铝合金中的析出相共106页文档
6系铝合金析出相mg2si -回复
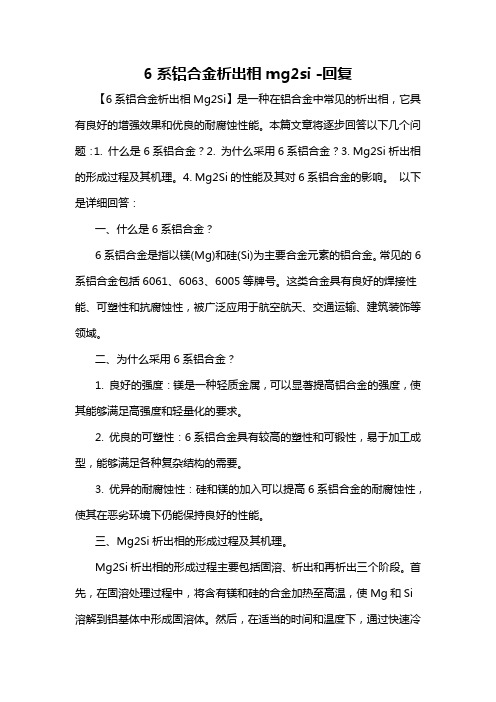
6系铝合金析出相mg2si -回复【6系铝合金析出相Mg2Si】是一种在铝合金中常见的析出相,它具有良好的增强效果和优良的耐腐蚀性能。
本篇文章将逐步回答以下几个问题:1. 什么是6系铝合金?2. 为什么采用6系铝合金?3. Mg2Si析出相的形成过程及其机理。
4. Mg2Si的性能及其对6系铝合金的影响。
以下是详细回答:一、什么是6系铝合金?6系铝合金是指以镁(Mg)和硅(Si)为主要合金元素的铝合金。
常见的6系铝合金包括6061、6063、6005等牌号。
这类合金具有良好的焊接性能、可塑性和抗腐蚀性,被广泛应用于航空航天、交通运输、建筑装饰等领域。
二、为什么采用6系铝合金?1. 良好的强度:镁是一种轻质金属,可以显著提高铝合金的强度,使其能够满足高强度和轻量化的要求。
2. 优良的可塑性:6系铝合金具有较高的塑性和可锻性,易于加工成型,能够满足各种复杂结构的需要。
3. 优异的耐腐蚀性:硅和镁的加入可以提高6系铝合金的耐腐蚀性,使其在恶劣环境下仍能保持良好的性能。
三、Mg2Si析出相的形成过程及其机理。
Mg2Si析出相的形成过程主要包括固溶、析出和再析出三个阶段。
首先,在固溶处理过程中,将含有镁和硅的合金加热至高温,使Mg和Si 溶解到铝基体中形成固溶体。
然后,在适当的时间和温度下,通过快速冷却或自然冷却使合金中的镁和硅重新聚集,形成Mg2Si析出相。
最后,经过再析出处理,通过热处理使Mg2Si的析出相变得更加均匀、细小,提高合金的力学性能。
Mg2Si析出相的形成机理是基于固溶体和析出相的热力学相互作用。
当合金冷却至固溶体的过饱和度时,析出相开始形成,其过程受到扩散速率的限制。
镁和硅原子在铝基体中的扩散会导致析出相的形核和生长,而固溶体中的晶格畸变和弹性应变则对其扩散和位错形成起到促进作用。
这种热力学和力学的相互作用使得Mg2Si析出相在合金中形成。
四、Mg2Si的性能及其对6系铝合金的影响。
Mg2Si析出相在6系铝合金中具有以下几个重要的性能和影响:1. 增强效果:Mg2Si析出相可以显著提高铝合金的强度和硬度,使其具有良好的抗拉、屈服和延展性能。
6系铝合金析出相mg2si
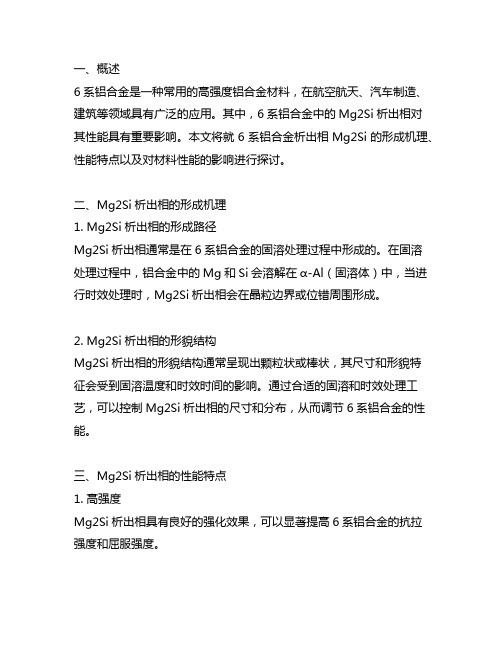
一、概述6系铝合金是一种常用的高强度铝合金材料,在航空航天、汽车制造、建筑等领域具有广泛的应用。
其中,6系铝合金中的Mg2Si析出相对其性能具有重要影响。
本文将就6系铝合金析出相Mg2Si的形成机理、性能特点以及对材料性能的影响进行探讨。
二、Mg2Si析出相的形成机理1. Mg2Si析出相的形成路径Mg2Si析出相通常是在6系铝合金的固溶处理过程中形成的。
在固溶处理过程中,铝合金中的Mg和Si会溶解在α-Al(固溶体)中,当进行时效处理时,Mg2Si析出相会在晶粒边界或位错周围形成。
2. Mg2Si析出相的形貌结构Mg2Si析出相的形貌结构通常呈现出颗粒状或棒状,其尺寸和形貌特征会受到固溶温度和时效时间的影响。
通过合适的固溶和时效处理工艺,可以控制Mg2Si析出相的尺寸和分布,从而调节6系铝合金的性能。
三、Mg2Si析出相的性能特点1. 高强度Mg2Si析出相具有良好的强化效果,可以显著提高6系铝合金的抗拉强度和屈服强度。
2. 硬度提高Mg2Si析出相的形成可以提高合金的硬度,从而增加其抗磨损性能。
3. 热稳定性Mg2Si析出相在高温下具有良好的热稳定性,能够有效抵抗高温下的软化和失稳现象。
四、Mg2Si析出相对材料性能的影响1. 强化效果Mg2Si析出相的形成可以有效提高6系铝合金的强度和硬度,改善材料的力学性能。
2. 热稳定性由于Mg2Si析出相的形成,6系铝合金在高温条件下仍能保持较高的强度和硬度,具有良好的耐热性能。
3. 形貌结构Mg2Si析出相的形貌结构对材料的韧性、断裂韧度等性能也有一定影响,需要进一步研究和优化。
五、总结与展望6系铝合金析出相Mg2Si对材料的性能有着重要影响,其形成机理、性能特点以及对材料性能的影响值得深入研究和探讨。
在今后的研究中,可以进一步优化合金的固溶和时效处理工艺,以期获得更好的Mg2Si析出相形貌和分布特征,从而提高6系铝合金的性能和应用范围。
六、参考文献[1] 王大勇. 6系铝合金析出相Mg2Si的研究进展[J]. 有色金属科学与工程, 2019(3): 15-19.[2] 张伟, 李静. 固溶处理对6061铝合金析出相Mg2Si形貌结构及性能的影响[J]. 材料工程, 2018, (6): 99-103.七、Mg2Si析出相的形貌结构和性能调控1. 形貌结构调控Mg2Si析出相的形貌结构对材料性能具有重要影响,因此在合金的制备过程中需要对其形貌结构进行有效调控。
al-mg-si-cu合金中晶界和晶内析出相粗化规律的研究

al-mg-si-cu合金中晶界和晶内析出相粗化
规律的研究
针对Al-Mg-Si-Cu合金中晶界和晶内析出相粗化规律的研究,可以从以下几个方面展开:
1. 合金中晶界析出相的粗化规律:晶界是合金中强化的薄弱区域,晶界析出相的粒子尺寸和分布对合金性能有重要影响。
可以通过扫描电镜观察晶界析出相的尺寸和形貌变化,然后通过粒度分析来定量描述其粗化过程。
实验上,可以制备具有不同热处理时间的样品,随后采用金相显微镜和透射电镜对晶界析出相进行观察和分析,得到粗化规律。
2. 合金中晶内析出相的粗化规律:晶内析出相是合金中颗粒状的强化相,其粒子尺寸和分布对合金的强度和耐磨性能影响较大。
同样通过金相显微镜和透射电镜观察晶内析出相的尺寸和形貌变化,并通过粒度分析来定量描述粗化过程。
可以制备具有不同热处理时间的样品,然后在室温下对其进行退火,观察晶内析出相的演化过程。
3. 影响晶界和晶内析出相粗化的因素:合金中晶界和晶内析出相的粗化受多种因素的影响,包括合金成分、热处理过程、应力等。
可以通过改变合金成分、热处理温度和时间,以及引入应力场等方式来探究这些因素对晶界和晶内析出相粗化的影响。
总的来说,在研究Al-Mg-Si-Cu合金中晶界和晶内析出相粗化规律时,需要综合运用金相显微镜、扫描电镜、透射电镜等分析技术,结合理论计算和实验探究方法,从不同角度和尺度对晶界和晶内析出相进行观察和分析,以揭示其粗化规律和影响因素,为合金优化设计和应用提供科学依据。
优选铝合金中的析出相
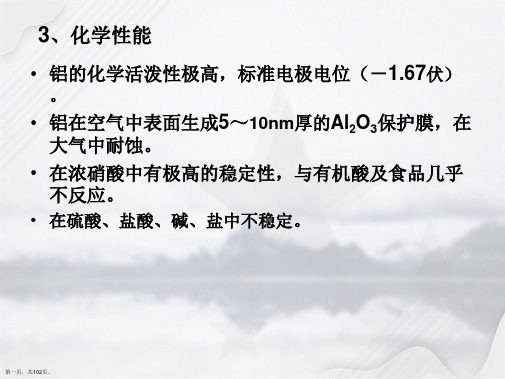
耐热硬铝: LY2: 较好的高温性能,用于制造在较高温度(150~250℃)
下工作的构件,如航空发动机内的压气机叶片。
第三十一页,共102页。
二、超硬铝 (Al-Zn-Mg-Cu系合金)
超硬铝是在Al-Zn-Mg合金基础上加Cu发展起来 的,它的强度超过硬铝,可达600~700MPa,所以称超 硬铝。第二次世界大战后,才开始大批生产和应用。 调质的45钢:σb=780~850 MPa
β:Mg2Al3
正方晶格
斜方晶格
立方晶格
面心立方
第二十二页,共102页。
随Mg含量增加,θ相减少,S相增加
Cu/Mg ≥8,
主要是θ相
Cu/Mg=8~4, 主要是θ+S相
Cu/Mg=4~1.5, 主要是S相,
S相中Cu/Mg=2.61
θ相析出序列的GP区和θ′是圆片状 S相析出序列的GP区和S′是针状
T7- 固溶处理,人工时效到过时效状态(稳定化处理的状态)
T8- 固溶处理后冷加工,然后进行人工时效的状态 T9- 固溶处理、人工时效、然后冷加工的状态
T10-从高温成形工序冷却,然后冷加工并进行人工时效的状态
TX51-通过拉伸消除应力的状态
TX52 - 通过压缩消除应力的状态 TX54 - 通过拉伸和压缩相结合的方法消除应力的状态
第十六页,共102页。
第二节 变形铝合金
简单地说:
硬铝
超硬铝 锻铝 防锈铝
综合机械性能好(不耐蚀)
室温强度最高 热塑性好 耐蚀性好,易成形,焊接性好(强度低)
第十七页,共102页。
第十八页,共102页。
一、 硬铝
1、一般特点 较好的综合机械性能
6系铝合金析出相mg2si -回复

6系铝合金析出相mg2si -回复“6系铝合金析出相Mg2Si”是一种理想的强化相,在铝合金中起到了重要的作用。
本文将一步一步回答有关这种析出相的相关问题,详细介绍其形成机制、影响因素以及应用等方面的知识。
首先,让我们从6系铝合金的基本概念开始。
6系铝合金是指以镁和硅作为合金元素的铝合金。
其中,镁的含量在1.2~2.2范围内,而硅的含量通常在0.3~1.0之间。
6系铝合金具有良好的强度、耐腐蚀性和可焊性等优点,广泛应用于航空航天、汽车、铁路、船舶和电子等领域。
接下来,我们将重点关注6系铝合金中的析出相Mg2Si。
Mg2Si是一种硬质、高熔点的铝镁硅化合物,具有良好的强化效果。
通过适当的热处理,可以在合金中形成均匀分布的Mg2Si相,从而提高铝合金的强度和硬度。
然而,要了解Mg2Si析出相的形成机制,我们需要深入研究合金的相变过程。
在一系列高温固溶热处理之后,6系铝合金中的Mg和Si溶解在铝基体中。
当合金经过快速冷却或在室温下保温时,Mg和Si会形成固溶体,其中一部分Mg会分解为Mg2Si析出相。
影响Mg2Si析出相形成的因素很多,包括合金成分、热处理工艺和冷却速率等。
首先,合金中镁和硅的含量要适中。
镁含量较低时,析出相的数量和尺寸都会减少,导致强化效果不明显。
而镁含量过高时,又会使得析出相聚集在一起,导致合金的塑性降低。
其次,合金的热处理工艺也起到了重要的影响。
适当的固溶温度和时间可以促进Mg和Si固溶在基体中,并为后续的析出相形成提供条件。
冷却速率也是一个关键因素,较快的冷却速率有助于形成细小均匀的Mg2Si析出相。
Mg2Si析出相在6系铝合金中的应用非常广泛。
首先,其强化效果可以显著提高合金的强度和硬度,使得合金在高温和冷变形条件下都具有优异的机械性能。
其次,Mg2Si析出相还可以提高合金的耐蚀性,使得合金在恶劣环境中具有更长的使用寿命。
此外,Mg2Si的形成还可以改善合金的抗热松弛性能,提高合金在高温下的稳定性。
铝合金中的析出相资料
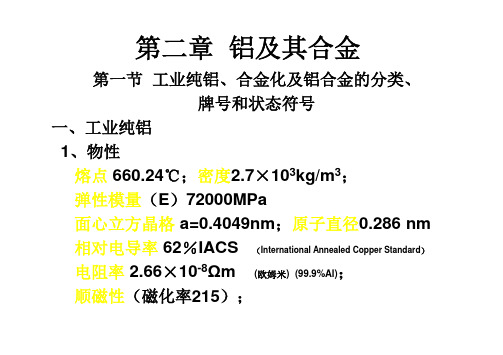
第二章铝及其合金第一节工业纯铝、合金化及铝合金的分类、牌号和状态符号一、工业纯铝1、物性熔点660.24℃;密度2.7×103kg/m3;弹性模量(E)72000MPa面心立方晶格a=0.4049nm;原子直径0.286nm 相对电导率62%IACS(International Annealed Copper Standard)电阻率2.66×10-8Ωm (欧姆米)(99.9%Al);顺磁性(磁化率215);3、化学性能•铝的化学活泼性极高,标准电极电位(-1.67伏)。
•铝在空气中表面生成5~10nm厚的Al2O3保护膜,在大气中耐蚀。
•在浓硝酸中有极高的稳定性,与有机酸及食品几乎不反应。
•在硫酸、盐酸、碱、盐中不稳定。
4、特点•质量轻•优秀的导电、传热和塑性变形性能•在大气中有良好的耐蚀性•强度低不适于作结构材料二、铝的合金化•合金化原理主要固溶强化和时效强化•固溶强化:元素溶解度大,与Al原子直径差大,例如Mg和Mn•时效强化:所加元素或形成的中间相,高温时在Al中有较大的溶解度,随温度降低溶解度急剧变小。
常加入的元素为Zn、Mg、Cu、Si、Li。
铝合金常加入的元素为Zn、Mg、Cu、Si、Li。
•在合金中可能形成:θ-CuAl2S-Al2CuMgη-MgZn2T-Al2Mg3Zn3β-Mg2Si δ-AlLiβ-Mg2Al3铝中的主要杂质:Fe、Si为了改善合金的塑性和抗蚀性,合金中常加入Mn、Cr、Zr、Ti、Cu等微量元素。
三、分类、牌号和状态符号(1)我国铝及其合金过去的分类和牌号:采用汉语拼音加阿拉伯数字表示纯铝:LG工业高纯铝;L工业纯铝变形铝合金分类及牌号:(L)(类)(序号)(状态)(2)美国变形铝合金牌号及状态牌号:用四位阿拉伯数字表示第一位数表示合金系(即加入最多的那种元素)第二位数表示原始合金或改进合金,0为原始合金,改进合金依次为1、2、3等最后两位数表示具体合金牌号,对于纯铝表示小数点后两位铝含量(1145-99.45%Al,1200-99.00%Al)目前我国变形铝合金牌号表示方法基本与美国相同,不同之处在于第二位不用阿拉伯数字,而是用英文字母:例如:7A04、7B04状态:F-加工态(热轧、挤压),不控制应变硬化量O-退火再结晶状态,强度最低、塑性最高W-固溶处理正在自然时效过程(不稳定)H-冷作硬化状态T-热处理状态应变硬化状态:H1-应变硬化。
铝合金中的析出相 ppt课件
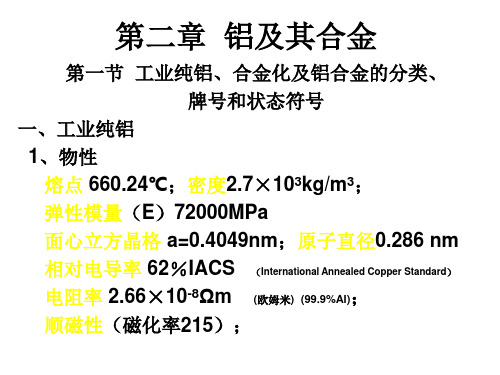
第二章 铝及其合金
第一节 工业纯铝、合金化及铝合金的分类、 牌号和状态符号
一、工业纯铝 1、物性 熔点 660.24℃;密度2.7×103kg/m3; 弹性模量(E)72000MPa 面心立方晶格 a=0.4049nm;原子直径0.286 nm 相对电导率 62%IACS (International Annealed Copper Standard) 电阻率 2.66×10-8Ωm ; (欧姆米) (99.9%Al) 顺磁性(磁化率215);
表示方法基本与美国相同,不同之处在于第 二位不用阿拉伯数字,而是用英文字母:
例如:7A04、7B04
状态:
F-加工态(热轧、挤压),不控制应变硬化量 O-退火再结晶状态,强度最低、塑性最高 W-固溶处理正在自然时效过程(不稳定) H-冷作硬化状态 T-热处理状态
应变硬化状态:
H1-应变硬化。 H2-应变硬化加不完全退火。 H3-应变硬化稳定处理。 H112-加工过程的应变硬化(不控制应变量)。 H321-加工过程的应变硬化(控制应变量)。 H116-特殊应变硬化。
有时加:Ti细化铸态晶粒
Be提高氧化膜的致密性,防止Mg的烧损。
典型合金的化学成分:
LY12(相当2024)Al-4.3Cu-1.5Mg-0.6Mn
LY11(相当2017)Al-4.3Cu-0.6Mg-0.6Mn
LY2
Al-2.9Cu-2.2Mg-0.6Mn
4、硬铝的热处理
除生产工序中的热处理外,硬铝的主要热处理是淬火时效
θ:CuAl2 S:Al2CuMg T:Mg32(CuAl)49
2系铝合金过饱和固溶体的析出序列
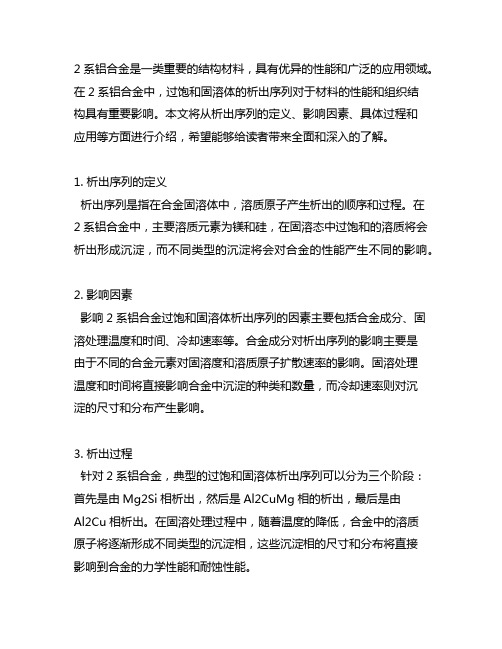
2系铝合金是一类重要的结构材料,具有优异的性能和广泛的应用领域。
在2系铝合金中,过饱和固溶体的析出序列对于材料的性能和组织结构具有重要影响。
本文将从析出序列的定义、影响因素、具体过程和应用等方面进行介绍,希望能够给读者带来全面和深入的了解。
1. 析出序列的定义析出序列是指在合金固溶体中,溶质原子产生析出的顺序和过程。
在2系铝合金中,主要溶质元素为镁和硅,在固溶态中过饱和的溶质将会析出形成沉淀,而不同类型的沉淀将会对合金的性能产生不同的影响。
2. 影响因素影响2系铝合金过饱和固溶体析出序列的因素主要包括合金成分、固溶处理温度和时间、冷却速率等。
合金成分对析出序列的影响主要是由于不同的合金元素对固溶度和溶质原子扩散速率的影响。
固溶处理温度和时间将直接影响合金中沉淀的种类和数量,而冷却速率则对沉淀的尺寸和分布产生影响。
3. 析出过程针对2系铝合金,典型的过饱和固溶体析出序列可以分为三个阶段:首先是由Mg2Si相析出,然后是Al2CuMg相的析出,最后是由Al2Cu相析出。
在固溶处理过程中,随着温度的降低,合金中的溶质原子将逐渐形成不同类型的沉淀相,这些沉淀相的尺寸和分布将直接影响到合金的力学性能和耐蚀性能。
4. 应用2系铝合金在航天航空、汽车制造、电子器件等领域具有广泛的应用。
对于不同的工程应用,我们可以通过控制过饱和固溶体析出序列来调节合金的力学性能、电学性能和耐蚀性能,从而满足不同领域的需求。
总结:2系铝合金过饱和固溶体的析出序列对于合金的性能和组织结构具有重要的影响。
通过对析出序列的深入研究和探讨,我们可以更好地了解合金的特性,指导合金的制备和工程应用,促进2系铝合金材料的发展和应用。
5. 控制方法控制2系铝合金过饱和固溶体析出序列的方法通常包括固溶处理和时效处理。
固溶处理是通过加热合金至固溶温度,使得固溶体中的溶质元素溶解形成均匀的固溶体。
而时效处理则是在固溶处理后,通过控制时间和温度使得沉淀相在合金中析出,从而调节合金的性能。
Al-Mg-Sc-Zr合金中初生相的析出行为
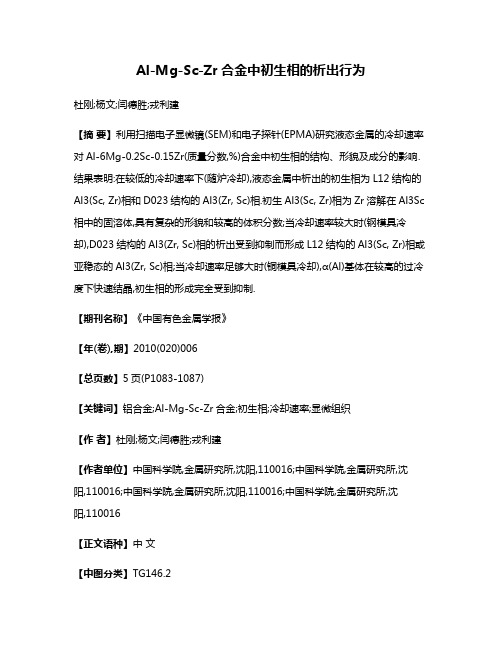
Al-Mg-Sc-Zr合金中初生相的析出行为杜刚;杨文;闫德胜;戎利建【摘要】利用扫描电子显微镜(SEM)和电子探针(EPMA)研究液态金属的冷却速率对Al-6Mg-0.2Sc-0.15Zr(质量分数,%)合金中初生相的结构、形貌及成分的影响.结果表明:在较低的冷却速率下(随炉冷却),液态金属中析出的初生相为L12结构的Al3(Sc, Zr)相和D023结构的Al3(Zr, Sc)相.初生Al3(Sc, Zr)相为Zr溶解在Al3Sc 相中的固溶体,具有复杂的形貌和较高的体积分数;当冷却速率较大时(钢模具冷却),D023结构的Al3(Zr, Sc)相的析出受到抑制而形成L12结构的Al3(Sc, Zr)相或亚稳态的Al3(Zr, Sc)相;当冷却速率足够大时(铜模具冷却),α(Al)基体在较高的过冷度下快速结晶,初生相的形成完全受到抑制.【期刊名称】《中国有色金属学报》【年(卷),期】2010(020)006【总页数】5页(P1083-1087)【关键词】铝合金;Al-Mg-Sc-Zr合金;初生相;冷却速率;显微组织【作者】杜刚;杨文;闫德胜;戎利建【作者单位】中国科学院,金属研究所,沈阳,110016;中国科学院,金属研究所,沈阳,110016;中国科学院,金属研究所,沈阳,110016;中国科学院,金属研究所,沈阳,110016【正文语种】中文【中图分类】TG146.2合金元素Sc具有强烈细化Al合金晶粒的作用。
当Al合金中Sc的含量超过共晶成分时,液相中能够形成大量的初生Al3Sc相颗粒并成为α(Al)基体的异质形核中心[1-3]。
加入Zr或Ti后不但明显降低形成初生相所需的最低 Sc含量还可以获得更好的细化晶粒的效果[4-5]。
YIN 等[6]研究发现铸态 Al-5Mg-0.2Sc-0.1Zr(质量分数,%) 合金的晶粒尺寸可被细化至42 μm,而Al-5Mg-0.6Sc(质量分数,%)合金的平均晶粒尺寸为72 μm。
2024铝合金中s相析出演化规律的tem研究 -回复
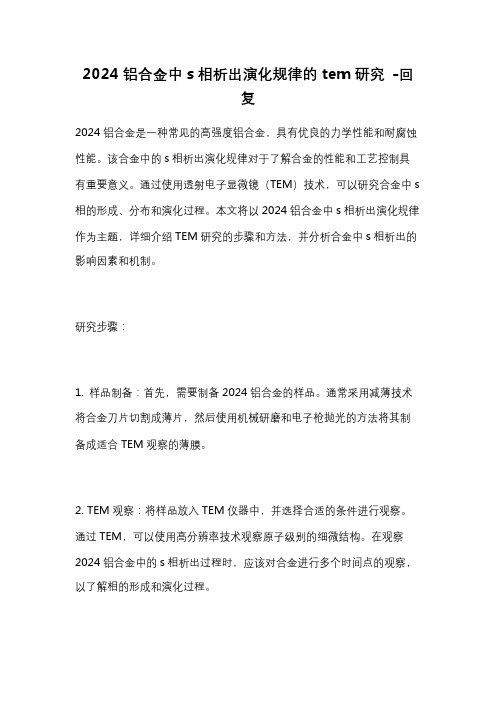
2024铝合金中s相析出演化规律的tem研究-回复2024铝合金是一种常见的高强度铝合金,具有优良的力学性能和耐腐蚀性能。
该合金中的s相析出演化规律对于了解合金的性能和工艺控制具有重要意义。
通过使用透射电子显微镜(TEM)技术,可以研究合金中s 相的形成、分布和演化过程。
本文将以2024铝合金中s相析出演化规律作为主题,详细介绍TEM研究的步骤和方法,并分析合金中s相析出的影响因素和机制。
研究步骤:1. 样品制备:首先,需要制备2024铝合金的样品。
通常采用减薄技术将合金刀片切割成薄片,然后使用机械研磨和电子枪抛光的方法将其制备成适合TEM观察的薄膜。
2. TEM观察:将样品放入TEM仪器中,并选择合适的条件进行观察。
通过TEM,可以使用高分辨率技术观察原子级别的细微结构。
在观察2024铝合金中的s相析出过程时,应该对合金进行多个时间点的观察,以了解相的形成和演化过程。
3. 形貌分析:通过TEM图像,可以观察到合金中s相的形貌。
根据图像的对比度和形状,可以初步确定s相的分布以及其形成方式。
此外,还可以使用扫描电子显微镜(SEM)来观察样品表面的细微结构,并结合EDS(能谱分析仪)技术来确定元素的分布情况。
4. 组织分析:通过TEM观察,可以区分合金中的晶粒和析出相。
通过选取合适的位置,使用选择区域电子衍射(SAED)技术来获取材料的衍射图像和衍射纹。
通过对衍射图像的解析,可以得到合金中晶粒的晶体结构和取向信息,从而进一步了解s相的形成机制。
5. 化学成分分析:通过TEM结合EDS技术,可以分析样品中的元素组成。
s相通常富含镍和铬等元素,通过定量分析EDS谱线的峰强度,可以得到s相的化学成分。
此外,还可以使用电子能量损失谱(EELS)技术来获取更准确的化学成分信息。
影响因素和机制分析:1. 成分效应:2024铝合金中添加的合金元素以及合金的化学成分对s相析出过程有重要影响。
例如,合金中的铜含量越高,会促使更多的s相析出。
al-mg-si-cu合金中晶界和晶内析出相粗化规律的研究

al-mg-si-cu合金中晶界和晶内析出相粗化规律的研究近年来,对于Al-Mg-Si系合金中晶界和晶内析出相粗化规律的研究得到了广泛的关注。
Al-Mg-Si系合金具有良好的力学性能和耐蚀性,因此在航空航天、汽车制造等领域具有广泛的应用潜力。
然而,晶界和晶内析出相的粗化会降低合金的力学性能和耐蚀性能,因此研究其粗化规律具有重要的科学意义和工程应用价值。
首先,晶界和晶内析出相的粗化是由原子扩散控制的。
晶界处原子扩散速率较快,且由于晶界具有高曲率,因此晶界附近的析出相较于晶内的析出相更容易粗化。
此外,晶界对原子扩散的阻碍作用也会导致晶界附近的析出相粗化。
通过对合金的热处理和退火等方法,可以促进晶界和晶内的析出相粗化。
其次,晶界和晶内析出相的粗化与合金的成分和热处理参数有关。
研究表明,Al-Mg-Si系合金中Mg和Si的含量以及合金的热处理温度和时间等参数都会影响晶界和晶内析出相的粗化。
适当的合金化元素添加和优化的热处理工艺可以有效地抑制析出相的粗化,提高合金的力学性能和耐蚀性能。
此外,晶界和晶内析出相的粗化与材料的应力状态和应变速率也有关。
研究表明,应力和应变可以通过改变晶界和晶内的原子扩散速率来影响析出相的粗化。
较低的应力和应变速率有利于阻止析出相的粗化,而较高的应力和应变速率则有助于促进析出相的粗化。
因此,合理的应力控制和应变速率可以有效地控制晶界和晶内析出相的粗化。
最后,晶界工程和控制晶内析出相的成核和生长是抑制晶界和晶内析出相粗化的有效方法。
晶界工程通过调控晶界的位置、密度和迁移速率等参数来抑制晶界附近的析出相的粗化。
控制晶内析出相的成核和生长可以通过合金化元素的加入、合金的热处理工艺以及外加应力等方法实现。
这些方法可以有效地控制晶界和晶内析出相的粗化,提高合金的性能。
综上所述,Al-Mg-Si系合金中晶界和晶内析出相粗化规律的研究是非常重要的。
该研究可以为合金的设计和制备提供理论指导,为合金的应用和发展提供技术支持。
X2A66铝锂合金时效过程中析出相演变及强化机制

第43卷第5期㊀㊀㊀㊀㊀㊀㊀㊀㊀㊀㊀㊀㊀固体火箭技术JournalofSolidRocketTechnology㊀㊀㊀㊀㊀㊀㊀㊀㊀㊀㊀㊀㊀㊀Vol.43No.52020X2A66铝锂合金时效过程中析出相演变及强化机制①史国栋1,2,周㊀俊3,王云峰4,王圆圆3,陆㊀政5,陈子勇1(1.北京工业大学材料学院,北京㊀100124;2.大连科天新材料有限公司,大连㊀116023;3.万丰奥特控股集团有限公司,新昌㊀312500;4.威海万丰镁业科技发展有限公司,威海㊀264209;5.北京航空材料研究院,北京㊀100095)㊀㊀摘要:通过透射电镜研究了X2A66铝锂合金单极时效过程中析出相的演变并进行量化统计,讨论了析出相的强化机制,结果表明,X2A66铝锂合金在165ħ单级人工时效的欠时效至峰值时效过程中析出相的析出序列为:α过饱和固溶体ңGP区+δ'+GPT1ңδ'+θ'+GPT1ңθ'+T1+δ'㊂时效温度为165ħ,时效时间为72h是X2A66铝锂合金的峰值时效,此时抗拉强度为591MPa,延伸率为6.88%㊂T1相为X2A66铝锂合金时效过程中的主要强化相,位错与T1相间的交互作用为切过和绕过的混合型㊂在峰值时效条件下,T1相的体积分数为17%,T1相强度增量为124.8MPa,T1相的强化贡献占析出相强化比值约为74.17%㊂关键词:X2A66铝锂合金;时效;析出相;强化机制中图分类号:V252㊀㊀㊀文献标识码:A㊀㊀㊀文章编号:1006⁃2793(2020)05⁃0618⁃06DOI:10.7673/j.issn.1006⁃2793.2020.05.011EvolutionandstrengtheningmechanismofprecipitatedphasesofX2A66aluminum⁃lithiumalloyduringagingSHIGuodong1,2,ZHOUJun3,WANGYunfeng4,WANGYuanyuan3,LUZheng5,CHENZiyong1∗(1.BeijingUniversityofTechnology,Beijing㊀100124,China;2.DalianKetianMaterialsCompanyLtd,Dalian㊀116023,China;3.WanfengAutoHoldingGroup,Xinchang㊀312500,China;4.WeihaiWanfengMagnesiumS&TDevelopmentcompanyLtd,Weihai㊀264209,China;5.BeijingInstituteofAeronauticalMaterials,Beijing㊀100095,China)Abstract:TheevolutionofprecipitatedphasesinX2A66aluminum⁃lithiumalloyduringunipolaragingwasstudiedbytrans⁃missonelectronmicroscope(TEM)andquantitativestatisticswereperformed,andstrengtheningmechanismofprecipitatedphaseswasdiscussed.Theresultsindicatethat:theprecipitationsequenceoftheprecipitatedphaseofX2A66aluminum⁃lithiumalloydur⁃ingtheagingprocessis:GPzone+δ'+GPT1ңδ'+θ'+GPT1ңθ'+T1+δ'.PeakagingofX2A66aluminum⁃lithiumalloyis165ħ/72hwiththetensilestrengthis591MPaandtheelongationis6.88%.T1phaseisthemainstrengtheningphaseinX2A66aluminum⁃lithiumalloyduringtheagingprocessandinteractionbetweendislocationandT1phaseisamixedtypeofcutandbypass.Underpeakagingconditions,thestrengthincrementofT1phaseis124.8MPa,andtheratioofthestrengtheningcontributionofT1phasetotheprecipitationphasestrengtheningisabout74.17%withthevolumefractionofT1phaseis17%.Keywords:X2A66Al⁃Lialloy;aging;precipitatedphases;strengtheningmechanism0㊀引言铝锂合金属于可热处理强化型铝合金,与传统2系和7系铝合金相比,具有低密度㊁弹性模量高㊁比强度和比刚度高㊁良好的抗损伤性等特点,被认为是21世纪航空航天工业最理想的轻质高强结构材料之一,在航空航天领域得到了广泛应用[1-2]㊂锂是自然界中密度最小的金属元素,每添加1%的锂在铝基体中,铝合金的弹性模量会提高6%,比弹性模量会增加9%,质量会减少3%㊂铝锂合金的生产工艺同普通铝合金没有更高的制备技术要求,并且与炭纤维增强等复合材料相比,它的制备成形㊁维修等都比较方便,其成本也816 ①收稿日期:2019⁃11⁃21;修回日期:2020⁃01⁃12㊂基金项目:国家自然科学基金(E041501)㊂作者简介:史国栋(1985 ),男,博士,研究方向为高性能铝合金及其复合材料㊂E⁃mail:sgd0222@163.com远远低于复合材料,被认为是21世纪最有竞争力的航空航天材料之一[3-5]㊂我国自主研发的X2A66铝锂合金是脱溶强化型铝合金,具有塑性好㊁强度高,良好的加工性能等优点,在航空航天领域具有良好的应用前景㊂在Al⁃Li二元合金中加入Cu元素可改变合金的沉淀序列,在合金时效过程中会析出富Cu的二元相θ'(Al2Cu)以及三元相T1(Al2CuLi),这两种析出相与δ'相一起复合沉淀起到复合强化的作用[6]㊂由于峰时效时X2A66锂合金中θ'及δ'体积分数较少,因此合金的强化作用主要来自于T1相的沉淀强化[7]㊂综上可知,研究X2A66铝锂时效过程中T1相的析出规律及强化机制是调控X2A66铝锂合金综合性能的关键㊂本文研究了X2A66铝锂合金单极时效过程中析出相的演变并进行了析出相的量化统计,讨论了析出相的强化机制,深入探讨该合金的析出相体积分数与性能之间的关系,为该合金在航空航天领域中的应用提供理论依据及工艺制定指导㊂1㊀实验方法实验所用的X2A66铝锂合金材料为航材院提供,其成分如表1所示㊂表1㊀X2A66合金成分Table1㊀X2A66Alloycomposition%㊀LiCuMgZrZnMnTiFeSiAl1.3 1.83.5 4.10.2 0.60.08 0.160.2 0.80.2 0.6<0.1<0.1<0.1Bal.㊀㊀对X2A66铝锂合金铸锭进行400ħ/12h+500ħ/36h双级均匀化处理和铸锭扒皮,然后进行热挤压,挤压温度为420ħ,挤压比为30,从而得到厚度为2mm的T型挤压板材㊂选取520ħ/80min为X2A66铝锂合金的固溶热处理条件,随后对材料进行单极时效㊂通过TEM观察分析材料的微观组织㊂TEM试样通过双喷电解法制备,电解液为25%HNO3+75%CH3OH混合溶液(体积比)㊂每个时效条件随机选取20个视场进行析出相体积分数统计㊂2㊀实验结果及讨论2.1㊀X2A66铝锂合金最佳时效制度图1为挤压态X2A66铝锂合金单级时效时硬度随时效时间的变化曲线,时效温度分别为145㊁165㊁185ħ㊂图1㊀挤压态X2A66铝锂合金不同单极时效条件硬度曲线Fig.1㊀HardnesscurvesofextrudedX2A66Al⁃Lialloyindifferentagingcondition由图1可知,在低温时效时初期(145ħ),随着时效时间增加,硬度增加缓慢,硬度在96h时达到峰值,硬度为155.3HV㊂当时效温度为165ħ时,硬度在时效72h后达到峰值,硬度为185.7HV㊂时效温度提高到185ħ时,在时效时间为16h时便达到峰值,硬度为174.2HV,随着时效时间增加,合金迅速进入过时效阶段㊂在单级时效制度下,合金在165ħ时效72h时可以获得最大的硬度值185.7HV㊂挤压态X2A66铝锂合金不同时效状态下的室温拉伸性能如表2所示㊂表2㊀X2A66铝锂合金挤压板材不同时效制度下的力学性能Table2㊀MechanicalpropertiesofX2A66Al⁃LialloyYieldstrengthRp0.2/MPaTensilestrengthRm/MPaElongationA/%As⁃extruded378.2492.411.2145ħ/96h405.2548.612.5165ħ/72h548.1591.86.8185ħ/16h521.7571.37.32.2㊀X2A66铝锂合金时效过程中析出相演变图2(a)为X2A66铝合金165ħ,2h时效下的TEM及HRTEM照片,图2(a)中插图为区域斑点衍射,透射照片的带轴为B=[001],从图中的区域半点衍射照片可发现沿(200)方向芒线,这种芒线的出现说明在时效初期,基体中有GP区的存在㊂区域斑点9162020年10月史国栋,等:X2A66铝锂合金时效过程中析出相演变及强化机制第5期衍射中出现二分之一(200)超点阵斑点,这种斑点的出现说明δ'相的存在㊂图2(b)为X2A66铝合金165ħ,4h时效下的TEM照片,图2(b)中插图为区域斑点衍射,(200)方向芒线表明析出相为θ',二分之一(200)斑点显示δ'相的存在㊂X2A66铝合金165ħ,4h时效条件下,θ'相尺寸约为20nm左右,垂直排列于基体中㊂图2(c)㊁(d)为X2A66铝合金165ħ,16h及24h时效下的TEM照片,斑点衍射中可发现沿[1⁃11]Al及[⁃111]Al方向的芒线,这个方向的芒线表明析出相为T1相㊂当时效时间为16h时,X2A66铝锂合金基体中的析出相为T1相㊁θ'相及δ'相㊂当时效温度至24h时,基体中出现大量T1相㊂当时效时间增加至40h时,主要析出相为大量的T1相及少量θ'相和δ'相,如图2(e)所示㊂当时效时间增加至72h时,基体内析出相的数量和尺寸均有所增加,尤其是T1相的数量增加的幅度比较大,且整体上呈现均匀弥散分布㊁交错排列的状态,如图2(f)㊂图2㊀X2A66铝合金165ħ时效下的TEM及HRTEM照片Fig.2㊀TEMimagesofX2A66Al⁃Lialloyagingat165ħ图3(a)为带轴B=[011]下X2A66铝合金165ħ,2h时效下HRTEM照片,图中插图为白色曲线选区放大图及傅里叶转化后的斑点衍射,斑点衍射中可以发现沿[11⁃1]Al及[1⁃11]Al方向的芒线,[11⁃1]Al及[1⁃11]Al方向的芒线T1相的典型特征,此析出相为纳米级针状GPT1相㊂图3(b)为B=[011]方向的透射照片,165ħ,4h时,基体中已经出现大量的GPT1相,尺寸约为20nm㊂X2A66铝锂合金时效初期,在位错及晶界处已经析出纳米级针状析出相,这种析出相为GPT1相㊂图3㊀X2A66铝锂合金165ħ时效过程中GPT1相形貌Fig.3㊀TEMimagesofGPT1morphologyinX2A66Al⁃Lialloyagingat165ħ由上面分析可知,X2A66铝锂合金经165ħ人工时效过程中的主要析出相包括:球形的δ'相㊁针状的GPT1相㊁针状的θ'相及板条状的T1相㊂在X2A66铝锂合金165ħ人工时效初期,基体存在GP区及δ'相,同时出现纳米级针状GPT1相㊂当时效时间增加至4h,合金基体中析出大量细小的针状θ'相,θ'相平均长度15nm,由于此时的析出相平均尺寸较小,所以强化效果有限,合金的硬度不高㊂当时效时间为16h时,X2A66铝锂合金基体中的主要析出相为T1相㊁θ'相及δ'相,此时GPT1相已经转变为T1相,长度大于70nm,宽度约为5nm㊂针状的θ'相尺寸也有所增加,长度约为40nm,宽度约为5nm㊂当时效24h后,合金基体中T1数量明显增多㊂当时效时间为40h,合金基体中析出大量T1相,δ'相及θ'相的数量急剧减少,有些区域已经无法直接观察到δ'相,这说明T1相是以消耗δ'相及θ'相的方式进行生长的;同时针状的θ'相尺寸缓慢长大,但是体积分数开始所减少,针状的θ'相与T1相的尺寸均达到了93nm左右㊂时效时间至72h时,X2A66铝锂合金达到峰值时效后,合金的硬度达到最大,此时X2A66铝锂合金中的主要析出相T1相和少量的针状的θ'相及δ'相㊂相比时效时间40h,T1相的体积分数大幅增加㊂此时合金内析出相的体积分数和析出相尺寸匹配最佳,合金硬度达到了峰值状态㊂综合可知,X2A66铝锂合金在165ħ单级人工时效的欠时效至峰值时效过程中析出相的析出序列为:α过饱和固溶体ңGP区+δ'+GPT1ңδ'+θ'+GPT1ңθ'+T1+δ'㊂图4(a)为X2A66铝合金165ħ时效过程中析出相体积分数曲线㊂X2A66铝合金在165ħ时效过程中,T1相体积分数随着时效时间的增加逐渐增多,在72h时体积分数约为17%,δ'相在时效4h后体积分数便逐渐减少㊂θ'相在时效初期体积分数急剧增多,在16h体积分数接近5%,随后逐渐变少㊂图4(b)为X2A66铝合金165ħ时效析出相平均尺寸曲线,结合026 2020年10月固体火箭技术第43卷图4(a)可知,随着时效时间的增加,δ'相的尺寸变化不大但体积分数减小㊂θ'相尺寸随着时效时间的增加在时效时间为24h时达到最大此时析出相平均长度为94nm,平均宽度为11.18nm,随后急剧减小㊂T1相尺寸在时效过程中尺寸一直增加,在达到峰值时效时,T1相平均长度为118.94nm,平均宽度为7.66nm㊂这也佐证了T1相是以消耗δ'相及θ'相的方式进行生长㊂时效中期,T1相较大的体积自由能变化和[111]剪切应变可促使T1相在基体位错处优先于针状的θ'相形核,在时效中后期,T相消耗δ'相和θ'相生长㊂(a)Volumefraction(b)Phasesize图4㊀X2A66铝合金165ħ时效变化曲线Fig.4㊀VolumefractionofphasesandphasesizeinX2A66alloyagingat165ħ2.3㊀X2A66铝锂合金中T1相的强化机制图5为X2A66铝合金165ħ时效力学性能曲线,对比图4(a)可发现,随着析出相体积分数的增加,合金的强度逐渐增加,但是当T1相体积分数大幅增加而θ'相及δ'相体积分数下降时,材料的强度没有下降反而迅速增高,从图中可见,欠时效时由于T1相析出量的急剧增加,合金抗拉强度增加较快,当接近峰值时效时,T1相强化作用增加平缓㊂从上述分析可知,在时效过程中,T1相为X2A66铝锂合金主要强化相,控制T1相的析出行为,可有效控制合金的综合力学性能㊂从图5可看出,当时效时间为24h时,材料抗拉强度为580MPa,延伸率为7%,此时,材料综合力学较好㊂当时效时间延长至72h时,材料抗拉强度最高,为591MPa,此时材料延伸率为6.88%㊂Fig.5㊀MechanicalpropertycurvesofX2A66alloyagingat165ħ对于铝合金来说,析出相的形态以及数量一定程度上决定了强化效应的大小[8-12]㊂图6(a)中X2A66铝锂合金165ħ,48h时效后常温拉断后变形区的TEM照片,从图中可看出T1相㊁δ'相以及θ'相与位错之间的相互作用㊂A处,位错以切过的方式通过δ'相,由于δ'相为超点阵结构,因此切过的位错成对出现㊂B㊁C处为T1相及θ'相与位错交互作用的情况,可观察到T1相及θ'相对位错运动的钉扎作用㊂图6(b)中的箭头所示为T1相与位错交互作用的情况,从图中可发现在T1相聚集的区域,位错存在两种形态,一种为较长的平直位错,一种较短的弯曲位错㊂因此可以说明位错切过T1相,但又不是单一切过,所以此时位错具有较大的弯曲角,同时位错线张力较大㊂图6(c)为X2A66铝锂合金165ħ,72h时效后常温拉断后变形区的HRTEM照片,插图为析出相区域斑点衍射照片及标定,通过分析可知析出相为T1相,图中T1相与铝基体界面处呈阶梯状,这是由于T1相被位错剪切造成的㊂当T1相的厚度只有几个纳米时,位错可以切过T1相位错且只能在特定的滑移面上切过沉淀相一次㊂随着T1相厚度尺寸增加,T1相由切过机制向绕过机制过渡㊂由于T1相宽面与基体高度共格,其厚度常被作为控制剪切到绕过过度的重要参数[13]㊂从上面的分析可知在时效过程中,T1相为X2A66铝锂合金主要强化相,而位错与T1相间的交互作用为切过和绕过的混合型,为求出T1相的强度增量,需要分别求出切过及绕过的T1相强度增量㊂定义位错切割机制转化为位错绕过机制时,析出相的临界半径为rc,则rc可以通过式(1)计算[13]:1262020年10月史国栋,等:X2A66铝锂合金时效过程中析出相演变及强化机制第5期2γrc+4Gεbrc+0.05ΔGb2(rc/b)0.85=Gb2(1)式中㊀ε为析出相与基体的错配度,其值为(2/3)(Δa/a);γ为T1相刃边界面能,γ=2.71J/m2;ΔG为基体与析出相的剪切模量差;G为[111]面铝基体的剪切模量;b为柏式矢量,b=0.284nm㊂图6㊀X2A66铝合金165ħ时效过程中析出相与位错的交互作用Fig.6㊀InteractionbetweenprecipitationphaseanddislocationinX2A66Al⁃Lialloyagingat165ħ㊀㊀通过式(4) 式(9)可算出T1相位错切割机制转化为位错绕过机制的临界半径rc=4.875nm㊂图7为165ħ时效72h后T1相的晶粒尺寸分布图,从图中数据可以统计出,在165ħ时效72h时,位错切过T1相的体积分数为1.73%,而位错绕过T1相的体积分数为14.57%㊂图1Fig.7㊀RelativefrequencyofT1phasesinX2A66Al⁃Lialloyagingat165ħ/72h在铝锂合金时效过程中,若析出相较软并且恰好位于位错线的滑移面上时,位错运动过程中受到析出相粒子阻碍时可强行切过析出相后继续向前运动㊂位错线切过析出相过程中,由于析出相被切割成两部分,从而使析出相表面能增加㊂有关切过机制的理论模型中,由于T1相刃边的半共格性以及较大的长厚比,因此位错切过刃边造成界面能的增加是位错运动能量的重要消耗源[14],同时需要考虑的参量较多,因此本文有关切过机制的计算中只考虑化学强化作用㊂根据Arclell脱溶相强化理论[13],化学强化作用的表达式为Δσcutting=[4γ3bL2sΓ]12(2)式中㊀Г为位错张力;Ls为1ns;ns为滑移面单位面积上所切的T1相数目㊂ns=ψ4dΔ(3)Г=2.026Gb24πlnRr(4)式中㊀r0和R分别为螺型位错的内外半径,r0=2b,R=dΔ㊂取[111]面铝基体的剪切模量G=30GN/m2,柏式矢量b=0.284nm,结合式子可算得析出相切过机制的强化增量㊂铝合金中析出的针状相位错绕过机制强化公式为[15]Δσbypassing=0.15MGbd(ψ(12)+1.84ψ+1.84ψ32)ln(2.632d2r0)(5)式中㊀r0=2b;M为Taylor常数;d为析出相长径;ψ为析出相体积分数㊂通过式(2)㊁式(5)计算可得T1相的强度增量:㊀㊀ΔσT1=ΔσT1cutting+ΔσT1bypassing=11.8MPa+113MPa=124.8MPa㊀㊀同时根据式(4)可求出δ'相以及θ'相的强度增量:Δσδ'=22.85MPa,Δσθ'=20.6MPa㊂X2A66铝锂合金在165ħ时效72h后基体中析出相的强度总增量Δσphase=ΔσT1+Δσδ'+Δσθ'=168.25MPa㊂经计算可知,T1相的强化贡献占所有析出相强化的比值约为74.17%㊂3㊀结论(1)X2A66铝锂合金在165ħ单级人工时效的欠226 2020年10月固体火箭技术第43卷时效至峰值时效过程中析出相的析出序列:α过饱和固溶体ңGP区+δ'+GPT1ңδ'+θ'+GPT1ңθ'+T1+δ'㊂X2A66铝锂合金时效初期,在位错及晶界处已经析出纳米级针状析出相,这种析出相为GPT1相㊂(2)X2A66铝合金在165ħ时效过程中,T1相体积分数随着时效时间的增加逐渐增多,在72h时体积分数约为17%,δ'相在时效4h后体积分数便逐渐减少㊂θ'相在时效初期体积分数急剧增多,在16h体积分数接近5%,随后逐渐变少㊂随着时效时间的增加,δ'相的尺寸变化不大但体积分数减小㊂θ'相尺寸在时效时间为24h时达到最大,此时析出相平均长度为94nm,平均宽度为11.18nm,随后急剧减小㊂T1相尺寸在时效过程中尺寸一直增加,在达到峰值时效时,T1相平均长度为118.94nm,平均宽度为7.66nm㊂(3)X2A66时效温度为165ħ,时效时间为72h时达到峰值时效,此时抗拉强度为591MPa,延伸率为6.88%㊂T1相为X2A66铝锂合金时效过程中的主要强化相,位错与T1相间的交互作用为切过和绕过的混合型㊂T1相的强度增量为124.8MPa,T1相的强化贡献占析出相强化的比值约为74.17%㊂参考文献:[1]㊀高文理,闫豪,冯朝辉.时效制度对2A97铝锂合金组织和力学性能的影响[J].中国有色金属学报,2014(5):1206⁃1211.GAOWL,YANH,FENGZH.Effectofagingtreatmentonmicrostructureandmechanicalpropertiesof2A97Al⁃Lialloy[J].TheChineseJournalofNonferrousMetals,2014(5):1206⁃1211.[2]㊀Araullo⁃PetersV,GaultB,GeuserFD.Microstructuralevolu⁃tionduringageingofAl⁃Cu⁃Li⁃xalloys[J].ActaMaterialia,2014,66:199⁃208.[3]㊀郑子樵,李劲风,陈志国.铝锂合金的合金化与微观组织演化[J].中国有色金属学报,2011,21(10).ZHENGZQ,LIJF,CHENZG.AlloyingandmicrostructuralevolutionofAl⁃Lialloy[J].TheChineseJournalofNonfer⁃rousMetals,2011,21(10).[4]㊀RiojaRJ.FabricationmethodstomanufactureisotropicAl⁃Lialloysandproductsforspaceandaerospaceapplications[J].MaterialsScience&EngineeringA(StructuralMaterials:Properties,MicrostructureandProcessing),1998,257(1):100⁃107.[5]㊀王东林,李世晨,王小宁.铝锂合金新型立方相的析出规律及机理[J].中国有色金属学报,2009(12):59⁃67.WANGDL,LISC,WANGXN.PrecipitationofnewcubicphaseinAl⁃Lialloysanditsmechanism[J].TheChineseJournalofNonferrousMetals,2009(12):59⁃67.[6]㊀翟彩华,冯朝辉,柴丽华,等.铝锂合金的发展及一种新型铝锂合金⁃X2A66[J].材料科学与工程学报,2015(2):302⁃306.ZHAICH,FENGZH,CHAILH.DevelopmentofAl⁃LiAl⁃loyandanewtypeofAl⁃LialloyX2A66[J].JournalofMa⁃terialsScienceandEngineering,2015(2):302⁃306.[7]㊀乔勇.X2A66铝锂合金固态相变及微观组织演变规律的研究[D].北京:北京工业大学,2016.QIAOY.Studyonsolidphasetransformationandmicrostruc⁃tureevolutionofX2A66Al⁃Lialloy[D].Beijing:Beijinguni⁃versityoftechnology,2016.[8]㊀BaumannSF,WilliamsDB.Anewmethodforthedetermi⁃nationoftheprecipitate⁃matrixinterfacialenergy[J].ScriptaMetallurgica,1984(6):611⁃616.[9]㊀NieJF,MuddleBC,PolmearIJ.Theeffectofprecipitateshapeandorientationondispersionstrengtheninginhighstrengthaluminiumalloys[J].MaterialsScienceForum,1996,217⁃222:1257⁃1262.[10]㊀周昌荣,潘青林.形变量和时效对Al⁃Cu⁃Li合金组织性能的影响[J].材料科学与工艺,2008(4):146⁃149.ZHOUCR,PANQL.EffectsofdeformationandagingonmicrostructureandtensilepropertyofAl⁃Cu⁃Lialloycontai⁃ningSc[J].MaterialsScienceandTechnology,2008(4):146⁃149.[11]㊀杨胜利,沈健,蒋鹏.热处理制度对新型Al⁃Cu⁃Li合金组织与性能的影响[J].中国有色金属学报,2018(6):1111⁃1119.YANGSL,SHENJ,JIANGP.Effectoftreatmentonmicro⁃structureandpropertiesofnewAl⁃Cu⁃Lialloy[J].TheChineseJournalofNonferrousMetals,2018(6):1111⁃1119.[12]㊀胡芳,郑子樵,钟继发.一种新型Al⁃Cu⁃Li合金性能[J].稀有金属材料与工程,2017(7):245⁃249.HUF,ZHENGZQ,ZHONGJF.PropertiesofnewAL⁃Cu⁃Lialloy[J].RareMetalMaterialsandEngineering,2017(7):245⁃249.[13]㊀ArdellAJ.Precipitationhardening[J].MetallurgicalTrans⁃actionsA,16(12):2131⁃2165.[14]㊀李晓玲,徐磊.2090铝锂合金T1相的强化作用及微量Ce的影响[J].中国有色金属学报,1999(3):98⁃103.LIXL,XUL.StrengtheningeffectofT1precipitatesandin⁃fluenceofminorceriumin2090Al⁃Lialloys[J].TheChi⁃neseJournalofNonferrousMetals,1999(3):98⁃103.[15]㊀LIUG,ZHANGGJ,DINGXD.Modelingthestrengtheningresponsetoagingprocessofheat⁃treatablealuminumalloyscontainingplate/disc⁃orrod/needle⁃shapedprecipitates[J].MaterialsScience&EngineeringA,344(12):113⁃124.(编辑:卓飞)3262020年10月史国栋,等:X2A66铝锂合金时效过程中析出相演变及强化机制第5期。
铝锂合金厚板中析出相分布及其点蚀行为的厚向不均匀性
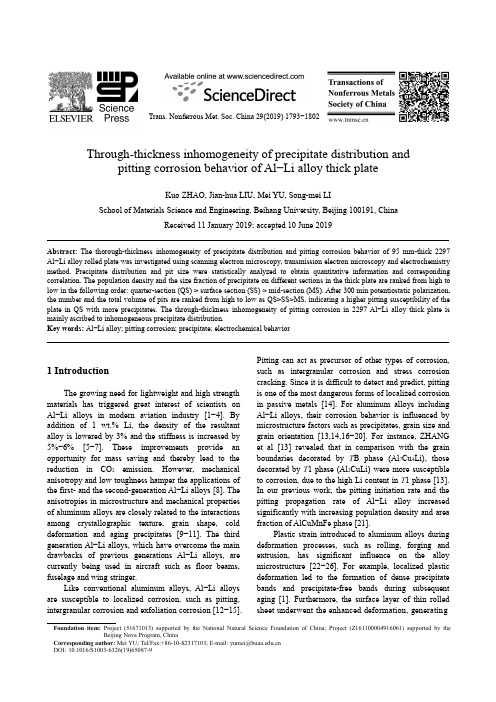
Trans.Nonferrous Met.Soc.China29(2019)1793−1802Through-thickness inhomogeneity of precipitate distribution andpitting corrosion behavior of Al−Li alloy thick plateKuo ZHAO,Jian-hua LIU,Mei YU,Song-mei LISchool of Materials Science and Engineering,Beihang University,Beijing100191,ChinaReceived11January2019;accepted10June2019Abstract:The thorough-thickness inhomogeneity of precipitate distribution and pitting corrosion behavior of95mm-thick2297 Al−Li alloy rolled plate was investigated using scanning electron microscopy,transmission electron microscopy and electrochemistry method.Precipitate distribution and pit size were statistically analyzed to obtain quantitative information and corresponding correlation.The population density and the size fraction of precipitate on different sections in the thick plate are ranked from high to low in the following order:quarter-section(QS)>surface section(SS)>mid-section(MS).After300min potentiostatic polarization, the number and the total volume of pits are ranked from high to low as QS>SS>MS,indicating a higher pitting susceptibility of the plate in QS with more precipitates.The through-thickness inhomogeneity of pitting corrosion in2297Al−Li alloy thick plate is mainly ascribed to inhomogeneous precipitate distribution.Key words:Al−Li alloy;pitting corrosion;precipitate;electrochemical behavior1IntroductionThe growing need for lightweight and high strength materials has triggered great interest of scientists on Al−Li alloys in modern aviation industry[1−4].By addition of1wt.%Li,the density of the resultant alloy is lowered by3%and the stiffness is increased by 5%−6%[5−7].These improvements provide an opportunity for mass saving and thereby lead to the reduction in CO2emission.However,mechanical anisotropy and low toughness hamper the applications of the first-and the second-generation Al−Li alloys[8].The anisotropies in microstructure and mechanical properties of aluminum alloys are closely related to the interactions among crystallographic texture,grain shape,cold deformation and aging precipitates[9−11].The third generation Al−Li alloys,which have overcome the main drawbacks of previous generations Al−Li alloys,are currently being used in aircraft such as floor beams, fuselage and wing stringer.Like conventional aluminum alloys,Al−Li alloys are susceptible to localized corrosion,such as pitting, intergranular corrosion and exfoliation corrosion[12−15].Pitting can act as precursor of other types of corrosion, such as intergranular corrosion and stress corrosion cracking.Since it is difficult to detect and predict,pitting is one of the most dangerous forms of localized corrosion in passive metals[14].For aluminum alloys including Al−Li alloys,their corrosion behavior is influenced by microstructure factors such as precipitates,grain size and grain orientation[13,14,16−20].For instance,ZHANG et al[13]revealed that in comparison with the grain boundaries decorated by T B phase(Al7Cu4Li),those decorated by T1phase(Al2CuLi)were more susceptible to corrosion,due to the high Li content in T1phase[13]. In our previous work,the pitting initiation rate and the pitting propagation rate of Al−Li alloy increased significantly with increasing population density and area fraction of AlCuMnFe phase[21].Plastic strain introduced to aluminum alloys during deformation processes,such as rolling,forging and extrusion,has significant influence on the alloy microstructure[22−26].For example,localized plastic deformation led to the formation of dense precipitate bands and precipitate-free bands during subsequent aging[1].Furthermore,the surface layer of thin rolled sheet underwent the enhanced deformation,generatingFoundation item:Project(51671013)supported by the National Natural Science Foundation of China;Project(Z161100004916061)supported by the Beijing Nova Program,ChinaCorresponding author:Mei YU;Tel/Fax:+86-10-82317103;E-mail:yumei@DOI:10.1016/S1003-6326(19)65087-9Kuo ZHAO,et al/Trans.Nonferrous Met.Soc.China29(2019)1793−1802 1794a surface layer with different microstructures from thebulk[27−29].During subsequent heat treatment,the enhanced deformation on the outmost surface of AA3005 rolled sheet promoted the precipitation of aging precipitates,resulting in an increased number of fine precipitates in comparison with the bulk alloy[27,28]. Whereas,these microstructural changes were not observed for AA5754alloy,due to its lower Mn and Fe contents.Thick rolled plate exhibits strain variation in a wider thickness range than thin rolled sheet does.FENG et al[24]investigated the microstructure of AA7050-T7451thick plate,and pointed out that recrystallization degree differs across thickness direction,resulting in an evident corrosion inhomogeneity.KNIGHT et al[30] revealed that the composition of7079alloy thick plate differs between1/6to1/2thickness positions.However, the through-thickness inhomogeneity in the precipitate distribution of Al−Li alloy thick plate and corresponding corrosion behavior have barely been reported.The aim of this study is to investigate the through-thickness inhomogeneities of precipitate distribution and pitting corrosion behavior of Al−Li alloy thick plate.The size and the number of precipitates on different sections of the thick plate were statistically analyzed.The pitting characteristics were studied by electrochemical tests and three-dimensional(3D)measurements.2ExperimentalThe as-received2297Al−Li alloy rolled plate with a thickness of95mm in T87condition was used in this work.The composition(in mass fraction)of the plate is: 1.53%Li,2.97%Cu,0.29%Mn,0.03%Mg,0.11%Zr, 0.028%Fe,0.024%Si,0.012%Ti and remainder of Al.Directions of the rolled plate are labeled as longitudinal direction(L),long transverse direction(T) and short transverse direction(S).The8mm-diameter cylinders were cut from surface-section(SS),quarter-section(QS)and mid-section(MS)of the thick plate,as shown in Fig.1,and named as SS sample,QS sample and MS sample,respectively.To minimize corrosion, samples were manually ground on stationary SiC grinding paper of5000grit in ethyl alcohol.Abraded samples were then ultrasonically cleaned in ethyl alcohol.The microstructure of the thick plate was studied by scanning electron microscope(SEM;Apollo300, Britain)and transmission electron microscope(TEM; JEOL2100,Japan).Transparent foils for TEM observation were obtained via twin-jet electro-polishing at20V using nitric acid and methanol solution(volume ratio3:7)at about−30°C.Chemical compositions of constituent particles were assessed by energy dispersive X-ray spectroscope(EDS;INCA PentaFETx3,Britain).Fig.1Schematic diagram of sampling positionThe test solution used in electrochemical tests and pitting corrosion measurements was borate-buffered solution at pH7.4,which contains0.18mol/L H3BO3, 0.005mol/L Na2B4O7and0.001mol/L NaCl.The corrosion product was ultrasonically cleaned in a solution containing50vol.%H3PO4+20g/L CrO3and in deionized water.The electrochemical tests were carried out on a Parstat VMC−4electrochemical workstation using a three-electrode configuration.A platinum sheet was used as the counter electrode,and a saturated calomel electrode(SCE)with Luggin capillary served as the reference electrode.All the potentials in this work referred to the SCE.The open circuit potential(OCP)of the thick plate was recorded for30min.Electrochemical impedance spectroscopy(EIS)tests were performed at the OCP in the frequency range from100kHz to10mHz using a10mV AC stimulus signal.In order to reach a steady state,the EIS tests were carried out after3h immersion.Potentiodynamic polarization measurements were commenced at−200mV relative to the OCP and scanned upwards at a scan rate of1mV/s until the anodic current density reached0.1mA/cm2.To estimate the pitting corrosion behavior of2297 Al−Li alloy thick plate,samples were potentiostatically polarized at−150mV(vs SCE)for300min.The working surface was analyzed under3D video microscope(RH−2000,Japan).The number,volume and mouth area of pits on different sections in the thick plate were statistically analyzed.All tests and measurements were performed at least triplicate for data reproducibility. 3Results3.1Microstructure of plateThe SEM images of the alloy are illustrated in Fig.2.The EDS results shown in Fig.2(d)indicate that the precipitates are rich in Al,Cu,Mn and Fe.Hence, these precipitates are hence identified as AlCuMnFe phase.For each section,30low-magnification(500×) SEM images and15high-magnification(5000×)SEMKuo ZHAO,et al/Trans.Nonferrous Met.Soc.China 29(2019)1793−18021795images were used to statistically analyze the size and the density of AlCuMnFe phase using ImageJ software.The size distribution histograms of AlCuMnFe phase in the thick plate are shown in Fig.3,and corresponding statistical data are listed in Table 1.For AlCuMnFe phase,the population density and the area fraction are ranked from high to low in the following order:QS>SS>MS,exhibiting through-thickness inhomogeneity.The TEM images of the thick plate viewed in <110>zone axis are shown in Fig.4,revealing the distribution of needle-like T 1precipitates with 10−295nm in length.Due to the needle-like morphology,the length of T 1phase was statistically analyzed using ImageJ software.To eliminate or decrease variation of the statistical analysis caused by TEM foil thickness,15TEM images were used to perform the statistical analysis for each section.The length distribution histograms of T 1phase on the three sections in the thick plate are presented in Fig.5,and the corresponding statistical data are presented in Table 2.For the three sections,the population density of T 1phase is ranked from high to low in the following order:QS>SS>MS.Similar to the area fraction of AlCuMnFe phase,the size fraction of T 1phase is defined by the length of T 1phase per unit area.The size fraction of T 1phase from high to low is in the following sequence:QS>SS>MS.The through-thickness variation in precipitate distribution is mainly attributed to the inhomogeneous strain and cooling rate [24,31,32].The equivalent strain and cooling rate decrease from surface to center of thick rolled plate [31,32].Dislocation caused by strain usually acts as nucleation site,promoting precipitation of aging precipitates.Hence,the population density of precipitate on MS is smaller than that on SS.On the other hand,cooling rate can also affect the precipitate distribution.The increase in cooling rate shortens isothermal duration,resulting in the reduction of precipitate propagation duration [32−34].The amount of fine precipitates hence decreases with increasing cooling rate.Thus,SS of the thick plate contains higher ratio of small precipitates (AlCuMnFe phase <1.5μm,T 1phase <60nm)in comparison with QS andMS.Fig.2SEM images of 2297Al−Li alloy thick plate at three positions:(a)SS;(b)QS;(c)MS;(d)EDSresultsFig.3Size distribution histograms of AlCuMnFe phase in 2297Al−Li alloy thick plate at three positions:(a)SS;(b)QS;(c)MSKuo ZHAO,et al/Trans.Nonferrous Met.Soc.China 29(2019)1793−18021796Table 1Statistical data of AlCuMnFe phase in 2297Al−Li alloy thick plate Sample Population density/mm −2Area fraction/%SS 1418.150.22QS 1678.170.38MS883.630.15Table 2Statistical data of T 1phase in 2297Al−Li alloy thick plate Sample Population density/μm −2Size fraction/μm −1SS 177.7711.98QS 213.5518.43MS133.6310.27Fig.4TEM images of 2297Al−Li alloy thick plate at three positions:(a)SS;(b)QS;(c)MSFig.5Distribution histograms of T 1phase in 2297Al−Li alloy thick plate at three positions:(a)SS;(b)QS;(c)MS3.2OCP testsThe OCP curves of the thick plate are shown in Fig.6.For the three sections of the thick plate,the OCP increases first,and then reaches a relative steady state.For the three sections,the OCP is ranked from high to low as MS>SS>QS.This signifies that SS sample is more active than MS sample,but less active than QS sample.3.3EIS measurementNyquist plots of 2297Al−Li alloy thick plate are presented in Fig.7.All the Nyquist plots exhibit similarKuo ZHAO,et al/Trans.Nonferrous Met.Soc.China 29(2019)1793−18021797depressed features.The Nyquist plots are characterized by two capacitive arcs and one inductive arc.In metal corrosion,the inductive arc is often attributed to the weakening process of oxide film and corrosion [35−37].Thus,the presence of inductive arc indicates that all the samples undergo corrosion during 3himmersion.Fig.6OCP curves of 2297Al−Li alloy thick plate at threepositionsFig.7Nyquist plots of 2297Al−Li alloy thick plate at three positionsThe equivalent electric circuit used to fit the EIS spectra is shown in Fig.8,where R s ,R f and R ct are the solution resistance,the resistance of passive film and the charge transfer resistance,respectively;Q f and Q dl are the constant phase elements associated with the origin surface capacitance and interfacial capacitance of the new interface originated from corrosion,respectively;R L and L are pseudo resistance and inductance,respectively.The fitted values of R f ,R ct and R L are shown in Fig.9.It is noticeable that R ct is significantly larger than R f and R L ,suggesting that the charge transfer process is the slowest step,i.e.the controlling step.For the three sections of the thick plate,the order of active dissolution resistance of the thick plate ranked by R ct from high to low is MS>SS>QS.Thus,the EIS tests indicate that the corrosion resistance of the thick plate descends in the order:MS>SS>QS.Fig.8Equivalent electric circuit used to fit EIS testsdataFig.9Fitted values of R f ,R ct and R L3.4Potentiodynamic polarization curvesThe potentiodynamic polarization curves of 2297Al−Li alloy thick plate at three positions are shown in Fig.10.The current plateau in polarization curves reveals the presence of protective air-formed oxide film.After the current plateau,the current density increases significantly with increasing potential,exhibiting an obvious pitting potential (φpit ).The noticeable current rise is generally related to the breakdown of the oxide film.The φcorr and φpit derived from Fig.10are listed in Table 3.The φcorr and φpit of the thick plate are ranked in the following order:MS>SS>QS,indicating that in comparison with SS sample and MS sample,QS sample is more active and has higher pitting susceptibility.QSFig.10Potentiodynamic polarization curves of 2297Al−Li alloy thick plate at three positionsKuo ZHAO,et al/Trans.Nonferrous Met.Soc.China 29(2019)1793−18021798sample exhibits a smaller difference between φpit and φcorr than SS and MS samples,suggesting a lower stability of oxide film.Potentiodynamic polarization tests reveal that the rank of the pitting susceptibility of the thick plate from high to low is QS>SS>MS.Table 3φcorr and φpit of 2297Al−Li alloy thick plate derived from potentiodynamic polarization curves Sample φcorr (vs SCE)/mV φpit (vs SCE)/mV SS −865.01±12.71−45.87±6.66QS −881.82±11.34−105.09±3.59MS−858.13±17.06−32.46±9.823.5Pitting corrosion testsCurrent densities of different samples recorded during potentiostatic polarization at −150mV are shown in Fig.11.Initially,the current density curves of the three samples show a current plateau,suggesting that air-formed oxide film can provide certain protection.After the current density plateau,noticeable current density rise is detected for QS and SS samples,while MS sample exhibits a relatively slow current rise.The current density rise indicates that stable pits initiate during long-term potentiostatic polarization at potential below the φpit .In the medium containing aggressive anions such as chloride,the oxide film on aluminum alloys becomes unstable and degrades locally,resulting in oxide film breakdown.After onset of pitting,the current densities of the three samples are ranked from high to low as QS>SS>MS,implying the order of the dissolution rate of the thick plate from high to low isQS>SS>MS.Fig.11Current densities of different samples recorded during potentiostatic polarization at −150mV3D morphologies of pits are presented in Fig.12.It can be clearly seen that all the samples exhibit pitting corrosion.Though the electrolyte in the pit cavity is highly corrosive ascribed to local acidification [38],intergranular corrosion is not detected.The influence of ultrasonic cleaning on 3D measurements of pit cavitiesis therefore believed to be negligible.The volume distribution histograms of pits are shown in Fig.13,and detail statistical data are listed in Table 4.QS sample exhibits a larger pit volume distribution in comparison with SS and MS samples.The order of pit number from high to low is QS>SS>MS,which is consistent with the order of precipitate population density,indicating that pit initiates more easily in the plate with more precipitates.The total pit volume and the total pit mouth area are from high to low in the following sequence:QS>SS>MS,consistent with the order of size fraction of precipitates.Thus,the statistical analysis demonstrates that the pitting corrosion resistance of the thick plate descends in the following order:MS>SS>QS.4DiscussionThe present results demonstrate that there is pronounced through-thickness inhomogeneity in precipitate distribution and pitting corrosion of 2297Al−Li alloy thick plate.Localized corrosion associated with precipitates has been widely reported [17,39−41].The electrochemical inhomogeneity in the alloy matrix resulted from precipitates has significant influence on the localized corrosion behavior of aluminum alloys [16,17].Electrochemical tests reflect the overall electro-chemical response of the entire electrode.OCP and φcorr are influenced by the kinetics of corrosion reactions.Therefore,precipitate distribution has noticeable impact on OCP and φcorr .T 1phase is anodic active in comparison with the alloy matrix [1,42].With increasing population density of T 1phase,2297Al−Li alloy tends to be more active,exhibiting more negative OCP and φcorr .AlCuMnFe phase,cathodic phase with respect to the matrix,induces active dissolution of surrounding matrix [21,43].The presence of AlCuMnFe phase hence results in a more negative electrode potential.As shown in Tables 1and 2,the population density of precipitates on the three sections of the plate from high to low is ranked in the following order:QS>SS>MS.Therefore,the OCP and φcorr descend in the order:MS>SS>QS.Besides,as shown in Fig.10,the current density at the same applied over-potential rises with increasing population density and area fraction of precipitates,confirming that the electrode containing more precipitates is more active.Precipitate distribution also shows noticeable effects on φpit .According to Ref.[4],precipitates with minimum dimensions above a critical size can result in a defeat of the oxide film,as the oxide film cannot cover the entire metal surface.Consequently,pits initiate more easily at the defect.The critical size was reported to be around 2.5nm for 6xxx Al alloys [20]and 3−8nm for 2xxx Al alloys [4].In this study,the thickness of T 1phase isKuo ZHAO,et al/Trans.Nonferrous Met.Soc.China 29(2019)1793−18021799Fig.12Morphologies of stable pits on SS (a,b),QS (c,d)and MS (e,f)samplesFig.13Volume distribution histograms of pits on SS (a),QS (b)and MS (c)samples Table 4Statistical data of pits generated on thick plate at three positionsSample N pits V t /μm 3S t /mm 2SS 524 4.91×107 3.55QS 117410.85×1079.42MS1861.70×1071.74N pits is the number of pits;V t is the total pit volume;S t is the total mouth areaof pitsgenerally smaller than 2nm,and hence it is less than the critical size required to induce pitting [4,20].Thus,it isdeduced that T 1phase shows negligible effect on φpit .For the three sections of the thick plate,the population density of AlCuMnFe phase is ranked from high to low as follows:QS>SS>MS.With increasing population density of AlCuMnFe phase,the number of active site increases.The alloy with more precipitates is hence more susceptible to pitting corrosion,exhibiting a more negative φpit .Thus,the φpit is the most positive for MS of the plate,intermediate for SS of the plate,and most negative for QS of the plate.The type,the population density and the size of precipitates show significant effects on pitting corrosionKuo ZHAO,et al/Trans.Nonferrous Met.Soc.China29(2019)1793−1802 1800of aluminum alloys.In neutrally aerated solution containing chloride,the dominant cathodic reaction isoxygen reduction,which readily takes place on cathodicprecipitates.As a result,hydroxyl ions,as the reactionproduct,cause localized alkalization near the cathodicprecipitates.For instance,the local pH around Al3Fe in AA6061alloy is measured to be9.5,which is much higher than the bulk pH6.3[44].It is widely acceptedthat aluminum oxide dissolves in solution with pH higherthan9[38,44].Thus,the localized alkalization aroundcathodic precipitates induces breakdown of oxide filmand active dissolution of surrounding matrix,resulting in pit initiation and propagation.T1phase shows negligible effect on pit initiation,while it can promote pitpropagation.The corrosion mechanism associated with T1phase containing active element Li and noble elementCu is similar to the preferential dissolution phenomenonrelated to S phase in AA2024alloy[45].At the beginning, T1phase acts as anodic in micro-galvanic,resulting in their anodic dissolution and corrosion.The activeelement Li is preferentially dissolved,and meanwhile noble element Cu is enriched.Consequently,the Cu-rich remnant becomes cathodic to its periphery,leading to the dissolution of the surrounding matrix.Therefore,with increasing population density and size fraction of precipitate,the active dissolution resistance of the thick plate decreases,exhibiting a lower R ct(Fig.9)and higher polarization current density(Fig.11).The dissolution of alloy matrix around larger precipitates results in larger cavity and severer electrolyte.Due to the autocatalytic nature of pits,the stable pit caused by larger precipitate tends to maintain continuous propagation for a longer duration.For the three sections of the thick plate,the peak current density descends in the following order: QS>SS>MS.Therefore,the larger population density and higher size fraction of precipitates result in more and larger pits on the plate.5Conclusions(1)Two precipitate phases are identified in the2297 Al−Li alloy thick plate:AlCuMnFe phase and T1phase. For the three sections,the two precipitate phases both exhibit inhomogeneous distribution.The population densities and the size fractions of the two precipitate phases are ranked from high to low in the following order:QS>SS>MS.(2)OCP,φcorr,φpit,R ct and R L of the plate are ranked from high to low as QS<SS<MS,indicating the pitting susceptibility of the thick plate descends in the following order:QS>SS>MS.After300min polarization at −150mV,the pit number,the total pit volume and the total pit mouth area of pits are ranked from high to low in the following order:QS>SS>MS,indicating the order of pit propagation rate of the plate from high to low is in the sequence:QS>SS>MS.(3)The pitting susceptibility and propagation rate of the plate closely are related to the precipitate distribution. For the three sampling positions,the plate in MS contains the sparsest precipitates of smallest area/size fractions,exhibiting the best pitting corrosion resistance. The through-thickness inhomogeneity of pitting corrosion in2297Al−Li alloy thick plate is mainly ascribed to inhomogeneous precipitate distribution. References[1]ZHANG Xin-xin,ZHOU Xiao-rong,HASHIMOTO T,LIU Bing,LUO Chen,SUN Zhi-hua,TANG Zhi-hui,LU Feng,MA Yan-long.Corrosion behaviour of2A97-T6Al−Cu−Li alloy:The influence of non-uniform precipitation[J].Corrosion Science,2018,132:1−8. [2]OTT N,YAN Yuan-ming,RAMAMURTHY S,KAIRY S,BIRBILISN.Auger electron spectroscopy analysis of grain boundary microchemistry in an Al−Cu−Li alloy[J].Scripta Materialia,2016, 119:17−20.[3]LIU Dan-yang,WANG Jie-xia,LI Jin-feng.Micostructures evolutionand mechanical properties disparity in2070Al−Li alloy with minor Sc addition[J].Transactions of Nonferrous Metals Society of China, 2018,28:2152−2162.[4]RALSTON K D,BIRBILIS N,CAV ANAUGH M K,WEYLAND M,MUDDLE B C,MARCEAU R K W.Role of nanostructure in pitting of Al−Cu−Mg alloys[J].Electrochimica Acta,2010,55:7834−7842.[5]ABD EL-ATY A,XU Yong,GUO Xun-zhong,ZHANG Shi-hong,MA Yan,CHEN Da-yong.Strengthening mechanisms,deformation behavior,and anisotropic mechanical properties of Al−Li alloys:A review[J].Journal of Advanced Research,2018,10:49−67.[6]BOIS-BROCHU A,BLAIS C,TCHITEMBO GOMA F A,LAROUCHE D.Modelling of anisotropy for Al−Li2099T83 extrusions and effect of precipitate density[J].Materials Science and Engineering A,2016,673:581−586.[7]OTT N,KAIRY S K,YAN Y M,BIRBILIS N.Evolution of grainboundary precipitates in an Al−Cu−Li alloy during aging[J].Metallurgical and Materials Transactions A,2017,48:51−56.[8]MA Y,ZHOU X,HUANG W,LIAO Y,CHEN X,ZHANG X,THOMPSON G E.Crystallographic defects induced localised corrosion in AA2099-T8aluminium alloy[J].Corrosion Engineering, Science and Technology,2014,50:420−424.[9]ES-SAID O S,PARRISH C J,BRADBERRY C A,HASSOUN J Y,PARISH R A,NASH A,SMYTHE N C,TRAN K N,RUPERTO T, LEE E W,MITCHELL D,VINQUIST C.Effect of stretch orientation and rolling orientation on the mechanical properties of2195 Al−Cu−Li alloy[J].Journal of Materials Engineering and Performance,2010,20:1171−1179.[10]RIOJA R J.Fabrication methods to manufacture isotropic Al−Lialloys and products for space and aerospace applications[J].Materials Science and Engineering A,1998,257:100−107.[11]WRONSKI S,BACROIX B.Microstructure evolution and grainrefinement in asymmetrically rolled aluminium[J].Acta Materialia, 2014,76:404−412.[12]GHOSH R,VENUGOPAL A,NARAYANAN P R,SHARMA S C,VENKITAKRISHNAN P V.Environmentally assisted cracking resistance of Al−Cu−Li alloy AA2195using slow strain rate test in3.5%NaCl solution[J].Transactions of Nonferrous Metals Societyof China,2017,27:241−249.[13]ZHANG X,ZHOU X,HASHIMOTO T,LINDSAY J,CIUCA O,LUO C,SUN Z,ZHANG X,TANG Z.The influence of grainKuo ZHAO,et al/Trans.Nonferrous Met.Soc.China29(2019)1793−18021801structure on the corrosion behaviour of2A97-T3Al−Cu−Li alloy[J].Corrosion Science,2017,116:14−21.[14]DONATUS U,TERADA M,OSPINA C R,QUEIROZ F M,FATIMA SANTOS BUGARIN A,COSTA I.On the AA2198-T851alloy microstructure and its correlation with localized corrosion behaviour[J].Corrosion Science,2018,131:300−309.[15]LI Jin-feng,HUANG Jia-lei,LIU Dan-yang,CHEN Yong-lai,ZHANG Xu-hu,MA Peng-cheng.Distribution and evolution ofaging precipitates in Al−Cu−Li alloy with high Li concentration[J].Transactions of Nonferrous Metals Society of China,2019,29: 15−24.[16]TIAN Wen-ming,LI Song-mei,CHEN Xin,LIU Jian-hua,YU Mei.Intergranular corrosion of spark plasma sintering assembled bimodal grain sized AA7075aluminum alloys[J].Corrosion Science,2016, 107:211−224.[17]LEBLANC P,FRANKEL G S.A study of corrosion and pittinginitiation of AA2024-T3using atomic force microscopy[J].Journal of the Electrochemical Society,2002,149:B239−B247.[18]RALSTON K D,BIRBILIS N,WEYLAND M,HUTCHINSON C R.The effect of precipitate size on the yield strength-pitting corrosion correlation in Al−Cu−Mg alloys[J].Acta Materialia,2010,58: 5941−5948.[19]ARTHANARI S,JANG J C,SHIN K S.Corrosion performance ofhigh pressure die-cast Al−6Si−3Ni and Al−6Si−3Ni−2Cu alloys in aqueous NaCl solution[J].Transactions of Nonferrous Metals Society of China,2018,28:2181−2189.[20]YAN Y,PEGUET L,GHARBI O,DESCHAMPS A,HUTCHINSONC R,KAIRY S K,BIRBILIS N.On the corrosion,electrochemistryand microstructure of Al−Cu−Li alloy AA2050as a function of ageing[J].Materialia,2018,1:25−36.[21]LIU Jian-hua,ZHAO Kuo,YU Mei,LI Song-mei.Effect of surfaceabrasion on pitting corrosion of Al−Li alloy[J].Corrosion Science, 2018,138:75−84.[22]MA Y,ZHOU X,LIAO Y,YI Y,WU H,WANG Z,HUANG W.Localised corrosion in AA2099-T83aluminium−lithium alloy:The role of grain orientation[J].Corrosion Science,2016,107:41−48. [23]KUMAR R,GUPTA A,KUMAR A,CHOUHAN R N,KHATIRKAR R K.Microstructure and texture development during deformation and recrystallisation in strip cast AA8011aluminum alloy[J].Journal of Alloys and Compounds,2018,742:369−382. [24]FENG Lei,PAN Qing-lin,WEI Li-li,HUANG Zhi-qi,LIU Zhi-ming.Through-thickness inhomogeneity of localized corrosion in 7050-T7451Al alloy thick plate[J].Journal of Central South University,2015,22:2423−2434.[25]MA Y,ZHOU X,THOMPSON G E,HASHIMOTO T,THOMSON P,FOWLES M.Distribution of intermetallics in an AA2099-T8 aluminium alloy extrusion[J].Materials Chemistry and Physics, 2011,126:46−53.[26]YE Xian-jue,GONG Xiao-juan,YANG Biao-biao,LI Yun-ping,NIEYan.Deformation inhomogeneity due to sample-anvil friction in cylindrical compression test[J].Transactions of Nonferrous Metals Society of China,2019,29:279−286.[27]AMBAT R,DAVENPORT A J,AFSETH A,SCAMANS G.Electrochemical behavior of the active surface layer on rolled aluminum alloy sheet[J].Journal of the Electrochemical Society, 2004,151:B53−B58.[28]AFSETH A,NORDLIEN J H,SCAMANS M G,NISANCIOGLU K.Influence of heat treatment and surface conditioning on filiform corrosion of aluminium alloys AA3005and AA5754[J].CorrosionScience,2001,43:2359−2377.[29]ZHOU X,LIU Y,THOMPSON G E,SCAMANS G M,SKELDON P,HUNTER J A.Near-surface deformed layers on rolled aluminum alloys[J].Metallurgical and Materials Transactions A,2010,42: 1373−1385.[30]KNIGHT S P,BIRBILIS N,MUDDLE B C,TRUEMAN A R,LYNCH S P.Correlations between intergranular stress corrosion cracking,grain-boundary microchemistry,and grain-boundary electrochemistry for Al−Zn−Mg−Cu alloys[J].Corrosion Science, 2010,52:4073−4080.[31]ZHANG Tao,WU Yun-xin,GONG Hai,SHI Wen-ze,JIANGFang-min.Analysis of strain variation in cross shear zone of plate during snake hot rolling[J].Journal of Central South University, 2017,24:296−302.[32]ZHANG Yu-xun,YI You-ping,HUANG Shi-quan,DONG Fei.Influence of quenching cooling rate on residual stress and tensile properties of2A14aluminum alloy forgings[J].Materials Science and Engineering A,2016,674:658−665.[33]ELGALLAD E M,ZHANG Z,CHEN X G.Effect of quenching rateon precipitation kinetics in AA2219DC cast alloy[J].Physica B: Condensed Matter,2017,514:70−77.[34]YIN Yuan,LUO Bing-hui,JING Hui-bo,BAI Zhen-hai,GAO Yang.Influences of quench cooling rate on microstructure and corrosion resistance of Al−Cu−Mg alloy based on the end-quenching test[J].Metallurgical and Materials Transactions B,2018,49:2241−2251. [35]LV Jin-long,LIANG Tong-xiang,WANG Chen,GUO Ting.Thepassive film characteristics of several plastic deformation2099Al−Li alloy[J].Journal of Alloys and Compounds,2016,662:143−149. [36]SONG Feng-xuan,ZHANG Xin-ming,LIU Sheng-dan,TAN Qi,LIDong-feng.The effect of quench rate and overageing temper on the corrosion behaviour of AA7050[J].Corrosion Science,2014,78: 276−286.[37]ZHANG G A,XU L Y,CHENG Y F.Mechanistic aspects ofelectrochemical corrosion of aluminum alloy in ethylene glycol–water solution[J].Electrochimica Acta,2008,53:8245−8252. [38]TIAN Wen-ming,LI Song-mei,WANG Bo,LIU Jian-hua,YU Mei.Pitting corrosion of naturally aged AA7075aluminum alloys with bimodal grain size[J].Corrosion Science,2016,113:1−16. [39]LI L L,ZHANG B,TIAN B,ZHOU Y,WANG J Q,HAN E H,KEW.SVET study of galvanic corrosion of AlMg2Si couple in aqueous solutions at different pH[J].Journal of the Electrochemical Society, 2017,164:C240−C249.[40]LUO Chen,ALBU S P,ZHOU Xiao-rong,SUN Zhi-hua,ZHANGXiao-yun,TANG Zhi-hui,THOMPSON G E.Continuous and discontinuous localized corrosion of a2xxx aluminium–copper–lithium alloy in sodium chloride solution[J].Journal of Alloys and Compounds,2016,658:61−70.[41]MA Yan-long,ZHOU Xiao-rong,MENG Xiao-min,HUANGWei-jiu,LIAO Yi,CHEN Xiao-li,YI Ya-nan,ZHANG Xin-xin, THOMPSON G E.Influence of thermomechanical treatments on localized corrosion susceptibility and propagation mechanism of AA2099Al−Li alloy[J].Transactions of Nonferrous Metals Society of China,2016,26:1472−1481.[42]LI J F,LI C X,PENG Z W,CHEN W J,ZHENG Z Q.Corrosionmechanism associated with T1and T2precipitates of Al−Cu−Li alloys in NaCl solution[J].Journal of Alloys and Compounds,2008, 460:688−693.[43]GRILLI R,BAKER M A,CASTLE J E,DUNN B,WATTS J F.Localized corrosion of a2219aluminium alloy exposed to a3.5% NaCl solution[J].Corrosion Science,2010,52:2855−2866. [44]PARK J O,PAIK C H,HUANG Y H,ALKIRE R C.Influence ofFe-rich intermetallic inclusions on pit initiation on aluminum alloys in aerated NaCl[J].Journal of the Electrochemical Society,1999, 146:517−523.[45]BOAG A,TAYLOR R J,MUSTER T H,GOODMAN N,MCCULLOCH D,RYAN C,ROUT B,JAMIESON D,HUGHES AE.Stable pit formation on AA2024-T3in a NaCl environment[J].Corrosion Science,2010,52:90−103.。
析出晶内析出相主要为β相晶界析出...

材料学专业毕业论文 [精品论文] 5A30与5083铝合金型材应力腐蚀行为的对比研究关键词:铝合金材料拉伸开裂应力腐蚀晶界分布摘要:本文采用慢应变速率拉伸(SSRT)法研究了5A30及5083铝合金型材的应力腐蚀开裂(SCC)行为并评定了它们的SCC敏感性。
借助SEM、TEM、OM、EDS、XRD、动电位扫描及阳极电流恒载荷拉伸等手段,研究了预变形量、稳定化处理、应变速率、极化电位对两合金型材SCC行为的影响规律;进而对比分析了两合金型材SCC行为的异同;从两合金型材在不同预变形量条件下的显微组织差异,分析了预变形量对两合金型材SCC敏感性影响规律的主要原因;进一步探讨了5xxx 系铝合金的SCC机理。
主要内容如下:⑴探明了预变形量、稳定化处理、应变速率、极化电位对5A30与5083铝合金型材SCC敏感性的影响规律。
结果表明,预变形量在0~15%范围内,两合金型材的SCC敏感性均随预变形量增加先减小后增大,在预变形量为10%时,SCC敏感性最小;预变形后的稳定化处理显著降低两合金型材的SCC敏感性;应变速率在10-4s-1~10-6s-1范围内,两合金型材的SCC敏感性随应变速率减小而增大;阳极极化显著增加两合金型材的SCC敏感性,阴极极化使其减小。
⑵探明了5A30与5083铝合金型材在不同条件下的断口形貌。
结果表明,两合金型材在空气中的SSRT断口形貌均呈韧窝状,具有明显的韧性断裂特征,而在3.5%NaCl溶液中的SSRT断口形貌呈解理状,具有脆性断裂特征,预变形量在0~15%范围内,断口的脆断特征随预变形量增加先减弱后增强,在预变形量为10%时,脆断特征最小;预变形后的稳定化处理使断口的脆断特征显著减弱;应变速率在10-4s-1~10-6s-1范围内,断口的脆断特征随应变速率减小而增强;阳极极化显著增强断口的脆断特征,阴极极化使其减弱。
⑶对比分析了5A30与5083铝合金型材慢应变速率拉伸SCC行为的异同。
Al-Cu-Mg-Si合金中σ相的析出行为研究

Al-Cu-Mg-Si合金中σ相的析出行为研究摘要以Al-Cu-Mg-Si合金为研究对象,通过光学显微镜、透射电镜和电子探针等手段研究了其中σ相的析出行为,并分析了合金中硅和镁元素对σ相析出的影响。
结果表明,当合金中硅和镁质量分数分别为0.5%和1.0%时,σ相开始析出的转变温度最低,且析出量最大;σ相析出过程中间隔时间越长,析出量越少。
此外还发现了合金旁边析出的α-Al固溶体颗粒会在σ相析出晶种上生长,对σ相的析出形态起到重要的影响。
本研究对于理解Al-Cu-Mg-Si合金中σ相的析出行为和对合金性能的影响具有一定意义。
关键词:Al-Cu-Mg-Si合金;σ相;析出行为;硅;镁AbstractIn this study, the precipitation behavior of σ phasein Al-Cu-Mg-Si alloy was investigated by means of optical microscope, transmission electron microscope and electron probe. The effects of silicon and magnesium elements on the p recipitation of σ phase in the alloy were analyzed. It was found that the transition temperature of the onset of σ phaseprecipitation was the lowest and the precipitation amount was the largest when the mass fractions of silicon and magnesium in the alloy were 0.5% and 1.0%, respectively. The longer the interval time during the σ precipitation process, the less the amount of precipitation. In addition, it was found that the α-Al solid solution particles precipitated beside the alloy grew on the seed crysta ls of σ phase precipitation and had an important influence on the precipitation morphology of σ phase. The results of this study are of certain significance for understanding the precipitation behavior of σ phasein Al-Cu-Mg-Si alloys and its effect on alloy properties.Keywords: Al-Cu-Mg-Si alloy; σ phase; precipitation behavior; silicon; magnesiuFurther analysis showed that the addition of silicon and magnesium in the Al-Cu-Mg-Si alloy had a significant effect on the precipitation behavior of σ phase. T he σ phase precipitation rate increased with the increase in silicon and magnesium content. This can be explained by the fact that silicon and magnesium played a crucial role in the formation of σ phase nuclei.Moreover, the study revealed that the morpho logy of σ phase precipitation was significantly influenced by the precipitation of α-Al solid solution particles. The α-Al solid solution particles acted as "seed crystals" for the growth of σ phase precipitation, resulting in a complex and heterogeneous precipitation morphology. This discovery sheds light on the mechanism of σ phase precipitation in Al-Cu-Mg-Si alloys.In summary, the complex precipitation behavior of σ phase in Al-Cu-Mg-Si alloys was characterized in this study. The results provide valuable insights into the precipitation kinetics and morphology of σ phase and its effect on alloy properties. The findings of this study can contribute to the development of new Al-Cu-Mg-Si alloys with improved propertiesThe complex precipitation behavior of σ phase in Al-Cu-Mg-Si alloys has significant implications for the design and application of these materials. One important consideration is the effect of σ phase precipitation on the mechanical properties of the alloy. While σ phase is generally consider ed to be detrimental to mechanical performance due to its inherent brittleness, recent studies have shown that controlled precipitation of σ phase can actuallyimprove both strength and toughness in some Al-Cu-Mg-Si alloys.Another important consideration is the effect of processing parameters on the formation and morphology of σ phase. The present study has shown that both aging temperature and Cu/Mg ratio can have asignificant impact on the precipitation behavior of σ phase, highlighting the need for careful control of processing conditions to achieve the desiredproperties in these alloys.In addition to improving our understanding of σ phase precipitation in Al-Cu-Mg-Si alloys, the present study also demonstrates the power of advanced microscopy techniques for characterizing complex precipitation behavior at the nanoscale. As materials science continues to push the limits of what is possible with advanced alloys, sophisticated characterization techniques will be increasingly necessary to gain insights into the underlying mechanisms governing material behavior.Overall, the findings of this study have important implications for the design and processing of Al-Cu-Mg-Si alloys, and provide a starting point for further investigation into the complex precipitation behaviorof these materials. By continuing to refine our understanding of the underlying mechanisms governing material behavior, we can develop new alloys with improved properties and better understand how to optimize processing conditions to achieve desired performance characteristicsIn addition to the design and processing implications mentioned above, the findings of this study also have broader implications for the use and application ofAl-Cu-Mg-Si alloys in various industry sectors. For example, the improved understanding of the precipitation behavior of these materials could help to develop new, high-strength alloys with improved properties for use in the aerospace and automotive industries. Lighter, stronger materials are always in demand in these industries as they can lead to improved fuel efficiency and overall performance.Furthermore, the findings of this study could also have implications for the manufacturing and processing of these alloys. By understanding how precipitation occurs and how it affects material properties, it may be possible to optimize processing conditions to minimize the amount of downtime required for aging and other heat treatments. This could lead to significant savings in both time and cost, making Al-Cu-Mg-Sialloys more accessible and practical for use in awider range of applications.Overall, the importance of materials science research and the development of new alloys cannot be overstated. Advancements in this area have the potential to revolutionize industries, improve product performance, and create new opportunities for growth and innovation. The findings of this study represent a significantstep forward in our understanding of the behavior ofAl-Cu-Mg-Si alloys and provide a valuable foundationfor future research and development in this fieldIn conclusion, the study highlights the importance of materials science research and the development of new alloys. The Al-Cu-Mg-Si alloy has been found to have remarkable mechanical properties and can be used in various applications. The study's findings provide a valuable foundation for future research and development in this field, which has the potential to revolutionize industries, improve product performance, and create new opportunities for growth and innovation. Therefore, the significance of materials science research in developing new alloys cannot be overstated。
铝合金偏析
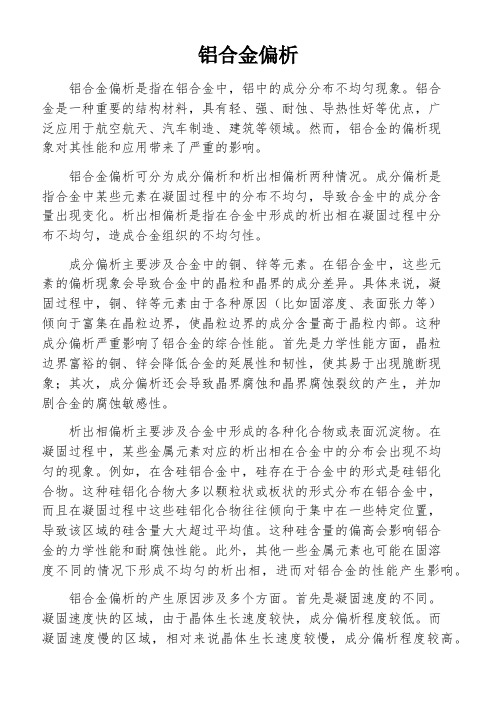
铝合金偏析铝合金偏析是指在铝合金中,铝中的成分分布不均匀现象。
铝合金是一种重要的结构材料,具有轻、强、耐蚀、导热性好等优点,广泛应用于航空航天、汽车制造、建筑等领域。
然而,铝合金的偏析现象对其性能和应用带来了严重的影响。
铝合金偏析可分为成分偏析和析出相偏析两种情况。
成分偏析是指合金中某些元素在凝固过程中的分布不均匀,导致合金中的成分含量出现变化。
析出相偏析是指在合金中形成的析出相在凝固过程中分布不均匀,造成合金组织的不均匀性。
成分偏析主要涉及合金中的铜、锌等元素。
在铝合金中,这些元素的偏析现象会导致合金中的晶粒和晶界的成分差异。
具体来说,凝固过程中,铜、锌等元素由于各种原因(比如固溶度、表面张力等)倾向于富集在晶粒边界,使晶粒边界的成分含量高于晶粒内部。
这种成分偏析严重影响了铝合金的综合性能。
首先是力学性能方面,晶粒边界富裕的铜、锌会降低合金的延展性和韧性,使其易于出现脆断现象;其次,成分偏析还会导致晶界腐蚀和晶界腐蚀裂纹的产生,并加剧合金的腐蚀敏感性。
析出相偏析主要涉及合金中形成的各种化合物或表面沉淀物。
在凝固过程中,某些金属元素对应的析出相在合金中的分布会出现不均匀的现象。
例如,在含硅铝合金中,硅存在于合金中的形式是硅铝化合物。
这种硅铝化合物大多以颗粒状或板状的形式分布在铝合金中,而且在凝固过程中这些硅铝化合物往往倾向于集中在一些特定位置,导致该区域的硅含量大大超过平均值。
这种硅含量的偏高会影响铝合金的力学性能和耐腐蚀性能。
此外,其他一些金属元素也可能在固溶度不同的情况下形成不均匀的析出相,进而对铝合金的性能产生影响。
铝合金偏析的产生原因涉及多个方面。
首先是凝固速度的不同。
凝固速度快的区域,由于晶体生长速度较快,成分偏析程度较低。
而凝固速度慢的区域,相对来说晶体生长速度较慢,成分偏析程度较高。
其次,合金中各元素的固溶度差异也是造成成分偏析的重要原因。
合金中比较难溶解的元素往往在凝固过程中首先析出,并富集在晶粒边界上。