285; for radiation spectrometers andspectroscopic techniques, see 29 30; for radiationmeasurement, detection and counting, see 2970)0760D 光度学和辐射度学(包括色度学,辐射探测入0762)photometry and radio metry(inc.colorimetry;0762detection of radiation)0760F 偏振测量术与椭园偏振测量术 polarimetryandellipsometry0760H 折射测量术与反射测量术 refractometryandreflectometry 0760L 干涉量度学 interferometry 0760P 光学显微术 opticalmicroscopy0762 辐射探测(测辐射热计、光电管、红外波与亚毫米波探测)detection of radiation (bolometers, photoelectriccells, IR. and submillimetre waves detection)0765 光谱学与光谱计(包括光声谱术) optical spectroscopy and spectrometers(inc.photoacoustic spectroscopy)0765E 紫外和可见光谱学与光谱仪UV and visible spectroscopy and spectrometers 0765G 红外光谱学与光谱仪IR spectroscopy and spectrometers0768 照相术、照相仪器与技术(光敏材料参见4270;照相过程的化学参见8250)photography, photographic instruments andtechniques(for light sensitive materials see also4270 for chemistry of photographic process seealso 8250)0775 质谱仪与质谱测定技术(质谱化学分析见8280)mass spectrometers and m ass spectrometrytechniques(for mass spectroscopic chemicalanalysis, see 8280)0777 粒子束的产生与处理;(2925基本粒子和核物理中的粒子源和靶;4180粒子束和粒子光学)particle beam production and handling;(2925particle sources and targets in elementary particle and nuclear physics, 4180 particle beamsand particle optics)0779 扫描探针显微术及其相关技术(包括扫描隧道显微术,原子力显微术、磁力显微术,摩擦力显微术,和近场扫描光学显微术,(结构测定方面,参见6116P)scanning prob e microscopy and relatedtechniques(inc.scanning tunnellingmicroscopy,atomic force microscopy,magneticforce microscopy,friction force microscopy,andnear field scanning opticalmicroscopy)(structure determination aspects, seealso 6116P)0780 电子与离子显微镜及其技术(6116D凝聚物质中的电子显微术;6116F凝聚物质中的场离子显微术)electron and ion microscopes andtechniques(6116D in condensed matter electronmicroscopy, 6116F field ion microscopy)0781 电子和离子谱仪及其相关技术(参见2930辐射谱仪和光谱技术)electron and ion spectrometers and relatedtechniques(see also 2930 radiation spectrometersan d spectroscopic techniques)0785 X射线与γ射线仪器与技术(包括穆斯堡尔谱仪和技术)X-ray, gamma-ray instruments and techniques(inc.Moessbauer spectrometers and technique s)0788 粒子干涉量度学和中子仪器(粒子束的产生与处理,参见0777;中子谱仪,参见 2930H ,原子干涉量度学,参见3580粒子光学,参见4180)particle interferometry and neutroninstrumentation(for particle beam production andhandling,see 0777;for neutron spectrometers,seealso 2930H;for atomic interferometry,see also3580;for particle optics,see also 4180)0790 专用设备中的其它论题other topics in specialised instrumentation1000 基本粒子物理与场(宇宙线见9440;高能实验技术和设备见 2900)THE PHYSICS OF ELEMENTARY PARTICLESAND FIELDS(for cosmic rays ,see 9440;for highenergy experimental techniques andinstrumentation, see 2900)1100 场和粒子的一般理论(0365量子力学;0370量子场论;0380散射的一般理论)general theory of fields and particles(0365quantum mechanics, 0370 theory of quantizedfields, 0380 general theory of scattering)1110 场论 fieldtheory 1110C 公理法 axiomaticapproach 1110E 拉氏函数和哈密顿函数法Lagrangian and Hamiltonian approach1110G 重正化 renormalization 1110J 渐近问题与特性asymptotic problems and properties1110L 非线性或非局域理论及模型nonlinear or nonlocal theories and models1110M 史文格源理论 Schwingersourcetheory 1110N 规范场论gauge field theories1110Q 相对论性波动方程relativistic wave equations1110S 束缚与非稳定态;贝特-沙耳皮特方程bound and unstable states; Bethe-Salpeterequations1110W 有限温度场论finite temperature field theory1117 弦理论和其他扩展物质理论(包括超弦和膜)theories of strings and other extendedobjects(inc.superstrings and membranes)1120 S-矩阵论 S-matrixtheory 1120D 散射矩阵和微扰论scattering matrix and perturbation theory1120F 色散关系和S矩阵的分析特性dispersion relations and analytic properties of the S-matrix1130 对称和守恒定律(0220群论) symmetry and conservation laws(0220 group theory)1130C 洛伦兹与庞加莱不变性Lorentz and Poincare invariance1130E 电荷共轭、宇称、时间反演和其它分立对称charge conjugation, parity, time reversal and otherdiscrete symmetries1130J SU(2)和SU(3)对称SU(2) and SU(3) symmetries1130K SU(4)对称 SU(4)symmetry 1130L 其他内部对称和高度对称other internal and higher symmetries1130N 非线性对称和动力学对称性(谱生成对称)nonlinear and dynamical symmetries (spectrum generating symmetries)1130P 超对称 supersymmetry1130Q 自发性对称破缺spontaneous symmetry breaking1130R 手征对称 chiralsymmetries 1140 流及其特性currents and their properties1140D 流的一般理论general theory of currents1140F 流代数的拉格朗日算法Lagrangian approach to current algebras1140H 部分守恒轴矢量流partially conserved axial vector currents 1150 色散关系与求和定则dispersion relations and sum rules1150E n/d法 n/dmethod 1150G 靴襻 bootstraps 1150J 交叉对称 crossingsymmetries 1150L 求和定则 sumrules1150N 多变量色散关系(包括曼德尔斯坦表象)multivariable dispersion relations(inc.Mandelstamrepresentation)1160 复合角动量;雷其(理论)体系(0380一般散射理论;1240强相互作用中的复合角动量)complex angular momentum; Reggeformalism(0380 general theory of scattering, 1240in strong interactions)1180 相对论性散射理论(0380一般散射理论)relativistic scattering theory (0380 general theoryof scattering)1180C 运动特性(螺旋性和不变振幅、运动奇异性等)kinematical properties (helicity and invariantamplitudes, kinematic singularities, etc.)1180E 部分波分析 partial wave analysis1180F 近似法(程函近似法,变分原理等) approximations (eikonal approximation, variational principles, etc)1180G 多道散射 multichannelscattering 1180J 多体散射和Faddeev方程Many-body scattering and Faddeev equation 1180L 多次散射 multiplescattering 1190 一般场论和粒子理论的其它论题other topics in general field and particle theory1200 具体理论和相互作用模型;粒子分类系统specific theories and interaction models; particlesystematics1210 统一场论和模型unified field theories and models1210B 电弱理论 electroweaktheories 1210C 统一化标准模型standard model of unification1210D 标准模型以外的统一模型(包括GUTS,颜色模型和SUSY模型)unified models beyond the standardmodel(inc.GUTS,technicolour and SUSY models)1220 电磁相互作用模型models of electromagnetic interactions1220D 量子电动力学的具体计算和极限specific calculations and limits of quantum electrodynamics1220F 量子电动力学的实验检验experimental tests of quantum electrodynamics 1225 引力相互作用模型(0460引力的量models for gravitational interactions(0460子论) quantum theory of gravitation)1230 弱相互作用模型models of weak interactions1230C 中子流 neutralcurrents 1230E 中间玻色子 intermediatebosons 1235 粒子的复合模型composite models of particles1235C 量子色动力学的一般特性(动力学,禁闭等)general properties of quantum chromodynamics(dynamics, confinement, etc.)1235E 量子色动力学在粒子特性和反应中的应用applications of quantum chromodynamics toparticle properties and reactions1235H 粒子的结构和反应的唯象复合模型(部分子模型,口袋模型等)phenomenological composite models of particlestructure and reactions (partons, bags, etc.)1235K 其它复合模型(包括复合夸克模型和轻子模型)other composite models( posite quarksand leptons)1240 强相互作用模型models of strong interactions1240E 统计模型 statisticalmodels1240F 靴襻模型 bootstrapmodels1240H 二重性和双关模型duality and dual models1240K 强子分类方案 hadronclassificationschemes1240M 复合角动量平面;雷其极点和割线(雷其子)(1160复合角动量,雷其体系的一般理论)complex angular momentum plane; Regge polesand cuts (Reggeons)(1160 for general theory)1240P 吸收模型,光学模型和程函模型(衍射和衍射生成模型见1240S)absorptive, optical, and eikonal models(fordiffraction and diffractive production models, see1240S)1240Q 势模型 potentialmodels1240R 边缘碰撞模型(一个或多个粒子交换) peripheral models (one or more particle exchange)1240S 多重边缘碰撞模型和多雷其模型(包括衍射和衍射生成模型)multiperipheral and multi Reggemodels(inc.diffraction and diffractive productionmodels)1240V 矢量介子优势 Vector-mesondominance 1270 强子质量公式hadron mass formulas1290 其它各种理论设想与模型miscellaneous theoretical ideas and models1300 具体基本粒子反应和唯象论specific elementary particle reactions and phenomenology1310 轻子间的弱相互作用和电磁相互作用weak and electromagnetic interactions of leptons1315 中微子相互作用(包括宇宙射线相互作用)neutrino interactions(inc.interactions involvingcosmic rays)1320 介子的轻子与半轻子衰变leptonic and semileptonic decays of mesons1320C π衰变 pidecays1320E K衰变 Kdecays1320G Ψ/J介子、Υ介子、Φ介子psi/J, upsilon, phi mesons1320H B介子轻子/半轻子衰变 Bmesonleptonic/semileptonicdecays 1320I f介子轻子/半轻子衰变 fmesonleptonic/semileptonicdecays 1320J 其它介子衰变other meson decays1325 介子的强子衰变 hadronicdecaysofmesons 1330 重子的衰变 decaysofbaryons1330C 轻子与半轻子衰变leptonic and semileptonic decays1330E 强子衰变 hadronicdecays 1335 轻子的衰变 decaysofleptons1338 中间玻色子和希格斯玻色子的衰变decays of intermediate and Higgs Bosons1340 电磁过程与特性electromagnetic processes and properties1340D 电磁质量差electromagnetic mass differences1340F 电磁形状因子、电矩和磁矩electromagnetic form factors; electric and magnetic moments1340H 电磁衰变 electromagneticdecays1340K 强相互作用和弱相互作用过程的电磁修正electromagnetic corrections to strong and weakinteraction processes1360 光子及带电轻子与强子的相互作用(中微子相互作用见1315)photon and charged lepton interactions withhadrons(for neutrino interactions, see 1315)1360F 弹性散射与康普顿散射elastic and Compton scattering1360H 总截面和单举(反应)截面(包括深度非弹性过程)total and inclusive crosssections(inc.deep-inelastic processes)1360K 介子产生 mesonproduction 1360M 介子共振产生 Meson-resonanceproduction 1360P 重子和重子共振产生baryon and baryon resonance production1365 电子-正电子碰撞产生强子hadron production by electron-positron collisions1375 强子诱发的低能和中能反应及散射(能量≤10GeV见1385)Hadron-induced low energy and intermediate energy reactions and scattering, energy ≤10GeV( for higher energies, see 1385)1375C 核子-核子相互作用,包括反核子和氘核等(能量≤10GeV;核中的核子-核子相互作用见2130)Nucleon-nucleon interactions, includingantinucleon, deuteron, etc. (energy ≤10GeV)(for n-n interactions in nuclei, see 2130)1375E 超子-核子相互作用(能量≤10GeV)Hyperon-nucleon interactions (energy ≤10 GeV)1375G π介子-重子相互作用(能量≤10GeV) Pion-baryon interactions (energy ≤10 GeV)1375J K介子-重子相互作用(能量≤10GeV) Kaon-baryon interactions (energy ≤10 GeV)1375L 介子-介子相互作用(能量≤10GeV)Meson-meson interactions (energy ≤10 GeV) 1380 光子-光子相互作用和散射Photon-photon interactions and scattering1385 强子诱发的高能和超高能相互作用(能量>10GeV)(低能情况见1375)Hadron-induced high-energy andsuper-high-energy interactions, energy > 10GeV(for low energies, see 1375)1385D 弹性散射(能量=10GeV) elastic scattering (energy = 10 GeV)1385F 非弹性散射、双粒子终态(能量>10GeV)inelastic scattering, two-particle final states(energy > 10 GeV)1385H 非弹性散射、多粒子终态(能量>10GeV)inelastic scattering, many-particle final states(energy>10GeV)1385K 单举反应,包括总截面(能量>10GeV)inclusive reactions, including total cross sections,(energy > 10 GeV)1385M 宇宙射线相互作用(9440宇宙线) cosmic ray interactions(9440 cosmic rays)1385N 强子诱发的高能相互作用(能量>1TeV)hadron induced very high energyinteractions(energy>1 TeV)1387 大Q2基本粒子相互作用中的射流jets in large-Q2 elementary particle interactions 1388 相互作用和散射中的极化polarisation in interactions and scattering1390 基本粒子的具体反应及唯象论的其它论题other topics in specific reactions andphenomenology of elementary particles1400 具体粒子的性质与共振properties of specific particles and resonances1420 重子与重子共振(包括反粒子) baryons and baryon resonances(inc.antiparticles) 1420C 中子 neutrons1420E 质子 protons1420G s=0时的重子共振baryon resonances with s=01420J 超子和超子共振hyperons and hyperon resonances1420P 双重子 dibaryons1440 介子和介子共振mesons and meson resonances1440D π介子 pimesons1440F K介子 Kmesons1440K Ρ介子、Ω介子和η介子rho, omega, and eta mesons1440L d介子和F介子 d and F mesons1440N Ψ/J介子、Υ介子、Φ介子psi/J, upsilon, phi mesons1440P 其它介子 othermesons1460 轻子 leptons1460C 电子和正电子 electronsandpositrons 1460E μ介子 muons1460G 中微子 neutrinos1460J 重轻子 heavyleptons 1480 其它粒子和假设粒子other and hypothetical particles1480A 光子 photons1480D 夸克和胶子 quarksandgluons 1480F 中间玻色子 intermediateBosons 1480H 磁单极子 magneticmonopoles1480J 超对称粒子(包括标量粒子,超粒子和超离子)Supersymmetric particles(inc.scalarparticles,superparticles and superions)1480K 其它(包括快子) others(inc.tachyons)2000 核物理学 NUCLEARPHYSICS 2100 核结构 nuclearstructure2110 核的一般和平均性质;核能级性质(按质量范围分类的具体核的性质见2700)general and average properties of nuclei;properties of nuclear energy levels(for propertiesof specific nuclei listed by mass ranges, see 2700)2110D 结合能和质量binding energy and masses2110F 形状、电荷、半径和形状因子shape, charge, radius and form factor s2110H 自旋、宇称和同位旋spin, parity, and isobaric spin2110J 谱因子 spectroscopicfactors 2110K 电磁矩 electromagneticmoments 2110M 能级密度和结构level density and structure2110P 单粒子能级结构single particle structure in levels2110R 集团能级结构(包括旋转能带) collective structure in levels(inc.rotational bands) 2110S 库仑效应 Coulombeffects2130 核力(1375C核子-核子相互作用) nuclear forces(1375C nucleon-nucleon interactions)2140 少核子系统 Few-nucleonsystems2160 核结构模型与方法(强子的原子和分子见3610)nuclear structure models and methods(forhadronic atoms and molecules, see 3610)2160C 壳层模型 shellmodel2160E 集体模型 collectivemodels 2160F 群论模型models based on group theory2160G 集团模型 clustermodels 2160J 哈特里-福克和随机-相位近似Hartree-Fock and random-phase approximations 2165 核物质 nuclearmatter 2180 超核 hypernuclei 2190 核结构的其它论题other topics in nuclear structure2300 放射性和电磁跃迁(8255放射化学)radioactivity and electromagnetic transitions(8255 radiochemistry)2320 电磁跃迁 electromagnetictransitions 2320C 寿命和跃迁几率lifetimes and transition probabilities2320E 角分布和校正测量angular distribution and correlation measurements2320G 多极混合比率 multipolemixingratios 2320J 多极矩阵元素 multipolematrixelements 2320L γ跃迁和能级gamma transitions and level energies2320N 内转换和核外效应internal conversion and extranuclear effects 2320Q 核共振荧光nuclear resonance fluorescence2340 β衰变;电子与μ子俘获beta decay; electron and muon capture2340B 弱相互作用和β衰变的轻子特性weak interaction and lepton aspects of beta decay2340H 核矩阵元和从β衰变推断核结构nuclear matrix elements and nuclear structure inferred from beta decay2360 α衰变 alphadecay 2390 核衰变和放射性的其它论题other topics in nuclear decay and radioactivity 2400 核反应和散射:总论nuclear reactions and scattering:general2410 核反应和散射模型与方法nuclear reaction and scattering models and methods2410D 耦合道和多体论方法coupled channel and many body theory methods2410F 平面和扭曲波玻恩近似法Plane- and distorted-wave Born approximations 2410H 光学模型和衍射模型optical and diffraction models2430 共振反应与散射resonance reactions and scattering2430C 巨共振 giantresonances 2430F 同位旋相似共振 isobaricanalogresonances 2450 直接反应 directreactions 2460 统计理论和涨落statistical theory and fluctuations2470 反应和散射中的极化polarization in reactions and scattering2475 裂变的一般性质general properties of fission2485 原子核和核形成过程的夸克模型quark models in nuclei and nuclear processes2490 核反应和散射的其它论题:一般问题other topics in nuclear reactions and scattering:general2500 核反应和散射:具体反应nuclear reactions and scattering:specific reactions2510 少核子系统的核反应与散射nuclear reactions and scattering involving few-nucleon systems2520 光致核反应和光子散射 photonuclearreactions and photon scattering 2530 轻子诱发反应与散射Lepton-induced reactions and scattering 2530C 电子和正电子反应与散射electron and positron reactions and scattering 2530E μ介子反应和散射muon reactions and scattering2530G 中微子反应和散射neutrino reactions and scattering。
量子力学索引英汉对照
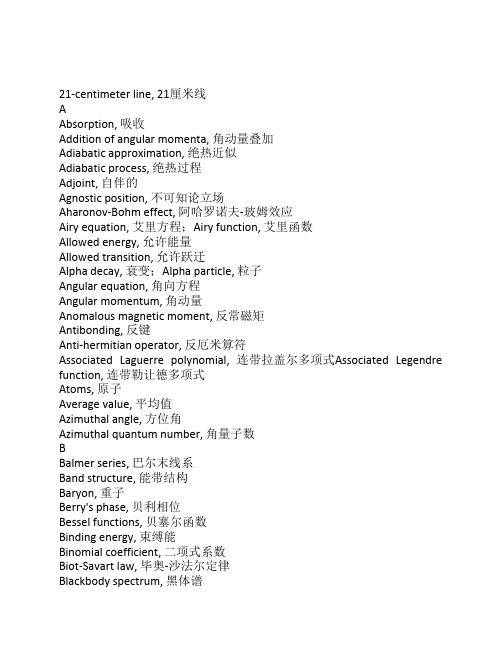
21-centimeter line, 21厘米线AAbsorption, 吸收Addition of angular momenta, 角动量叠加Adiabatic approximation, 绝热近似Adiabatic process, 绝热过程Adjoint, 自伴的Agnostic position, 不可知论立场Aharonov-Bohm effect, 阿哈罗诺夫-玻姆效应Airy equation, 艾里方程;Airy function, 艾里函数Allowed energy, 允许能量Allowed transition, 允许跃迁Alpha decay, 衰变;Alpha particle, 粒子Angular equation, 角向方程Angular momentum, 角动量Anomalous magnetic moment, 反常磁矩Antibonding, 反键Anti-hermitian operator, 反厄米算符Associated Laguerre polynomial, 连带拉盖尔多项式Associated Legendre function, 连带勒让德多项式Atoms, 原子Average value, 平均值Azimuthal angle, 方位角Azimuthal quantum number, 角量子数BBalmer series, 巴尔末线系Band structure, 能带结构Baryon, 重子Berry's phase, 贝利相位Bessel functions, 贝塞尔函数Binding energy, 束缚能Binomial coefficient, 二项式系数Biot-Savart law, 毕奥-沙法尔定律Blackbody spectrum, 黑体谱Bloch's theorem, 布洛赫定理Bohr energies, 玻尔能量;Bohr magneton, 玻尔磁子;Bohr radius, 玻尔半径Boltzmann constant, 玻尔兹曼常数Bond, 化学键Born approximation, 玻恩近似Born's statistical interpretation, 玻恩统计诠释Bose condensation, 玻色凝聚Bose-Einstein distribution, 玻色-爱因斯坦分布Boson, 玻色子Bound state, 束缚态Boundary conditions, 边界条件Bra, 左矢Bulk modulus, 体积模量CCanonical commutation relations, 正则对易关系Canonical momentum, 正则动量Cauchy's integral formula, 柯西积分公式Centrifugal term, 离心项Chandrasekhar limit, 钱德拉赛卡极限Chemical potential, 化学势Classical electron radius, 经典电子半径Clebsch-Gordan coefficients, 克-高系数Coherent States, 相干态Collapse of wave function, 波函数塌缩Commutator, 对易子Compatible observables, 对易的可观测量Complete inner product space, 完备内积空间Completeness, 完备性Conductor, 导体Configuration, 位形Connection formulas, 连接公式Conservation, 守恒Conservative systems, 保守系Continuity equation, 连续性方程Continuous spectrum, 连续谱Continuous variables, 连续变量Contour integral, 围道积分Copenhagen interpretation, 哥本哈根诠释Coulomb barrier, 库仑势垒Coulomb potential, 库仑势Covalent bond, 共价键Critical temperature, 临界温度Cross-section, 截面Crystal, 晶体Cubic symmetry, 立方对称性Cyclotron motion, 螺旋运动DDarwin term, 达尔文项de Broglie formula, 德布罗意公式de Broglie wavelength, 德布罗意波长Decay mode, 衰变模式Degeneracy, 简并度Degeneracy pressure, 简并压Degenerate perturbation theory, 简并微扰论Degenerate states, 简并态Degrees of freedom, 自由度Delta-function barrier, 势垒Delta-function well, 势阱Derivative operator, 求导算符Determinant, 行列式Determinate state, 确定的态Deuterium, 氘Deuteron, 氘核Diagonal matrix, 对角矩阵Diagonalizable matrix, 对角化Differential cross-section, 微分截面Dipole moment, 偶极矩Dirac delta function, 狄拉克函数Dirac equation, 狄拉克方程Dirac notation, 狄拉克记号Dirac orthonormality, 狄拉克正交归一性Direct integral, 直接积分Discrete spectrum, 分立谱Discrete variable, 离散变量Dispersion relation, 色散关系Displacement operator, 位移算符Distinguishable particles, 可分辨粒子Distribution, 分布Doping, 掺杂Double well, 双势阱Dual space, 对偶空间Dynamic phase, 动力学相位EEffective nuclear charge, 有效核电荷Effective potential, 有效势Ehrenfest's theorem, 厄伦费斯特定理Eigenfunction, 本征函数Eigenvalue, 本征值Eigenvector, 本征矢Einstein's A and B coefficients, 爱因斯坦A,B系数;Einstein's mass-energy formula, 爱因斯坦质能公式Electric dipole, 电偶极Electric dipole moment, 电偶极矩Electric dipole radiation, 电偶极辐射Electric dipole transition, 电偶极跃迁Electric quadrupole transition, 电四极跃迁Electric field, 电场Electromagnetic wave, 电磁波Electron, 电子Emission, 发射Energy, 能量Energy-time uncertainty principle, 能量-时间不确定性关系Ensemble, 系综Equilibrium, 平衡Equipartition theorem, 配分函数Euler's formula, 欧拉公式Even function, 偶函数Exchange force, 交换力Exchange integral, 交换积分Exchange operator, 交换算符Excited state, 激发态Exclusion principle, 不相容原理Expectation value, 期待值FFermi-Dirac distribution, 费米-狄拉克分布Fermi energy, 费米能Fermi surface, 费米面Fermi temperature, 费米温度Fermi's golden rule, 费米黄金规则Fermion, 费米子Feynman diagram, 费曼图Feynman-Hellman theorem, 费曼-海尔曼定理Fine structure, 精细结构Fine structure constant, 精细结构常数Finite square well, 有限深方势阱First-order correction, 一级修正Flux quantization, 磁通量子化Forbidden transition, 禁戒跃迁Foucault pendulum, 傅科摆Fourier series, 傅里叶级数Fourier transform, 傅里叶变换Free electron, 自由电子Free electron density, 自由电子密度Free electron gas, 自由电子气Free particle, 自由粒子Function space, 函数空间Fusion, 聚变Gg-factor, g-因子Gamma function, 函数Gap, 能隙Gauge invariance, 规范不变性Gauge transformation, 规范变换Gaussian wave packet, 高斯波包Generalized function, 广义函数Generating function, 生成函数Generator, 生成元Geometric phase, 几何相位Geometric series, 几何级数Golden rule, 黄金规则"Good" quantum number, "好"量子数"Good" states, "好"的态Gradient, 梯度Gram-Schmidt orthogonalization, 格莱姆-施密特正交化法Graphical solution, 图解法Green's function, 格林函数Ground state, 基态Group theory, 群论Group velocity, 群速Gyromagnetic railo, 回转磁比值HHalf-integer angular momentum, 半整数角动量Half-life, 半衰期Hamiltonian, 哈密顿量Hankel functions, 汉克尔函数Hannay's angle, 哈内角Hard-sphere scattering, 硬球散射Harmonic oscillator, 谐振子Heisenberg picture, 海森堡绘景Heisenberg uncertainty principle, 海森堡不确定性关系Helium, 氦Helmholtz equation, 亥姆霍兹方程Hermite polynomials, 厄米多项式Hermitian conjugate, 厄米共轭Hermitian matrix, 厄米矩阵Hidden variables, 隐变量Hilbert space, 希尔伯特空间Hole, 空穴Hooke's law, 胡克定律Hund's rules, 洪特规则Hydrogen atom, 氢原子Hydrogen ion, 氢离子Hydrogen molecule, 氢分子Hydrogen molecule ion, 氢分子离子Hydrogenic atom, 类氢原子Hyperfine splitting, 超精细分裂IIdea gas, 理想气体Idempotent operaror, 幂等算符Identical particles, 全同粒子Identity operator, 恒等算符Impact parameter, 碰撞参数Impulse approximation, 脉冲近似Incident wave, 入射波Incoherent perturbation, 非相干微扰Incompatible observables, 不对易的可观测量Incompleteness, 不完备性Indeterminacy, 非确定性Indistinguishable particles, 不可分辨粒子Infinite spherical well, 无限深球势阱Infinite square well, 无限深方势阱Inner product, 内积Insulator, 绝缘体Integration by parts, 分部积分Intrinsic angular momentum, 内禀角动量Inverse beta decay, 逆衰变Inverse Fourier transform, 傅里叶逆变换KKet, 右矢Kinetic energy, 动能Kramers' relation, 克莱默斯关系Kronecker delta, 克劳尼克LLCAO technique, 原子轨道线性组合法Ladder operators, 阶梯算符Lagrange multiplier, 拉格朗日乘子Laguerre polynomial, 拉盖尔多项式Lamb shift, 兰姆移动Lande g-factor, 朗德g-因子Laplacian, 拉普拉斯的Larmor formula, 拉摩公式Larmor frequency, 拉摩频率Larmor precession, 拉摩进动Laser, 激光Legendre polynomial, 勒让德多项式Levi-Civita symbol, 列维-西维塔符号Lifetime, 寿命Linear algebra, 线性代数Linear combination, 线性组合Linear combination of atomic orbitals, 原子轨道的线性组合Linear operator, 线性算符Linear transformation, 线性变换Lorentz force law, 洛伦兹力定律Lowering operator, 下降算符Luminoscity, 照度Lyman series, 赖曼线系MMagnetic dipole, 磁偶极Magnetic dipole moment, 磁偶极矩Magnetic dipole transition, 磁偶极跃迁Magnetic field, 磁场Magnetic flux, 磁通量Magnetic quantum number, 磁量子数Magnetic resonance, 磁共振Many worlds interpretation, 多世界诠释Matrix, 矩阵;Matrix element, 矩阵元Maxwell-Boltzmann distribution, 麦克斯韦-玻尔兹曼分布Maxwell's equations, 麦克斯韦方程Mean value, 平均值Measurement, 测量Median value, 中位值Meson, 介子Metastable state, 亚稳态Minimum-uncertainty wave packet, 最小不确定度波包Molecule, 分子Momentum, 动量Momentum operator, 动量算符Momentum space wave function, 动量空间波函数Momentum transfer, 动量转移Most probable value, 最可几值Muon, 子Muon-catalysed fusion, 子催化的聚变Muonic hydrogen, 原子Muonium, 子素NNeumann function, 纽曼函数Neutrino oscillations, 中微子振荡Neutron star, 中子星Node, 节点Nomenclature, 术语Nondegenerate perturbationtheory, 非简并微扰论Non-normalizable function, 不可归一化的函数Normalization, 归一化Nuclear lifetime, 核寿命Nuclear magnetic resonance, 核磁共振Null vector, 零矢量OObservable, 可观测量Observer, 观测者Occupation number, 占有数Odd function, 奇函数Operator, 算符Optical theorem, 光学定理Orbital, 轨道的Orbital angular momentum, 轨道角动量Orthodox position, 正统立场Orthogonality, 正交性Orthogonalization, 正交化Orthohelium, 正氦Orthonormality, 正交归一性Orthorhombic symmetry, 斜方对称Overlap integral, 交叠积分PParahelium, 仲氦Partial wave amplitude, 分波幅Partial wave analysis, 分波法Paschen series, 帕邢线系Pauli exclusion principle, 泡利不相容原理Pauli spin matrices, 泡利自旋矩阵Periodic table, 周期表Perturbation theory, 微扰论Phase, 相位Phase shift, 相移Phase velocity, 相速Photon, 光子Planck's blackbody formula, 普朗克黑体辐射公式Planck's constant, 普朗克常数Polar angle, 极角Polarization, 极化Population inversion, 粒子数反转Position, 位置;Position operator, 位置算符Position-momentum uncertainty principles, 位置-动量不确定性关系Position space wave function, 坐标空间波函数Positronium, 电子偶素Potential energy, 势能Potential well, 势阱Power law potential, 幂律势Power series expansion, 幂级数展开Principal quantum number, 主量子数Probability, 几率Probability current, 几率流Probability density, 几率密度Projection operator, 投影算符Propagator, 传播子Proton, 质子QQuantum dynamics, 量子动力学Quantum electrodynamics, 量子电动力学Quantum number, 量子数Quantum statics, 量子统计Quantum statistical mechanics, 量子统计力学Quark, 夸克RRabi flopping frequency, 拉比翻转频率Radial equation, 径向方程Radial wave function, 径向波函数Radiation, 辐射Radius, 半径Raising operator, 上升算符Rayleigh's formula, 瑞利公式Realist position, 实在论立场Recursion formula, 递推公式Reduced mass, 约化质量Reflected wave, 反射波Reflection coefficient, 反射系数Relativistic correction, 相对论修正Rigid rotor, 刚性转子Rodrigues formula, 罗德里格斯公式Rotating wave approximation, 旋转波近似Rutherford scattering, 卢瑟福散射Rydberg constant, 里德堡常数Rydberg formula, 里德堡公式SScalar potential, 标势Scattering, 散射Scattering amplitude, 散射幅Scattering angle, 散射角Scattering matrix, 散射矩阵Scattering state, 散射态Schrodinger equation, 薛定谔方程Schrodinger picture, 薛定谔绘景Schwarz inequality, 施瓦兹不等式Screening, 屏蔽Second-order correction, 二级修正Selection rules, 选择定则Semiconductor, 半导体Separable solutions, 分离变量解Separation of variables, 变量分离Shell, 壳Simple harmonic oscillator, 简谐振子Simultaneous diagonalization, 同时对角化Singlet state, 单态Slater determinant, 斯拉特行列式Soft-sphere scattering, 软球散射Solenoid, 螺线管Solids, 固体Spectral decomposition, 谱分解Spectrum, 谱Spherical Bessel functions, 球贝塞尔函数Spherical coordinates, 球坐标Spherical Hankel functions, 球汉克尔函数Spherical harmonics, 球谐函数Spherical Neumann functions, 球纽曼函数Spin, 自旋Spin matrices, 自旋矩阵Spin-orbit coupling, 自旋-轨道耦合Spin-orbit interaction, 自旋-轨道相互作用Spinor, 旋量Spin-spin coupling, 自旋-自旋耦合Spontaneous emission, 自发辐射Square-integrable function, 平方可积函数Square well, 方势阱Standard deviation, 标准偏差Stark effect, 斯塔克效应Stationary state, 定态Statistical interpretation, 统计诠释Statistical mechanics, 统计力学Stefan-Boltzmann law, 斯特番-玻尔兹曼定律Step function, 阶跃函数Stem-Gerlach experiment, 斯特恩-盖拉赫实验Stimulated emission, 受激辐射Stirling's approximation, 斯特林近似Superconductor, 超导体Symmetrization, 对称化Symmetry, 对称TTaylor series, 泰勒级数Temperature, 温度Tetragonal symmetry, 正方对称Thermal equilibrium, 热平衡Thomas precession, 托马斯进动Time-dependent perturbation theory, 含时微扰论Time-dependent Schrodinger equation, 含时薛定谔方程Time-independent perturbation theory, 定态微扰论Time-independent Schrodinger equation, 定态薛定谔方程Total cross-section, 总截面Transfer matrix, 转移矩阵Transformation, 变换Transition, 跃迁;Transition probability, 跃迁几率Transition rate, 跃迁速率Translation,平移Transmission coefficient, 透射系数Transmitted wave, 透射波Trial wave function, 试探波函数Triplet state, 三重态Tunneling, 隧穿Turning points, 回转点Two-fold degeneracy , 二重简并Two-level systems, 二能级体系UUncertainty principle, 不确定性关系Unstable particles, 不稳定粒子VValence electron, 价电子Van der Waals interaction, 范德瓦尔斯相互作用Variables, 变量Variance, 方差Variational principle, 变分原理Vector, 矢量Vector potential, 矢势Velocity, 速度Vertex factor, 顶角因子Virial theorem, 维里定理WWave function, 波函数Wavelength, 波长Wave number, 波数Wave packet, 波包Wave vector, 波矢White dwarf, 白矮星Wien's displacement law, 维恩位移定律YYukawa potential, 汤川势ZZeeman effect, 塞曼效应。
Quantum Mechanics
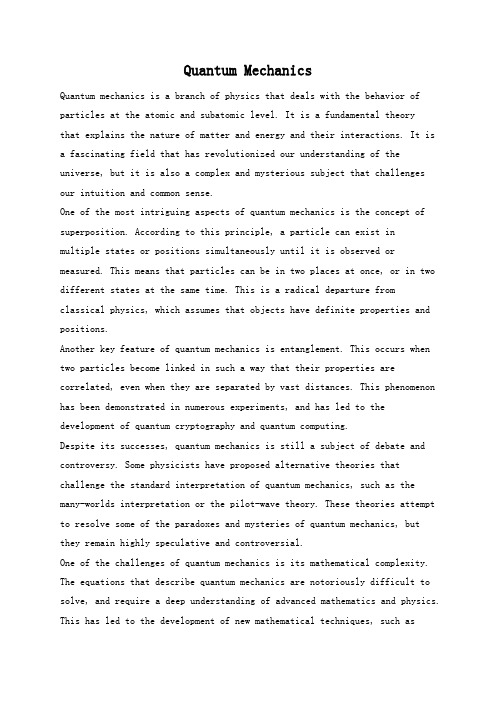
Quantum MechanicsQuantum mechanics is a branch of physics that deals with the behavior of particles at the atomic and subatomic level. It is a fundamental theorythat explains the nature of matter and energy and their interactions. It is a fascinating field that has revolutionized our understanding of the universe, but it is also a complex and mysterious subject that challenges our intuition and common sense.One of the most intriguing aspects of quantum mechanics is the concept of superposition. According to this principle, a particle can exist inmultiple states or positions simultaneously until it is observed or measured. This means that particles can be in two places at once, or in two different states at the same time. This is a radical departure fromclassical physics, which assumes that objects have definite properties and positions.Another key feature of quantum mechanics is entanglement. This occurs when two particles become linked in such a way that their properties are correlated, even when they are separated by vast distances. This phenomenon has been demonstrated in numerous experiments, and has led to the development of quantum cryptography and quantum computing.Despite its successes, quantum mechanics is still a subject of debate and controversy. Some physicists have proposed alternative theories that challenge the standard interpretation of quantum mechanics, such as the many-worlds interpretation or the pilot-wave theory. These theories attempt to resolve some of the paradoxes and mysteries of quantum mechanics, but they remain highly speculative and controversial.One of the challenges of quantum mechanics is its mathematical complexity. The equations that describe quantum mechanics are notoriously difficult to solve, and require a deep understanding of advanced mathematics and physics. This has led to the development of new mathematical techniques, such asmatrix mechanics and wave mechanics, which have revolutionized the field of quantum mechanics.Another challenge of quantum mechanics is its philosophical implications. The concept of superposition and entanglement challenges our notions of causality, determinism, and objective reality. It raises questions aboutthe nature of consciousness and the role of the observer in shaping reality. These philosophical questions are still being debated by physicists and philosophers, and have profound implications for our understanding of the universe and our place in it.Despite its challenges, quantum mechanics has led to numerous technological innovations, such as lasers, transistors, and MRI machines. It has also opened up new avenues of research, such as quantum field theory and string theory, which seek to unify quantum mechanics with general relativity and other branches of physics. It is a field that continues to captivate and inspire scientists and laypeople alike, and promises to unlock many more secrets of the universe in the years to come.。
二能级系统中的布居数反转
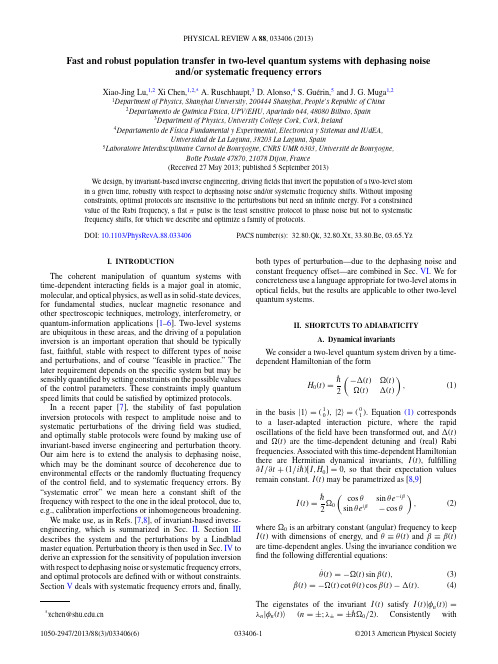
DOI: 10.1103/PhysRevA.88.033406
PACS number(s): 32.80.Qk, 32.80.Xx, 33.80.Be, 03.65.Yz
I. INTRODUCTION
The coherent manipulation of quantum systems with time-dependent interacting fields is a major goal in atomic, molecular, and optical physics, as well as in solid-state devices, for fundamental studies, nuclear magnetic resonance and other spectroscopic techniques, metrology, interferometry, or quantum-information applications [1–6]. Two-level systems are ubiquitous in these areas, and the driving of a population inversion is an important operation that should be typically fast, faithful, stable with respect to different types of noise and perturbations, and of course “feasible in practice.” The later requirement depends on the specific system but may be sensibly quantified by setting constraints on the possible values of the control parameters. These constraints imply quantum speed limits that could be satisfied by optimized protocols.
SCI分区——物理类期刊列表
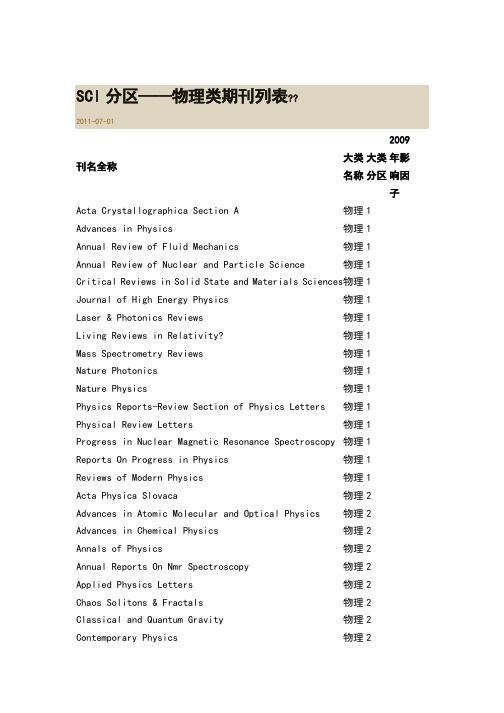
SCI分区——物理类期刊列表?? 2011-07-01刊名全称大类名称大类分区2009年影响因子Acta Crystallographica Section A物理1 Advances in Physics物理1 Annual Review of Fluid Mechanics物理1 Annual Review of Nuclear and Particle Science物理1 Critical Reviews in Solid State and Materials Sciences物理1 Journal of High Energy Physics物理1 Laser & Photonics Reviews物理1 Living Reviews in Relativity?物理1 Mass Spectrometry Reviews物理1 Nature Photonics物理1 Nature Physics物理1 Physics Reports-Review Section of Physics Letters物理1 Physical Review Letters物理1 Progress in Nuclear Magnetic Resonance Spectroscopy物理1 Reports On Progress in Physics物理1 Reviews of Modern Physics物理1 Acta Physica Slovaca物理2 Advances in Atomic Molecular and Optical Physics物理2 Advances in Chemical Physics物理2 Annals of Physics物理2 Annual Reports On Nmr Spectroscopy物理2 Applied Physics Letters物理2 Chaos Solitons & Fractals物理2 Classical and Quantum Gravity物理2 Contemporary Physics物理2Europhysics Letters物理2 European Physical Journal C物理2 Journal of Applied Crystallography物理2 Journal of Chemical Physics物理2 Journal of Magnetic Resonance物理2 Journal of the Mechanics and Physics of Solids物理2 Journal of Physics G-Nuclear and Particle Physics物理2 Journal of Raman Spectroscopy物理2 Journal of Rheology物理2 Journal of Statistical Mechanics-Theory and Experiment物理2 Journal of Synchrotron Radiation物理2 Laser Physics Letters物理2 New Journal of Physics物理2 Nuclear Fusion物理2 Nuclear Physics B物理2 Optics Express物理2 Optics Letters物理2 Physics Letters B物理2 Physics of Plasmas物理2 Physical Review A物理2 Physical Review B物理2 Physical Review C物理2 Physical Review D物理2 Physical Review E物理2 Physics Today物理2 Plasma Physics and Controlled Fusion物理2 Plasma Processes and Polymers物理2 Plasma Sources Science & Technology物理2 Progress in Electromagnetics Research-Pier?物理2 Progress in Optics?物理2 Progress in Particle and Nuclear Physics物理2 Quantum Information & Computation物理2 Spectrochimica Acta Part B-Atomic Spectroscopy物理2Acta Crystallographica Section B-Structural Science物理3 Advances in Theoretical and Mathematical Physics?物理3 Annalen Der Physik物理3 Applied and Computational Harmonic Analysis物理3 Applied Mechanics Reviews?物理3 Applied Optics物理3 Applied Physics B-Lasers and Optics物理3 Applied Physics Express物理3 Applied Rheology?物理3 Archive For Rational Mechanics and Analysis物理3 Atomic Data and Nuclear Data Tables物理3 Chaos物理3 Chinese Physics物理3 Communications in Computational Physics物理3 Communications in Mathematical Physics物理3 Computers & Fluids物理3 Computational Mechanics物理3 Computer Physics Communications物理3 Current Applied Physics物理3 Engineering Fracture Mechanics物理3 European Journal of Mechanics A-Solids物理3 European Physical Journal A物理3 European Physical Journal B物理3 European Physical Journal D物理3 General Relativity and Gravitation物理3 International Journal of Mass Spectrometry物理3 International Journal of Multiphase Flow物理3 International Journal of Solids and Structures物理3 Journal of the Acoustical Society of America物理3 Journal of Applied Physics物理3 Journal of Computational Physics物理3 Journal of Crystal Growth物理3 Journal of Electromagnetic Waves and Applications物理3Journal of Fluid Mechanics物理3Journal of Luminescence物理3Journal of Magnetism and Magnetic Materials物理3Journal of Molecular Spectroscopy物理3Journal of Non-Newtonian Fluid Mechanics物理3Journal of Optics A-Pure and Applied Optics物理3Journal of the Optical Society of America A-Optics Image物理3 Science and VisionJournal of the Optical Society of America B-Optical物理3 PhysicsJournal of Physics A-Mathematical and Theoretical?物理3Journal of Physics B-Atomic Molecular and Optical物理3 PhysicsJournal of Physics D-Applied Physics物理3Journal of the Physical Society of Japan物理3Journal of Physics-Condensed Matter物理3Journal of Quantitative Spectroscopy & Radiative物理3 TransferJournal of Statistical Physics物理3Journal of Turbulence物理3Jetp Letters物理3Molecular Physics物理3Nuclear Data Sheets物理3Nuclear Physics A物理3Optics Communications物理3Philosophical Magazine Letters物理3Physics of Fluids物理3Physics Letters A物理3Physical Mesomechanics?物理3Physical Review Special Topics-Accelerators and Beams物理3Physical Review Special Topics-Physics Education物理3 Research?Physica Status Solidi-Rapid Research Letters物理3Physica A物理3Physica D物理3Physics-Uspekhi物理3Progress of Theoretical Physics物理3Rheologica Acta物理3Rivista Del Nuovo Cimento物理3Siam Journal On Applied Dynamical Systems物理3Solid State Communications物理3Solid-State Electronics物理3Solid State Ionics物理3Solid State Nuclear Magnetic Resonance物理3Spectrochimica Acta Part A-Molecular and Biomolecular物理3 SpectroscopySuperconductor Science & Technology物理3Theoretical and Computational Fluid Dynamics物理3Acoustics Australia?物理4Acoustical Physics物理4Acta Acustica United With Acustica物理4Acta Crystallographica Section C-Crystal Structure物理4 CommunicationsActa Crystallographica Section E-Structure Reports物理4 OnlineActa Mechanica物理4Acta Mechanica Sinica物理4Acta Mechanica Solida Sinica物理4Acta Physica Polonica A物理4Acta Physica Polonica B物理4Acta Physica Sinica物理4Advances in Imaging and Electron Physics?物理4American Journal of Physics物理4Annales Henri Poincare物理4Annales De Physique物理4Applied Acoustics物理4Applied Magnetic Resonance物理4Archives of Acoustics?物理4Archive of Applied Mechanics物理4Atomic Spectroscopy物理4Brazilian Journal of Physics物理4Canadian Journal of Physics物理4Central European Journal of Physics物理4Chinese Optics Letters物理4Chinese Journal of Chemical Physics物理4Chinese Journal of Physics物理4Chinese Physics B物理4Chinese Physics C物理4Chinese Physics Letters物理4Communications in Theoretical Physics物理4Concepts in Magnetic Resonance Part A物理4Concepts in Magnetic Resonance Part B-Magnetic物理4 Resonance EngineeringCondensed Matter Physics物理4Continuum Mechanics and Thermodynamics物理4Contributions to Plasma Physics物理4Comptes Rendus Mecanique物理4Comptes Rendus Physique物理4Cryogenics物理4Crystal Research and Technology物理4Crystallography Reports物理4Doklady Physics物理4Dynamical Systems-An International Journal物理4European Journal of Mass Spectrometry物理4European Journal of Mechanics B-Fluids物理4European Journal of Physics物理4European Physical Journal-Applied Physics物理4European Physical Journal-Special Topics?物理4Experimental Mechanics物理4Ferroelectrics Letters Section物理4Few-Body Systems物理4Fiber and Integrated Optics物理4Fluid Dynamics Research物理4Fortschritte Der Physik-Progress of Physics物理4Foundations of Physics物理4Granular Matter物理4High Energy Physics and Nuclear Physics-Chinese Edition物理4High Pressure Research物理4High Temperature物理4Ieee Transactions On Plasma Science物理4Indian Journal of Physics and Proceedings of the Indian物理4 Association For the Cultivation of ScienceIndian Journal of Pure & Applied Physics物理4Infrared Physics & Technology物理4International Journal of Applied Electromagnetics and物理4 MechanicsInternational Journal of Computational Fluid Dynamics物理4International Journal of Exergy物理4International Journal of Fracture物理4International Journal of Geometric Methods in Modern物理4 PhysicsInternational Journal of Modern Physics A物理4International Journal of Modern Physics B物理4International Journal of Modern Physics C物理4International Journal of Modern Physics E-Nuclear物理4 PhysicsInternational Journal of Non-Linear Mechanics物理4International Journal For Numerical Methods in Fluids物理4International Journal of Quantum Information物理4International Journal of Theoretical Physics物理4International Journal of Thermophysics物理4Journal of Applied Mechanics and Technical Physics?物理4Journal of Applied Mechanics-Transactions of the Asme物理4Journal of Computational Acoustics物理4Journal of Electron Spectroscopy and Related Phenomena物理4Journal of the European Optical Society-Rapid物理4 PublicationsJournal of Experimental and Theoretical Physics物理4Journal of Geometry and Physics物理4Journal of Laser Applications物理4Journal of Low Frequency Noise Vibration and Active物理4 ControlJournal of Low Temperature Physics物理4Journal of Mathematical Fluid Mechanics物理4Journal of Mathematical Physics物理4Journal of Mechanics物理4Journal of Modern Optics物理4Journal of Near Infrared Spectroscopy物理4Journal of Non-Equilibrium Thermodynamics物理4Journal of Nonlinear Mathematical Physics物理4Journal of Nonlinear Optical Physics & Materials物理4Journal of the Optical Society of Korea?物理4Journal of Optical Technology物理4Journal of Plasma Physics物理4Journal of Russian Laser Research物理4Journal of Superconductivity and Novel Magnetism物理4Journal of Theoretical and Applied Mechanics?物理4Journal of Thermal Science and Technology物理4Japanese Journal of Applied Physics Part 1-Regular物理4 Papers Short Notes & RevLaser Focus World物理4Laser Physics物理4Letters in Mathematical Physics物理4Liquid Crystals物理4Lithuanian Journal of Physics物理4Low Temperature Physics物理4Magnetohydrodynamics?物理4Meccanica物理4Mechanics Based Design of Structures and Machines物理4Mechanics Research Communications物理4Mechanika?物理4Modern Physics Letters A物理4Modern Physics Letters B物理4Molecular Crystals and Liquid Crystals物理4Multibody System Dynamics物理4Nonlinearity物理4Nuclear Instruments & Methods in Physics Research物理4 Section A-Accelerators SpNuclear Instruments & Methods in Physics Research物理4 Section B-Beam InteractioNuclear Science and Techniques?物理4Nukleonika物理4Nuovo Cimento Della Societa Italiana Di Fisica物理4 B-General Physics RelativityOptica Applicata物理4Optics and Laser Technology物理4Optical Review物理4Optics and Spectroscopy物理4Optik物理4Phase Transitions物理4Phonetica物理4Photonics Spectra物理4Physics of Atomic Nuclei物理4Physics of Particles and Nuclei物理4Physics in Perspective物理4Physica Scripta物理4Physics of the Solid State物理4Physica Status Solidi B-Basic Research物理4Physics World物理4 Physica B物理4 Physica C物理4 Physica E物理4 Plasma Devices and Operations物理4 Plasma Physics Reports物理4 Plasma Science & Technology物理4 Pmm Journal of Applied Mathematics and Mechanics物理4 Pramana-Journal of Physics物理4 Problems of Atomic Science and Technology?物理4 Progress in Computational Fluid Dynamics物理4 Progress of Theoretical Physics Supplement物理4 Quarterly Journal of Mechanics and Applied Mathematics物理4 Quantum Information Processing物理4 Radiation Effects and Defects in Solids物理4 Regular & Chaotic Dynamics物理4 Reports On Mathematical Physics物理4 Revista Brasileira De Ensino De Fisica物理4 Reviews in Mathematical Physics物理4 Revista Mexicana De Fisica物理4 Romanian Journal of Physics?物理4 Romanian Reports in Physics?物理4 Russian Journal of Mathematical Physics物理4 Science in China Series G-Physics Mechanics & Astronomy物理4 Semiconductors and Semimetals?物理4 Semiconductors物理4 Shock and Vibration物理4 Shock Waves物理4 Solid State Physics?物理4 Sound and Vibration物理4 Spectroscopy Letters物理4 Spectroscopy-An International Journal物理4 Spectroscopy物理4Studies in History and Philosophy of Modern Physics物理4Superlattices and Microstructures物理4Symmetry Integrability and Geometry-Methods and物理4 ApplicationsTechnical Physics Letters物理4Technical Physics物理4Theoretical and Mathematical Physics物理4Topics in Applied Physics物理4Transport Theory and Statistical Physics物理4Wave Motion物理4Waves in Random and Complex Media物理4X-Ray Spectrometry物理4Zeitschrift Fur Kristallographie物理4Zeitschrift Fur Kristallographie-New Crystal物理4 StructuresZeitschrift Fur Naturforschung Section A-A Journal of物理4 Physical SciencesZeitschrift Fur Physikalische Chemie-International物理4 Journal of Research in P。
临界自组织SOC和BTW模型的平均场方法计算
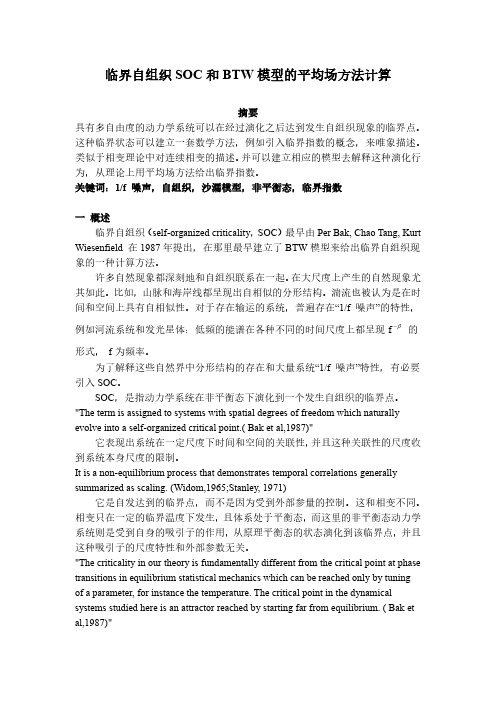
临界自组织 SOC 和 BTW 模型的平均场方法计算
摘要 具有多自由度的动力学系统可以在经过演化之后达到发生自组织现象的临界点。 这种临界状态可以建立一套数学方法,例如引入临界指数的概念,来唯象描述。 类似于相变理论中对连续相变的描述。 并可以建立相应的模型去解释这种演化行 为,从理论上用平均场方法给出临界指数。 关键词:1/f 噪声,自组织,沙漏模型,非平衡态,临界指数 一 概述 临界自组织 (self-organized criticality, SOC) 最早由 Per Bak, Chao Tang, Kurt Wiesenfield 在 1987 年提出,在那里最早建立了 BTW 模型来给出临界自组织现 象的一种计算方法。 许多自然现象都深刻地和自组织联系在一起。 在大尺度上产生的自然现象尤 其如此。比如,山脉和海岸线都呈现出自相似的分形结构。湍流也被认为是在时 间和空间上具有自相似性。对于存在输运的系统,普遍存在“1/f 噪声”的特性, 例如河流系统和发光星体:低频的能谱在各种不同的时间尺度上都呈现 f 的 形式, f 为频率。 为了解释这些自然界中分形结构的存在和大量系统“1/f 噪声”特性,有必要 引入 SOC。 SOC,是指动力学系统在非平衡态下演化到一个发生自组织的临界点。 "The term is assigned to systems with spatial degrees of freedom which naturally evolve into a self-organized critical point.( Bak et al,1987)" 它表现出系统在一定尺度下时间和空间的关联性, 并且这种关联性的尺度收 到系统本身尺度的限制。 It is a non-equilibrium process that demonstrates temporal correlations generally summarized as scaling. (Widom,1965;Stanley, 1971) 它是自发达到的临界点,而不是因为受到外部参量的控制。这和相变不同。 相变只在一定的临界温度下发生,且体系处于平衡态,而这里的非平衡态动力学 系统则是受到自身的吸引子的作用,从原理平衡态的状态演化到该临界点,并且 这种吸引子的尺度特性和外部参数无关。 "The criticality in our theory is fundamentally different from the critical point at phase transitions in equilibrium statistical mechanics which can be reached only by tuning of a parameter, for instance the temperature. The critical point in the dynamical systems studied here is an attractor reached by starting far from equilibrium. ( Bak et al,1987)"
强磁场下的固体物理研究进展
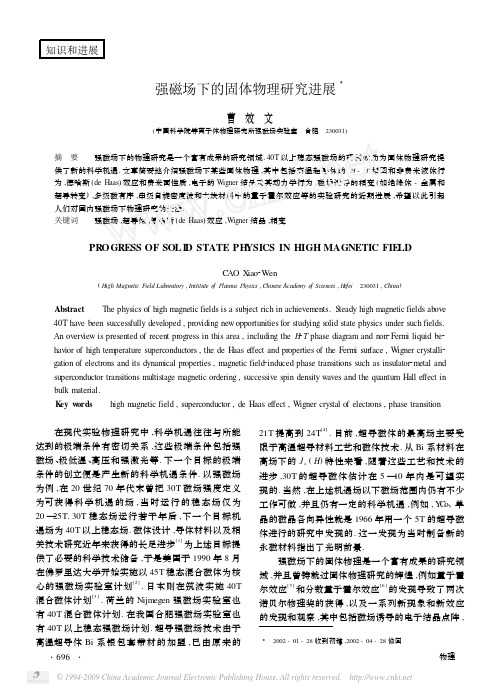
知识和进展强磁场下的固体物理研究进展3曹 效 文(中国科学院等离子体物理研究所强磁场实验室 合肥 230031)摘 要 强磁场下的物理研究是一个富有成果的研究领域.40T 以上稳态强磁场的研制成功为固体物理研究提供了新的科学机遇.文章简要地介绍强磁场下某些固体物理,其中包括高温超导体的H -T 相图和非费米液体行为,德哈斯(de Haas )效应和费米面性质,电子的Wigner 结晶及其动力学行为,磁场诱导的相变(如绝缘体-金属和超导转变),多级磁有序,串级自旋密度波和大块材料中的量子霍尔效应等的实验研究的近期进展,希望以此引起人们对国内强磁场下物理研究的关注.关键词 强磁场,超导体,德哈斯(de Haas )效应,Wigner 结晶,相变PR OGRESS OF SOLI D STATE PH YSICS IN HIGH MAGNETIC FIE LDC AO X iao 2Wen(H igh Magnetic Field Laboratory ,Institute o f Plasma Physics ,Chinese Academy o f Sciences ,H e fei 230031,China )Abstract The physics of high magnetic fields is a subject rich in achievements.S teady high magnetic fields above 40T have been success fully developed ,providing new opportunities for studying solid state physics under such fields.An overview is presented of recent progress in this area ,including the H 2T phase diagram and non 2Ferm i liquid be 2havior of high tem perature superconductors ,the de Haas effect and properties of the Ferm i surface ,W igner crystalli 2gation of electrons and its dynam ical properties ,magnetic field 2induced phase transitions such as insulator 2metal and superconductor transitions multistage magnetic ordering ,successive spin density waves and the quantum Hall effect in bulk material.K ey w ords high magnetic field ,superconductor ,de Haas effect ,W igner crystal of electrons ,phase transition3 2002-01-28收到初稿,2002-04-28修回 在现代实验物理研究中,科学机遇往往与所能达到的极端条件有密切关系,这些极端条件包括强磁场、极低温、高压和强激光等.下一个目标的极端条件的创立便是产生新的科学机遇条件.以强磁场为例,在20世纪70年代末曾把30T 磁场强度定义为可获得科学机遇的场,当时运行的稳态场仅为20—25T.30T 稳态场运行若干年后,下一个目标机遇场为40T 以上稳态场.磁体设计,导体材料以及相关技术研究近年来获得的长足进步[1]为上述目标提供了必要的科学技术储备,于是美国于1990年8月在佛罗里达大学开始实施以45T 稳态混合磁体为核心的强磁场实验室计划[2].日本则在筑波实施40T 混合磁体计划[3].荷兰的Nijmegen 强磁场实验室也有40T 混合磁体计划.在我国合肥强磁场实验室也有40T 以上稳态强磁场计划.超导强磁场技术由于高温超导体Bi 系银包套带材的加盟,已由原来的21T 提高到24T [4].目前,超导磁体的最高场主要受限于高温超导材料工艺和磁体技术.从Bi 系材料在高场下的J c (H )特性来看,随着这些工艺和技术的进步,30T 的超导磁体估计在5—10年内是可望实现的.当然,在上述机遇场以下磁场范围内仍有不少工作可做,并且仍有一定的科学机遇,例如,Y C o 5单晶的磁晶各向异性就是1966年用一个5T 的超导磁体进行的研究中发现的.这一发现为当时制备新的永磁材料指出了光明前景.强磁场下的固体物理是一个富有成果的研究领域,并且曾铸就过固体物理研究的辉煌,例如量子霍尔效应[5]和分数量子霍尔效应[6]的发现导致了两次诺贝尔物理奖的获得,以及一系列新现象和新效应的发现和观察,其中包括磁场诱导的电子结晶点阵,・696・物理即Wigner固体[7],磁场诱导的绝缘体-金属转变和超导电性[8]等.有关强磁场下的科学研究讨论会和半导体物理都有定期的国际会议,强关联电子系统的国际会议也含有可观数量的强磁场下的研究内容.强磁场下的物理研究课题颇多,这里仅介绍某些方面,并借此引起国内物理学界对强磁场下物理研究的关注.1 强磁场下的高温超导体研究和低温超导体相比,高温超导体的超导转变温度Tc和上临界场H c2均高出近一个量级,即T c约为102K,H c2(0)高于102T.这么高的临界参量预示着高温超导体的潜在应用前景及其可观的经济价值,同时也丰富了超导物理的研究内容,例如与强磁场密切相关的H-T相图和以Jc(H)为中心的磁通动力学性质的研究等.到目前为止,这些研究多数仅限于液氮温区,对于高温超导体来说,由于Tc为百K量级,这一温度范围仍限于T c附近;所用磁场也多数限于10T以下,这对于临界场高于100T的高温超导体来说,也仅属于低场.更低温区的研究是必要的,但是要求更高的磁场,例如日本筑波40T稳态场磁体系统的建立就是以高温超导体为主要目标的. 111 高温超导体的H-T相图高温超导体H-T相图的一个显著特点是,在下临界场Hc1(T)与上临界场H c2(T)之间的混合态区域内存在着一个新的相变线———不可逆线I L(图中标为Hirr),如图1所示.这个新相交线的性质可以大致归纳为:对于无孪晶界和明显缺陷的单晶,I L 是一个由涡旋点阵态到涡旋液态的熔化线,这个相变属于一级相变;对于存在有效钉扎作用的缺陷的样品,如存在着明显无序的薄膜和有明显缺陷的单晶,I L是一个由涡旋玻璃固态到液态的转变,并且属于二级相变.不可逆线上下的不同涡旋状态表明了磁通钉扎强度的改变,因而I L上下的临界电流密度及其行为应该是有区别的.近来的实验结果[9]表明,在I L上下存在着Jc的剧烈变化,并遵守不同的温度关系和磁场关系.由此可以看出,I L是一个对材料结构(它直接影响Jc行为)敏感的参量,这与H c1(T)和H c2(T)是材料的本征参量形成鲜明对照.已有由于钉扎强度的改善,I L也随之抬高的有关报道.图1 Y BCO超导薄膜在磁场HΠΠc位形下的H-T相图[10] 关于高温超导体耗散(dissipation)行为的研究进一步表明,在I L和Hc2(T)之间还存在一个新的相界HK(T)线的证据[10].H K(T)线把涡旋液态分成两个区:在I L与HK(T)之间涡旋之间是关联的(corre2 lated),涡旋运动具有激活的特征;在H K(T)与H c2(T)之间,涡旋之间是非关联的,其运动以扩散运动为特征.关于这方面研究的报道仍较少,其相变性质也有待进一步研究.以上研究,一方面大多限于Tc附近温区,向更低温区扩展要求更高的磁场强度.另一方面,I L和H K(T)的性质和起源尚未得到完全一致的认识. 112 强磁场下的J c(H)特性虽然高的超导转变温度和高的上临界场预示着高温超导体的潜在应用前景,但最终决定其大规模应用前景的是在一定温度下的Jc(H)特性,通常要求Jc值高于104AΠcm2.高温超导体与低温超导体的Jc(H)关系的比较研究显示[11],在412K,Bi系银包套带材在15T以上磁场范围的Jc明显高于低温超导体,而Y BC O的C VD膜在77K的J c值,在25T以上磁场范围也明显地高于低温超导体.这些高温超导体Jc(H)的一个显著特点是,直到30T的高场仍未出现显著下降,这对高场应用十分有利.但是,我们必须记住,超导体J c(H)特性是一个对材料结构因素(如缺陷和第二相的存在等)敏感的临界参量,因此它强烈地取决于成材工艺.但到目前为止,Y BC O和Bi系材料中什么样的缺陷对钉扎是最有效的,仍不清楚.因而,任何一家生产者对其产品进行高场检验都是必须的.另一方面,高温超导材料在高场下与磁通运动特性密切相关的稳定性等问题也尚缺少系统的仔细研究.・796・31卷(2002年)11期113 强磁场下的正常态性质含铜氧化物超导体在T c 以上温区的面内电阻ρab 的线性行为及其与面外电阻ρc 的半导体行为的共存[12]常常被作为非费米液体的证据[13].这两种相反的电阻温度关系是否可以扩展到远离T c 的低温区,并作为一种正常态基态性质是一个不清楚的问题.一个最直接的方法是用强磁场抑制其超导电性来进行T c 以下温区的正常态性质的研究.但是这一方法是困难的,因为该类超导体上临界场很高,如前面所述.因此,选择一个T c 较低的同类材料和提高所能达到的场强是人们所希望的.Ando 等[14]利用61T 场强的脉冲场研究了Bi 2Sr 2CuO y 单晶(T c =13K )在T c 以下温区的正常态各向异性电阻行为.结果表明,直到0166K (T ΠT c =0105)仍然保持着上述的面内和面外电阻的温度关系行为,即仍表明一个非费米液体性质.其实,含铜氧化物超导体还存在一个面内和面外电阻行为相反的磁输运行为,这就是在高场区面内电阻ρab 表现出正磁阻,而面外电阻ρc 则呈现出负磁阻[15].进一步的研究表明,随着磁场的增加,ρab (H )趋于饱和[14],而ρc (H )则趋于线性减小[16].这种相反的磁电阻行为的起源尚不清楚,可能与T c 以上温区电阻的相反行为有关.有人认为ρc (H )的负磁电阻行为与双极化子超导理论相一致[16],或者被认为与态密度项对涨落电导的贡献有关,或与赝能隙的磁场关系有关,即负磁电阻意味着赝能隙随磁场的增加而减小.实际上,高温超导体正常态的非费米液体行为的一个直接证明是由Hill 等[17]近来刚刚完成的,他们用强磁场抑制了电子型氧化物超导体(Pr ,Ce )2CuO 4(T c =20K )的超导电性,并测量了在极低温下正常态的热导和电导.试验结果表明,二者之间的比值违反了维德曼-弗兰兹定律(Wiedeman -Franz law ),并强烈地表明存在着电子的自旋-电荷分离态.由于维德曼-弗兰兹定律是费米液体理论的一个固有结果,因此上述结果是高温超导体的非费米液体行为的第一个直接证明[18].为了确认费米液体图像对这类超导体的不适用性,对不同超导体及其不同化学掺杂量样品的重复测量是必要的.在高温超导体中还普遍存在着另一个反常的正常态输运行为,霍尔角C ot θH =ρxx Πρxy ∝T 2,并且也被作为非费米液体的实验证据.但近来也有C ot θH ∝T 关系的报道[19],这一结果与费米液体的物理图像是一致的.2 强磁场下费米面性质研究磁场对固体中载流子运动的重要影响之一是量子化效应.在一个均匀磁场中,电子作环绕磁力线的螺旋运动.在一恒定磁场下,其回转频率ω0=qB Πm 3.如果在垂直于磁场方向施加一频率为ω=ω0的交变场,其能量将被电子共振吸收,这就是回旋共振现象.随着磁场增大,电子的这种螺旋运动会形成一个个高度简并的朗道(Landau )能级,当这些朗道能级与费米面相切时,就会出现磁化率、电阻或比热等物理量随磁场的振荡现象,并且这些振荡与磁场的倒数1ΠH 呈周期结构.磁化率随1ΠH 呈现的周期性振荡称为德哈斯-范阿尔芬(de Haas -van Al 2phen ,dHvA )效应,类似的电阻周期性振荡称为舒布尼科夫-德哈斯(Shubnikov -de Haas ,SdH )效应.为了清楚地显现出de Haas 效应,要求满足两个条件:ω0τµ1和ω0>k B T ,式中τ是电子的自由运动时间.由ω0τµ1,要求尽可能高的磁场强度和高纯度的单晶;为满足ω0>k B T 要求实验必须在足够低的温度下进行,通常在1K 以下温度进行,低温也有利于τ值的提高.电子能带结构是凝聚态物质物理性质的核心问题,而基于de Haas 效应的费米面及其性质的实验研究是了解电子能带结构的最直接和最有效的方法.自de Haas 效应发现以来,新的合成材料的不断出现和磁场强度的不断提高,使得费米面及其性质研究的内容进一步丰富,并使其一直是凝聚态物理研究中的前沿课题,例如一个时期以来有机超导体[20]和以稀土元素化合物为主体的强关联体系[21]的费米面及其性质研究等.这里值得一提的有两项实验研究:一个是Y BC O 高温超导体的dHvA 效应.Mueller 等[22]在Los Alam os 国家实验室在214—412K 温区采用100T 脉冲磁场观察到了Y BC O 的dHvA 效应,经傅里叶变换处理的结果,表明三个独立的基频分别为0153,0178和3115kT.K ido 等[23]在118—311K 温度范围内,用场强为27T 的直流磁场,观察到频率为0154kT 的dHvA 效应,与Mueller 的0153kT 基本一致.由上述两个实验,我们可以得出两个重要结论:(1)Y BC O 高温超导体存在着费米面;(2)在上临界场H c2以下的混合态能够观察到dHvA 效应,而传统认为,H >H c2是观察这一效应的必要条件.基于这一结论,在低温A15超导体V 3Si 上获得了类似结果[24].・896・物理另一个值得一提的费米面研究实验是β″(BE DT -TTF)SF5CH2CF2S O3有机超导体的SdH效应.通常观察的是与磁场垂直的面内电阻ρxx(H)的de Haas 振荡.但Nam等[25]近来用60T脉冲场第一次观察到层间电阻ρzz随磁场的振荡,并且电导最小值即电阻振荡峰值与温度的关系呈现出热激活行为,这一结果对有机超导体费米面及其性质的认识无疑提供了新的信息.3 电子的Wigner结晶磁场对固体中载流子运动的另一重要影响是维度效应.在一个低载流子浓度的三维系统中,当磁场足够强(例如ωτµ1)时,电子运动轨道呈圆柱形,电子的运动实际上只沿单一方向发生.在一个垂直于磁场的二维系统中,磁场把输运载流子捕获在它的最低朗道能级上,载流子的运动轨道被限制在平面内,其迁移动能大大降低,系统实际上成为准零维的.在一个处于低温下的低载流子密度的系统中,可以出现“磁冻结”状态的局域化.当磁长度lc=( ΠeB)1Π2可以和载流子的平均距离a0相比拟时,就会出现载流子的有序排列,即凝聚成电子结晶点阵,这就是所谓的Wigner结晶.这种电子的磁冻结现象是数十年来电子-电子相关能量观察的顶点.在输运测量中,当“磁冻结”发生时,将伴随着电阻率的急剧增大,实际上发生了金属-绝缘体转变.Wigner电子结晶已先后在低载流子浓度的二维电子气系统[26]和三维系统[7,27]中观察到.不难看出,磁场引起的输运电子局域化,磁冻结和Wigner结晶的实质是磁场诱导的输运载流子运动维度减小的结果.在二维电子气系统中,lc趋近于a0也是导致分数量子霍尔效应的条件.在实验中,随着磁场的增大,系统首先进入分数量子霍尔效应态,然后,最终进入Wigner 结晶态[26].近来G lass on[28]利用输运测量观察了Wigner结晶中的动力学有序化;Li等[29]利用微波共振实验研究了二维电子系统中载流子-载流子和载流子-杂质互作用之间的竞争在高场绝缘相中的作用,结果表明,在载流子-载流子互作用为主的系统中形成Wigner结晶,而在载流子-杂质互作用占支配地位的系统中则形成Wigner玻璃态.4 磁场诱导的相变411 绝缘体-金属和超导转变K hmelnitskii[30]从理论上提出,如果一个系统是全局域的,那么在磁场中可能恢复到退局域态.一个典型的实验结果是[31]:Si掺杂的G aAs异质结在H=5T附近发生半导体-金属转变,在H<5T时表现为负的电阻温度系数,在H≥5T时则呈现出正的电阻温度系数.近来,碳纳米管的实验也表明了类似的磁场诱导的绝缘体-金属相变[32].近来,Uji等[8,33]在实验中发现,对于准二维绝缘材料λ-(BETS)2FeCl4,当平行于层面的磁场达到1015T时,系统发生绝缘体-金属转变;当磁场增加到18T时发生超导转变,相应的Tc=0104K,然后随着磁场增加,Tc升高.遗憾的是,该实验中的磁场仅能达到20T.紧接着,Balicas等[33]利用塔拉哈西国家强磁场实验室的45T稳态场,研究了不同温度下的磁电阻R(H)和不同磁场下的电阻转变R(T),如图2所示.结果表明,Tc的最高值为412K,对应的磁场值是33T.然后,随着磁场的进一步增加,Tc降低,如图2(b)所示.Uji等[8]认为,上述磁场诱导的超导电性是由于平行于传导层的强磁场抑制了轨道效应;Balicas等[33]则认为是由于外加磁场补偿了定向排列的Fe3+离子的交换场所致,即可以依照Jaccar2 ieo-Peter效应解释.图2 (a)λ-(BETS)2FeCl4单晶体的电阻R的磁场关系,测量的温度间隔为0125K;(b)电阻转变的温度关系.磁场诱导的超 导转变的最高温是33T附近的412K[33]磁场诱导的绝缘体-金属转变的另一个例子是含锰氧化物的巨磁电阻效应.这种相变应归结为磁场诱导的载流子的退局域化效应.但是这类实验通常仅要求10T以下的低磁场.对于在低温下处于反铁磁态的掺杂的钙钛矿锰氧化物,更强的磁场会导致一个绝缘体-金属转变,实际上是一种反铁磁-铁磁转变,并伴随着电荷有序或轨道有序相的融・996・31卷(2002年)11期化[34].412 磁场诱导的磁相变在含有稀土元素的材料中,由于f电子往往呈现出强关联效应,继而导致各种反常态,磁有序反常是其中之一.一个典型的例子是,在CeP的磁相图中有六个以上的磁有序相存在[35],在磁化强度的磁场关系中表现为六个台阶,并且这些磁有序相的临界场在1ΠH坐标上几乎是等间隔的,这相应于朗道能级与费米面相切.在PrC o2Si2系统中也观察到类似的反常磁有序现象[36].这种串级磁有序的机制尚不清楚.413 有机导体中磁场诱导的串级自旋密度波和量子霍尔效应以Bechgaard盐为基础的有机材料[通式(T MTSF)2X,X=PF6,AsF6,ClO4,ReO4等]通常具有准一维或准二维特性,库仑作用占支配地位,因此,自旋密度波(S DW)基态是有利的.另一方面,某些有机导体在某个临界压力Pc以上是超导的,如(T MTSF)2PF6等.有些常压下就是超导体,如(T MTSF)2ClO4等.当沿着c方向施加一个超过临界场的强磁场时,可观察到一系列的金属-S DW相变,例如在(T MTSF)2ClO4中,这一串级金属-S DW 相变发生在3—27T磁场范围,热力学测量证明这些相变属于一级相变.此外,霍尔效应测量表明,每个S DW相对应的霍尔效应都是量子化的,这是第一个在大块材料上观察到的量子霍尔效应.串级自旋密度波和量子霍尔效应被认为是近年来有机材料研究中的两个重要发现[37],并且与有关理论预计是一致的[38].5 强磁场下的纳米材料当金属颗粒直径减小到纳米尺度时,金属颗粒的电子态成为3D阱或W ood-Sax on势的本征态.由于这个本征态是用球形谐振波函数描述的,所以纳米颗粒的电子态完全不同于大块金属的布洛赫波函数[39].纳米材料中的晶粒尺寸与一些基本物理量,如德布罗意波长和超导相关长度等可以相比拟,电子运动出现限域性,量子尺寸效应以及强关联性.这些使得纳米材料呈现出一系列不同寻常的性质.强磁场对固体性质影响可归结为磁场对电子运动行为的影响,如前面有关部分所述.当磁长度lc= ( ΠeB)1Π2达到纳米材料晶粒量级(如B=25T时,l c =5175nm)时,纳米材料会呈现出怎样的物理性质,是值得关注的问题.6 结束语本文简要的介绍了强磁场下固体物理研究的某些方面及其进展,由此可以了解强磁场在现代物理研究中的重要作用,同时还可以看到这些研究大多是在1K以下的极低温条件下进行的.因此,在获得强磁场条件的同时还必须佐以必要的极低温条件.参考文献[1]曹效文.物理,1996,25:552[Cao X W.Wuli(Physics),1996,25:552(in Chinese)][2]Brooks J et al.Physica B,1994,197:19;Muller J R et al.IEEET ransition M agnetics,1994,30:1563[3]Inone K et al.Physica B,1992,177:7;1994,201:517[4]Ohkura K et al.Appl.Phys.Lett.,1995,67:1923[5]V on K litzing K et al.Phys.Rev.Lett.,1980,45:494[6]S tümer H L et al.Phys.Rev.Lett.,1983,50:1953[7]R osenbaum T F et al.Phys.Rev.Lett.,1985,54:241[8]Uji S et al.Nature(London),2001,410:908[9]Cao X W,W ang Z H,Li K B.Physica C,1998,305:68[10]Palstra T T M et al.Phys.Rev.B,1990,41:6621;Puzmak R et al.Phys.Rev.B,1995,52:3756;Chien T R et al.Phys.Rev.Lett.,1991,66:3075;Cao X W,W ang Z H,Li K B.Phys.Rev.B,2000,62:12552;Cao X W,W ang Z H,Xu X J.Phys.Rev.B,2002,65:064521[11]Nakagawa Y et al.Physica B,1994,201:49[12]Iye Y.Ed.G insberg D M.Physical Properties of H igh T em peratureSuperconductorsⅢ.S ingapore:W orld Scientific,1991[13]Anders on P W.Science,1992,256:1526[14]Ando Y et al.Phys.Rev.Lett.,1996,77:2065[15]Y an Y F et al.Phys.Rev.B,1995,52:R751[16]Z avaritsky V N,S pring ford M,Alexadror A S.Physica B,2001,294—295:363[17]H ill R W et al.Nature,2001,414:711[18]Behnia K.Nature,2001,414:696[19]Vedeneev S I,Jansen A G M,W yder P.Physica B,2000,284—288:1023[20]W onitza J.Ferm i sur face of low dimensional organic metals and su2perconductors.S pringer T racts in M orden Physics,V ol.134.Berlin:S pringer,1996[21]Physica B,2000,281—282:736—786多篇文章[22]Mueller F M et al.Bull.Am.Phys.S oc.,1990,35:550[23]K ido G et al.Proc.2nd ISSP Int.Sym p.on Physics and Chem istryof Oxide Superconductors.T oky o:S pringer;Physica B,1992,177:46[24]Mueller F M.Physica B,1992,177:41[25]Nam S et al.Phys.Rev.Lett.,2001,87:117001・7・物理[26]W illiams F I B et al .Phys.Rev.Lett.,1991,66:3285;S ontos M Bet al .Phys.Rev.Lett.,1992,68:1188;R odgers P J et al .PhysicaB ,1993,184:95[27]Shayegan M et al .Phys.Rev.B ,1985,31:6123;Dupuis N ,M ont 2ambaux.Phys.Rev.Lett.,1992,68:357;Brossard L et al .Eur 2Phys.J.B ,1998,1:439[28]G lass on P et al .Phys.Rev.Lett.,2001,87:176802[29]Li C C et al .Phys.Rev.B ,2000,61:10905[30]K hmelnitskii D E.Phys Lett.,1984,106A :182[31]Jiang H W et al .Physica B ,1994,197:449[32]Fujiwara A et al .Physica B ,2001,298:541[33]Balicas L et al .Phys.Rev.Lett.,2001,87:067002[34]G arcia 2Landa B et al .Physica B ,2001,294—295:107;Hayashi Tet al .Physica B ,2001,294—295:115[35]Suzuki T et al .Physica B ,1995,206Π207:771[36]Sugiyama K et al .Physica B ,1992,177:275[37]M ontambaux G et al .Physica B ,1992,177:339[38]Chaikin P M et al .Physica B ,1992,177:353[39]Pedersen J et al .Nature ,1991,353:733;H ori H et al .Physica B ,2001,294—295:292・物理新闻・一种测试“复杂性”的新方法(A N ew W ay to Measuring Complexity ) 对于一个生物系统,我们应该如何去测定它的复杂性呢?最近美国哈佛大学医学院和葡萄牙里斯本大学的M.C osta 教授及其研究小组提出了一个新的设想,他们认为疾病与衰老可以用信息的损失来定量描述.换句话说,一个生物组织(或器官)的复杂性是与它对环境的适应性和它的功能性的发挥有着密切的关系,而疾病与衰老将会降低生物组织(器官)的复杂性,使得它们不容易适应环境的变化以及抵抗灾变事件的能力.但是传统对复杂性的描述常常是与这种“信息损失理论”相矛盾的,按信息科学的观点来说,一个系统的复杂性是由该系统能生成多少新的信息量来确定的.如果我们用一个具有心律不齐或者有心房颤动的病人作试验,从他的心电图上可发现其复杂性要比一个健康人大得多;这是因为在心脏病患者的心电图中可观察到许多的无规振荡(即白噪声),而无规振荡是可以连续不断地产生“新”信息量的.也就是说,心电图上前一时刻的心律跳动是无法预测他下一时刻的心律跳动的,因此这是一个具有较高复杂性的系统.与此相反,一个健康人的心电图中,他的心律跳动是遵守1Πf 噪声规则的,因此它所需的信息量较少,也就是它的复杂性程度较低.为什么会产生这种矛盾呢?M.C osta 教授的研究组认为,生物组织的内部存在着时间尺度上的多重性,因此对复杂性的量度需要用多标度的时间尺度来测定,为此他们在计算生物系统复杂性时使用了“多标度熵(multi 2scale entropy 简称MSE )”的新概念.具体的计算方法如下:将一个记录有30000次心律跳动的时间序列进行粗粒化,就是用20个跳动作为一个单位,计算出每一个单位的平均心律跳动,用这些平均数重构成一个新的时间序列并测出它的不可预测性,反复进行粗粒化并测定不可预测性.如果不可预测性高,表示信息量大,也就是该生物系统的复杂性程度高.他们采用不同长度的心律跳动(从2—20个)作为划分单位来重复计算其不可预测性.显然这种多尺度的测量方法可以揭示出信息量在不同时间尺度下的复杂排列.将一个健康的年青人与一个患有心律不齐和心房颤动的老年病人的心电图作对比,利用MSE 算法后,可以发现始终保持着健康的心脏要比衰老有病的心脏具有较高的复杂性.(云中客 摘自Phys.Rev.Lett.,5August 2002)・107・31卷(2002年)11期。
分形几何在Hamilton系统统计物理中的应用

分形几何在Hamilton系统统计物理中的应用分形几何(fractal geometry)在科学与数学领域中扮演着重要的角色。
它是一门研究非整数维度空间的几何学,其特点是重复自相似的形态,具有无限细节和复杂性。
在统计物理学中,分形几何被广泛应用于研究Hamilton系统(Hamiltonian systems)以及统计力学问题。
本文将介绍分形几何在Hamilton系统统计物理中的应用。
一、分形几何在Hamilton系统建模中的应用Hamilton系统是描述系统动力学的一种数学模型,它由Hamilton函数(Hamiltonian)和Hamilton方程组组成。
分形几何的自相似性特征使其成为研究Hamilton系统的理想工具。
通过分形几何建模,我们可以更好地理解和描述系统的自相似性行为,以及系统的不可序行为等重要性质。
例如,在分形几何中,分形维度是描述物体复杂性的一个重要参数。
在研究Hamilton系统时,我们可以通过计算系统的分形维度来揭示系统的动力学特征。
分形维度的计算方法可以通过使用分形维度估计算法,如盒计数法(box counting method)等来实现。
通过计算分形维度,我们可以获得有关系统自相似性的重要信息,从而对系统的动力学以及相变行为做出更精确的描述和预测。
二、分形几何在Hamilton系统相变问题中的应用相变是系统从一个相到另一个相的突变过程,广泛存在于自然界的各个领域。
在研究Hamilton系统的相变问题时,分形几何提供了一种有力的分析工具。
分形几何可以帮助我们分析系统在相变过程中的自相似性行为,进而揭示系统内部的临界行为。
例如,当系统接近临界点时,分形几何揭示了系统内部的自相似性结构,这对于我们理解系统的临界行为至关重要。
通过分形几何的分析方法,我们可以获得相变点附近的分形维度、分形特征等信息,从而更好地理解和预测系统相变的行为。
三、分形几何在Hamilton系统的混沌动力学中的应用混沌动力学是指一类极为复杂且高度敏感于初始条件的动力学系统。
朗之万动力学 英文
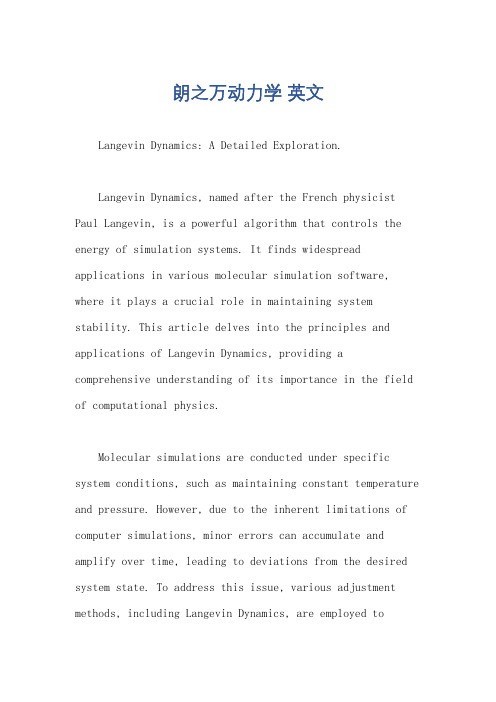
朗之万动力学英文Langevin Dynamics: A Detailed Exploration.Langevin Dynamics, named after the French physicist Paul Langevin, is a powerful algorithm that controls the energy of simulation systems. It finds widespread applications in various molecular simulation software, where it plays a crucial role in maintaining system stability. This article delves into the principles and applications of Langevin Dynamics, providing a comprehensive understanding of its importance in the field of computational physics.Molecular simulations are conducted under specific system conditions, such as maintaining constant temperature and pressure. However, due to the inherent limitations of computer simulations, minor errors can accumulate and amplify over time, leading to deviations from the desired system state. To address this issue, various adjustment methods, including Langevin Dynamics, are employed tocontinuously correct the system.The fundamental principle of Langevin Dynamics lies in its description of particles undergoing Brownian motion in a thermal bath. In this model, the microscopic details of particle-solvent interactions are ignored, and the interactions are instead described statistically. The collisions between the solvent molecules and the particles are represented by two components: a random force acting on the particle and a frictional term that slows down the particle's motion.The Langevin equation, which underlies Langevin Dynamics, is a dynamical equation that describes the motion of a Brownian particle. In this equation, the particle's mass (m), friction coefficient (ξ), and velocity (v(t)) are considered. The external force acting on the particleis denoted by F(t), while the random force is represented by X(t). The frictional term is proportional to theparticle's velocity, and the random force captures the solvent effects at a given temperature (T).In the context of molecular simulations, Langevin Dynamics offers several advantages. Firstly, it efficiently controls the system's energy, ensuring that it remains close to the desired value. Secondly, by introducing a frictional term, Langevin Dynamics simulates thedissipative effects of the surrounding medium, thus providing a more realistic representation of particle motion. Additionally, the random force accounts for the stochastic nature of interactions in the system, further enhancing the realism of the simulation.Langevin Dynamics finds widespread applications in various fields of computational physics. For instance, itis commonly used in simulations of soft matter systems, where it enables the study of complex behaviors such asself-assembly and phase transitions. Additionally, Langevin Dynamics has been employed in simulations of biological systems, including proteins and membranes, to understand their dynamic properties and interactions.In conclusion, Langevin Dynamics is a powerful algorithm that controls the energy of simulation systems,ensuring system stability and realism. Its fundamental principle lies in the description of particles undergoing Brownian motion in a thermal bath, where the microscopic details of particle-solvent interactions are ignored and described statistically. The Langevin equation, which underlies this dynamics, provides a mathematical framework for simulating particle motion under the influence of external forces, friction, and random interactions. The widespread applications of Langevin Dynamics in computational physics demonstrate its importance in understanding and modeling complex systems.。
QuantumATK机器学习强迫场与物理学模型说明书
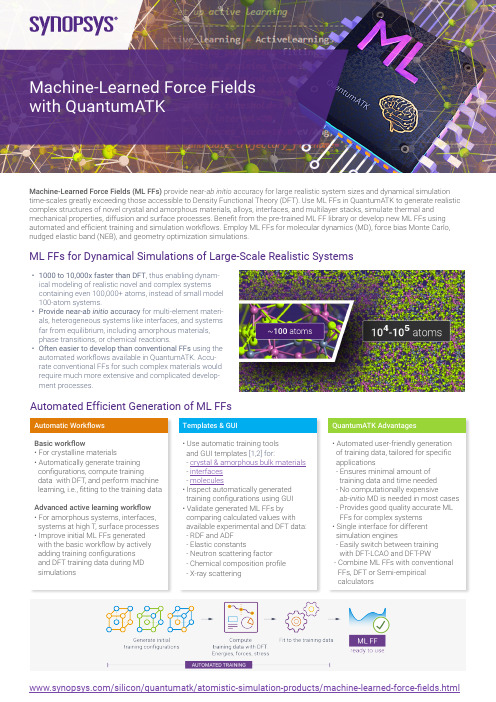
Machine-Learned Force Fields with QuantumATK/silicon/quantumatk/atomistic-simulation-products/machine-learned-force-fields.htmlTemplates & GUI • Use automatic training tools and GUI templates [1,2] for : - crystal & amorphous bulk materials - interfaces - molecules • Inspect automatically generated training configurations using GUI • Validate generated ML FFs by comparing calculated values with available experimental and DFT data: - RDF and ADF - Elastic constants - Neutron scattering factor - Chemical composition profile- X-ray scattering QuantumATK Advantages• Automated user-friendly generation of training data, tailored for specific applications - Ensures minimal amount of training data and time needed - No computationally expensive ab-initio MD is needed in most cases - Provides good quality accurate ML FFs for complex systems • Single interface for different simulation engines - Easily switch between training with DFT-LCAO and DFT-PW - Combine ML FFs with conventional FFs, DFT or Semi-empirical calculatorsAutomatic Workflows Basic workflow • For crystalline materials • Automatically generate training configurations, compute training data with DFT, and perform machine learning, i.e., fitting to the training data Advanced active learning workflow • For amorphous systems, interfaces, systems at high T, surface processes • Improve initial ML FFs generated with the basic workflow by actively adding training configurations and DFT training data during MDsimulations Machine-Learned Force Fields (ML FFs) provide near -ab initio accuracy for large realistic system sizes and dynamical simulation time-scales greatly exceeding those accessible to Density Functional Theory (DFT). Use ML FFs in QuantumATK to generate realistic complex structures of novel crystal and amorphous materials, alloys, interfaces, and multilayer stacks, simulate thermal and mechanical properties, diffusion and surface processes. Benefit from the pre-trained ML FF library or develop new ML FFs using automated and efficient training and simulation workflows. Employ ML FFs for molecular dynamics (MD), force bias Monte Carlo, nudged elastic band (NEB), and geometry optimization simulations.• 1000 to 10,000x faster than DFT , thus enabling dynam-ical modeling of realistic novel and complex systems containing even 100,000+ atoms, instead of small model 100-atom systems.• Provide near-ab initio accuracy for multi-element materi-als, heterogeneous systems like interfaces, and systems far from equilibrium, including amorphous materials, phase transitions, or chemical reactions.• Often easier to develop than conventional FFs using the automated workflows available in QuantumATK. Accu -rate conventional FFs for such complex materials would require much more extensive and complicated develop-ment processes.ML FFs for Dynamical Simulations of Large-Scale Realistic SystemsAutomated Efficient Generation of ML FFs2Synopsys QuantumATK Team Fruebjergvej 3DK-2100 Copenhagen DENMARK /quantumatk ***********************+45 333 32 300©2022 Synopsys, Inc. All rights reserved. Synopsys is a trademark of Synopsys, Inc. in the United States and other countries. A list of Synopsys trademarks is available at https:///copyright.html . All other names mentioned herein are trademarks of their respective owners.Application Examples of Machine-Learned Force FieldsStructure Generation of Amorphous Materials: Generate amorphous structures for PCRAM, ReRAM and FeRAM novel memories, solar cell and other applications. In this example, 80 ps ML FF - MD generated am-SiO 2 structure of 600 atoms in 11 minutes, whereas it took 10 days to generate 72-atom structure with DFT-MD on 16 cores. Structural parameters obtained with ML FFs are in a good agreement with DFT and experimental results.development applications, such as high-k metal gate (HKMG) (using Multilayer builder GUI ) and MRAM magnetic tunnel junction engineering. This example shows a generated structure of Structure Generation of Glassy Amorphous Materials: Generate glassy amorphous materials with impurities for optoelectronic applications. In this example, ML FF – MD is used to simulate a large- scale 120,000 atom size sodium silicate glass with Na impurities, (Na 2O)2(SiO 2)40000 at 2500 K.Thermal Property Simulations : Simulate thermal conductance using ML FFs with ns -long reverse non-equilibrium MD (RNEMD) simulations for developing PCRAM and evaluating self-heating and heat dissipation in devices. Examples include simulating thermal conductance in bulk Ge 2Sb 2Te 5 (2300 atoms), Ge 2Sb 2Te 5/Si (882 atoms) and Si/GaAs (864 atoms) interfaces, monolayer MoS 2(108,000 atoms). Calculated values are in a good agreement with experimentaland DFT results where available.Built-in Library of Ready-to-Use Machine-Learned Force Fields►QuantumATK offers Moment Tensor Potentials (MTPs) ML FFs implemented by the QuantumATK team in-house. ►MTPs provide high robust accuracy with lower computational cost compared to other ML FFs [3,4]. ►Benefit from the pre-trained ready-to-use high-quality MTP library [5,6] or develop MTPs for new materials, interfaces and surface processes by using automatic generation workflows.Tutorial and video on automatic ML FF training tools and GUI templates[1] Tutorial: https:///tutorials/mtp_hfo2/mtp_hfo2.html [2] Video: https:///watch?v=6BrrVotzjnc[3] A. V. Shapeev. Moment tensor potentials: a class of systematically improvable interatomic potentials. Multiscale Model. & Simul. 14, 1153 (2016).[4] Y. Zuo, C. Chen, X. Li, Z. Deng, Y. Chen, J. Behler, G. Csányi, A. V. Shapeev, A. P . Thompson, M. A. Wood, and S. Ping Ong. Performance and cost assessment of machine learning interatomic potentials . J. Phys. Chem. A 124, 731(2020).[5] ML FF features: https:///silicon/quantumatk/resources/feature-list.html#MLforcefield[6] Materials in ML FF library: https:///manual/ForceField.html#pretrained-moment-tensor-potential-mtp-parameter-sets。
- 1、下载文档前请自行甄别文档内容的完整性,平台不提供额外的编辑、内容补充、找答案等附加服务。
- 2、"仅部分预览"的文档,不可在线预览部分如存在完整性等问题,可反馈申请退款(可完整预览的文档不适用该条件!)。
- 3、如文档侵犯您的权益,请联系客服反馈,我们会尽快为您处理(人工客服工作时间:9:00-18:30)。
Institute for Theoretical Physics, University of Innsbruck, Innsbruck A-6020, Austria Institute for Quantum Optics and Quantum Information of the Austrian Academy of Sciences, A-6020 Innsbruck, Austria 3 Department of Physics, University of Auckland, Private Bag 92019, Auckland, New Zealand (Dated: February 4, 2008) We present an optical cavity QED configuration that is described by a dissipative version of the Lipkin-Meshkov-Glick model of an infinitely coordinated spin system. This open quantum system exhibits both first- and second-order non-equilibrium quantum phase transitions as a single, effective field parameter is varied. Light emitted from the cavity offers measurable signatures of the critical behavior, including that of the spin-spin entanglement.
Given these interesting and topical features of the LMG model, it follows that the physical realization of a system described by such a model would provide a valuable test bed for studies of quantum critical phenomena and entanglement. Here we propose an open-system (i.e., dissipative) version of the LMG model based on the collective interaction of an ensemble of atoms with laser fields and field modes of a high-finesse optical resonator. In the spirit of a recent proposal for realizing the Dicke model [12], our scheme employs Raman transitions between a pair of atomic ground states and the relevant energy scales (e.g., h, λ) are set by light shifts of the atomic levels and Raman transition rates and detunings. The open nature of this system, a consequence of the external driving fields and cavity mode losses, introduces a number of important differences from, and, arguably, advantages over, the closed, Hamiltonian LMG system: (i) thermal equilibrium phase transitions are replaced by dynamical, non-equilibrium phase transitions, (ii) the cavity output fields offer quantitative measures of properties of the collective-spin system, including entanglement, in the critical regime, and (iii) it is possible to observe both first- and second-order quantum phase transitions as a single effective field parameter, h, is varied. We consider N atoms coupled via electric dipole transitions to three laser fields and to a pair of independent (e.g., orthogonally-polarized) optical cavity modes. The atomic level and excitation scheme is shown in Fig. 1, together with a possible ring-cavity setup. At the location of the atoms, the cavity and laser fields are copropagating traveling waves, with sufficiently broad beam waists so as to ensure homogeneous atom-field couplings. These fields combine to drive Raman transitions between two stable electronic ground states of the atoms, |0 and |1 (energies ω0 = 0 and ω1 , respectively, with = 1) via the excited atomic states |r and |s (energies ωr and ωs ). The laser fields have optical frequencies ωr0 , ωs0 , and ωr1 , and couple to the atomic transitions with Rabi frequencies Ωr0 , Ωs0 , and Ωr1 . Cavity field a, at frequency ωa , couples to the transitions |0 ↔ |r and
2 2 HLMG = −2hJz − (2λ/N )(Jx + γJy ),
(1)
where {Jx , Jy , Jz } are collective angular momentum operators for N spin-1/2 particles, h and λ are effective magnetic field and spin-spin interaction strengths, respectively, and γ ∈ [−1, 1] is an anisotropy parameter. This system, in which each spin interacts identically with every other spin, exhibits critical behavior at zero temperature; in particular, either first- or second-order equilibrium quantum phase transitions may occur, depending on the choice of λ and γ , as the ratio h/λ is varied across a critical value [6]. Notably, the second-order transition involves a change from a unique ground state (normal phase) to a pair of macroscopically displaced degenerate ground states (broken phase). Entanglement in the system displays the above-mentioned critical behavior, reaching, in particular, a pronounced maximum at the critical p3v2 [quant-ph] 1 Feb 2008
PACS numbers: 42.50.Fx, 42.50.Pq, 03.65.Ud, 73.43.Nq
Remarkable advances with trapped, ultra-cold atomic gases have opened up exciting new avenues of research into strongly interacting many-body quantum systems [1]. Exquisite control of both motional and electronic degrees of freedom of cold atoms can enable one to “tailor” atom-atom interactions and thereby implement a variety of systems that exhibit, in particular, quantum critical phenomena, i.e., transitions between distinct quantum phases, driven by quantum fluctuations, in response to variations of an effective field or interaction strength around some critical value. Recently, important insights into such transitions have been obtained from theoretical studies of the quantum entanglement properties of critical spin systems (see, e.g., [2, 3, 4, 5, 6, 7, 8, 9, 10]). Bipartite entanglement measures characterizing entanglement between a pair of spins (e.g., the concurrence) or between two blocks of spins (e.g., the entanglement entropy) can display marked critical behavior and scaling at quantum critical points. In this context, a simple but very useful example is the Lipkin-Meshkov-Glick (LMG) model [11], which is described by the Hamiltonian