喷雾热解法--2009
宋怀河 喷雾热解法 -回复

宋怀河喷雾热解法-回复宋怀河喷雾热解法是一种独特的化学分析方法,广泛应用于物质成分的检测和分离。
它通过将样品溶解在溶剂中,然后喷雾成雾状,借助高温将溶剂蒸发,从而实现对样品中成分的分离和测定。
该方法具有操作简便、分析速度快、结果准确的特点,因此被广泛应用于化学、食品、制药等领域。
在宋怀河喷雾热解法的实施过程中,一般包括以下几个步骤:样品制备、溶剂选择、加热条件设置、喷雾热解、分析检测以及数据处理。
首先,样品制备是进行宋怀河喷雾热解法的前提。
样品的选择和处理对最终的分析结果有着至关重要的影响。
根据不同的分析目的,样品可以是固体、液体或气体。
对于固体样品,可以通过研磨、颗粒筛选等方法将其制备成适合溶解的形式。
而对于液体样品,则需要根据样品的特性选择合适的处理方法,如稀释、过滤等。
样品制备的目的是将样品中的目标成分有效地溶解并转移到溶剂中,以便进行后续的操作和分析。
其次,溶剂的选择对于宋怀河喷雾热解法的成败至关重要。
溶剂的选择应基于以下几个因素:样品的性质、目标成分的特性以及分析的需求。
一般来说,溶剂应具有较好的溶解性,能够有效地将样品中的目标成分溶解并将其转移到气相中。
此外,溶剂还应具有较低的沸点和蒸气压,以便在高温环境下迅速蒸发,从而实现目标成分的分离和测定。
常见的溶剂有甲醇、乙醇、二甲苯等。
设置适当的加热条件是宋怀河喷雾热解法成功实施的关键。
加热条件的选择应考虑到样品的性质和目标成分的特性。
一般来说,加热的温度和时间应足够高和足够长,以保证样品中的目标成分充分分离和蒸发。
温度的选择应尽量接近目标成分的沸点,从而实现高效的热解。
在操作中还应控制好加热的速度和稳定性,以确保样品的均匀加热和分析结果的准确性。
通过上述步骤的准备,样品可以进入喷雾热解的阶段。
在喷雾热解过程中,样品溶液会经过一个喷雾器,将其转化为雾状物。
喷雾器的选择应考虑到样品的溶解性、分析需求以及仪器的性能。
常见的喷雾器有射流分离器、雾化器等。
抵押房贷数量英国2009年2月增加4%
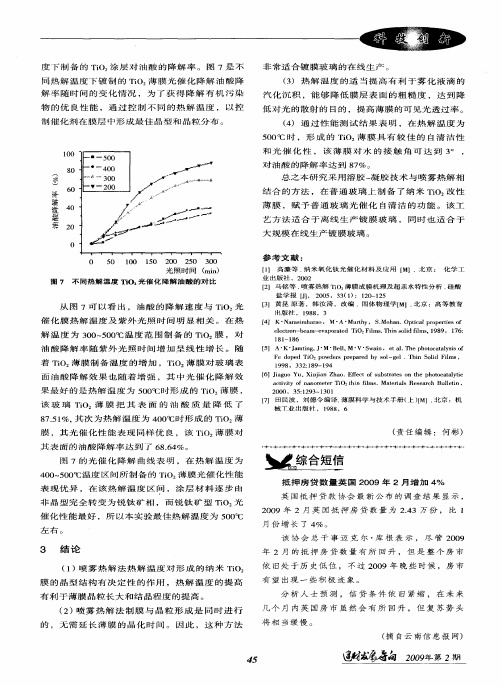
果 最 好 的 是 热 解 温 度 为 5 0 C时 形 成 的 T O 薄 膜 , 0 ̄ i
[] A ’ J mtn , ’ ’ l, ‘ S i 5 K‘a ig J M BelM V‘ wan,e . ep oo aay i f ta Th h te tlsso 1
e e to —b a lc r n e m—e a o a e O2 l s Th n s l l s l 8 , 1 6 v p r t d Ti m . i o i f m , 9 9 Fi d i 7 : 1 一l 6 81 8
油 酸 降 解 率 随 紫 外 光 照 时 间 增 加 呈 线 性 增 长 。 随
[] 黄 昆 原 著 ,韩 汝 漪 ,改 编 .固体 物 理 学 [ 3 M】. 京 :高 等 教 育 北
出版 社 , 18 9 8, 3
『] K ’ rsmh ro, M ‘ ’ rh 4 Naai aa A Mu ty, S. h n.Opia rp riso Mo a t lp o ete f c
a tvt f n n m ee O2t i ims cii o a o y t rTi h n f l 。M a e i l e e r h Bu l t , tra s R s a c lei n 2 o o O, 3 : 2 3 3 5 1 9 -1 01
该 玻 璃 T O,薄 膜 把 其 表 面 的 油 酸 质 量 降 低 了 i 8 .l 其 次 为 热 解 温 度 为 4 0 75 %, 0 ℃时 形 成 的 T O i 薄 膜 ,其 光 催 化 性 能 表 现 同 样 优 良 ,该 T O, 膜 对 i 薄 其 表 面 的 油 酸 降 解 率 达 到 了 6 .4 。 86 % 图 7的 光 催 化 降 解 曲 线 表 明 , 在 热 解 温 度 为
纳米粉末的制备方法
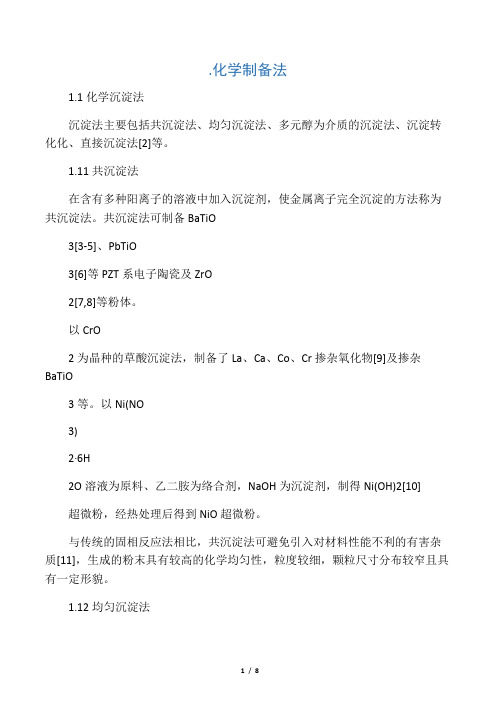
.化学制备法1.1化学沉淀法沉淀法主要包括共沉淀法、均匀沉淀法、多元醇为介质的沉淀法、沉淀转化化、直接沉淀法[2]等。
1.11共沉淀法在含有多种阳离子的溶液中加入沉淀剂,使金属离子完全沉淀的方法称为共沉淀法。
共沉淀法可制备BaTiO3[3-5]、PbTiO3[6]等PZT系电子陶瓷及ZrO2[7,8]等粉体。
以CrO2为晶种的草酸沉淀法,制备了La、Ca、Co、Cr掺杂氧化物[9]及掺杂BaTiO3等。
以Ni(NO3)2·6H2O溶液为原料、乙二胺为络合剂,NaOH为沉淀剂,制得Ni(OH)2[10]超微粉,经热处理后得到NiO超微粉。
与传统的固相反应法相比,共沉淀法可避免引入对材料性能不利的有害杂质[11],生成的粉末具有较高的化学均匀性,粒度较细,颗粒尺寸分布较窄且具有一定形貌。
1.12均匀沉淀法在溶液中加入某种能缓慢生成沉淀剂的物质,使溶液中的沉淀均匀出现,称为均匀沉淀法。
本法克服了由外部向溶液中直接加入沉淀剂而造成沉淀剂的局部不均匀性。
本法多数在金属盐溶液中采用尿素热分解生成沉淀剂NH4OH,促使沉淀均匀生成。
制备的粉体有Al、Zr、Fe、Sn的氢氧化物[12-17]及Nd2(CO3)3[18,19]等。
1.13xx沉淀法许多无机化合物可溶于多元醇,由于多元醇具有较高的沸点,可大于100°C,因此可用高温强制水解反应制备纳米颗粒[20]。
例如Zn(HAC)2·2H2O溶于一缩二乙醇(DEG),于100-220°C 下强制水解可制得单分散球形ZnO纳米粒子。
又如使酸化的FeCl3---乙二醇---水体系强制水解可制得均匀的Fe(III)氧化物胶粒[21]。
1.14沉淀转化法本法依据化合物之间溶解度的不同,通过改变沉淀转化剂的浓度、转化温度以及表面活性剂来控制颗粒生长和防止颗粒团聚。
例如:以Cu(NO3)2·3H2O、Ni(NO3)2·6H2O为原料,分别以Na2CO3、NaC2O4为沉淀剂,加入一定量表面活性剂,加热搅拌,分别以NaC2O3、NaOH为沉淀转化剂,可制得CuO、Ni(OH)2、NiO超细粉末[22]。
超声喷雾热解法制备Co9S8纳米球壳

第 7卷第 3期 20 0 7年 9月
南京师范大学学报 ( 程技术版 ) 工 JU N LO A J GN R A NV R IY E GN E IGA DT C N L G DSO ) O R A FN NI O M LU IE S ( N IE RN N E H O O YE I N N T
a o t 0 m.A p s i l g o t c a im sp p s d b u 0 n 5 o sb e rw h me h ns wa r o e . o Ke r s y wo d :C 9 8 n n s el , l a o i p a y o y i o , a o h l ut s n c s r y p r lss S s r
用固相反应法 , 例如 , 在石英管中, 按化学计量 比混合 固体的单质硫和单质钴 , 在高温时可以合成 c 。。 os化 合物 J将钴的硫酸盐与氢气或硫化氢气体混合 , 55C . 在 2  ̄ 时反应也能够得到固体 C 。 近几年 已有 oS[ . 7 3
文献 报道水 热法 低温合 成纳米 c 。多晶 J水 热法 制备 的 晶体具有 结 晶性好 、 量优 等 特点 . os , 质 目前 , c 。 os 的多种形 貌 已经被合 成 …J而其纳 米球壳 的制备却 鲜见 报道 . , 超声 喷雾 热解技术 能够 得到 均匀 和微
Ho lw 9 8Na o h l y t e ie y Ul a o i p a y o y i M e h d l o Co n s el S n h sz d b t s n c S r y P r lss S s r to
W a g De g h ,Y n Pe ge ,W a g Sh n y e n n z i i n f i n e g u
雾化热解法制备活性氧化性
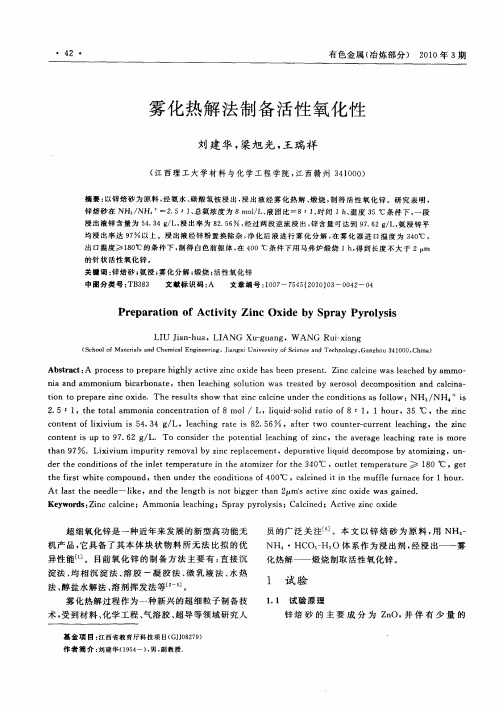
d rt ec n i o so h n e e e a u ei h t mie o h 4  ̄ ,o t ttmp r t r e h o d t n f ei ltt mp r t r n t ea o z rf rt e3 0C i t u l e ea u e≥ 1 0 ℃ ,g t e 8 e
锌 焙 砂 在 NH3NH 一2 5; 、 氨 浓 度 为 8mo/ 液 固 比 =8: 、 间 1h 温 度 3 / 4 . I总 l L、 1时 、 5℃ 条 件 下 , 段 一 浸 出 液 锌含 量 为 5. 4g L, 出率 为 8 . 6 , 过 两 段 逆 流 浸 出 , 含 量 可 达 到 9 . 2g L, 浸 锌平 4 3 / 浸 2 5 经 锌 76 / 氨 均 浸 出 率 达 9 以 上 。浸 出液 经 锌 粉 置 换 除 杂 , 化 后 液 进 行 雾 化 分 解 , 雾 化 器 进 口温 度 为 3 02 7 净 在 4* , ( 出 口温度 ≥ 10(的 条件 下 , 得 白色 前 躯 体 , 4 0℃条 件 下 用 马 弗 炉 煅 烧 1h 得 到 长度 不 大 于 2 r 8" 2 制 在 0 , n 的 针状 活性 氧化 锌 。 关键词 : 焙砂 ; 锌 氨浸 ; 化 分 解 ; 烧 ; 性 氧 化 锌 雾 煅 活
・
4 2・
有色金 属 ( 冶炼 部分 ) 2 1 0 0年 3 期
宋怀河 喷雾热解法
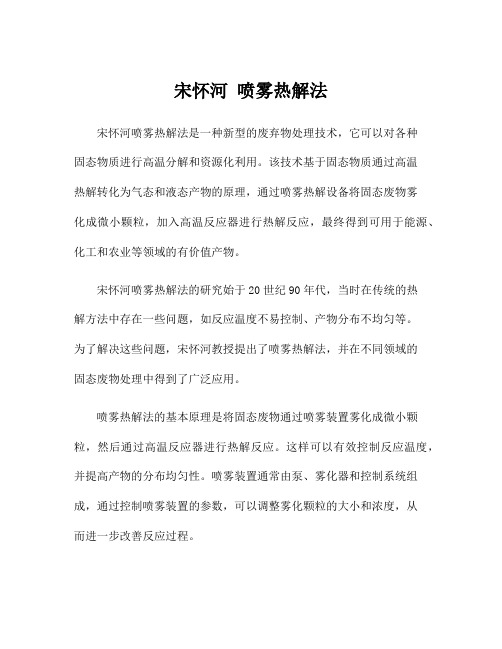
宋怀河喷雾热解法宋怀河喷雾热解法是一种新型的废弃物处理技术,它可以对各种固态物质进行高温分解和资源化利用。
该技术基于固态物质通过高温热解转化为气态和液态产物的原理,通过喷雾热解设备将固态废物雾化成微小颗粒,加入高温反应器进行热解反应,最终得到可用于能源、化工和农业等领域的有价值产物。
宋怀河喷雾热解法的研究始于20世纪90年代,当时在传统的热解方法中存在一些问题,如反应温度不易控制、产物分布不均匀等。
为了解决这些问题,宋怀河教授提出了喷雾热解法,并在不同领域的固态废物处理中得到了广泛应用。
喷雾热解法的基本原理是将固态废物通过喷雾装置雾化成微小颗粒,然后通过高温反应器进行热解反应。
这样可以有效控制反应温度,并提高产物的分布均匀性。
喷雾装置通常由泵、雾化器和控制系统组成,通过控制喷雾装置的参数,可以调整雾化颗粒的大小和浓度,从而进一步改善反应过程。
在喷雾热解反应中,固态废物经过高温和催化剂的作用,发生热解分解反应。
固态废物中的有机物质会转化为气态产物,包括油气和气体。
同时,一部分无机物质会转化为液态产物,如石蜡和沥青。
这些产物可以进一步利用,用于能源生产和化工原料的制备。
喷雾热解法的优点主要体现在以下几个方面:首先,该技术对固态废物的处理能力较强。
不同于传统的焚化、填埋等处理方法,喷雾热解法可以处理多种废物,包括固体和半固体废物,如废纸、废塑料、废橡胶和废油渣等。
这可以有效解决废物处理中的资源浪费和环境污染问题。
其次,喷雾热解法具有较高的热效率。
热效率是衡量热解技术性能的重要指标,它可以反映废物在热解过程中的能量利用效率。
与传统的热解方法相比,喷雾热解法具有更高的热效率,可以更充分地利用废物中的化学能,提高能源回收效果。
此外,喷雾热解法还具有较好的产物分布控制性。
通过调整喷雾装置的参数,可以控制反应温度和雾化颗粒的大小,从而影响产物的分布和形态。
这对于资源化利用来说非常重要,例如可用于发电的油气和可用于化工生产的液态产物。
喷雾热解法资料.
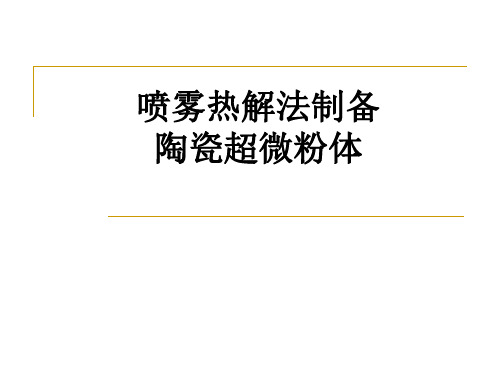
金属盐溶液
冻结液滴 溶剂升华
喷 雾 (10~20μm)
热风中溶 高温介质中 溶剂蒸发+
剂蒸发
溶剂蒸发
热分解
金属盐粒子
热分解
分类 冷冻干燥法
氧化物粒子 喷雾干燥法 热煤油法 喷雾热解法
喷雾干燥装置图
喷雾干燥法的特点
原料盐必须能溶于溶剂中 快速干燥,粉体呈球形; 粉体组分均一,纯度高; 可用于造粒。 如镍、铁、锌混合硫酸盐的制备,粒径约10~20μm
盐→200nm软铁氧体微粉
喷雾热解法
较为新颖的方法,最早出现于60年代末; 溶剂蒸发与金属盐热解在瞬间同时发生,生成产物与
原料盐具有不同的化学组成;也称为喷雾焙烧法,火 焰喷雾法,溶液蒸发分解法等。 喷雾可进入加热的反应器或喷至高温火焰两种方法, 一般用可燃性溶剂,以利用其燃烧热
喷雾热解装置图
1m 500nm
0.5M-g2-600
0.5M-g2-700
还原剂种类对粉体形貌的影响
1m
0.5M-g2-500
500nm 500nm
0.5M-u2-500
0.5M-u2-600
烧结体的SEM
0.5M-500
0.5M-g2-500 0.5M-u2-500 0.5M-u2-600
SDC样品的电导率与温度的关系
致密Ni的制备
改变以表面反应(沉淀)为主的反应方式,到以 体内反应(沉淀)为主的反应方式
方法:在Ni(NO3)3溶液中加入一定的氨水,使 之与Ni2+形成络合物
反应方程式
(a) T1 = 200 oC,
T2=400 oC, pure NiO;
(b) T1= 300 oC, T2 . =800oC, containing Ni and NiO;
锂离子电池磷酸铁锂正极材料的制备及改性研究进展
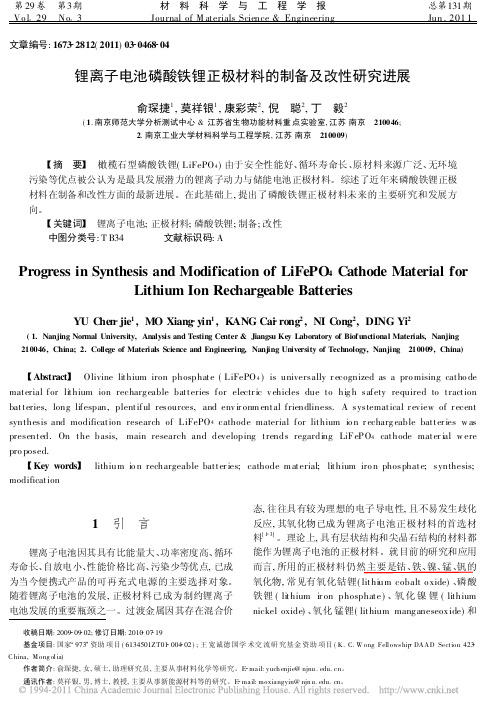
第29卷 第3期Vo l 29 No 3材 料 科 学 与 工 程 学 报Journal of M aterials Science &Engineering 总第131期Jun.2011文章编号:1673 2812(2011)03 0468 04锂离子电池磷酸铁锂正极材料的制备及改性研究进展俞琛捷1,莫祥银1,康彩荣2,倪 聪2,丁 毅2(1.南京师范大学分析测试中心&江苏省生物功能材料重点实验室,江苏南京 210046;2.南京工业大学材料科学与工程学院,江苏南京 210009)摘 要 橄榄石型磷酸铁锂(LiFePO 4)由于安全性能好、循环寿命长、原材料来源广泛、无环境污染等优点被公认为是最具发展潜力的锂离子动力与储能电池正极材料。
综述了近年来磷酸铁锂正极材料在制备和改性方面的最新进展。
在此基础上,提出了磷酸铁锂正极材料未来的主要研究和发展方向。
关键词 锂离子电池;正极材料;磷酸铁锂;制备;改性中图分类号:T B34 文献标识码:AProgress in Synthesis and Modification of LiFePO 4Cathode Material forLithium Ion Rechargeable BatteriesYU C hen jie 1,MO Xiang yin 1,KANG Cai rong 2,NI C ong 2,DING Yi 2(1.Nanjing Normal University,Analysis and Testing Center &Jiangsu Key Laboratory of Biof unctional Materials,Nanjing 210046,China;2.College of Materials Science and Engineering,Nanjing University of Technology,Nanjing 210009,China)Abstract Olivine lithium iron phosphate (LiFePO 4)is universally r ecognized as a pro mising catho de material for lithium ion recharg eable batteries for electr ic v ehicles due to hig h safety required to traction batteries,long lifespan,plentiful resources,and env ir onm ental friendliness.A systematical r eview of r ecent synthesis and modification research of LiFePO 4cathode material for lithium io n r echarg eable batter ies w as presented.On the basis,main research and developing trends regarding LiFePO 4cathode mater ial w ere pro posed.Key words lithium io n rechargeable batter ies;cathode m aterial;lithium iro n phosphate;synthesis;modification收稿日期:2009 09 02;修订日期:2010 07 19基金项目:国家 973 资助项目(6134501ZT01 004 02);王宽诚德国学术交流研究基金资助项目(K.C.W ong Fellows hip DAAD Section 423 C hina,M ong olia)作者简介:俞琛捷,女,硕士,助理研究员,主要从事材料化学等研究。
- 1、下载文档前请自行甄别文档内容的完整性,平台不提供额外的编辑、内容补充、找答案等附加服务。
- 2、"仅部分预览"的文档,不可在线预览部分如存在完整性等问题,可反馈申请退款(可完整预览的文档不适用该条件!)。
- 3、如文档侵犯您的权益,请联系客服反馈,我们会尽快为您处理(人工客服工作时间:9:00-18:30)。
The characteristics of Ni–Co–Mn–O precursor and Li(Ni 1/3Co 1/3Mn 1/3)O 2cathode powders prepared by spray pyrolysisSeo Hee Ju,Yun Chan Kang *Department of Chemical Engineering,Konkuk University,1Hwayang-dong,Gwangjin-gu,Seoul 143-701,Republic of KoreaReceived 18February 2008;received in revised form 9May 2008;accepted 12June 2008Available online 22July 2008AbstractNi–Co–Mn–O precursor powders with spherical shape and dense structure were prepared by spray pyrolysis from a spray solution containing a drying control chemical additive (DCCA)and polymeric precursors.In contrast,the Ni–Co–Mn–O precursor powders obtained from a spray solution without additives had a hollow and porous morphology.Ni–Co–Mn–O precursor powders with a spherical shape and dense structure yielded Li(Ni 1/3Co 1/3Mn 1/3)O 2cathode powders with a spherical shape and fine size by means of a solid-state reaction with lithium hydroxide.The mean size of the spherical cathode powder was 1.1m m.The discharge capacity of the Li(Ni 1/3Co 1/3Mn 1/3)O 2powders with spherical shape and filled morphology was 195mA h g À1at a current density of 0.1C.The discharge capacities of the cathode powders with spherical shape and filled morphology at 558C decreased from 183to 154mA h g À1by the 30th cycle at a current density of 0.5C.#2008Elsevier Ltd and Techna Group S.r.l.All rights reserved.Keywords:A.Powders:gas-phase reaction;E.Batteries1.IntroductionThe electrochemical performance of a cathode in a secondary lithium battery is strongly affected by powder properties such as the powder morphology,specific surface area,crystallinity,and material composition [1–7].With respect to the powder morphology,spherical powders with narrow size distributions exhibit a better electrochemical performance than powders with irregular morphologies because of their high packing density [4–9].Fine-sized cathode powders have been extensively investigated with a view to improving the capacity and power output of secondary lithium batteries.A combined process involving liquid solution and solid-state reaction methods was developed to produce cathode powders with spherical shape and large size [10,11].Precursor powders with spherical shape were prepared by liquid solution methods such as coprecipitation.Precursor powders with spherical shape and dense morphology formed spherical-shaped cathode powders by means of a solid-state reaction with lithium components.A combined process involving a gas-phasereaction and solid-state reaction was also studied to produce these cathode powders [5,12,13].The precursor powders obtained by spray pyrolysis—a type of gas-phase reaction—had spherical shape and fine size.However,the characteristics of the cathode powders obtained by the solid-state reaction method were affected by the morphologies of the precursor powder obtained by spray pyrolysis.Li(Ni 1/3Co 1/3Mn 1/3)O 2powders have been extensively studied from the viewpoint of applications as a cathode material for lithium ion batteries because of their high capacity,high working voltage,and high stability [4,5,14–17].In a previous study,Ni–Co–Mn–O precursor powders with sphe-rical shape and fine size were prepared by ultrasonic spray pyrolysis [5].On the other hand,Li(Ni 1/3Co 1/3Mn 1/3)O 2cathode powders formed by solid-state reaction method using Ni–Co–Mn–O precursor powders obtained by spray pyrolysis had non-spherical shape.The spherical shape of the precursor powders disappeared after solid-state reaction at high temperature.In this study,the precursor powders of Ni–Co–Mn–O components were prepared by spray pyrolysis.The precursor powders prepared by the conventional spray pyrolysis process using aqueous spray solutions had a hollow and porous structure because of fast drying and decomposition rates of droplets or powders inside the hot wall reactor.Therefore,in/locate/ceramintAvailable online at Ceramics International 35(2009)1205–1210*Corresponding author.Tel.:+82220496010;fax:+8224583504.E-mail address:yckang@konkuk.ac.kr (Y .C.Kang).0272-8842/$34.00#2008Elsevier Ltd and Techna Group S.r.l.All rights reserved.doi:10.1016/j.ceramint.2008.06.007this study,a drying control chemical additive (DCCA)and polymeric precursors were applied to a spray solution of nickel,cobalt,and manganese components in order to improve the morphologies of the precursor powders obtained by spray pyrolysis.Li(Ni 1/3Co 1/3Mn 1/3)O 2cathode powders with sphe-rical shape and fine size were prepared by a solid-state reaction method using the Ni–Co–Mn–O precursor powders obtained by spray pyrolysis.The charge/discharge capacities of the prepared Li(Ni 1/3Co 1/3Mn 1/3)O 2cathode powders were inves-tigated.2.ExperimentalThe spray pyrolysis system comprises a droplet generator,quartz reactor,and powder collector [18].A 1.7-MHz ultrasonic spray generator with six vibrators was used to generate a large amount of droplets;these are carried into a high-temperature tubular reactor by a carrier gas.The droplets and powders evaporated,decomposed,and/or crystallized in the quartz reactor.The length and diameter of the quartz reactor are 1200and 50mm,respectively.The reactor temperature was maintained at 9008C.The flow rate of the air used as the carrier gas was 10l/min.The precursor solution was prepared by dissolving a stoichiometric ratio of 1:1:1nickel nitrate hexahydrate [Ni(NO 3)2Á6H 2O,Aldrich],cobalt nitrate hexahy-drate [Co(NO 3)2Á6H 2O,Aldrich],and manganese acetate tetrahydrate [Mn(CH 3COO)Á4H 2O,Aldrich]salts in distilledwater.The overall solution concentration of the nickel,cobalt,and manganese components was 0.5M.DCCA,citric acid,and ethylene glycol were added to the spray solution to control the morphology of the precursor powders.The concentrations of N ,N -dimethylformamide used as DCCA were changed from 0.3to 1.5M.The concentrations of citric acid and ethylene glycol were both 0.1M.The Ni–Co–Mn–O precursor powders obtained by spray pyrolysis were reacted with lithium hydroxide by the solid-state reaction method.The mixture of precursor powders and lithium hydroxide was post-treated at a temperature of 8008C for 3h under air atmosphere.The lithium component added to the spray solution reacted with the precursor powders exceeded the stoichiometric amount by 3%so that Li(Ni 1/3Co 1/3Mn 1/3)O 2could be obtained.The crystal structures of the as-prepared and post-treated powders were investigated using X-ray diffractometry (XRD,RIGAKU DMAX-33)using Cu K a radiation at room temperature in the 2u range 10–808.The morphological characteristics of the powders were investigated using scanning electron microscopy (SEM,JEOL JSM-6060)and high-resolution transmission electron microscopy (TEM,FEI,TECHNAI 300K).The compositions of the powders were analyzed by using energy dispersive X-ray (EDX).The pore structures of the precursor powders were measured using the mercury porosimetry (AutoPore49500).The charge/discharge capacities of the prepared Li(Ni 1/3Co 1/3Mn 1/3)O 2powders were measured.The cathode electrode was madeofFig.1.SEM photographs of the as-prepared powders.(a)Aqueous solution.(b)0.1M CA/EG.(c)0.7M DCCA.(d)0.1M CA/EG,0.7M DCCA.S.H.Ju,Y.C.Kang /Ceramics International 35(2009)1205–1210120612mg of Li(Ni1/3Co1/3Mn1/3)O2compounds mixed with4mg of a conductive binder(3.2mg of teflonized acetylene black and0.8mg of graphite)that was pressed between two aluminum plates and then vacuum-dried overnight at1208C. The lithium metal and polypropylenefilm were used as the anode and the separator,respectively.The electrolyte used (TECHNO Semichem.Co.)was1M LiPF6in a1:1mixture by volume of EC/DMC.The entire cell was assembled in a glove box under an argon atmosphere.The initial charge/discharge capacities of the samples were measured through cycling in the 2.8–4.5V potential range at a constant current density of0.1C. The cycle performances of the cathode powders at an elevated temperature of558C were measured at a constant current density of0.5C.3.Results and discussionThe Ni–Co–Mn–O precursor powders were prepared by spray pyrolysis from spray solutions with and without additives.The effects of polymeric precursors and DCCA on the morphologies of the as-prepared and post-treated precursor powders were investigated.The as-prepared powders obtained by spray pyrolysis from the spray solutions with citric acid and ethylene glycol used as polymeric precursors had residual carbon components within the powders.Therefore,the as-prepared powders obtained by spray pyrolysis were post-treated at a temperature of8008C for3h for the removal of carbon components.The post-treatment of the as-prepared powders was also effective in clarifying the crystal structures of the components comprising the precursor powders.In this study, the post-treated powders were defined as the precursor powders. Fig.1shows SEM photographs of the as-prepared powders obtained by spray pyrolysis.The as-prepared powders obtained from the spray solution without additives had a size of the order of microns,in addition to a spherical shape and hollow morphology.The addition of citric acid and ethylene glycol to the spray solution slightly improved the structure of the as-prepared powders.The as-prepared powders formed from the polymeric precursor solution had a morefilled structure than those formed from the spray solution without additives.The as-prepared powders obtained from the spray solution with DCCA also had a hollow morphology.The addition of DCCA to the spray solutions with polymeric precursors improved the morphological characteristics of the as-prepared powders. The concentrations of the DCCAwere varied from0.3to1.5M. The as-prepared powders obtained from the spray solutions with polymeric precursors and DCCA had a spherical shape and filled morphology irrespective of the concentrations of DCCA. Thefilled inner structure of the as-prepared powders could be estimated from the fractured powders.The as-prepared powders obtained from the spray solutions with polymeric precursors and DCCA had a more smooth surface andfilled morphology than those obtained from the spray solutions without additives.Fig.2shows SEM photograph of the precursor powders.The as-prepared powders(Fig.1(d))were post-treated at a temperature of8008C.The precursor powders formed from the spray solution without additives had a hollow and porous structure.However,the precursor powders obtained from the spray solutions with polymeric precursors and DCCA had a spherical shape andfilled morphology as shown in Fig.2. The mean size of the precursor powders(Fig.2)as measured from the SEM photograph was1.1m m.Fig.3shows the pore size distributions,and cumulative intrusion and extrusion volumes of the precursor powders obtained from the spray solutions with and without additives. The concentrations of citric acid,ethylene glycol,and DCCA were0.1,0.1,and0.7M,respectively.The precursor powders obtained from the aqueous spray solution had larger pore volumes of meso and macro pores than those obtained from the spray solution with additives.Fig.3(b)shows the cumulative intrusion and extrusion volumes measured by mercury porosimetry.The total pore volume of the precursor powders obtained from the spray solutions with and without additives were0.9and1.1ml/g,respectively.The precursor powders with hollow and porous structures that were prepared from the post-treatment of the as-prepared powders obtained from the aqueous spray solutionformed Fig.2.SEM photograph of the precursorpowders.Fig. 3.The pore size distributions and cumulative pore volumes of the precursor powders.(a)Pore size distributions.(b)Cumulative pore volumes of intrusion and extrusion.S.H.Ju,Y.C.Kang/Ceramics International35(2009)1205–12101207Li(Ni 1/3Co 1/3Mn 1/3)O 2cathode powders with a non-spherical shape and fractured structure after the solid-state reaction with lithium hydroxide,as shown in Fig.4(a).Precursor powders with a hollow morphology formed cathode powders with a hollow morphology and fractured structure.However,the Li(Ni 1/3Co 1/3Mn 1/3)O 2cathode powders (Fig.4(b))prepared from the precursor powders with spherical shape and filled morphologies,shown in Fig.2,had spherical shapes and filled morphologies.A TEM photograph of the cathode powders (Fig.4(b))shown in Fig.5shows the filled internal structure.Therefore,controlling the morphology of the precursor powders obtained by spray pyrolysis was of importance in the preparation of the Li(Ni 1/3Co 1/3Mn 1/3)O 2cathode powders with fine size and regular morphology by the solid-state reaction with lithium hydroxide.The mean size of the cathode powder (Fig.4(b))was 1.1m m.The compositions and crystal structures of the as-prepared,precursor and cathode powders were investigated from the EDX and XRD spectra.The as-prepared and precursor powders were formed from the spray solutions with DCCA and polymeric precursors.The concentration of DCCA was0.7M.In the EDX spectra,the composition ratios of the nickel,cobalt,and manganese components were analyzed.Table 1shows the compositions of the powders measured from the EDX spectra.The composition ratios of the nickel,cobalt,and manganese components were maintained in the as-prepared,precursor,and cathode powders.Fig.6shows the XRD spectra of the as-prepared,precursor,and cathode powders.In the XRD spectra,the as-prepared and precursor powders exhibited sharp crystal structures of Co 3O 4.The crystal structures of the nickel and manganese components could not be suitably observed in the XRD spectra of the as-prepared and precursor powders.In this study,the Ni–Co–Mn–O precursor powders were considered as mixtures of Mn 2O 3,Co 3O 4,and NiO [19].The prepared cathode powders had pure phases of Li(Ni 1/3Co 1/3Mn 1/3)O 2.The mean crystallite size of the cathode powders was calculated by Scherrer’s equation to be 33nm.The charge/discharge capacities of the cathode powders prepared from the precursor powders with different morphol-ogies were investigated.Fig.7shows the initialcharge/Fig.4.SEM photographs of the Li(Ni 1/3Co 1/3Mn 1/3)O 2cathode powders.(a)Aqueous solution.(b)0.1M CA/EG,0.7MDCC.Fig.5.TEM photograph of the cathode powders obtained from the spray solution with additives.Table 1Compositions of the as-prepared,precursor and cathode powders Samples Composition(Ni:Co:Mn atomic ratios)Average of composition As-prepared(1.11:1.06:1.00) 1.10:1.05:1.00(1.05:1.00:1.01)(1.13:1.09:1.00)Precursor (1.05:1.01:1.00) 1.05:1.00:1.01(1.05:1.00:1.03)(1.04:1.01:1.00)Cathode(1.04:1.02:1.00) 1.04:1.00:1.00(1.03:1.00:1.01)(1.06:1.00:1.01)S.H.Ju,Y.C.Kang /Ceramics International 35(2009)1205–12101208discharge curves of the Li/Li(Ni 1/3Co 1/3Mn 1/3)O 2cells cycled at 2.8–4.5V at a constant current density of 0.1C.The initial discharge capacity of the Li(Ni 1/3Co 1/3Mn 1/3)O 2powders prepared from the precursor powders with hollow morphology,which were obtained from the spray solution without additives,was 179mA h g À1.However,the initial discharge capacity of the Li(Ni 1/3Co 1/3Mn 1/3)O 2powders prepared from the pre-cursor powders with filled morphology,which were obtained from the spray solution with citric acid,ethylene glycol,and DCCA,was 195mA h g À1.The prepared cathode powders had similar discharge capacity to those of the powders prepared by various preparation methods [5,13,15].The concentrations of DCCA added to the spray solutions to control the morphologies of the precursor powders did not affect the initial discharge capacities of the cathode powders prepared by the solid-state reaction with lithium hydroxide.The cycle performances of the cathode powders with hollow and filled morphologies at an elevated temperature of 558C are shown in Fig.8.The discharge capacities of the cathode powders with filled morphology decreased from 183to154mA h g À1by the 30th cycle at a current density of 0.5C.On the other hand,the discharge capacities of the cathode powders with hollow morphology decreased from 164to 120mA h g À1by the 30th cycle at a current density of 0.58C.4.ConclusionsLi(Ni 1/3Co 1/3Mn 1/3)O 2cathode powders with spherical shape and filled morphology were prepared by the solid-state reaction method using precursor powders with controlled morphology.The morphology of the Ni–Co–Mn–O precursor powders obtained by spray pyrolysis affected the morphol-ogy of the Li(Ni 1/3Co 1/3Mn 1/3)O 2cathode powders.The precursor powders with hollow and porous structures formed Li(Ni 1/3Co 1/3Mn 1/3)O 2cathode powders with hollow and fractured morphology.The precursor powders with spherical shape and dense structure,which were obtained from the spray solutions with DCCA and polymeric precursors,formed Li(Ni 1/3Co 1/3Mn 1/3)O 2cathode powders with sphe-rical shape and filled morphology.The prepared Li(Ni 1/3Co 1/3Mn 1/3)O 2cathode powders had good charge/discharge capacities.References[1]K.Matsuda,I.Taniguchi,Relationship between the electrochemical andparticle properties of LiMn 2O 4prepared by ultrasonic spray pyrolysis,J.Power Sources 132(2004)156–160.[2]I.Taniguchi,Powder properties of partially substituted LiM x Mn 2Àx O 4(M =Al,Cr,Fe and Co)synthesized by ultrasonic spray pyrolysis,Mater.Chem.Phys.92(2005)172–179.[3]Y .K.Sun,D.W.Kim,Y .M.Choi,Synthesis and characterization of spinelLiMn 2Àx Ni x O 4for lithium/polymer battery applications,J.Power Sources 79(1999)231–237.[4]Z.Bakenov,I.Taniguchi,Electrochemical performance of nanostructuredLiM x Mn 2Àx O 4(M =Co and Al)powders at high charge–discharge opera-tions,Solid State Ionics 176(2005)1027–1034.[5]S.H.Park,C.S.Yoon,S.G.Kang,H.S.Kim,S.I.Moon,Y .K.Sun,Synthesisand structural characterization of layered Li[Ni 1/3Co 1/3Mn 1/3]O 2cathodeFig.6.XRD spectra of the powders:(a)as-prepared,(b)precursor,(c)cathode.Fig.7.Charge/discharge curves of the cathode powders prepared from spray solution with and without additives at constant current density of 0.1C.Fig.8.Cycling performances at elevated temperatures (558C)of the cathode powders prepared from spray solution with and without additives at a constant current density of 0.5C.S.H.Ju,Y.C.Kang /Ceramics International 35(2009)1205–12101209materials by ultrasonic spray pyrolysis method,Electrochim.Acta49(2004) 557–563.[6]I.Taniguchi,D.Song,M.Wakihara,Electrochemical properties of LiM1/6Mn11/6O4(M=Mn,Co,Al and Ni)as cathode materials for Li-ion batteries prepared by ultrasonic spray pyrolysis method,J.Power Sources 109(2002)333–339.[7]I.Taniguchi,C.K.Lim,D.Song,M.Wakihara,Particle morphology andelectrochemical performances of spinel LiMn2O4powders synthesized using ultrasonic spray pyrolysis method,Solid State Ionics146(2002) 239–247.[8]S.H.Park,Y.K.Sun,Synthesis and electrochemical properties of5Vspinel LiNi0.5Mn1.5O4cathode materials prepared by ultrasonic spray pyrolysis method,Electrochim.Acta50(2004)434–439.[9]S.W.Oh,S.H.Park,C.W.Park,Y.K.Sun,Structural and electrochemicalproperties of layered Li[Ni0.5Mn0.5]1Àx Co x O2positive materials synthe-sized by ultrasonic spray pyrolysis method,Solid State Ionics171(2004) 167–172.[10]G.H.Kim,S.T.Myung,H.S.Kim,Y.K.Sun,Synthesis of sphericalLi[Ni(1/3Àz)CO(1/3Àz)Mn(1/3Àz)Mg z]O2as positive electrode material for lithium-ion battery,Electrochim.Acta51(2006)2447–2453.[11]T.H.Cho,S.M.Park,M.Yoshio,T.Hirai,Y.Hideshima,Effect ofsynthesis condition on the structural and electrochemical properties of Li[Ni1/3Mn1/3Co1/3]O2prepared by carbonate co-precipitation method,J.Power Sources142(2005)306–312.[12]S.W.Oh,S.H.Park,J.H.Kim,Y.C.Bae,Y.K.Sun,Improvement ofelectrochemical properties of LiNi0.5Mn1.5O4spinel material byfluorine substitution,J.Power Sources157(2006)464–470.[13]S.H.Ju,H.C.Jang,Y.C.Kang,Al-doped Ni-rich cathode powdersprepared from the precursor powders withfine size and spherical shape, Electrochim.Acta52(2007)7286–7292.[14]N.Yabuuchi,T.Ohzuku,Novel lithium insertion material of LiCo1/3Ni1/3Mn1/3O2for advanced lithium-ion batteries,J.Power Sources119–121 (2003)171–174.[15]S.Patoux,M.M.Doeff,Direct synthesis of LiNi1/3Co1/3Mn1/3O2fromnitrate precursors,mun.6(2004)767–772.[16]D.C.Li,T.Muta,L.Q.Hang,M.Yoshio,H.Noguchi,Effect of synthesismethod on the electrochemical performance of LiNi1/3Mn1/3Co1/3O2,J.Power Sources132(2004)150–155.[17]H.C.Wu,Z.Z.Guo,M.H.Yang,C.H.Lu,T.Y.Wu,I.Taniguchi,Spherical nanostructured LiNi1/3Co1/3Mn1/3O2powders prepared via an ultrasonic spray pyrolysis process,Chem.Lett.34(2005)1398–1399.[18]J.R.Sohn,Y.C.Kang,H.D.Park,Morphological control of Y2O3:Euphosphor particles by adding polymeric precursors in spray pyrolysis,Jpn.J.Appl.Phys.41(2002)3006–3009.[19]S.W.Oh,S.H.Park,K.Amine,Y.K.Sun,Synthesis and characterization ofspherical morphology[Ni0.4Co0.2Mn0.4]3O4materials for lithium second-ary batteries,J.Power Sources160(2006)558–562.S.H.Ju,Y.C.Kang/Ceramics International35(2009)1205–1210 1210。