Predictive Control in Power Electronics and Drives
电力电子专业词汇.

电力电子专业英语词汇Absorber Circuit 吸收电路AC/AC Frequency Converter 交交变频电路AC power control交流电力控制AC Power Controller交流调功电路AC Power Electronic Switch交流电力电子开关Ac V oltage Controller交流调压电路Asynchronous Modulation异步调制Baker Clamping Circuit贝克箝位电路Bi-directional Triode Thyristor双向晶闸管Bipolar Junction Transistor-- BJT双极结型晶体管Boost-Buck Chopper升降压斩波电路Boost Chopper升压斩波电路Boost Converter升压变换器Bridge Reversible Chopper桥式可逆斩波电路Buck Chopper 降压斩波电路Buck Converter降压变换器Commutation 换流Conduction Angle 导通角Constant V oltage Constant Frequency --CVCF 恒压恒频Continuous Conduction--CCM (电流)连续模式Control Circuit 控制电路Cuk Circuit CUK 斩波电路Current Reversible Chopper 电流可逆斩波电路Current Source Type Inverter--CSTI 电流(源)型逆变电路Cycloconvertor 周波变流器DC-AC-DC Converter 直交直电路DC Chopping 直流斩波DC Chopping Circuit 直流斩波电路DC-DC Converter 直流-直流变换器Device Commutation 器件换流Direct Current Control 直接电流控制Discontinuous Conduction mode (电流)断续模式displacement factor 位移因数distortion power 畸变功率double end converter 双端电路driving circuit 驱动电路electrical isolation 电气隔离fast acting fuse 快速熔断器fast recovery diode 快恢复二极管fast revcovery epitaxial diodes 快恢复外延二极管fast switching thyristor 快速晶闸管field controlled thyristor 场控晶闸管flyback converter 反激电流forced commutation 强迫换流forward converter 正激电路frequency converter 变频器full bridge converter 全桥电路full bridge rectifier 全桥整流电路full wave rectifier 全波整流电路gate turn-off thyristor——GTO 可关断晶闸管general purpose diode 普通二极管giant transistor——GTR 电力晶体管half bridge converter 半桥电路hard switching 硬开关high voltage IC 高压集成电路hysteresis comparison 带环比较方式indirect current control 间接电流控制indirect DC-DC converter 直接电流变换电路insulated-gate bipolar transistor---IGBT 绝缘栅双极晶体管intelligent power module---IPM 智能功率模块integrated gate-commutated thyristor---IGCT 集成门极换流晶闸管inversion 逆变latching effect 擎住效应leakage inductance 漏感light triggered thyristo---LTT 光控晶闸管line commutation 电网换流load commutation 负载换流loop current 环流背板backplane带隙电压参考Band gap voltage reference工作台电源benchtop supply方块图Block Diagram波特图Bode Plot自举Bootstrap桶形电容bucket capcitor机架chassis恒流源constant current source铁芯饱和Core Sataration交叉频率crossover frequency纹波电流current ripple逐周期Cycle by Cycle周期跳步cycle skipping死区时间Dead Time核心温度DIE Temperature非使能,无效,禁用,关断Disable主极点dominant pole 主极点使能,有效,启用Enable额定值ESD Rating ESD评估板Evaluation Board超过下面的规格使用可能引起永久的设备损害或设备故障.建议不要工作在电特性表规定的参数范围以外. Exceeding the specifications below may result in permanent damage to the device, or device malfunction. Operation outside of the parameters specified in the Electrical Characteristics section is not implied.下降沿Failling edge品质因数figure of merit浮充电压float charge voltage反驰式功率级flyback power stage前向压降orward voltage drop自由运行free-running续流二极管Freewheel diode满负载Full load栅极驱动gate drive栅极驱动级gate drive stage图gerber plot Gerber接地层ground plane电感单位(亨利) Henry人体模式Human Body Model滞回Hysteresis涌入电流inrush current反相Inverting抖动jittery结点Junction开尔文连接Kelvin connection引脚框架Lead Frame无铅Lead Free电平移动level-shift电源调整率Line regulation负载调整率load regulation批号Lot Number低压差Low Dropout密勒Miller节点node非反相Non-Inverting新颖的novel关断状态off state电源工作电压Operating supply voltage输出驱动级out drive stage异相Out of Phase产品型号Part NumberP沟道MOSFET P-channel MOSFET相位裕度Phase margin开关节点Phase Node便携式电子设备portable electronics掉电power down电源正常Power Good功率地Power Groud节电模式Power Save Mode上电Power up下拉pull down上拉pull up逐脉冲Pulse by Pulse推挽转换器push pull converter斜降ramp down斜升ramp up冗余二极管redundant diode电阻分压器resistive divider振铃ringing纹波电流ripple current上升沿rising edge检测电阻sense resistor序列电源Sequenced Power Supplys直通,同时导通shoot-through杂散电感stray inductances子电路sub-circuit基板substrate电信Telecom热性能信息Thermal Information散热片thermal slug阈值Threshold振荡电阻timing resistor线路,走线,引线Trace传递函数Transfer function 跳变点Trip Point 跳变点匝数比(初级匝数/次级匝数)turns ratio (Np / Ns)欠压锁定Under V oltage Lock Out (UVLO)电压参考V oltage Reference伏秒积voltage-second product零极点频率补偿zero-pole frequency compensation拍频beat frequency单击电路one shots缩放scaling等效串联电阻ESR地电位Ground平衡带隙trimmed bandgap压差dropout voltage大容量电容large bulk capacitance断路器circuit breaker电荷泵charge pump过冲overshoot元件设备三绕组变压器:three-column transformer ThrClnTrans双绕组变压器:double-column transformer DblClmnTrans 电容器:Capacitor并联电容器:shunt capacitor电抗器:Reactor母线:Busbar输电线:TransmissionLine发电厂:power plant断路器:Breaker刀闸(隔离开关):Isolator分接头:tap电动机:motor状态参数有功:active power无功:reactive power电流:current容量:capacity电压:voltage档位:tap position有功损耗:reactive loss无功损耗:active loss功率因数:power-factor功率:power功角:power-angle电压等级:voltage grade空载损耗o-load loss铁损:iron loss铜损:copper loss空载电流o-load current阻抗:impedance正序阻抗:positive sequence impedance负序阻抗egative sequence impedance零序阻抗:zero sequence impedance电阻:resistor电抗:reactance电导:conductance电纳:susceptance无功负载:reactive load 或者QLoad有功负载: active load PLoad遥测:YC(telemetering)遥信:YX励磁电流(转子电流):magnetizing current定子:stator功角:power-angle上限:upper limit下限:lower limit并列的:apposable高压: high voltage低压:low voltage中压ddle voltage电力系统power system发电机generator励磁excitation励磁器excitor电压voltage电流current母线bus变压器transformer升压变压器step-up transformer高压侧high side输电系统power transmission system输电线transmission line固定串联电容补偿fixed series capacitor compensation 稳定stability电压稳定voltage stability功角稳定angle stability暂态稳定transient stability电厂power plant能量输送power transfer交流AC装机容量installed capacity电网power system落点drop point开关站switch station双回同杆并架double-circuit lines on the same tower 变电站transformer substation补偿度degree of compensation高抗high voltage shunt reactor无功补偿reactive power compensation故障fault调节regulation裕度magin三相故障three phase fault故障切除时间fault clearing time极限切除时间critical clearing time切机generator triping高顶值high limited value强行励磁reinforced excitation线路补偿器LDC(line drop compensation)机端generator terminal静态static (state)动态dynamic (state)单机无穷大系统one machine - infinity bus system 机端电压控制A VR电抗reactance电阻resistance功角power angle有功(功率) active power无功(功率) reactive power功率因数power factor无功电流reactive current下降特性droop characteristics斜率slope额定rating变比ratio参考值reference value电压互感器PT分接头tap下降率droop rate仿真分析simulation analysis传递函数transfer function框图block diagram受端receive-side裕度margin同步synchronization失去同步loss of synchronization阻尼damping摇摆swing保护断路器circuit breaker电阻:resistance电抗:reactance阻抗:impedance电导:conductance电纳:susceptance导纳:admittance电感:inductance电容: capacitance电源专业词汇(二)coupling 耦合intermittent 周期的dislocation 错位propeller 螺旋桨switchgear 配电装置dispersion 差量flange 法兰盘dielectric 介电的binder 胶合剂alignment 定位elastomer 合成橡胶corollary 必然的结果rabbet 插槽vent 通风孔subtle 敏感的gearbox 变速箱plate 电镀crucial 决定性的flexible 柔性的technics 工艺ultimate 最终的resilience 弹性vendor 自动售货机partition 分类rigid 刚性的prototype 样机diagram 特性曲线interfere 干涉compatible 兼容的simulation 模拟clutch 离合器refinement 精加工fixture 夹具torque 扭矩responsive 敏感的tensile 拉伸cushion 减震器rib 肋strength 强度packing 包装metallized 金属化stress 应力mitigate 减轻trade off 折衷方案yield 屈伸line shaft 中间轴matrix 母体inherent 固有的spindle 主轴aperture 孔径conformance 适应性axle 心轴turbulence 扰动specification 规范semipermanent 半永久性的enclosure 机壳specialization 规范化bolt 螺栓oscillation 振幅calling 职业nut 螺母anneal 退火vitalize 激发screw 螺丝polymer 聚合体revelation 揭示fastner 紧固件bind 凝固dissemination 分发rivit 铆钉mount 支架booster 推进器hub 轴套distortion 变形contractual 契约的coaxial 同心的module 模块verdict 裁决crank 曲柄slide 滑块malfunction 故障inertia 惰性medium 介质allegedly 假定active 活性的dissipation 损耗controversy 辩论lubrication 润滑assembly 总装dictate 支配graphite 石墨encapsulate 封装incumbent 义不容辞的derivative 派生物adhesive 粘合剂validation 使生效contaminate 沾染turbine 涡轮procurement 收购asperity 粗糙bearing 支撑架mortality 失败率metalworking 金属加工isostatic 均衡的shed light on 阐明viscous 粘稠的osculate 接触adversely 有害的grinding 研磨i mperative 强制的consistency 连续性corrosin 侵蚀lattice 晶格fitness 适应性flush 冲洗fracture 断裂warrant 保证inhibitor 防腐剂diffusivity 扩散率turning 车工dispersant 分散剂vice versa 反之亦然ways 导轨deteriorate 降低tribological 摩擦的hybrid 混合物neutralize 平衡screen 屏蔽ID=inside diameterpulley 滑轮exclusion 隔绝OD=outside diameterhydraulic 液压的insulation 绝缘reciprocate 往复运动delicate 精密的elaborate 加工dress 精整dampen 阻尼incontrovertible 无可争议的by and large 大体上pivotal 中枢的luminous 发光的plastic 塑胶utilitarian 功利主义out of round 失园organic 有机的grass root 基层premature 过早的film 薄膜state-of-the -art 技术发展水平guard 防护罩polyester 聚酯blade 托板permeate 渗入epoxy 环氧的carrier 载体spillage 溢出polypropylene 聚丙烯chuck 卡盘erosion 浸蚀photoconductive 光敏的infeed 横向进给routine 程序miniaturization 小型化lapping 抛光postprocess 后置处理asynchronism 异步milling 洗削solder-bump 焊点synchronization 同步speciality 专业grid 栅格respond 响应stroke 行程impedance 阻抗feedback 反馈attachment 备件approximately 大约aberrance 畸变tapered 楔形的purported 据说steady 稳态的casting 铸件consumable 消费品dynamic 动态的index 换档inductance 电感transient 瞬态的stop 挡块capacitance 电容coordinate 坐标contour 轮廓resistance 电容curve 曲线machine center 加工中心audion 三极管diagram 特性曲线capitalize 投资diode 二极管history 关系曲线potentiometer 电位器transistor 晶体管gradient 斜率know-how 实践知识choker 扼流圈parabola 抛物线potted 封装的filter 滤波器root 根mechatronics 机电一体化transformer 变压器eigenvalue 特征值stem from 起源于fuse 保险丝function 函数rule-based 基于规则的annular core 磁环vector 向量consolidation 巩固radiator 散热器reciprocal 倒数energize 激发regulator 稳压器virtual value 有效值synchronous 同时发生bobbin 骨架square root 平方根socket 插孔tape 胶带cube 立方polarity 极性ceramic capacitor 瓷片电容integral 积分armature 电枢electrolytic C 电解电容differential 微分installment 分期付款self-tapping screw 自攻螺丝hisgram 直方图lobe 凸起footprint 封装ratio 比率plunge 钻入resin 松香grade down 成比例降低servo 伺服机构solderability 可焊性proportion 比例dedicated 专用的shock 机械冲击inverse ratio 反比interpolation 插补endurance 耐久性direct ratio 正比compensation 校正initial value 初始值plus 加upload 加载flashing 飞弧subtract 减overload 过载canned 千篇一律的multiply 乘lightload 轻载lot 抽签divide 除stagger 交错排列parallel 并联impedance 阻抗traverse 横向in series 串联damp 阻尼longitudinal 纵向的equivalent 等效的reactance 电抗latitudinal 横向的terminal 终端admittance 导纳restrain 约束creep 蠕动susceptance 电纳square 平方Hyperlink 超级连接spring 触发memo 备忘录wastage 损耗presentation 陈述principle 原理binder 打包planer 刨床source program 源程序Client-Server Model客户机server 服务器table 表query 查询form 表单report 报表macro 宏module 模块field 字段record 记录电源专业词汇(三)printed circuit 印制电路printed wiring 印制线路printed board 印制板printed circuit board 印制板电路printed wiring board 印制线路板printed component 印制元件printed contact 印制接点printed board assembly 印制板装配board 板rigid printed board 刚性印制板flexible printed circuit 挠性印制电路flexible printed wiring 挠性印制线路flush printed board 齐平印制板metal core printed board 金属芯印制板metal base printed board 金属基印制板mulit-wiring printed board 多重布线印制板molded circuit board 模塑电路板discrete wiring board 散线印制板micro wire board 微线印制板buile-up printed board 积层印制板surface laminar circuit 表面层合电路板B2it printed board 埋入凸块连印制板chip on board 载芯片板buried resistance board 埋电阻板mother board 母板daughter board 子板backplane 背板bare board 裸板copper-invar-copper board 键盘板夹心板dynamic flex board 动态挠性板static flex board 静态挠性板break-away planel 可断拼板cable 电缆flexible flat cable (FFC) 挠性扁平电缆membrane switch 薄膜开关hybrid circuit 混合电路thick film 厚膜thick film circuit 厚膜电路thin film 薄膜thin film hybrid circuit 薄膜混合电路interconnection 互连conductor trace line 导线flush conductor 齐平导线transmission line 传输线crossover 跨交edge-board contact 板边插头stiffener 增强板substrate 基底real estate 基板面conductor side 导线面component side 元件面solder side 焊接面printing 印制grid 网格pattern 图形conductive pattern 导电图形non-conductive pattern 非导电图形legend 字符mark 标志base material 基材laminate 层压板metal-clad bade material 覆金属箔基材copper-clad laminate (CCL) 覆铜箔层压板composite laminate 复合层压板thin laminate 薄层压板basis material 基体材料prepreg 预浸材料bonding sheet 粘结片preimpregnated bonding sheer 预浸粘结片epoxy glass substrate 环氧玻璃基板mass lamination panel 预制内层覆箔板core material 内层芯板bonding layer 粘结层film adhesive 粘结膜unsupported adhesive film 无支撑胶粘剂膜cover layer (cover lay) 覆盖层stiffener material 增强板材copper-clad surface 铜箔面foil removal surface 去铜箔面unclad laminate surface 层压板面base film surface 基膜面adhesive faec 胶粘剂面plate finish 原始光洁面matt finish 粗面length wise direction 纵向cross wise direction 模向cut to size panel 剪切板ultra thin laminate 超薄型层压板A-stage resin A阶树脂B-stage resin B阶树脂C-stage resin C阶树脂epoxy resin 环氧树脂phenolic resin 酚醛树脂polyester resin 聚酯树脂polyimide resin 聚酰亚胺树脂bismaleimide-triazine resin 双马来酰亚胺三嗪树脂acrylic resin 丙烯酸树脂melamine formaldehyde resin 三聚氰胺甲醛树脂polyfunctional epoxy resin 多官能环氧树脂brominated epoxy resin 溴化环氧树脂epoxy novolac 环氧酚醛fluroresin 氟树脂silicone resin 硅树脂silane 硅烷polymer 聚合物amorphous polymer 无定形聚合物crystalline polamer 结晶现象dimorphism 双晶现象copolymer 共聚物synthetic 合成树脂thermosetting resin 热固性树脂thermoplastic resin 热塑性树脂photosensitive resin 感光性树脂epoxy value 环氧值dicyandiamide 双氰胺binder 粘结剂adesive 胶粘剂curing agent 固化剂flame retardant 阻燃剂opaquer 遮光剂plasticizers 增塑剂unsatuiated polyester 不饱和聚酯polyester 聚酯薄膜polyimide film (PI) 聚酰亚胺薄膜polytetrafluoetylene (PTFE) 聚四氟乙烯reinforcing material 增强材料glass fiber 玻璃纤维E-glass fibre E玻璃纤维D-glass fibre D玻璃纤维S-glass fibre S玻璃纤维glass fabric 玻璃布non-woven fabric 非织布glass mats 玻璃纤维垫yarn 纱线filament 单丝strand 绞股weft yarn 纬纱warp yarn 经纱denier 但尼尔warp-wise 经向thread count 织物经纬密度weave structure 织物组织plain structure 平纹组织grey fabric 坏布woven scrim 稀松织物bow of weave 弓纬end missing 断经mis-picks 缺纬bias 纬斜crease 折痕waviness 云织fish eye 鱼眼feather length 毛圈长mark 厚薄段split 裂缝twist of yarn 捻度size content 浸润剂含量size residue 浸润剂残留量finish level 处理剂含量size 浸润剂couplint agent 偶联剂finished fabric 处理织物polyarmide fiber 聚酰胺纤维aromatic polyamide paper 聚芳酰胺纤维纸breaking length 断裂长height of capillary rise 吸水高度wet strength retention 湿强度保留率whitenness 白度ceramics 陶瓷conductive foil 导电箔copper foil 铜箔rolled copper foil 压延铜箔annealed copper foil 退火铜箔thin copper foil 薄铜箔adhesive coated foil 涂胶铜箔resin coated copper foil 涂胶脂铜箔composite metallic material 复合金属箔carrier foil 载体箔invar 殷瓦foil profile 箔(剖面)轮廓shiny side 光面matte side 粗糙面treated side 处理面stain proofing 防锈处理double treated foil 双面处理铜箔shematic diagram 原理图logic diagram 逻辑图printed wire layout 印制线路布设master drawing 布设总图computer aided drawing 计算机辅助制图computer controlled display 计算机控制显示placement 布局routing 布线layout 布图设计rerouting 重布simulation 模拟logic simulation 逻辑模拟circit simulation 电路模拟timing simulation 时序模拟modularization 模块化layout effeciency 布线完成率MDF databse 机器描述格式数据库design database 设计数据库design origin 设计原点optimization (design) 优化(设计) predominant axis 供设计优化坐标轴table origin 表格原点mirroring 镜像drive file 驱动文件intermediate file 中间文件manufacturing documentation 制造文件queue support database 队列支撑数据库component positioning 元件安置graphics dispaly 图形显示scaling factor 比例因子scan filling 扫描填充rectangle filling 矩形填充region filling 填充域physical design 实体设计logic design 逻辑设计logic circuit 逻辑电路hierarchical design 层次设计top-down design 自顶向下设计bottom-up design 自底向上设计net 线网digitzing 数字化design rule checking 设计规则检查router (CAD) 走(布)线器net list 网络表subnet 子线网objective function 目标函数post design processing (PDP) 设计后处理interactive drawing design 交互式制图设计cost metrix 费用矩阵engineering drawing 工程图block diagram 方块框图moze 迷宫component density 元件密度traveling salesman problem 回售货员问题degrees freedom 自由度out going degree 入度incoming degree 出度manhatton distance 曼哈顿距离euclidean distance 欧几里德距离network 网络array 阵列segment 段logic 逻辑logic design automation 逻辑设计自动化separated time 分线separated layer 分层definite sequence 定顺序conduction (track) 导线(通道)conductor width 导线(体)宽度conductor spacing 导线距离conductor layer 导线层conductor line/space 导线宽度/间距conductor layer No.1 第一导线层round pad 圆形盘square pad 方形盘diamond pad 菱形盘oblong pad 长方形焊盘bullet pad 子弹形盘teardrop pad 泪滴盘snowman pad 雪人盘V-shaped pad V形盘annular pad 环形盘non-circular pad 非圆形盘isolation pad 隔离盘monfunctional pad 非功能连接盘offset land 偏置连接盘back-bard land 腹(背)裸盘anchoring spaur 盘址land pattern 连接盘图形land grid array 连接盘网格阵列annular ring 孔环component hole 元件孔mounting hole 安装孔supported hole 支撑孔unsupported hole 非支撑孔via 导通孔plated through hole (PTH) 镀通孔access hole 余隙孔blind via (hole) 盲孔buried via hole 埋孔buried blind via 埋,盲孔any layer inner via hole 任意层内部导通孔all drilled hole 全部钻孔toaling hole 定位孔landless hole 无连接盘孔interstitial hole 中间孔landless via hole 无连接盘导通孔pilot hole 引导孔terminal clearomee hole 端接全隙孔dimensioned hole 准尺寸孔via-in-pad 在连接盘中导通孔hole location 孔位hole density 孔密度hole pattern 孔图drill drawing 钻孔图assembly drawing 装配图datum referan 参考基准printed circuit 印制电路printed wiring 印制线路printed board 印制板printed circuit board 印制板电路printed wiring board 印制线路板printed component 印制元件printed contact 印制接点printed board assembly 印制板装配board 板rigid printed board 刚性印制板flexible printed circuit 挠性印制电路flexible printed wiring 挠性印制线路flush printed board 齐平印制板metal core printed board 金属芯印制板metal base printed board 金属基印制板mulit-wiring printed board 多重布线印制板molded circuit board 模塑电路板discrete wiring board 散线印制板micro wire board 微线印制板buile-up printed board 积层印制板surface laminar circuit 表面层合电路板B2it printed board 埋入凸块连印制板chip on board 载芯片板buried resistance board 埋电阻板mother board 母板daughter board 子板backplane 背板bare board 裸板copper-invar-copper board 键盘板夹心板dynamic flex board 动态挠性板static flex board 静态挠性板break-away planel 可断拼板cable 电缆flexible flat cable (FFC) 挠性扁平电缆membrane switch 薄膜开关hybrid circuit 混合电路thick film 厚膜thick film circuit 厚膜电路thin film 薄膜thin film hybrid circuit 薄膜混合电路interconnection 互连conductor trace line 导线flush conductor 齐平导线transmission line 传输线crossover 跨交edge-board contact 板边插头stiffener 增强板substrate 基底real estate 基板面conductor side 导线面component side 元件面solder side 焊接面printing 印制grid 网格pattern 图形conductive pattern 导电图形non-conductive pattern 非导电图形legend 字符mark 标志base material 基材laminate 层压板metal-clad bade material 覆金属箔基材copper-clad laminate (CCL) 覆铜箔层压板composite laminate 复合层压板thin laminate 薄层压板basis material 基体材料prepreg 预浸材料bonding sheet 粘结片preimpregnated bonding sheer 预浸粘结片epoxy glass substrate 环氧玻璃基板mass lamination panel 预制内层覆箔板core material 内层芯板bonding layer 粘结层film adhesive 粘结膜unsupported adhesive film 无支撑胶粘剂膜cover layer (cover lay) 覆盖层stiffener material 增强板材copper-clad surface 铜箔面foil removal surface 去铜箔面unclad laminate surface 层压板面base film surface 基膜面adhesive faec 胶粘剂面plate finish 原始光洁面matt finish 粗面length wise direction 纵向cross wise direction 模向cut to size panel 剪切板ultra thin laminate 超薄型层压板A-stage resin A阶树脂B-stage resin B阶树脂C-stage resin C阶树脂epoxy resin 环氧树脂phenolic resin 酚醛树脂polyester resin 聚酯树脂polyimide resin 聚酰亚胺树脂bismaleimide-triazine resin 双马来酰亚胺三嗪树脂acrylic resin 丙烯酸树脂melamine formaldehyde resin 三聚氰胺甲醛树脂polyfunctional epoxy resin 多官能环氧树脂brominated epoxy resin 溴化环氧树脂epoxy novolac 环氧酚醛fluroresin 氟树脂silicone resin 硅树脂silane 硅烷polymer 聚合物amorphous polymer 无定形聚合物crystalline polamer 结晶现象dimorphism 双晶现象copolymer 共聚物synthetic 合成树脂thermosetting resin 热固性树脂thermoplastic resin 热塑性树脂photosensitive resin 感光性树脂epoxy value 环氧值dicyandiamide 双氰胺binder 粘结剂adesive 胶粘剂curing agent 固化剂flame retardant 阻燃剂opaquer 遮光剂plasticizers 增塑剂unsatuiated polyester 不饱和聚酯polyester 聚酯薄膜polyimide film (PI) 聚酰亚胺薄膜polytetrafluoetylene (PTFE) 聚四氟乙烯reinforcing material 增强材料glass fiber 玻璃纤维E-glass fibre E玻璃纤维D-glass fibre D玻璃纤维S-glass fibre S玻璃纤维glass fabric 玻璃布non-woven fabric 非织布glass mats 玻璃纤维垫yarn 纱线filament 单丝strand 绞股weft yarn 纬纱warp yarn 经纱denier 但尼尔warp-wise 经向thread count 织物经纬密度weave structure 织物组织plain structure 平纹组织grey fabric 坏布woven scrim 稀松织物bow of weave 弓纬end missing 断经mis-picks 缺纬bias 纬斜crease 折痕waviness 云织fish eye 鱼眼feather length 毛圈长mark 厚薄段split 裂缝twist of yarn 捻度size content 浸润剂含量size residue 浸润剂残留量finish level 处理剂含量size 浸润剂couplint agent 偶联剂finished fabric 处理织物polyarmide fiber 聚酰胺纤维aromatic polyamide paper 聚芳酰胺纤维纸breaking length 断裂长height of capillary rise 吸水高度wet strength retention 湿强度保留率whitenness 白度ceramics 陶瓷conductive foil 导电箔copper foil 铜箔rolled copper foil 压延铜箔annealed copper foil 退火铜箔thin copper foil 薄铜箔adhesive coated foil 涂胶铜箔resin coated copper foil 涂胶脂铜箔composite metallic material 复合金属箔carrier foil 载体箔invar 殷瓦foil profile 箔(剖面)轮廓shiny side 光面matte side 粗糙面treated side 处理面stain proofing 防锈处理double treated foil 双面处理铜箔shematic diagram 原理图logic diagram 逻辑图printed wire layout 印制线路布设master drawing 布设总图computer aided drawing 计算机辅助制图computer controlled display 计算机控制显示placement 布局routing 布线layout 布图设计rerouting 重布simulation 模拟logic simulation 逻辑模拟circit simulation 电路模拟timing simulation 时序模拟modularization 模块化layout effeciency 布线完成率MDF databse 机器描述格式数据库design database 设计数据库design origin 设计原点optimization (design) 优化(设计) predominant axis 供设计优化坐标轴table origin 表格原点mirroring 镜像drive file 驱动文件intermediate file 中间文件manufacturing documentation 制造文件queue support database 队列支撑数据库component positioning 元件安置graphics dispaly 图形显示scaling factor 比例因子scan filling 扫描填充rectangle filling 矩形填充region filling 填充域physical design 实体设计logic design 逻辑设计logic circuit 逻辑电路hierarchical design 层次设计top-down design 自顶向下设计bottom-up design 自底向上设计net 线网digitzing 数字化design rule checking 设计规则检查router (CAD) 走(布)线器net list 网络表subnet 子线网objective function 目标函数post design processing (PDP) 设计后处理interactive drawing design 交互式制图设计cost metrix 费用矩阵engineering drawing 工程图block diagram 方块框图moze 迷宫component density 元件密度traveling salesman problem 回售货员问题degrees freedom 自由度out going degree 入度incoming degree 出度manhatton distance 曼哈顿距离euclidean distance 欧几里德距离network 网络array 阵列segment 段logic 逻辑logic design automation 逻辑设计自动化separated time 分线separated layer 分层definite sequence 定顺序conduction (track) 导线(通道)conductor width 导线(体)宽度conductor spacing 导线距离conductor layer 导线层conductor line/space 导线宽度/间距conductor layer No.1 第一导线层round pad 圆形盘square pad 方形盘diamond pad 菱形盘oblong pad 长方形焊盘bullet pad 子弹形盘teardrop pad 泪滴盘snowman pad 雪人盘V-shaped pad V形盘annular pad 环形盘non-circular pad 非圆形盘isolation pad 隔离盘monfunctional pad 非功能连接盘offset land 偏置连接盘back-bard land 腹(背)裸盘anchoring spaur 盘址land pattern 连接盘图形land grid array 连接盘网格阵列annular ring 孔环component hole 元件孔mounting hole 安装孔supported hole 支撑孔unsupported hole 非支撑孔via 导通孔plated through hole (PTH) 镀通孔access hole 余隙孔blind via (hole) 盲孔buried via hole 埋孔buried blind via 埋,盲孔any layer inner via hole 任意层内部导通孔all drilled hole 全部钻孔toaling hole 定位孔landless hole 无连接盘孔interstitial hole 中间孔landless via hole 无连接盘导通孔pilot hole 引导孔terminal clearomee hole 端接全隙孔dimensioned hole 准尺寸孔via-in-pad 在连接盘中导通孔hole location 孔位hole density 孔密度hole pattern 孔图drill drawing 钻孔图assembly drawing 装配图datum referan 参考基准coupling 耦合intermittent 周期的dislocation 错位propeller 螺旋桨switchgear 配电装置dispersion 差量flange 法兰盘dielectric 介电的binder 胶合剂alignment 定位elastomer 合成橡胶corollary 必然的结果rabbet 插槽vent 通风孔subtle 敏感的gearbox 变速箱plate 电镀crucial 决定性的flexible 柔性的technics 工艺ultimate 最终的resilience 弹性vendor 自动售货机partition 分类rigid 刚性的prototype 样机diagram 特性曲线interfere 干涉compatible 兼容的simulation 模拟clutch 离合器refinement 精加工fixture 夹具torque 扭矩responsive 敏感的tensile 拉伸cushion 减震器rib 肋strength 强度packing 包装metallized 金属化stress 应力mitigate 减轻trade off 折衷方案yield 屈伸line shaft 中间轴matrix 母体inherent 固有的spindle 主轴aperture 孔径conformance 适应性axle 心轴turbulence 扰动specification 规范semipermanent 半永久性的enclosure 机壳specialization 规范化bolt 螺栓oscillation 振幅calling 职业nut 螺母anneal 退火vitalize 激发screw 螺丝polymer 聚合体revelation 揭示fastner 紧固件bind 凝固dissemination 分发rivit 铆钉mount 支架booster 推进器hub 轴套distortion 变形contractual 契约的coaxial 同心的module 模块verdict 裁决crank 曲柄slide 滑块malfunction 故障inertia 惰性medium 介质allegedly 假定active 活性的dissipation 损耗controversy 辩论lubrication 润滑assembly 总装dictate 支配graphite 石墨encapsulate 封装incumbent 义不容辞的derivative 派生物adhesive 粘合剂validation 使生效contaminate 沾染turbine 涡轮procurement 收购asperity 粗糙bearing 支撑架mortality 失败率metalworking 金属加工isostatic 均衡的shed light on 阐明viscous 粘稠的osculate 接触adversely 有害的grinding 研磨imperative 强制的consistency 连续性corrosin 侵蚀lattice 晶格fitness 适应性flush 冲洗fracture 断裂warrant 保证inhibitor 防腐剂diffusivity 扩散率turning 车工dispersant 分散剂vice versa 反之亦然ways 导轨deteriorate 降低tribological 摩擦的hybrid 混合物neutralize 平衡screen 屏蔽ID=inside diameterpulley 滑轮exclusion 隔绝OD=outside diameterhydraulic 液压的insulation 绝缘reciprocate 往复运动delicate 精密的elaborate 加工dress 精整dampen 阻尼incontrovertible 无可争议的by and large 大体上pivotal 中枢的luminous 发光的plastic 塑胶utilitarian 功利主义out of round 失园organic 有机的grass root 基层premature 过早的film 薄膜state-of-the -art 技术发展水平guard 防护罩polyester 聚酯blade 托板permeate 渗入epoxy 环氧的carrier 载体spillage 溢出polypropylene 聚丙烯chuck 卡盘erosion 浸蚀photoconductive 光敏的infeed 横向进给routine 程序miniaturization 小型化lapping 抛光postprocess 后置处理asynchronism 异步milling 洗削solder-bump 焊点synchronization 同步speciality 专业grid 栅格respond 响应stroke 行程impedance 阻抗feedback 反馈attachment 备件approximately 大约aberrance 畸变tapered 楔形的purported 据说steady 稳态的casting 铸件consumable 消费品dynamic 动态的index 换档inductance 电感transient 瞬态的stop 挡块capacitance 电容coordinate 坐标contour 轮廓resistance 电容curve 曲线machine center 加工中心audion 三极管diagram 特性曲线capitalize 投资diode 二极管history 关系曲线potentiometer 电位器transistor 晶体管gradient 斜率know-how 实践知识choker 扼流圈parabola 抛物线potted 封装的filter 滤波器root 根mechatronics 机电一体化transformer 变压器eigenvalue 特征值stem from 起源于fuse 保险丝function 函数rule-based 基于规则的annular core 磁环vector 向量consolidation 巩固radiator 散热器reciprocal 倒数energize 激发regulator 稳压器virtual value 有效值synchronous 同时发生bobbin 骨架square root 平方根socket 插孔tape 胶带cube 立方polarity 极性ceramic capacitor 瓷片电容integral 积分armature 电枢electrolytic C 电解电容differential 微分installment 分期付款self-tapping screw 自攻螺丝hisgram 直方图lobe 凸起footprint 封装ratio 比率plunge 钻入resin 松香grade down 成比例降低servo 伺服机构solderability 可焊性proportion 比例dedicated 专用的shock 机械冲击inverse ratio 反比interpolation 插补endurance 耐久性direct ratio 正比compensation 校正initial value 初始值plus 加upload 加载flashing 飞弧subtract 减overload 过载canned 千篇一律的multiply 乘lightload 轻载lot 抽签divide 除stagger 交错排列parallel 并联impedance 阻抗traverse 横向in series 串联damp 阻尼longitudinal 纵向的equivalent 等效的reactance 电抗latitudinal 横向的terminal 终端admittance 导纳restrain 约束creep 蠕动susceptance 电纳square 平方Hyperlink 超级连接spring 触发memo 备忘录wastage 损耗presentation 陈述principle 原理binder 打包planer 刨床source program 源程序Client-Server Model客户机server 服务器table 表query 查询form 表单report 报表macro 宏module 模块field 字段record 记录。
网络控制系统的时延补偿
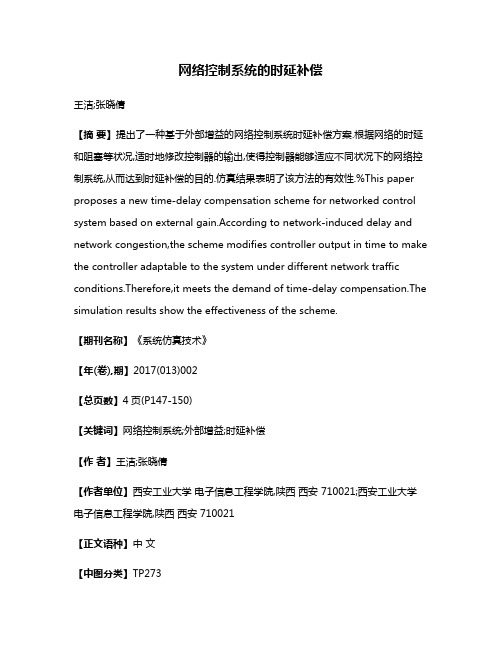
网络控制系统的时延补偿王洁;张晓倩【摘要】提出了一种基于外部增益的网络控制系统时延补偿方案.根据网络的时延和阻塞等状况,适时地修改控制器的输出,使得控制器能够适应不同状况下的网络控制系统,从而达到时延补偿的目的.仿真结果表明了该方法的有效性.%This paper proposes a new time-delay compensation scheme for networked control system based on external gain.According to network-induced delay and network congestion,the scheme modifies controller output in time to make the controller adaptable to the system under different network traffic conditions.Therefore,it meets the demand of time-delay compensation.The simulation results show the effectiveness of the scheme.【期刊名称】《系统仿真技术》【年(卷),期】2017(013)002【总页数】4页(P147-150)【关键词】网络控制系统;外部增益;时延补偿【作者】王洁;张晓倩【作者单位】西安工业大学电子信息工程学院,陕西西安 710021;西安工业大学电子信息工程学院,陕西西安 710021【正文语种】中文【中图分类】TP273网络的引入使得网络控制系统可以方便地得到维护并可以形成远程控制系统。
然而,网络诱导时延的存在会使网络控制系统性能下降甚至造成系统不稳定[1]。
如果网络时延是时变的和随机的,那么系统性能降低会加剧,同时不稳定的概率会加大。
电力电子技术课程设计--三相可控整流技术的工程应用
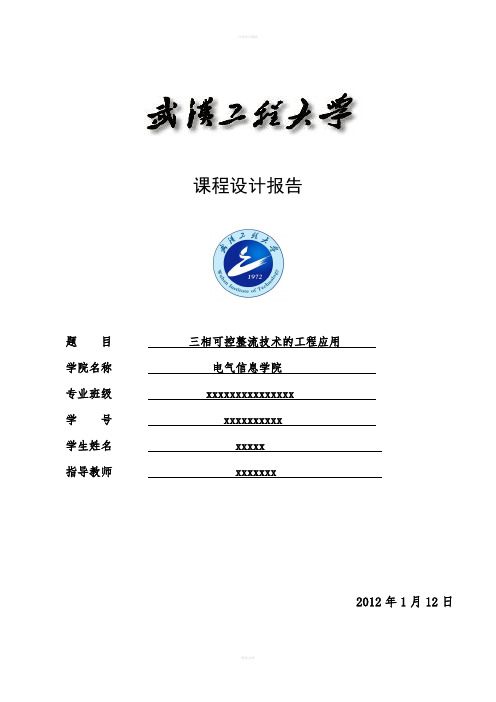
课程设计报告题目三相可控整流技术的工程应用学院名称电气信息学院专业班级 xxxxxxxxxxxxxxx学号 xxxxxxxxxx学生姓名 xxxxx指导教师 xxxxxxx2012年1月12日摘要电力电子技术在电力系统中有着非常广泛的应用。
据估计,发达国家在用户最终使用的电能中,有60%以上的电能至少经过一次以上电力电子变流装置的处理。
电力系统在通向现代化的进程中,电力电子技术是关键技术之一。
可以毫不夸张地说,如果离开电力电子技术,电力系统的现代化就是不可想象的。
整流电路技术在工业生产上应用极广。
如调压调速直流电源、电解及电镀的直流电源等。
整流电路就是把交流电能转换为直流电能的电路。
大多数整流电路由变压器、整流主电路和滤波器等组成。
它在直流电动机的调速、发电机的励磁调节、电解、电镀等领域得到广泛应用。
整流电路尤其是三相桥式可控整流电路是电力电子技术中最为重要也是应用得最为广泛的电路,不仅应用于一般工业,也广泛应用于交通运输、电力系统、通信系统、能源系统及其他领域。
因此对三相桥式可控整流电路的相关参数和不同性质负载的工作情况进行对比分析与研究具有很强的现实意义,这不仅是电力电子电路理论学习的重要一环,而且对工程实践的实际应用具有预测和指导作用。
关键词:电力电子三相桥式可控电路整流AbstractPower electronics technology has a very wide range of applications in the power system. It is estimated that in developed countries more than 60% of the electrical energy at least through the end-use of electricity, more than once device processing power electronic converters. Power system in the process leading to the modern power electronics technology is one of the key technologies. It is no exaggeration to say that, if you leave power electronics technology, the modernization of the electric power system is unthinkable.Rectifier circuit technology has very wide application in industrial production. Such as voltage variable speed DC power supply, electrolysis and electroplating DC power. The rectifying circuit is the AC power is converted to DC power circuit. Most of the rectifier circuit by the transformer, rectifier circuit, and filters. It has been widely used in the field of DC motor speed control, generator excitation regulator, electrolysis, electroplating.Rectifier circuit, especially the three-phase bridge controlled rectifier circuit is the most important and the most widely used application circuit in the power electronics technology is not only used in general industrial, is also widely used in the transportation, electric power systems, communication systems, energy systems and other fields. Comparative analysis and study of the three-phase bridge controlled rectifier circuit parameters and the different nature of the work load has great practical significance, this is not only an important part of the learning power electronic circuit theory and engineering practice The practical application of predictive and guiding role.Key words:Power electronic Three-phase bridge controlled circuit Rectifier目录摘要 (2)一.设计任务书 (5)二.设计说明 (6)2.1设计目的 (6)2.2作用 (6)2.3技术指标 (6)三.设计方案的选择 (7)3.1三相桥式可控整流电路原理 (7)3.2三相桥式可控整流电路原理图 (7)3.3三相桥式可控整流电路工作波形 (8)3.4总设计框图 (10)四.触发电路的设计 (11)五.保护电路的设计 (12)5.1过电压保护 (12)5.2过电流保护 (13)六.参数的计算 (14)七.器件选择清单 (15)八.三相桥式可控整流电路的工程应用 (16)九.心得体会 (16)参考文献 (17)一.设计任务书院系:xxxxxxxxx年级:xxxxxx专业班级:xxxxxxxxxx二.设计说明2.1设计目的合理运用所学知识,进行电力电子电路和系统设计的能力,理解和掌握常用的电力电子电路及系统的主电路、控制电路和保护电路的设计方法,掌握元器件的选择计算方法。
永磁同步电机鲁棒预测电流控制器设计
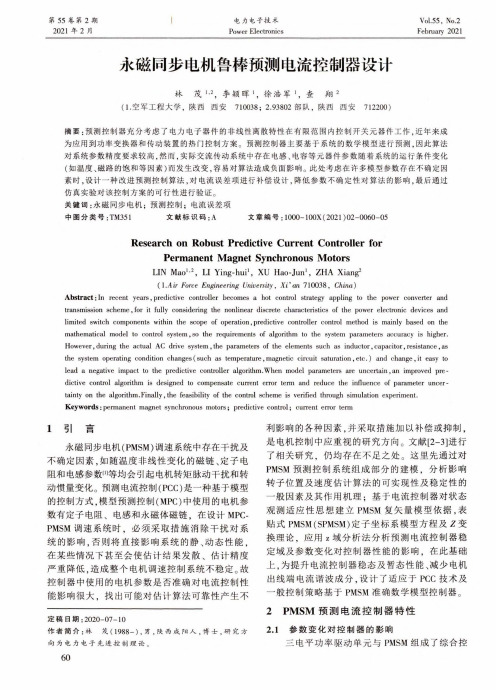
第55卷第2期2021年2月电力电子技术Power ElectronicsVol.55,No.2February2021永磁同步电机鲁棒预测电流控制器设计林茂李颖晖1,徐浩军1,查翔2(1.空军工程大学,陕西西安710038; 2.93802部队,陕西西安712200)摘要:预测控制器充分考虑了电力电子器件的非线性离散特性在有限范围内控制开关元器件工作,近年来成为应用到功率变换器和传动装置的热门控制方案。
预测控制器主要基于系统的数学模型进行预测,因此算法对系统参数精度要求较高,然而,实际交流传动系统中存在电感、电容等元器件参数随着系统的运行条件变化(如温度、磁路的饱和等因素)而发生改变,容易对算法造成负面影响。
此处考虑在许多模型参数存在不确定因素时,设计一种改进预测控制算法,对电流误差项进行补偿设计,降低参数不确定性对算法的影响,最后通过仿真实验对该控制方案的可行性进行验证。
关键词:永磁同步电机;预测控制;电流误差项中图分类号:TM351文献标识码:A文章编号:1000-100X(2021)02-0060-05Research on Robust Predictive Current Controller forPermanent Magnet Synchronous MotorsLIN Mao L2,LI Ying-hui1,XU Hao-Jun1,ZHA Xiang2(l.Air Force Engineering University,Xi*an710038,China)Abstract:In recent years,predictive controller becomes a hot control strategy appling to the power converter and transmission scheme,for it fully considering the nonlinear discrete characteristics of the power electronic devices and limited switch components within the scope of operation, predictive controller control method is mainly based on the mathematical model to control system,so the requirements of algorithm to the system parameters accuracy is higher. However,during the actual AC drive system, the parameters of the elements such as inductor, capacitor,resistance,as the system operating condition changes(such as temperature,magnetic circuit saturation,etc.)and change,it easy to lead a negative impact to the predictive controller algorithm.When model parameters are uncertain,an improved predictive control algorithm is designed to compensate current error term and reduce the influence of parameter uncertainty on the algorithm.Finally,the feasibility of the control scheme is verified through simulation experiment. Keywords:permanent magnet synchronous motors;predictive control;current error term1引言永磁同步电机(PMSM)调速系统中存在干扰及不确定因素,如随温度非线性变化的磁链、定子电阻和电感参数"等均会引起电机转矩脉动干扰和转动惯量变化。
艾默生DELTAV系统常见英语单词编译
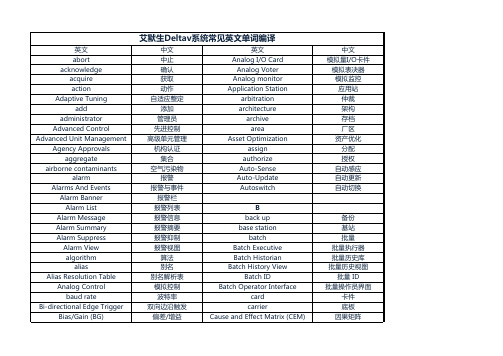
艾默生Deltav系统常见英文单词编译
中文
英文
中止
Analog I/O Card
确认
Analog Voter
获取
Analog monitor
动作
Application Station
自适应整定
arbitration
添加
architecture
管理员
archive
先进控制
area
高级单元管理
Asset Optimization
手动模式
Marine Certified
海上认证
Master Recipe
主配方
matrix
矩阵
Media Converter
媒介转换器
Mid Selector (MID)
中值选择器
Migrate Database
迁移数据库
Model Predictive Control Process 模型预估控制过程仿真
别名解析表
Batch ID
模拟控制
Batch Operator Interface
波特率
card
双向边沿触发
carrier
偏差/增益
Cause and Effect Matrix (CEM)
中文 模拟量I/O卡件
模拟表决器 模拟监控 应用站
仲裁 架构 存档 厂区 资产优化 分配 授权 自动感应 自动更新 自动切换
diode Discrete I/O Card
Discrete Input Discrete Output
download dry contact Dynamo set
Extensible Parameter External Phase
211202179_多模块双有源全桥变换器的模型预测控制
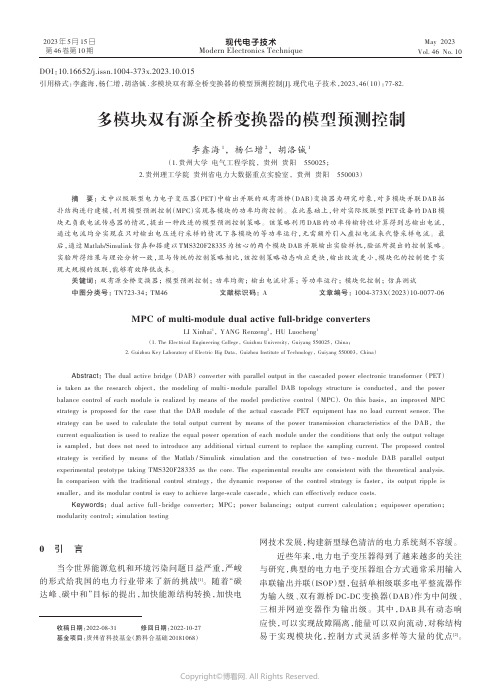
现代电子技术Modern Electronics TechniqueMay 2023Vol.46No.102023年5月15日第46卷第10期0引言当今世界能源危机和环境污染问题日益严重,严峻的形式给我国的电力行业带来了新的挑战[1]。
随着“碳达峰、碳中和”目标的提出,加快能源结构转换,加快电网技术发展,构建新型绿色清洁的电力系统刻不容缓。
近些年来,电力电子变压器得到了越来越多的关注与研究,典型的电力电子变压器组合方式通常采用输入串联输出并联(ISOP )型,包括单相级联多电平整流器作为输入级、双有源桥DC⁃DC 变换器(DAB )作为中间级、三相并网逆变器作为输出级。
其中,DAB 具有动态响应快,可以实现故障隔离,能量可以双向流动,对称结构易于实现模块化,控制方式灵活多样等大量的优点[2]。
DOI :10.16652/j.issn.1004⁃373x.2023.10.015引用格式:李鑫海,杨仁增,胡洛铖.多模块双有源全桥变换器的模型预测控制[J].现代电子技术,2023,46(10):77⁃82.多模块双有源全桥变换器的模型预测控制李鑫海1,杨仁增2,胡洛铖1(1.贵州大学电气工程学院,贵州贵阳550025;2.贵州理工学院贵州省电力大数据重点实验室,贵州贵阳550003)摘要:文中以级联型电力电子变压器(PET )中输出并联的双有源桥(DAB )变换器为研究对象,对多模块并联DAB 拓扑结构进行建模,利用模型预测控制(MPC )实现各模块的功率均衡控制。
在此基础上,针对实际级联型PET 设备的DAB 模块无负载电流传感器的情况,提出一种改进的模型预测控制策略。
该策略利用DAB 的功率传输特性计算得到总输出电流,通过电流均分实现在只对输出电压进行采样的情况下各模块的等功率运行,无需额外引入虚拟电流来代替采样电流。
最后,通过Matlab/Simulink 仿真和搭建以TMS320F28335为核心的两个模块DAB 并联输出实验样机,验证所提出的控制策略。
电子信息双语课后翻译
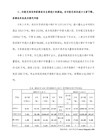
3.4A machine is run by an electic motor via a conveyor belt.
3.5The printing technique was spread from China to Europe.
4.1It is always desired to produce a well-regulated output voltage,in the presence of variations in the input voltage and load current.
6.2Another possible energy source is nuclear fusion.This process has not been fully developed,due to the extremely high temperatures that are produced as the fusion of atoms takes place.
7.3When placed in a strong electric field,carbon atoms can arrange themselves into sheets,which then fold up into a set of nested tubes.
7.4One application that may be commercially produced soon is nanometer-sized whiskers for use in lightweight com
1.5An electric motor consists of a magnet and an armature,which is a coil of wire mounted on a shaft that rotates inside the magnet's magnetic field.
2020年(电子行业企业管理)转贴电子专业英语
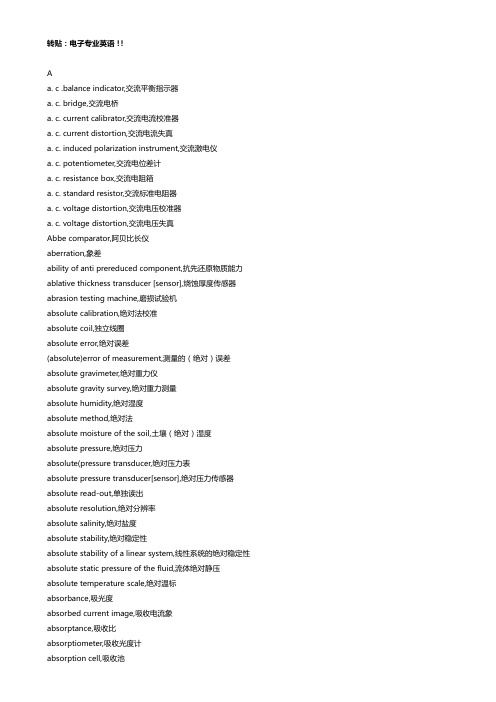
转贴:电子专业英语!!Aa. c .balance indicator,交流平衡指示器a. c. bridge,交流电桥a. c. current calibrator,交流电流校准器a. c. current distortion,交流电流失真a. c. induced polarization instrument,交流激电仪a. c. potentiometer,交流电位差计a. c. resistance box,交流电阻箱a. c. standard resistor,交流标准电阻器a. c. voltage distortion,交流电压校准器a. c. voltage distortion,交流电压失真Abbe comparator,阿贝比长仪aberration,象差ability of anti prereduced component,抗先还原物质能力ablative thickness transducer [sensor],烧蚀厚度传感器abrasion testing machine,磨损试验机absolute calibration,绝对法校准absolute coil,独立线圈absolute error,绝对误差(absolute)error of measurement,测量的(绝对)误差absolute gravimeter,绝对重力仪absolute gravity survey,绝对重力测量absolute humidity,绝对湿度absolute method,绝对法absolute moisture of the soil,土壤(绝对)湿度absolute pressure,绝对压力absolute(pressure transducer,绝对压力表absolute pressure transducer[sensor],绝对压力传感器absolute read-out,单独读出absolute resolution,绝对分辨率absolute salinity,绝对盐度absolute stability,绝对稳定性absolute stability of a linear system,线性系统的绝对稳定性absolute static pressure of the fluid,流体绝对静压absolute temperature scale,绝对温标absorbance,吸光度absorbed current image,吸收电流象absorptance,吸收比absorption coefficient,吸收系数absorption correction,吸收修正absorption edges,吸收边absorption factor,吸收系数absorption hygrometer,吸收温度表absorption spectrum,吸收光谱absorption X-ray spectrometry,吸收X射线谱法absorptivity,吸收率absorptivity of an absorbing,吸引材料的吸收率abstract system,抽象系统abundance sensityivity,丰度灵敏度AC-ACLVDT displacement transducer,交流差动变压器式位移传感器accelerated test,加速试验accelerationg voltage,加速电压acceleration,加速度acceleration error coefficient,加速度误差系数acceleration of gravity,重力加速度acceleration simulator,加速度仿真器acceleration transducer[sensor],加速度传感器accelerometer,加速度计acceptance of the mass filter,滤质器的接收容限acceptance test,验[交]收检验access,存取access time,存取时间accessibility,可及性accessories of testing machine,试验机附件accessory(for a measuring instrument),(测量仪表的)附件accessory hardware,附属硬件accessory of limited interchangeability,有限互换附件accumulated error,积累误差accumulated time difference,累积时差accumulative raingauge,累积雨量器accumulator,累加器accuracy,精[准]确度accuracy class,精[准]确度等级accuracy limit factor(of a protective current transformer), (保护用电流互感器的)精确度极限因数accuracy of measurement,测量精[准]确度accuracy of the wavelength,波长精确度accuracy rating,精确度限acetylene(pressure)gauge,乙炔压力表acetylene regulator,乙炔减压器acoustic current meter,声学海流计acoustic element,声学元件acoustic emission,声发射acoustic emission amplitude,声发射振幅acoustic emission analysis system,声发射分析系统acoustic emission detection system,声发射检测系统acoustic emission detector,声发射检测仪acoustic emission energy,声发射能量acoustic emission event,声发射事件acoustic emission preamplifier,声发射前置放大器acoustic emission pulser,声发射脉冲发生器acoustic emission rate,声发射率acoustic emission signal processor[conditioner],声发射信号处理器acoustic emission rate,声发射信号acoustic emission source location and analysis system,声发射源定位及分析系统acoustic emission source location system,声发射源定位系统acoustic emission source,声发射源acoustic emission spectrum,声发射频谱acoustic emission technique,声发射技术acoustic emission transducer[sensor],声发射换能器acoustic fatigue,声疲劳acoustic impedance,声阻抗acoustic logging instrument,声波测井仪acoustic malfunction,声失效acoustic matching layer,声匹配层acoustic(quantity)transducer[sensor],声(学量)传感器acoustic ratio,声比acoustic releaser,声释放器acoustic resistance,声阻acoustic thermometer,声学温度计;声波温度表acoustic tide gauge,回声验潮仪acoustic transponder,声应答器acoustical frequency electric,声频大地电场仪acoustical hologram,声全息图acoustical holography,声全息acoustical holography by electron-beam scanning,电子束扫描声全息acoustical holography by laser scanning,激光束扫描声全息acoustical holography by mechanical scanning,机械扫查声全息acoustical imaging by Bragg diffraction,布拉格衍射声成像acoustical impedance method,声阻法acquisition time,取数据时间actinometer,光能计;直接日射强度表;日射表(active)energy meter,(有功)电度表active gauge length,有效基长active gauge width,有效基宽active metal indicated electrode,活性金属指示电极active remote sensing,主动遥感active transducer[sensor],有源传感器activity,活度activity coefficient,活度系数actual material calibration,实物校准actual time of observation,实际观测时间actual transformation ratio of voltage transformer,电压互感器的实际变化actral transformation ratio of current transformer,电流互感器的实际变化actual value,实际值actual voltage ratio,实际电压比actuator,执行机构;驱动器actuator bellows,执行机构波纹管actuator load,执行机构负载actuator power unit,执行机构动力部件actuator sensor interface(ASI),执行器传感器接口actuator shaft,执行机构输出轴actuator spring,执行机构弹簧actuator stem,执行机构输出杆actuator stem force,执行机构刚度actuator travel characteristic,执行机构行程特性adaptation layer,适应层adaptive control,(自)适应控制adaptive control system,适应控制系统adaptive controller,适应控制器adaptive prediction,适应预报adaptive telemetering system,适应遥测系统adder,加法器addition method,叠加法additional correction,补充修正additivity of mass spectra,质谱的可迭加性address,地址adiabatic calorimeter,绝热式热量计adjust buffer total ion strength,总离子强度调节缓冲剂adjustable cistern barometer,动槽水银气压表adjustable relative humidity range,相对湿度可调范围adjustable temperature range,温度可调范围adjuster,调整机构;调节器adjustment,调整adjustment bellows,调节波纹管adjustment device,调整装置adjusting pin,校正针adsorbent,吸附剂adsorption chromatography,吸附色谱法aerial camera,航空照相机aerial remote sensing,航空遥感aerial surveying camera,航摄仪aerodynamic balance,空气动力学天平aerodynamic noise,气体动力噪声aerograph,高空气象计aerogravity survey,航空重力测量aerometeorograph,高空气象计aerosol,县浮微料;气溶胶aging of column,柱老化agitator,搅拌器agricultural analyzer,农用分析仪air-borne gravimeter,航空重力仪air capacitor,空气电容器air consumption,耗气量air damper,空气阻尼器air-deployable buoy,空投式极地浮标air-drop automatic station,空投自动气象站air duct,风道air gun,空气枪air inlet,进风口air lock,气锁阀air-lock device,锁气装置air outlet,回风口air pressrue balance,空气压力天平air pressure test,空气压力试验air sleeve,风(向)袋PCB用基材词汇中英文对照PCB用基材词汇中英文对照1、基材:base material2、层压板:laminate5、单面覆铜箔层压板:single-sided copper-clad laminate6、双面覆铜箔层压板:double-sided copper-clad laminate7、复合层压板:composite laminate8、薄层压板:thin laminate9、金属芯覆铜箔层压板:metal core copper-clad laminate10、金属基覆铜层压板:metal base copper-clad laminate11、挠性覆铜箔绝缘薄膜:flexible copper-clad dielectric film12、基体材料:basis material13、预浸材料:prepreg14、粘结片:bonding sheet15、预浸粘结片:preimpregnated bonding sheer16、环氧玻璃基板:epoxy glass substrate17、加成法用层压板:laminate for additive process18、预制内层覆箔板:mass lamination panel19、内层芯板:core material20、催化板材:catalyzed board ,coated catalyzed laminate21、涂胶催化层压板:adhesive-coated catalyzed laminate22、涂胶无催层压板:adhesive-coated uncatalyzed laminate23、粘结层:bonding layer24、粘结膜:film adhesive25、涂胶粘剂绝缘薄膜:adhesive coated dielectric film26、无支撑胶粘剂膜:unsupported adhesive film27、覆盖层:cover layer (cover lay)28、增强板材:stiffener material29、铜箔面:copper-clad surface30、去铜箔面:foil removal surface31、层压板面:unclad laminate surface32、基膜面:base film surface33、胶粘剂面:adhesive faec34、原始光洁面:plate finish35、粗面:matt finish36、纵向:length wise direction37、模向:cross wise direction38、剪切板:cut to size panel39、酚醛纸质覆铜箔板:phenolic cellulose paper copper-clad laminates (phenolic/paper CCL)40、环氧纸质覆铜箔板:epoxide cellulose paper copper-clad laminates (epoxy/paper CCL)41、环氧玻璃布基覆铜箔板:epoxide woven glass fabric copper-clad laminates42、环氧玻璃布纸复合覆铜箔板:epoxide cellulose paper core, glass cloth surfaces copper-clad laminates43、环氧玻璃布玻璃纤维复合覆铜箔板:epoxide non woven/woven glass reinforced copper-clad laminates44、聚酯玻璃布覆铜箔板:ployester woven glass fabric copper-clad laminateslamimates47、环氧合成纤维布覆铜箔板:epoxide synthetic fiber fabric copper-clad laminates48、聚四乙烯玻璃纤维覆铜箔板:teflon/fiber glass copper-clad laminates49、超薄型层压板:ultra thin laminate50、陶瓷基覆铜箔板:ceramics base copper-clad laminates51、紫外线阻挡型覆铜箔板:UV blocking copper-clad laminates101个热处理常用词汇1. indication 缺陷2. test specimen 试样3. bar 棒材4. stock 原料5. billet 方钢,钢方坯6. bloom 钢坯,钢锭7. section 型材8. steel ingot 钢锭9. blank 坯料,半成品10. cast steel 铸钢11. nodular cast iron 球墨铸铁12. ductile cast iron 球墨铸铁13. bronze 青铜14. brass 黄铜15. copper 合金16. stainless steel不锈钢17. decarburization 脱碳18. scale 氧化皮19. anneal 退火20. process anneal 进行退火21. quenching 淬火22. normalizing 正火23. Charpy impact text 夏比冲击试验24. fatigue 疲劳25. tensile testing 拉伸试验26. solution 固溶处理27. aging 时效处理28. Vickers hardness维氏硬度29. Rockwell hardness 洛氏硬度30. Brinell hardness 布氏硬度31. hardness tester硬度计32. descale 除污,除氧化皮等33. ferrite 铁素体37. iron carbide 渗碳体38. solid solution 固溶体39. sorbite 索氏体40. bainite 贝氏体41. pearlite 珠光体42. nodular fine pearlite/ troostite屈氏体43. black oxide coating 发黑44. grain 晶粒45. chromium 铬46. cadmium 镉47. tungsten 钨48. molybdenum 钼49. manganese 锰50. vanadium 钒51. molybdenum 钼52. silicon 硅53. sulfer/sulphur 硫54. phosphor/ phosphorus 磷55. nitrided 氮化的56. case hardening 表面硬化,表面淬硬57. air cooling 空冷58. furnace cooling 炉冷59. oil cooling 油冷60. electrocladding /plating 电镀61. brittleness 脆性62. strength 强度63. rigidity 刚性,刚度64. creep 蠕变65. deflection 挠度66. elongation 延伸率67. yield strength 屈服强度68. elastoplasticity 弹塑性69. metallographic structure 金相组织70. metallographic test 金相试验71. carbon content 含碳量72. induction hardening 感应淬火73. impedance matching 感应淬火74. hardening and tempering 调质75. crack 裂纹79. rolling 轧(件)80. drawing 拉(件)81. shot blasting 喷丸(处理)82. grit blasting 喷钢砂(处理)83. sand blasting 喷砂(处理)84. carburizing 渗碳85. nitriding 渗氮86. ageing/aging 时效87. grain size 晶粒度88. pore 气孔89. sonim 夹砂90. cinder inclusion 夹渣91. lattice晶格92. abrasion/abrasive/rub/wear/wearing resistance (property) 耐磨性93. spectrum analysis光谱分析94. heat/thermal treatment 热处理95. inclusion 夹杂物96. segregation 偏析97. picking 酸洗,酸浸98. residual stress 残余应力99. remaining stress 残余应力100. relaxation of residual stress 消除残余应力101. stress relief 应力释放PLANStep 2:Analyze The ProblemIdentify the processes that impact the problem and select oneList the steps in the process as it currently existsMap the ProcessValidate the map of the processIdentify potential cause of the problemCollect and analyze data related to the problemVerify or revise the original problem statementIdentify root causes of the problemCollect additional data if needed to verify root causesDOStep 3:DevelopSolutionsEstablish criteria for selecting a solutionGenerate potential solutions that will address the root causes of the problem Select a solutionGain approval and supporter the chosen solutionPlan the solutionStep 4:Implement a SolutionImplement the chosen solution on a trial or pilot basisIf the Problem Solving Process is being used in conjunction with the Continuous Improvement Process, return to Step 6 of the Continuous Improvement ProcessIf the Problem Solving Process is being used as a standalone, continue to Step 5CHECKStep 5:Evaluate The ResultsGather data on the solutionAnalyze the data on the solutionAchieved the Desired Goal? If YES, go to Step 6.If NO, go back to Step 1.ACTStep 6:Standardize The Solution (and Capitalize on New Opportunities)Identify systemic changes and training needs for full implementationAdopt the solutionPlan ongoing monitoring of the solutionContinue to look for incremental improvements to refine the solution转贴:FPC专业术语中英对照FPC专业术语中英对照行业术语 2004-10-5 中国PCB技术网DDate Code ——周期代码,用来表明产品生产的时间。
电气控制英文参考文献(精选120个最新)

改革开放以来,随着我国工业的迅速发展和科学技术的进步,电气控制技术在工业上的运用也越来越广泛,对于一个国家的科技水平高低来说,电气控制技术水平是一项重要的衡量因素.电气控制技术主要以电动机作为注重的对象,通过一系列的电气控制技术,买现生产或者监控的自动化.下面是搜索整理的电气控制英文参考文献,欢迎借鉴参考。
电气控制英文参考文献一: [1]Laiqing Xie,Yugong Luo,Donghao Zhang,Rui Chen,Keqiang Li. Intelligent energy-saving control strategy for electric vehicle based on preceding vehicle movement[J]. Mechanical Systems andSignal Processing,2019,130. [2]F.N. Tan,Q.Y. Wong,W.L. Gan,S.H. Li,H.X. Liu,F. Poh,W.S. Lew. Electric field control for energy efficient domain wallinjection[J]. Journal of Magnetism and Magnetic Materials,2019,485. [3]N. Nursultanov,W.J.B. Heffernan,M.J.W.M.R. van Herel,J.J. Nijdam. Computational calculation of temperature and electrical resistance to control Joule heating of green Pinus radiata logs[J]. Applied Thermal Engineering,2019,159. [4]Min Cheng,Junhui Zhang,Bing Xu,Ruqi Ding,Geng Yang. Anti-windup scheme of the electronic load sensing pump via switchedflow/power control[J]. Mechatronics,2019,61. [5]Miles L. Morgan,Dan J. Curtis,Davide Deganello. Control of morphological and electrical properties of flexographic printed electronics through tailored ink rheology[J]. OrganicElectronics,2019,73. [6]Maciej ?awryńczuk,Pawe?Oc?oń. Model Predictive Control and energy optimisation in residential building with electric underfloor heating system[J]. Energy,2019,182. [7]Lorenzo Niccolai,Alessandro Anderlini,GiovanniMengali,Alessandro A. Quarta. Electric sail displaced orbit control with solar wind uncertainties[J]. Acta Astronautica,2019,162. [8]Patrik Beňo,Matej Kubi?. Control and stabilization of single-wheeled electric vehicle with BLDC engine[J]. Transportation Research Procedia,2019,40. [9]André Murilo,Rafael Rodrigues,Evandro Leonardo SilvaTeixeira,Max Mauro Dias Santos. Design of a Parameterized Model Predictive Control for Electric Power Assisted Steering[J]. Control Engineering Practice,2019,90. [10]Kazusa Yamamoto,Olivier Sename,Damien Koenig,Pascal Moulaire. Design and experimentation of an LPV extended state feedback control on Electric Power Steering systems[J]. Control EngineeringPractice,2019,90. [11]Pedro de A. Delou,Julia P.A. de Azevedo,Dinesh Krishnamoorthy,Maurício B. de Souza,Argimiro R. Secchi. Model Predictive Control with Adaptive Strategy Applied to an Electric Submersible Pump in a Subsea Environment[J]. IFACPapersOnLine,2019,52(1). [12]Unal Yilmaz,Omer Turksoy,Ahmet Teke. Intelligent control of high energy efficient two-stage battery charger topology forelectric vehicles[J]. Energy,2019,186. [13]Qiuyi Guo,Zhiguo Zhao,Peihong Shen,Xiaowen Zhan,Jingwei Li. Adaptive optimal control based on driving style recognition forplug-in hybrid electric vehicle[J]. Energy,2019,186. [14]Leonid Lobanov,Nikolai Pashсhin. Electrodynamic treatment by electric current pulses as effective method of control of stress-strain states and improvement of life of welded structures[J]. Procedia Structural Integrity,2019,16. [15]Evangelos Pournaras,Seoho Jung,Srivatsan Yadhunathan,Huiting Zhang,Xingliang Fang. Socio-technical smart grid optimization via decentralized charge control of electric vehicles[J]. Applied Soft Computing Journal,2019,82. [16]Guoming Huang,Xiaofang Yuan,Ke Shi,Xiru Wu. A BP-PID controller-based multi-model control system for lateral stability of distributed drive electric vehicle[J]. Journal of the Franklin Institute,2019,356(13). [17]Ioannis Kalogeropoulos,Haralambos Sarimveis. Predictive control algorithms for congestion management in electric power distribution grids[J]. Applied Mathematical Modelling,2020,77. [18]Junjun Zhu,Zhenpo Wang,Lei Zhang,David G. Dorrell.Braking/steering coordination control for in-wheel motor drive electric vehicles based on nonlinear model predictive control[J]. Mechanism and Machine Theory,2019,142. [19]Jiechen Wu,Junjie Hu,Xin Ai,Zhan Zhang,Huanyu Hu. Multi-time scale energy management of electric vehicle model-based prosumers by using virtual battery model[J]. Applied Energy,2019,251. [20]G. Coorey,D. Peiris,T. Usherwood,L. Neubeck,J. Mulley,J. Redfern. An Internet-Based Intervention Integrated with the Primary Care Electronic Health Record to Improve Cardiovascular Disease Risk Factor Control: a Mixed-Methods Evaluation of Acceptability, Usage Trends and Persuasive Design Characteristics[J]. Heart, Lung and Circulation,2019,28. [21]Félice Lê-Scherban,Lance Ballester,Juan C. Castro,Suzanne Cohen,Steven Melly,Kari Moore,James W. Buehler. Identifying neighborhood characteristics associated with diabetes and hypertension control in an urban African-American population usinggeo-linked electronic health records[J]. Preventive Medicine Reports,2019,15. [22]Yuekuan Zhou,Sunliang Cao. Energy flexibility investigation of advanced grid-responsive energy control strategies with thestatic battery and electric vehicles: A case study of a high-rise office building in Hong Kong[J]. Energy Conversion and Management,2019,199. [23]D. Aravindh,R. Sakthivel,B. Kaviarasan,S. MarshalAnthoni,Faris Alzahrani. Design of observer-based non-fragile load frequency control for power systems with electric vehicles[J]. ISA Transactions,2019,91. [24]Augusto Matheus dos Santos Alonso,Danilo IglesiasBrandao,Tommaso Caldognetto,Fernando Pinhabel Maraf?o,Paolo Mattavelli. A selective harmonic compensation and power control approach exploiting distributed electronic converters inmicrogrids[J]. International Journal of Electrical Power and Energy Systems,2020,115. [25]Hay Wong,Derek Neary,Eric Jones,Peter Fox,Chris Sutcliffe. Benchmarking spatial resolution in electronic imaging for potential in-situ Electron Beam Melting monitoring[J]. Additive Manufacturing,2019,29. [26]Yunfei Bai,Hongwen He,Jianwei Li,Shuangqi Li,Ya-xiong Wang,Qingqing Yang. Battery anti-aging control for a plug-in hybrid electric vehicle with a hierarchical optimization energy management strategy[J]. Journal of Cleaner Production,2019,237. [27]N. Samartin-Veiga,A.J. González-Villar,M.T. Carrillo-de-la-Pe?a. Neural correlates of cognitive dysfunction in fibromyalgia patients: Reduced brain electrical activity during the execution ofa cognitive control task[J]. NeuroImage: Clinical,2019,23. [28]Masato Nakaya,Shinta Watanabe,Jun Onoe. Control of electric, optical, thermal properties of C 60 films by electron-beam irradiation[J]. Carbon,2019,152. [29]R. Saadi,M.Y. Hammoudi,O. Kraa,M.Y. Ayad,M. Bahri. A robust control of a 4-leg floating interleaved boost converter for fuel cell electric vehicle application[J]. Mathematics and Computers in Simulation,2019. [30]Frederik Banis,Daniela Guericke,Henrik Madsen,Niels Kj?lstad Poulsen. Supporting power balance in Microgrids with Uncertain Production using Electric Vehicles and Indirect Control ? ? This work has been supported by ENERGINET.DK under the project microgrid positioning - uGrip and the CITIES project.[J]. IFAC PapersOnLine,2019,52(4). 电气控制英文参考文献二: [31]Huijuan Luo,Jinpeng Yu,Chong Lin,Zhanjie Liu,Lin Zhao,Yumei Ma. Finite-time dynamic surface control for induction motors with input saturation in electric vehicle drive systems[J]. Neurocomputing,2019. [32]Peter K. Joseph,D. Elangovan,G. Arunkumar. Linear control of wireless charging for electric bicycles[J]. Applied Energy,2019,255. [33]Yu Congyang,Zhu Dequan,Wang Chaoxian,Zhu Lin,Chu Tingting,Jen Tien-Chien,Liao Juan. Optimizing Electric Adjustment Mechanism Using the Combination of Multi-body Dynamics and Control[J]. Procedia Manufacturing,2019,35. [34]Hussein Termous,Xavier Moreau,Clovis Francis,Hassan Shraim. Effect of fractional order damping control on braking performancefor electric vehicles ? ? This work was supported by the Lebanese research program and the AUF-CNRSL-UL program.[J]. IFAC PapersOnLine,2019,52(5). [35]Manuel Schwartz,Florian Siebenrock,S?ren Hohmann. Model Predictive Control Allocation of an Over-actuated Electric Vehicle with Single Wheel Actuators[J]. IFAC PapersOnLine,2019,52(8). [36]Di Wu,Nikitha Radhakrishnan,Sen Huang. A hierarchical charging control of plug-in electric vehicles with simpleflexibility model[J]. Applied Energy,2019,253. [37]Abhishek Nayak,Rubi Rana,Sukumar Mishra. Frequency Regulation by Electric Vehicle during Grid Restoration using Adaptive Optimal Control[J]. IFAC PapersOnLine,2019,52(4). [38]Nicolò Robuschi,Mauro Salazar,Pol Duhr,FrancescoBraghin,Christopher H. Onder. Minimum-fuel Engine On/Off Control for the Energy Management of a Hybrid Electric Vehicle via Iterative Linear Programming ? ? We thank Ferrari S.p.A. for supporting this project.[J]. IFAC PapersOnLine,2019,52(5). [39]Anas A. Ahmed,M.R. Hashim,Marzaini Rashid. Control of the structural, electrical and optical properties of spin coated NiO films by varying precursor molarity[J]. Thin Solid Films,2019,690. [40]Wilco van Harselaar,Niels Schreuders,Theo Hofman,Stephan Rinderknecht. Improved Implementation of Dynamic Programming on the Example of Hybrid Electric Vehicle Control[J]. IFACPapersOnLine,2019,52(5). [41]Jose A. Matute,Mauricio Marcano,Sergio Diaz,Joshue Perez. Experimental Validation of a Kinematic Bicycle Model Predictive Control with Lateral Acceleration Consideration ? ? This project has received funding from the Electronic Component Systems for European Leadership Joint Undertaking under grant agreement No 737469 (AutoDrive Project). This Joint Undertaking receives support fromthe European Union Horizon 2020 research and innovation programmeand Germany, Austria, Spain, Italy, Latvia, Belgium, Netherlands, Sweden, Finland, Lithuania, Czech Republic, Romania,[J]. IFAC PapersOnLine,2019,52(8). [42]Vladislav S. Gromov,Oleg I. Borisov,Sergey S. Shavetov,AntonA. Pyrkin,FatimatB. Karashaeva. Modeling and Control of Robotic Systems Course: from Fundamentals to Applications ? ? The work was written with the support of the Ministry of Science and Higher Education of the Russian Federation, project unique identifier RFMEFI57818X0271 “Adaptive Sensorless Control for Synchronous Electric Drives in Intelligent Robotics and Transport Systems”.[J]. IFAC PapersOnLine,2019,52(9). [43]H. Mbarak,A.K. Kodeary,S.M. Hamidi,E. Mohajarani,Y. Zaatar. Control of nonlinear refractive index of AuNPs doped with nematic liquid crystal under external electric field[J]. Optik,2019,198. [44]Yanzhao Jia,Rabee Jibrin,Yutaro Itoh,Daniel G?rges. Energy-Optimal Adaptive Cruise Control for Electric Vehicles in Both Time and Space Domain based on Model Predictive Control[J]. IFAC PapersOnLine,2019,52(5). [45]Lukas Engbroks,Daniel G?rke,Stefan Schmiedler,TobiasG?decke,Bastian Beyfuss,Bernhard Geringer. Combined energy and thermal management for plug-in hybrid electric vehicles -analyses based on optimal control theory ? ? This work has been performed within the Daimler AG in Stuttgart, Germany in cooperation with the Institute for Powertrains and Automotive Technology at Vienna University of Technology, Austria.[J]. IFAC PapersOnLine,2019,52(5). [46]Jean Kuchly,Dominique Nelson-Gruel,Alain Charlet,Yann Chamaillard,Cédric Nouillant. Projected Gradient and ModelPredictive Control : Optimal Energy and Pollutants Management for Hybrid Electric Vehicle[J]. IFAC PapersOnLine,2019,52(5). [47]Pier Giuseppe Anselma,Yi Huo,Joel Roeleveld,Giovanni Belingardi,Ali Emadi. From Off-line to On-line Control of a Multimode Power Split Hybrid Electric Vehicle Powertrain[J]. IFAC PapersOnLine,2019,52(5). [48]Xiaoyong Zhu,Deyang Fan,Zixuan Xiang,Li Quan,Wei Hua,Ming Cheng. Systematic multi-level optimization design and dynamiccontrol of less-rare-earth hybrid permanent magnet motor for all-climatic electric vehicles[J]. Applied Energy,2019,253. [49]. Engineering - Industrial Engineering; Findings from Southwest Jiaotong University Provides New Data about Industrial Engineering (Optimal Energy Management and Control In Multimode Equivalent Energy Consumption of Fuel Cell/supercapacitor of Hybrid Electric Tram)[J]. Energy Weekly News,2019. [50]. SK Planet Co. Ltd.; Patent Issued for Electronic Stamp System For Security Intensification, Control Method Thereof, And Non-Transitory Computer Readable Storage Medium Having ComputerProgram Recorded Thereon (USPTO 10,361,857)[J]. Computers, Networks & Communications,2019. [51]. Energy - Electric Power; Study Data from National Institute of Technology Calicut Update Understanding of Electric Power (Modified switching scheme-based explicit torque control of brush-less direct current motor drive)[J]. Energy Weekly News,2019. [52]. Energy; Findings from School of Mechanical Engineering Reveals New Findings on Energy (Deep Reinforcement Learning of Energy Management With Continuous Control Strategy and Traffic Information for a Series-parallel Plug-in Hybrid Electric Bus)[J]. Energy Weekly News,2019. [53]. Energy - Electric Power; Reports Outline Electric Power Study Results from Dalian Maritime University (Direct VoltageControl of Stand-alone Dfig Under Asymmetric Loads Based On Non-singular Terminal Sliding Mode Control and Improved Extended State Observer)[J]. Energy Weekly News,2019. [54]. Energy - Electric Power; Studies from Xi'an Jiao Tong University Add New Findings in the Area of Electric Power (A model predictive control approach for matching uncertain wind generation with PEV charging demand in a microgrid)[J]. Energy WeeklyNews,2019. [55]. Energy - Electric Power; Researchers from Northwestern Polytechnical University Discuss Findings in Electric Power (Decoupling Start Control Method for Aircraft Wound-rotor Synchronous Starter-generator Based On Main Field Current Estimation)[J]. Energy Weekly News,2019. [56]. Energy - Electric Power; Wuhan University Reports Findings in Electric Power (Adjustable virtual inertia control of supercapacitors in PV-based AC microgrid cluster)[J]. Energy Weekly News,2019. [57]. Lg Electronic Inc.; Researchers Submit Patent Application, "Method And Apparatus For Monitoring Control Channel In Unlicensed Band", for Approval (USPTO 20190229825)[J]. Computers, Networks & Communications,2019. [58]. Special Conditions: Pilatus Aircraft Ltd., Model PC-12/47E Airplanes; Electronic Engine Control System Installation[J]. The Federal Register / FIND,2019,84(158). [59]. Apple Inc.; Patent Issued for Offset Control For Assembling An Electronic Device Housing (USPTO 10,368,457)[J]. Computers, Networks & Communications,2019. [60]. Mitsubishi Electric Corporation; Researchers Submit Patent Application, "Synchronization Control System And Control Device",for Approval (USPTO 20190238071)[J]. Computers, Networks & Communications,2019. 电气控制英文参考文献三: [61]. Technology - Cybernetics; Findings from North ChinaElectric Power University Provides New Data about Cybernetics (Hierarchical Distributed Model Predictive Control of Standalone Wind/solar/battery Power System)[J]. Energy Weekly News,2019. [62]. Nidec Corporation; "Motor Control System And Electric Power Steering System" in Patent Application Approval Process (USPTO 20190233002)[J]. Energy Weekly News,2019. [63]. Mobvoi Information Technology Co. LTD.; Researchers Submit Patent Application, "Display Device, Electronic Device And Display Control Method For Screen", for Approval (USPTO 20190235540)[J]. Computers, Networks & Communications,2019. [64]. Engineering - Power Delivery; Studies from North China Electric Power University Have Provided New Data on Power Delivery (Fault Tripping Criteria In Stability Control Device Adapting ToHalf-wavelength Ac Transmission Line)[J]. Energy Weekly News,2019. [65]. Samsung Electronics Co. Ltd.; "Electronic Device For Sensing Biometric Information And Control Method Thereof" in Patent Application Approval Process (USPTO 20190231235)[J]. Medical Patent Business Week,2019. [66]Asiabar Aria Noori,Kazemi Reza. A direct yaw momentcontroller for a four in-wheel motor drive electric vehicle using adaptive sliding mode control[J]. Proceedings of the Institution of Mechanical Engineers,2019,233(3). [67]. Energy - Electrical Energy Systems; New Electrical Energy Systems Findings Has Been Reported by Investigators at University of Sfax (Constrained design and control of trapezoidal waves-forms hybrid excitation synchronous motor increasing energy accumulator lifetime)[J]. Energy Weekly News,2019. [68]. Energy; Findings from School of Mechanical Engineering Has Provided New Data on Energy (Considering Well-to-Wheels Analysis in Control Design: Regenerative Suspension Helps to Reduce Greenhouse Gas Emissions from Battery Electric Vehicles)[J]. Energy Weekly News,2019. [69]. Mitsubishi Electric Corporation; Patent Application Titled "Electric-Power Control Device, Electric Motor, Air-Conditioning Apparatus, And Method For Manufacturing Electric Motor" Published Online (USPTO 20190242594)[J]. Energy Weekly News,2019. [70]. Energy; Reports Summarize Energy Study Results from Warsaw University of Technology (Model Predictive Control and energy optimisation in residential building with electric underfloorheating system)[J]. Energy Weekly News,2019. [71]. Energy - Nuclear Power; Researchers from Korea Electric Power Corporation Report New Studies and Findings in the Area of Nuclear Power (Development of Anti-windup Pi Control and Bumpless Control Transfer Methodology for Feedwater Control System)[J]. Energy Weekly News,2019. [72]. Energy - Electric Power; Data on Electric Power Discussed by Researchers at School of Electrical and Electronics Engineering (Analysis of the Performance Characteristics and Arm Current Control for Modular Multilevel Converter With Asymmetric Arm Parameters)[J]. Energy Weekly News,2019. [73]. Energy - Electric Power; Study Findings on Electric Power Are Outlined in Reports from University of Technology (Direct power control for VSC-HVDC systems: An application of the global tracking passivity-based PI approach)[J]. Energy Weekly News,2019. [74]Allous Manel,Mrabet Kais,Zanzouri Nadia. Fast fault-tolerant control of electric power steering systems in the presence of actuator fault[J]. Proceedings of the Institution of Mechanical Engineers,2019,233(12). [75]. Energy - Electric Power; Researchers from College of Engineering Detail New Studies and Findings in the Area of Electric Power (Power Control Strategy of Photovoltaic Plants for Frequency Regulation In a Hybrid Power System)[J]. Energy Weekly News,2019. [76]. Energy - Electric Power; Researchers at Shiv Nadar University Report New Data on Electric Power (Methods for overcoming misalignment effects and charging control of a dynamic wireless electric vehicle charging system)[J]. Energy Weekly News,2019. [77]Zhang Bing,Zong Changfu,Chen Guoying,Li Guiyuan. An adaptive-prediction-horizon model prediction control for path tracking in a four-wheel independent control electric vehicle[J]. Proceedings of the Institution of Mechanical Engineers,2019,233(12). [78]Ren Yue,Zheng Ling,Yang Wei,Li Yinong. Potential field–based hierarchical adaptive cruise control for semi-autonomous electric vehicle[J]. Proceedings of the Institution of MechanicalEngineers,2019,233(10). [79]. Energy - Electric Power; Data from University of the Basque Country Advance Knowledge in Electric Power (Sliding Mode Control of an Active Power Filter With Photovoltaic Maximum Power Tracking)[J]. Energy Weekly News,2019. [80]Izadbakhsh Alireza,Kheirkhahan Payam. Adaptive fractional-order control of electrical flexible-joint robots: Theory and experiment[J]. Proceedings of the Institution of Mechanical Engineers,2019,233(9). [81]Yang Weiwei,Liang Jiejunyi,Yang Jue,Zhang Nong. Optimal control of a novel uninterrupted multi-speed transmission for hybrid electric mining trucks[J]. Proceedings of the Institution of Mechanical Engineers,2019,233(12). [82]Guercioni Guido Ricardo,Vigliani Alessandro. Gearshiftcontrol strategies for hybrid electric vehicles: A comparison of powertrains equipped with automated manual transmissions and dual-clutch transmissions[J]. Proceedings of the Institution of Mechanical Engineers,2019,233(11). [83]. Energy - Electric Power; Findings from PontificalUniversity Provides New Data on Electric Power (A Communication-free Reactive-power Control Strategy In Vsc-hvdc Multi-terminal Systems To Improve Transient Stability)[J]. Energy Weekly News,2019. [84]. Energy - Electric Power; Findings from Yazd University in the Area of Electric Power Reported (An adaptive time-graded control method for VSC-MTDC networks)[J]. Energy Weekly News,2019. [85]Liu Hui,Li Xunming,Wang Weida,Han Lijin,Xin Huibin,Xiang Changle. Adaptive equivalent consumption minimisation strategy and dynamic control allocation-based optimal power management strategy for four-wheel drive hybrid electric vehicles[J]. Proceedings of the Institution of Mechanical Engineers,2019,233(12). [86]. Networks - Neural Networks; Findings on Neural Networks Reported by Investigators at School of Electrical Engineering and Automation (Stability Analysis of Fractional Order Hopfield Neural Networks With Optimal Discontinuous Control)[J]. Computers, Networks & Communications,2019. [87]. Energy - Electric Power; Researchers from NanjingUniversity of Aeronautics and Astronautics Describe Findings in Electric Power (Synchronous Vibration Control for a Class of Cross-coupled Antisymmetric Msr Systems)[J]. Energy Weekly News,2019. [88]. Energy - Electric Power; Investigators at Chung Ang University Detail Findings in Electric Power (Flexible Risk Control Strategy Based On Multi-stage Corrective Action With Energy Storage System)[J]. Energy Weekly News,2019. [89]. Energy - Electric Power; Findings in Electric Power Reported from National Institute of Technology (An adaptive PI control scheme to balance the neutral-point voltage in a solar PV fed grid connected neutral point clamped inverter)[J]. Energy Weekly News,2019. [90]Najjari Behrouz,Mirzaei Mehdi,Tahouni Amin. Constrained stability control with optimal power management strategy for in-wheel electric vehicles[J]. Proceedings of the Institution of Mechanical Engineers,2019,233(4). 电气控制英文参考文献四: [91]. Energy - Wind Farms; Investigators at School of Electrical Power Detail Findings in Wind Farms (Theoretical Study On Control Strategy of Grid-connected High Voltage Ride Through In Doubly-fed Wind Farm)[J]. Energy Weekly News,2019. [92]. Kia Motors Corporation; Patent Issued for Wireless Charging Control Apparatus And Method For Optimal Charging By Adjusting The Inclination Of The Electric Vehicle Being Charged (USPTO10,399,449)[J]. Computers, Networks & Communications,2019. [93]. Energy; New Data from Institute of Electrical Engineering Illuminate Findings in Energy (Charging-Discharging Control Strategy for a Flywheel Array Energy Storage System Based on the Equal Incremental Principle)[J]. Energy Weekly News,2019. [94]. Science - Applied Sciences; Findings from North China Electric Power University Broaden Understanding of Applied Sciences (Coordinated Frequency Control Strategy with the Virtual Battery Model of Inverter Air Conditionings)[J]. Science Letter,2019. [95]. Science - Materials Science; Studies from Tsinghua University in the Area of Materials Science Described (ElectricField Control of Neel Spin-orbit Torque In an Antiferromagnet)[J]. Science Letter,2019. [96]. Electronics - Power Electronics; Studies from Nanjing University of Aeronautics and Astronautics Have Provided New Data on Power Electronics (Wireless battery charging control for electric vehicles: a user-involved approach)[J]. Computers, Networks & Communications,2019. [97]Kivanc,Ustun. Dynamic control of electronic differential in the field weakening region[J]. International Journal ofElectronics,2019,106(10). [98]Mohit Batra,John McPhee,Nasser L. Azad. Real-time model predictive control of connected electric vehicles[J]. Vehicle System Dynamics,2019,57(11). [99]Kim Daihyun,Echelmeier Austin,Cruz Villarreal Jorvani,Gandhi Sahir,Quintana Sebastian,Egatz-Gomez Ana,Ros Alexandra. Electric Triggering for Enhanced Control of Droplet Generation.[J].Analytical chemistry,2019,91(15). [100]Kurien Caneon,Srivastava Ajay Kumar. Impact of Electric Vehicles on Indirect Carbon Emissions and Role of Engine Post-Treatment Emission Control Strategies.[J]. Integrated environmental assessment and management,2019. [101]Aravindh D,Sakthivel R,Kaviarasan B,Anthoni SMarshal,Alzahrani Faris. Design of observer-based non-fragile loadfrequency control for power systems with electric vehicles.[J]. ISA transactions,2019,91. [102]Chen Xianzhe,Zhou Xiaofeng,Cheng Ran,Song Cheng,Zhang Jia,Wu Yichuan,Ba You,Li Haobo,Sun Yiming,You Yunfeng,Zhao Yonggang,Pan Feng. Electric field control of Néel spin-orbit torque in an antiferromagnet.[J]. Nature materials,2019,18(9). [103]Lê-Scherban Félice,Ballester Lance,Castro Juan C,Cohen Suzanne,Melly Steven,Moore Kari,Buehler James W. Identifying neighborhood characteristics associated with diabetes and hypertension control in an urban African-American population using geo-linked electronic health records.[J]. Preventive medicine reports,2019,15. [104]Samartin-Veiga N,González-Villar A J,Carrillo-de-la-Pe?a M T. Neural correlates of cognitive dysfunction in fibromyalgia patients: Reduced brain electrical activity during the execution of a cognitive control task.[J]. NeuroImage. Clinical,2019,23. [105]Leibel Sydney,Weber Rachel. Utilizing a PhysicianNotification System in the EPIC Electronic Medical Record to Improve Pediatric Asthma Control: A Quality Improvement Project.[J].Clinical pediatrics,2019,58(11-12). [106]Bernacka-Wojcik Iwona,Huerta Miriam,Tybrandt Klas,Karady Michal,Mulla Mohammad Yusuf,Poxson David J,Gabrielsson Erik O,Ljung Karin,Simon Daniel T,Berggren Magnus,Stavrinidou Eleni. Implantable Organic Electronic Ion Pump Enables ABA Hormone Delivery for Control of Stomata in an Intact Tobacco Plant.[J]. Small (Weinheim an der Bergstrasse, Germany),2019. [107]Stoynova Nevena,Laske Christoph,Plewnia Christian. Combining electrical stimulation and cognitive control training to reduce concerns about subjective cognitive decline.[J]. Brainstimulation,2019,12(4). [108]Bettano Amy,Land Thomas,Byrd Alice,Svencer Susan,Nasuti Laura. Using Electronic Referrals to Address Health Disparities and Improve Blood Pressure Control.[J]. Preventing chronicdisease,2019,16. [109]Xu Meng,Yan Jian-Min,Guo Lei,Wang Hui,Xu Zhi-Xue,Yan Ming-Yuan,Lu Yun-Long,Gao Guan-Yin,Li Xiao-Guang,Luo Hao-Su,ChaiYang,Zheng Ren-Kui. Nonvolatile Control of the Electronic Properties of In<sub>2- x </sub>Cr<sub> x </sub>O<sub>3</sub> Semiconductor Films by Ferroelectric Polarization Charge.[J]. ACS appliedmaterials & interfaces,2019,11(35). [110]Gao Tao,Mirzadeh Mohammad,Bai Peng,Conforti Kameron M,Bazant Martin Z. Active control of viscous fingering using electricfields.[J]. Nature communications,2019,10(1). [111]Chaux Robin,Treussier Isabelle,Audeh Bissan,Pereira Suzanne,Hengoat Thierry,Paviot Béatrice Trombert,Bousquet Cedric. Automated Control of Codes Accuracy in Case-Mix Databases by Evaluating Coherence with Available Information in the Electronic Health Record.[J]. Studies in health technology andinformatics,2019,264. [112]Bolat Mustafa Suat,Cinar Onder,Asci Ramazan,Buyukalpelli Recep. A novel method for pain control: infiltration free local anesthesia technique (INFLATE) for transrectal prostatic biopsy using transcutaneous electrical nerve stimulation (TENS).[J]. International urology and nephrology,2019. [113]Cruz Chad D,Yuan Jennifer,Climent Clàudia,Tierce NathanT,Christensen Peter R,Chronister Eric L,Casanova David,Wolf Michael O,Bardeen Christopher J. Using sulfur bridge oxidation to control electronic coupling and photochemistry in covalent anthracene dimers.[J]. Chemical science,2019,10(32). [114]Zhou Canliang,Sun Linfeng,Zhang Fengquan,Gu Chenjie,Zeng Shuwen,Jiang Tao,Shen Xiang,Ang Diing Shenp,Zhou Jun. Electrical Tuning of the SERS Enhancement by Precise Defect DensityControl.[J]. ACS applied materials & interfaces,2019,11(37). [115]Taeho Park,Hyeongcheol Lee. Optimal Supervisory Control Strategy for a Transmission-Mounted Electric Drive Hybrid Electric Vehicle[J]. International Journal of AutomotiveTechnology,2019,20(4). [116]Zoé Magalh?es,André Murilo,Renato V. Lopes. Development and evaluation with MIL and HIL simulations of a LQR-based upper-level electronic stability control[J]. Journal of the Brazilian Society of Mechanical Sciences and Engineering,2019,41(8). [117]Justin Roger Mboupda Pone,Victor Kamdoum Tamba,Guillaume Honore Kom,Mathieu Jean Pierre Pesdjock,Alain Tiedeu,Martin Kom. Numerical, electronic simulations and experimental analysis of a no-equilibrium point chaotic circuit with offset boosting and partial amplitude control[J]. SN Applied Sciences,2019,1(8). [118]Alberto Cavallo,Antonio Russo,Giacomo Canciello.Hierarchical control for generator and battery in the more electric aircraft[J]. Science China Information Sciences,2019,62(9). [119]Ying Liu,Kai Cao,Jingjun Liu,Zhengping Zhang,Jing Ji,Feng Wang,Zhilin Li. Electrodeposition of copper-doped SnS thin films and their electric transmission properties control for thermoelectric enhancement[J]. Journal of Materials Science: Materials in Electronics,2019,30(17). [120]Feng Tian,Liqi Sui,Yuanfan Zeng,Bo Li,Xingyue Zhou,Lijun Wang,Hongxu Chen. Hardware Design and Test of a Gear-ShiftingControl System of a Multi-gear Transmission for ElectricVehicles[J]. Automotive Innovation,2019,2(3).。
输入功率反馈控制输出功率不稳定的解决方法

输入功率反馈控制输出功率不稳定的解决方法In the field of power electronics, feedback control is often employed to regulate the output power of a device. However, in certain cases, such as when dealing with non-linear loads or varying source voltages, the output power can become unstable. In this write-up, we will explore some effective methods to address the instability issue associated with feedback control of input power to output power.在电力电子领域,通常使用反馈控制来调节设备的输出功率。
在某些情况下,如处理非线性负载或变化的电源电压时,输出功率可能会变得不稳定。
在本文中,我们将探讨一些有效的方法来解决反馈控制输入功率到输出功率的不稳定性问题。
One approach to mitigate unstable output power is the use of compensators. Compensators are designed to modify the response characteristics of a system and improve its stability. Various types of compensators can be utilized, such as proportional-integral-derivative (PID) controllersor lead-lag compensators. These compensators provide additional gain and phase shift in specific frequency ranges, which helps stabilize the system's response.缓解输出功率不稳定的一种方法是使用补偿器(compensators)。
Power Electronics and Drives

Power Electronics and Drives Power electronics and drives play a crucial role in modern technology, impacting various aspects of our daily lives. From electric vehicles to renewable energy systems, power electronics and drives are essential for efficient energy conversion and control. In this article, we will explore the significance of power electronics and drives, their applications, challenges, and future prospects. First and foremost, it's important to understand the significance of power electronics and drives in today's world. These technologies are the backbone of electric vehicles, renewable energy systems, industrial automation, and consumer electronics. They enable the efficient conversion of electrical power, control the speed and torque of electric motors, and provide a reliable and stable power supply for various applications. Without power electronics and drives, the transition towards sustainable energy and the advancement of electric transportation would not be possible. One of the key applications of power electronics and drives is in electric vehicles (EVs). With the global push towards reducing carbon emissions and combating climate change, EVs have gainedsignificant attention as a cleaner and more sustainable mode of transportation. Power electronics and drives are essential components in EVs, controlling the power flow from the battery to the electric motor, managing the vehicle's energy efficiency, and providing a smooth and responsive driving experience. As the demand for EVs continues to rise, the role of power electronics and drives in enabling this transition becomes increasingly vital. In addition to EVs, power electronics and drives are also integral to renewable energy systems. With the growing adoption of solar, wind, and other forms of renewable energy, the need for efficient power conversion and grid integration has become paramount. Power electronics enable the conversion of DC power generated by solar panels or wind turbines into AC power suitable for the grid. Moreover, they facilitate grid stabilization, voltage control, and seamless integration of renewable energy sources, thereby contributing to a more sustainable and resilient power infrastructure. Despite their numerous benefits and applications, power electronics and drives also face several challenges. One of the primary challenges is the need for higher efficiency and reliability. As the demand for energy-efficient technologies continues to grow, there is a constant pressure to improve the efficiency of power electronic converters and motor drives. This requires advancements in semiconductor materials, converter topologies, and control algorithms to minimize power losses and enhance overall system performance.Another challenge is the integration of power electronics and drives with smartgrid technologies. With the increasing digitalization and decentralization ofpower systems, there is a need for power electronic devices and drives to communicate and coordinate with grid management systems. This integration presents technical and regulatory challenges, as it requires standardization, cybersecurity measures, and interoperability among different grid components. Looking ahead,the future of power electronics and drives holds great promise. Advancements inwide-bandgap semiconductors such as silicon carbide (SiC) and gallium nitride (GaN) are poised to revolutionize power electronics by enabling higher efficiency,higher temperature operation, and greater power density. These materials have the potential to drive the next generation of power electronic devices and motor drives, paving the way for more compact, lightweight, and energy-efficient systems. Furthermore, the advent of digital control and advanced algorithms, such as model predictive control and artificial intelligence, is expected to enhance the performance and flexibility of power electronics and drives. These technologies enable predictive maintenance, adaptive control, and real-time optimization,thereby improving the reliability and responsiveness of power electronic systemsin diverse operating conditions. In conclusion, power electronics and drives are indispensable technologies that underpin the modern energy landscape and drive the transition towards sustainable and efficient power systems. From electric vehicles to renewable energy integration, these technologies continue to shape the way we generate, convert, and utilize electrical energy. While they face challenges in terms of efficiency, integration, and performance, ongoing research and innovation hold the key to unlocking their full potential and ushering in a new era of power electronics and drives.。
A Model Predictive Control System for a Hybrid
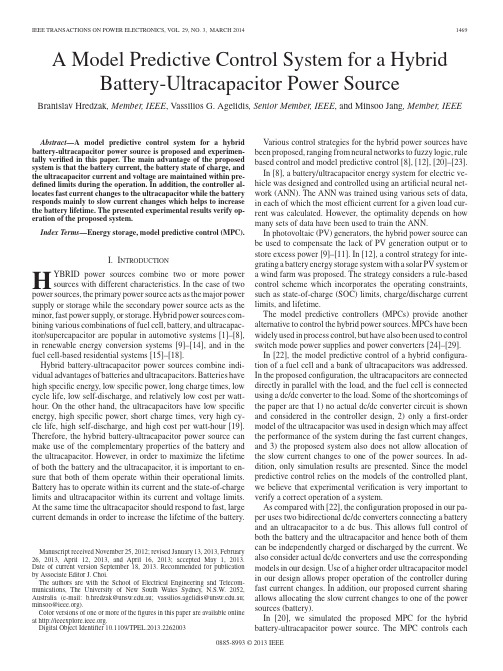
A Model Predictive Control System for a HybridBattery-Ultracapacitor Power SourceBranislav Hredzak,Member,IEEE,Vassilios G.Agelidis,Senior Member,IEEE,and Minsoo Jang,Member,IEEEAbstract—A model predictive control system for a hybrid battery-ultracapacitor power source is proposed and experimen-tally verified in this paper.The main advantage of the proposed system is that the battery current,the battery state of charge,and the ultracapacitor current and voltage are maintained within pre-defined limits during the operation.In addition,the controller al-locates fast current changes to the ultracapacitor while the battery responds mainly to slow current changes which helps to increase the battery lifetime.The presented experimental results verify op-eration of the proposed system.Index Terms—Energy storage,model predictive control(MPC).I.I NTRODUCTIONH YBRID power sources combine two or more powersources with different characteristics.In the case of two power sources,the primary power source acts as the major power supply or storage while the secondary power source acts as the minor,fast power supply,or storage.Hybrid power sources com-bining various combinations of fuel cell,battery,and ultracapac-itor/supercapacitor are popular in automotive systems[1]–[8], in renewable energy conversion systems[9]–[14],and in the fuel cell-based residential systems[15]–[18].Hybrid battery-ultracapacitor power sources combine indi-vidual advantages of batteries and ultracapacitors.Batteries have high specific energy,low specific power,long charge times,low cycle life,low self-discharge,and relatively low cost per watt-hour.On the other hand,the ultracapacitors have low specific energy,high specific power,short charge times,very high cy-cle life,high self-discharge,and high cost per watt-hour[19]. Therefore,the hybrid battery-ultracapacitor power source can make use of the complementary properties of the battery and the ultracapacitor.However,in order to maximize the lifetime of both the battery and the ultracapacitor,it is important to en-sure that both of them operate within their operational limits. Battery has to operate within its current and the state-of-charge limits and ultracapacitor within its current and voltage limits. At the same time the ultracapacitor should respond to fast,large current demands in order to increase the lifetime of the battery.Manuscript received November25,2012;revised January13,2013,February 26,2013,April12,2013,and April16,2013;accepted May1,2013. Date of current version September18,2013.Recommended for publication by Associate Editor J.Choi.The authors are with the School of Electrical Engineering and Telecom-munications,The University of New South Wales Sydney,N.S.W.2052, Australia(e-mail: b.hredzak@.au;vassilios.agelidis@.au; minsoo@).Color versions of one or more of thefigures in this paper are available online at .Digital Object Identifier10.1109/TPEL.2013.2262003Various control strategies for the hybrid power sources have been proposed,ranging from neural networks to fuzzy logic,rule based control and model predictive control[8],[12],[20]–[23]. In[8],a battery/ultracapacitor energy system for electric ve-hicle was designed and controlled using an artificial neural net-work(ANN).The ANN was trained using various sets of data, in each of which the most efficient current for a given load cur-rent was calculated.However,the optimality depends on how many sets of data have been used to train the ANN.In photovoltaic(PV)generators,the hybrid power source can be used to compensate the lack of PV generation output or to store excess power[9]–[11].In[12],a control strategy for inte-grating a battery energy storage system with a solar PV system or a wind farm was proposed.The strategy considers a rule-based control scheme which incorporates the operating constraints, such as state-of-charge(SOC)limits,charge/discharge current limits,and lifetime.The model predictive controllers(MPCs)provide another alternative to control the hybrid power sources.MPCs have been widely used in process control,but have also been used to control switch mode power supplies and power converters[24]–[29]. In[22],the model predictive control of a hybrid configura-tion of a fuel cell and a bank of ultracapacitors was addressed. In the proposed configuration,the ultracapacitors are connected directly in parallel with the load,and the fuel cell is connected using a dc/dc converter to the load.Some of the shortcomings of the paper are that1)no actual dc/dc converter circuit is shown and considered in the controller design,2)only afirst-order model of the ultracapacitor was used in design which may affect the performance of the system during the fast current changes, and3)the proposed system also does not allow allocation of the slow current changes to one of the power sources.In ad-dition,only simulation results are presented.Since the model predictive control relies on the models of the controlled plant, we believe that experimental verification is very important to verify a correct operation of a system.As compared with[22],the configuration proposed in our pa-per uses two bidirectional dc/dc converters connecting a battery and an ultracapacitor to a dc bus.This allows full control of both the battery and the ultracapacitor and hence both of them can be independently charged or discharged by the current.We also consider actual dc/dc converters and use the corresponding models in our e of a higher order ultracapacitor model in our design allows proper operation of the controller during fast current changes.In addition,our proposed current sharing allows allocating the slow current changes to one of the power sources(battery).In[20],we simulated the proposed MPC for the hybrid battery-ultracapacitor power source.The MPC controls each0885-8993©2013IEEEFig.1.Block diagram of the model predictive control system of the hybrid battery-ultracapacitor power source.of the converters while maintaining the modulation indices,the SOC of the battery,the battery current,the ultracapacitor volt-age and current,and the total current within predefined limits. However,no experimental verification of the system operating with model predictive control was documented in[20].In this paper,we experimentally verify and improve the model predictive control system proposed in[20]by:1)adding voltage sensors for the battery and the ultracapacitor,2)using more ad-vanced battery model,and3)augmenting the objective function of the MPC in order to minimize the variations in the ultracapac-itor voltage.The main advantage of the proposed control system is that it provides a uniform approach to design a control system that ensures the operation of the hybrid power source within predefined limits while allocating the fast current changes to the ultracapacitor.The remainder of the paper is organised as follows.Section II provides a general description of the proposed control system and explains the principles of operation of the system.Section III describes the complete state-space model of the hybrid power source.Section IV gives the procedure for the design of the pro-posed MPC.Experimental results and parameters of the hybrid power source and the MPC are given in Section V.Finally,the conclusions of thstudy are summarized in Section VI.II.C ONTROL S YSTEMA.DescriptionFig.1shows a block diagram of the proposed control system for the hybrid battery-ultracapacitor power source.Both the battery and the ultracapacitor are connected to the half-bridge dc/dc converters via inductors(L b,R b)and(L c,R c), where(L,R)are the values for the inductance and the equivalent series resistance of the inductor,respectively.The output total current isfiltered by inductor(L f l,R f l)and capacitor C f l. Each dc/dc converter is driven by a standard PWM generator which generates the switching signals for the upper and the lower switches with a duty cycle d.The input to the PWM generator is a modulation index m defined as m=(1−d).The converters operate in the continuous conduction mode.The reference input to the MPC is the total required current i total req(1)i total req=p total reqV bus(1) where p total req is the total power that is to be supplied(dis-charging)or stored(charging)by the hybrid power source and V bus is the dc bus voltage assumed to be constant.For simplicity, it is assumed that the efficiency of the conversion is ideal,i.e., 100%.Further inputs to the MPC are the total measured current i total,measured battery current i batt,measured ultracapac-itor current i cap,measured battery voltage v batt,measured ultracapacitor voltage v cap and the constraints m batt min,max, m cap min,max,SOC min,max,i batt min,max,v cap min,max, i cap min,max,i total min,max.The constraint SOC min,max sets the minimum and the maximum limits for the SOC of the battery.Similarly,i batt min,max sets the minimum and the maximum limits for the battery current,v cap min,max for the ultracapacitor voltage,i cap min,max for the ultracapacitor current,i total min,max for the total current,m batt min,max for the battery dc/dc converter modulation index,and m cap min,max for the ultracapacitor dc/dc converter modulation index.The outputs from the MPC are the modulation indices m batt,m cap for the battery and the ultracapacitor dc/dc con-verter,respectively.B.Principle of OperationThe MPC predicts the modulation indices m batt and m cap for the battery and the ultracapacitor dc/dc converter,respectively, so that the total current i total matches the required total current i total req subject to specified constraints.Additional requirement on the controller is that the ultracapacitor should respond to the fast current changes while the battery should respond mainly to the slow current changes.HREDZAK et al.:MODEL PREDICTIVE CONTROL SYSTEM FOR A HYBRID BATTERY-ULTRACAPACITOR POWER SOURCE1471Fig.2.(a)Equivalent circuit of the battery.(b)Equivalent circuit of the dc/dc converter.The following section describes the complete model of thehybrid battery-ultracapacitor power source required to design the MPC.The MPC design is given in Section IV .III.M ODEL OF THE H YBRID B ATTERY -U LTRACAPACITORP OWER S OURCE A.Battery and the DC/DC Converter ModelWe use an RC battery model and parameters of an 8Ah sealed valve-regulated lead-acid battery cell by Hawker given and experimentally verified in [30].The equivalent circuit of the RC battery model is shown in Fig.2(a).The equivalent mean model of the dc/dc converter connected to the battery through a filtering inductance (L b ,R b )is shown in Fig.2(b)and can be described asL bdi battdt+R b i batt =v batt −m batt V bus .(2)Combining (2)with the state-space model of the RC battery model derived and given in [30]a continuous-time domain state-space model of the battery and the dc/dc converter is expressed as˙x batt =A batt x batt +B batt m batt y batt =C batt x batt(3)where x batt =[v C bv C sv batti batt ]Ty batt =[v C b v batt i batt ]TA batt =⎡⎢⎢⎢⎢⎢⎣a 11a 120b 11a 21a 220b 21a 310a 33b 31001L b −R b L b ⎤⎥⎥⎥⎥⎥⎦B batt =−V bus L bT ,C batt =⎡⎢⎣100000100001⎤⎥⎦a 11=−1C bulk (R e +R surf ),a 12=1C bulk (R e +R surf )a 21=1C surf (R e +R surf ),a 22=−1C surf (R e +R surf )TABLE IS YSTEM M ODEL PARAMETERSa 31=R e +R surf C surf (R e +R surf )2−R surf R e +R 2surfC bulk R e (R e +R surf )2a 33=R surfR e C bulk (R e +R surf )−1C surf (R e +R surf )b 11=−R surf C bulk (R e +R surf ),b 21=−R eC surf (R e +R surf )b 31=R t R surfC bulk R e (R e +R surf )−R t C surf (R e +R surf )−R e R surf +R 2eC surf (R e +R surf )2where C bulk is a bulk capacitor to characterize the ability of the battery to store charge,C surf is a surface capacitance to model surface capacitance and diffusion effects,R bulk is an end resistance,R surf is a surface resistance,and R t is a terminal resistance [30].The battery state vector x batt consists of three state equations [v C b ,v C s ,v batt ]T corresponding to the RC battery model and one state equation [i batt ]due to the filtering inductance (L b ,R b ).As shown in Fig.2,the RC battery model consists of only two storage elements (two capacitors)and hence two state equa-tions [v C b ,v C s ]T are sufficient to obtain the state-space model.However,we believe that the introduction of the redundant third state variable [v batt ]into the RC model (please see [30]for further details)improves the robustness of the estimator to pos-sible model parameters mismatch.Therefore,we have directly adopted the battery state-space model given in [30].As graphically shown in [30],the SOC of the battery is ap-proximately linearly dependant on the bulk capacitor voltage v C b and hence it can be estimated from v C b asSOC =k SOC v C b +q SOC(4)where the values of k SOC and q SOC were obtained by linearapproximation of the graph given in [30].The values of k SOC ,q SOC ,the resistances and capacitances of the battery model are given in Table I.B.Ultracapacitor and the DC/DC Converter ModelThe ultracapacitor is modeled using three time constants’equivalent circuit shown in Fig.3(a)[31],[32].The equivalent average model of the dc/dc converter con-nected to the ultracapacitor through a filtering inductance (L c ,R c )is shown in Fig.3(b)and can be described asL cdi capdt+R c i cap =v cap −m cap V bus .(5)1472IEEE TRANSACTIONS ON POWER ELECTRONICS,VOL.29,NO.3,MARCH2014Fig.3.(a)Equivalent circuit of the ultracapacitor.(b)Equivalent circuit of the dc/dc converter.Combining(5)with the state-space model of the three time constants’equivalent circuit,a continuous-time domain state-space model of the ultracapacitor and the dc/dc converter is given by˙x cap=A cap x cap+B cap m capy cap=C cap x cap(6) where x cap=[v f v m v s i cap]T,y cap=[v cap i cap]T, A cap,R x,B cap and C cap shown at the bottom of the page,and R f,C f,R m,C m,R s,C s are the resistances and capacitances of the fast,medium,and slow branches respectively,and R leak is a leakage resistance.The values of the resistances and capacitances of the capacitor model are given in Table I.C.Output Current ModelThe total output current i total in the continuous time domain is(7)i total=1s2L f l C f l+sR f l C f l+1i totals(7)where i totals is the total output current from the dc/dc converters(see Fig.1)given byi totals =f(i batt,m batt,i cap,m cap)=i batt m batt+i cap m cap(8)and1s2L f l C f l+sR f l C f l+1is the transfer function of the low-passLCfilter formed by the inductor(L f l,R f l)and the capacitorC f l.Equation(8)can be linearized around an operating pointP=[i battm batti capm cap]T asi totals=f(i batt,m batt,i cap,m cap)=i batt m batt+i cap m cap≈f(P)+∇f|z=P(z−P)=i batt k batt+i cap k cap(9)where∇=[∂∂i b a t t∂∂m b a t t∂∂i c a p∂∂m c a p],z=[i batt m batt i capm cap]T,i batt=0,m batt=0.5(v C b m a x+v C b m i n)V b u s=k batt,i cap=0,m cap=0.5(v c a p m a x+v c a p m i n)V b u s=k cap.Then,after substituting(9)into(7)the linearized total outputcurrent i total in the continuous time domain is given byi total=1s2L f l C f l+sR f l C f l+1(i batt k batt+i cap k cap).(10)The state-space model of the linearized total output current(10)is˙x it o t a l=A it o t a lx it o t a l+B it o t a li batti capy=i total=C it o t a lx it o t a l(11)wherex it o t a l=[v Cf li Lf l]T,A it o t a l=⎡⎢⎢⎣−1C f l1L f l−R f lL f l⎤⎥⎥⎦B it o t a l=⎡⎣k battC f lk capC f l00⎤⎦,Ci t o t a l=[01].IV.M ODEL P REDICTIVE C ONTROLLERThis sectionfirst derives the complete state-space model ofthe hybrid power source.Then,it describes the procedure usedto design the MPC and the estimator.A cap=⎡⎢⎢⎢⎢⎢⎢⎢⎢⎢⎢⎢⎢⎢⎣R xR2fC f−1R f C fR xR f C f R mR xR f C f R s−R xR f C fR xR m C m R fR xR2m C m−1R m C mR xR m C m R s−R xR m C mR xR s C s R fR xR s C s R mR xR2s C s−1R s C s−R xR s C sR xR f L cR xR m L cR xR s L c−R x−R cL c⎤⎥⎥⎥⎥⎥⎥⎥⎥⎥⎥⎥⎥⎥⎦R x=1R f+1R m+1R s+1R leak−1,B cap=000−V busL cTC cap=⎡⎣R xR fR xR mR xR s−R x0001⎤⎦HREDZAK et al.:MODEL PREDICTIVE CONTROL SYSTEM FOR A HYBRID BATTERY-ULTRACAPACITOR POWER SOURCE1473plete State-Space Model of the Hybrid Power SourceFirst,the state-space models of(3),(6),and(11)are aug-mented into the state-space model⎡⎢⎣˙x batt˙x cap˙xi t o t a l⎤⎥⎦=⎡⎢⎣A batt04×404×204×4A cap04×2M N A it o t a l⎤⎥⎦⎡⎢⎣x battx capx it o t a l⎤⎥⎦+⎡⎢⎣B batt04×104×1B cap02×102×1⎤⎥⎦m battm capy total=⎡⎢⎢⎢⎢⎢⎢⎢⎢⎣v C bv batti battv capi capi total⎤⎥⎥⎥⎥⎥⎥⎥⎥⎦=⎡⎢⎣C batt03×403×202×4C cap02×201×401×4[01]⎤⎥⎦⎡⎢⎣x battx capx it o t a l⎤⎥⎦(12)whereM=01×2B it o t a l1101×20,N=01×3B it o t a l1201×30.Then,the zero-order discretization of the state-space model (12)is augmented with the stateΔi batt(k+1)=i batt(k+1)−i batt(k)(13) and with an output integrator(state x dist)driven by white noise n(k)to compensate for any unmeasured disturbances and model inaccuracies affecting the total output current.The resulting augmented model is the complete discrete state-space model of the hybrid power source⎡⎢⎢⎢⎢⎢⎢⎣x batt(k+1)Δi batt(k+1) x cap(k+1)x it o t a l(k+1) x dist(k+1)⎤⎥⎥⎥⎥⎥⎥⎦=⎡⎢⎢⎢⎢⎢⎢⎣Φbatt04×104×404×204×1ΦΔib a t t001×401×2004×404×1Φcap04×204×1Φit o t a l2×402×1Φi t o t a l2×4Φi t o t a l2×202×101×4001×401×21⎤⎥⎥⎥⎥⎥⎥⎦×⎡⎢⎢⎢⎢⎢⎢⎣x batt(k)Δi batt(k)x cap(k)x it o t a l(k)x dist(k)⎤⎥⎥⎥⎥⎥⎥⎦+⎡⎢⎢⎢⎢⎢⎢⎢⎣Γbatt04×104×1Γbatt410004×1Γcap04×1Γit o t a l2×1Γi t o t a l2×102×100T s⎤⎥⎥⎥⎥⎥⎥⎥⎦×⎡⎢⎣m batt(k)m cap(k)n(k)⎤⎥⎦(14)y total(k)=⎡⎢⎢⎢⎢⎢⎢⎢⎢⎢⎢⎢⎢⎢⎣v C b(k)v batt(k)i batt(k)Δi batt(k)v cap(k)i cap(k)i total(k)⎤⎥⎥⎥⎥⎥⎥⎥⎥⎥⎥⎥⎥⎥⎦=⎡⎢⎢⎢⎣C batt03×103×403×301×4101×401×302×402×1C cap02×301×4001×4[011]⎤⎥⎥⎥⎦×⎡⎢⎢⎢⎢⎢⎢⎢⎣x batt(k)Δi batt(k)x cap(k)x it o t a l(k)x dist(k)⎤⎥⎥⎥⎥⎥⎥⎥⎦.In(14),the matricesΦbatt,Γbatt,Φcap,Γcap,Φit o t a l,Γit o t a lare the system matrices obtained from the zero-order hold dis-cretization of the continuous state-space model(12)and thestate equationΔi batt(k+1)isΔi batt(k+1)=i batt(k+1)−i batt(k)=ΦΔib a t tx batt(k)+Γbatt41m batt(k)(15)whereΦΔib a t t=[Φbatt41Φbatt42Φbatt43(Φbatt44−1)].The state(15)represents a battery current change and is usedby the MPC to minimize the variations in the battery current.Asa result,the battery responds mainly to slow current changes.B.Model Predictive ControllerThe MPC is designed using the model predictive toolbox pro-vided in MATLAB[33].In order to design the MPC,the modelpredictive toolbox requires to specify the model of the system,the manipulated variables,the measured and the unmeasuredoutputs,constraints,and the objective function.It also allows todesign an estimator.In our case,the discrete state-space model is given by(14),themanipulated variables are m cap and m batt,the measured outputsare v batt,i batt,v cap,i cap,i total,and the unmeasured outputs arethe battery model bulk capacitor voltage v C b(corresponding toSOC)and the battery current changeΔi batt.The MPC action at time k is obtained by minimizing theobjective functionJ=p−1i=0(|w it o t a l(i total(k+i+1|k)−i total req(k+i+1))|2+wΔib a t t r e qΔi batt(k+i+1|k)2+w vc a p(v cap(k+i+1|k)−v cap req(k+i+1))2)+ρε(16)subject to constraintsm batt,min−Q m b a t t,min≤m batt(k+i|k)≤m batt,max−Q mb a t t,maxm cap,min−Q m c a p,min≤m cap(k+i|k)1474IEEE TRANSACTIONS ON POWER ELECTRONICS,VOL.29,NO.3,MARCH 2014≤m cap ,max −Q m c a p ,maxv C b,min −Q v C b ,min ≤v C b (k +i +1|k )≤v C b,max −Q v C b ,maxi batt ,min −Q i b a t t ,min ≤i batt (k +i +1|k )≤i batt ,max −Q i b a t t ,maxv cap ,min −Q v c a p ,min ≤v cap (k +i +1|k )≤v cap ,max −Q v c a p ,maxi cap ,min −Q i c a p ,min ≤i cap (k +i +1|k )≤i cap ,max −Q i c a p ,maxi total ,min −Q i t o t a l ,min ≤i total (k +i +1|k )≤i total ,max −Q i t o t a l ,maxwhere the required capacitor voltage is v cap req =(v cap ,max −v cap ,min )/2,(k +i |k )denotes the value predicted for time (k +i )based on the information available at time k ;p is the prediction horizon selected to be equal the control horizon;w i t o t a l ,w Δi b a t t r e q ,w v c a p are nonnegative weights for the corresponding variable;Q m b a t t ,min max ,Q m c a p ,min max ,Q v C b ,min max ,Q i b a t t ,min max ,Q v c a p ,min max ,Q i c a p ,min max ,Q i t o t a l ,min max are nonnegative values that quantify the relax-ation of the corresponding constraint and the weight ρεpenalizes the violation of the constraints.If the Q is zero then the con-straint is hard and cannot be violated and if the value is positive then the larger the value the softer the constraint.The value of ρεis chosen as ρε=105max {w i t o t a l ,w Δi b a t t r e q ,w v c a p }[33].The nonzero value of the weight w i t o t a l determines the weigh-tage on the deviation of the measured output total current i total from the reference current i total ref .The nonzero value of the weight w Δi b a t t r e q determines the weightage on how much the variation in the battery current is minimized.The larger the value of w Δi b a t t r e q the more ag-gressively is the MPC controller minimizing the change in the battery current Δi batt .Hence,the weight w Δi b a t t r e q determines the sharing of the current between the battery and the ultraca-pacitor.The larger the weight w Δi b a t t r e q the more the battery does not respond to the fast current changes and the more the ultracapacitor has to respond to the fast current changes.Exper-imental results shown in Section V illustrate the effect of the value of the weight w Δi b a t t r e q on the current sharing (in Fig.7w Δi b a t t r e q =50and in Fig.11w Δi b a t t r e q =1).As compared with the objective function proposed in [20]we added a term w v c a p (v cap (k +i +1|k )−v cap req (k +i +1)) 2into the objective function (16).The nonzero value of the weight w v c a p determines how much the variation in the capacitor voltage v cap is minimized.As a result,the control system minimizes variation in v cap and attempts to maintain the capacitor voltage around the required reference value v cap req .The advantage of adding this term into the objective function is that it helps to avoid the saturation of the capacitor voltage to the minimum constraint v cap ,min or the maximum constraint v cap ,max .The value of w v c a p has to be chosen carefully as too high value would result in minimization of variation in the capacitor current i cap .C.EstimatorThe complete state-space model (14)can be rewritten in a more compact form asx total (k +1)=Φtotal x total (k )+Γtotal u total (k )y total (k )=C total x total (k ).(17)As the true states x total (k )of the complete state-space model (14),(17)are not available to the controller,predictions are ob-tained from a state estimator.The estimated states ˆx total (k )are computed from the measured outputs y meas (k )by the stationary Kalman state estimator (18)[33],[34]ˆx total (k |k )=ˆxtotal (k |k −1)+M (y meas (k )−ˆy meas (k ))ˆx total (k +1|k )=Φtotal ˆx total (k |k )+Γtotal u total (k )ˆy meas (k )=C meas ˆx total (k |k −1)(18)where y meas (k )=[v batt (k )i batt (k )v cap (k )i cap (k )i total (k )]Tand the matrix C meas is a matrix of the rows of C total cor-responding to the measured outputs y meas (k ).In order to find the gain matrix M in (18)the state-space model (17)is augmented with the noise vectors w (k)and v (k)to the state-space modelx total (k +1)=Φtotal x total (k )+Γtotal u total (k )+Gw (k )y meas (k )=C meas x total (k )+v (k )(19)where the process noise vector w (k)and the measurement noise vector v (k)are zero-mean white noises with covariance matrices Q and R ,respectively,and G is the noise gain matrix relating the process noise to the state variables.In our case G is chosen to be an identity matrix.The innovation gain matrix M is then given byM =PC T meas C meas PC Tmeas +R −1(20)where the matrix P is the covariance matrix of the state estima-tion error.The covariance matrix P is obtained as the solution to the Riccati equationP =Φtotal PΦT total −ΦTotal PC T meas C meas PC Tmeas+R −1C meas PΦT total +Q .(21)In order to find the gain matrix M using (20)and (21),first,the covariance matrices R and Q have to be specified.For the covariance matrix R ,we assumed that the measure-ment noise signals are uncorrelated since they arise from differ-ent sensors.Hence,R is a diagonal matrix and each diagonal element represents the variance of the noise in the particular measurement channel.The larger the diagonal element R ii of the R matrix the weaker is the measurement-based updating of the state estimates.A large element R ii tells the Kalman filter that there is relatively more noise on the measured out-puts than the states.In our experimental setup,the noises in the measured voltages are much smaller as compared with the noises in the measured currents which include ripples due to the converter switching (Note :the ripple is not visible in the ex-perimental results as the converter switching frequency f switchHREDZAK et al.:MODEL PREDICTIVE CONTROL SYSTEM FOR A HYBRID BATTERY-ULTRACAPACITOR POWER SOURCE1475 is20kHz while the data are collected at a sampling period ofT s=0.01s—due to the limitations of the DSPACE controllerboard).Initially,we set all the diagonal elements of R to one.After some empirical tuning the matrix R was selected as R=diag(1×10−5,1×105,1×10−5,1×105,1×105).The selection of the parameters of the covariance matrix Q iscomplicated by the fact that the knowledge of the system dis-turbances,noises,and the model inaccuracies is very limited.We assumed that the disturbances,noises,and the model inac-curacies are uncorrelated which lead to a diagonal matrix Q.Initially,all the diagonal elements of Q can be set to a constantvalue and then each diagonal element can be tuned by observingthe estimator responsefirst in simulation and then in real time.The larger the diagonal element Q ii of the Q matrix the strongeris the measurement-based updating of the corresponding stateestimates.A large element Q ii tells the Kalmanfilter that thevariation in the corresponding real state variable is assumed tobe large and that there is relatively more noise on the states thanthe measured outputs.However,too large Q ii may cause theestimates to become too noisy.Initially,we set all the diagonalelements of Q to one.After extensive tuning the matrix Q wasselected as Q=diag(1×10−7,1,10,10,10,5,100,100,0.01,1,10,1×105).Physical understanding of the model can also help in tuningthe Kalmanfilter as it provides a guide on the expected val-ues of the gain matrix M.For example,the capacitor currentmeasurement should not correct the battery states and hence thecorresponding elements of M should be zero.The estimator matrix M calculated using(20)and(21)forthe aforementioned given Q,R matrices is(22).(Note1:in(22),all elements of M with absolute value less than1×10−4were made zero“by hand,”Note2:the“kalman”function inMATLAB[35]can also be used to calculate the matrix M).M=⎡⎢⎢⎢⎢⎢⎢⎢⎢⎢⎢⎢⎢⎢⎢⎢⎢⎢⎢⎢⎢⎢⎢⎢⎢⎢⎣3×10−400004×10−40000 1000001×10−40003×10−40000 000.999900000.83222×10−40001.84394×10−4000000000000000000000.6180⎤⎥⎥⎥⎥⎥⎥⎥⎥⎥⎥⎥⎥⎥⎥⎥⎥⎥⎥⎥⎥⎥⎥⎥⎥⎥⎦.(22)V.E XPERIMENTAL R ESULTSTo verify the proposed control system,we have built a low power experimental prototype.A photograph of the experimen-tal prototype’s setup is shown in Fig.4.In our setup,we used three100F Maxwell ultracapacitors connected in series and three8Ah Cyclon Hawker sealed valve-regulatedlead–acid Fig.4.Photograph of the experimental setup.battery cells connected in series.Parameters of the battery,the ultracapacitor and the inductors are given in Table I and were found as follows.The values of the resistances and capacitances of the battery model were calculated as three times the values of the resistances and one third of the values of the capacitances given in reference [30]for one battery cell.The values of the resistances and capacitances of the ultra-capacitor model were calculated by substituting the equivalent series resistance(ESR)=15mΩ(from datasheet),the nominal capacitance C0=100F(from datasheet),and the number of cells in series N=3into formulas given by the manufacturer in[31].The values of the inductances L b and L c were calculated as[36]L b,c≈V inΔi batt,cap f switch1−V inV bus(23)where for the battery V in=V batt nom=6V(nominal battery voltage),for the ultracapacitor V in=v cap req=4V(required capacitor voltage),Δi batt,cap is the selected maximum high-frequency current ripple(0.25A),and f switch is the switch-ing frequency.Then,commercially available inductors with the nearest value were selected as given in Table I.The value of the low-pass LCfilter inductance L f l was se-lected to be equal to the commercially available inductors L b and L c selected previously.The value of the capacitor C f l was calculated asC f l=1L f l(2πf f l)2(24)for the cutoff frequency f f l=1kHz in order tofilter out the high-frequency current ripple.Then,a commercially available capacitor with the nearest value was selected as given in Table I. After designing the MPC in MATLAB software using the model predictive toolbox[33]we used Simulink and the。
Advanced Control Theory
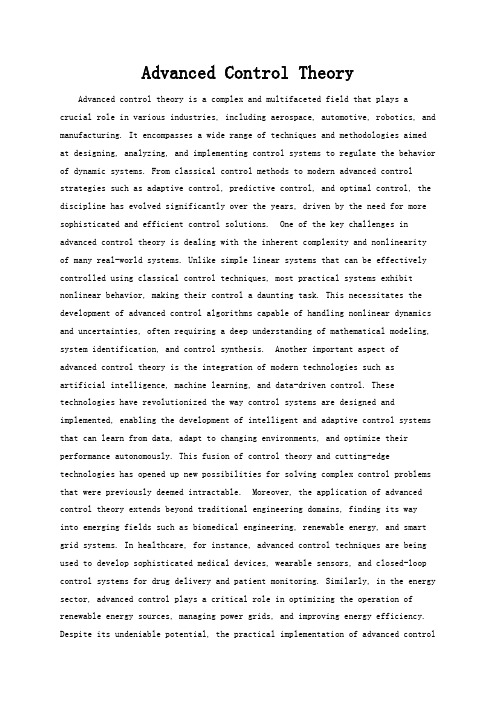
Advanced Control TheoryAdvanced control theory is a complex and multifaceted field that plays a crucial role in various industries, including aerospace, automotive, robotics, and manufacturing. It encompasses a wide range of techniques and methodologies aimed at designing, analyzing, and implementing control systems to regulate the behavior of dynamic systems. From classical control methods to modern advanced control strategies such as adaptive control, predictive control, and optimal control, the discipline has evolved significantly over the years, driven by the need for more sophisticated and efficient control solutions. One of the key challenges in advanced control theory is dealing with the inherent complexity and nonlinearity of many real-world systems. Unlike simple linear systems that can be effectively controlled using classical control techniques, most practical systems exhibit nonlinear behavior, making their control a daunting task. This necessitates the development of advanced control algorithms capable of handling nonlinear dynamics and uncertainties, often requiring a deep understanding of mathematical modeling, system identification, and control synthesis. Another important aspect of advanced control theory is the integration of modern technologies such asartificial intelligence, machine learning, and data-driven control. These technologies have revolutionized the way control systems are designed and implemented, enabling the development of intelligent and adaptive control systems that can learn from data, adapt to changing environments, and optimize their performance autonomously. This fusion of control theory and cutting-edge technologies has opened up new possibilities for solving complex control problems that were previously deemed intractable. Moreover, the application of advanced control theory extends beyond traditional engineering domains, finding its wayinto emerging fields such as biomedical engineering, renewable energy, and smart grid systems. In healthcare, for instance, advanced control techniques are being used to develop sophisticated medical devices, wearable sensors, and closed-loop control systems for drug delivery and patient monitoring. Similarly, in the energy sector, advanced control plays a critical role in optimizing the operation of renewable energy sources, managing power grids, and improving energy efficiency. Despite its undeniable potential, the practical implementation of advanced controltheory is not without its challenges. The high computational complexity of advanced control algorithms, coupled with the stringent real-time requirements of many applications, poses significant implementation challenges. Furthermore, the need for accurate system modeling, identification, and parameter estimation can be a daunting task, especially for systems with complex dynamics and uncertainties. Additionally, the inherent trade-offs between performance, robustness, and complexity in advanced control design further complicate the practical deployment of these techniques. From a broader perspective, the societal implications of advanced control theory are profound. The advancements in control technology have the potential to revolutionize various aspects of our daily lives, from autonomous vehicles and smart infrastructure to personalized healthcare and sustainable energy systems. However, this also raises important ethical and societal considerations, such as the impact of automation on employment, the ethical use of autonomous systems, and the potential risks associated with overreliance on advanced control technology. In conclusion, advanced control theory represents a fascinating and rapidly evolving discipline with far-reaching implications for various industries and societal domains. While the field presents immense opportunities for innovation and advancement, it also poses significant technical, practical, and ethical challenges that need to be carefully addressed. As researchers and practitioners continue to push the boundaries of control theory, it is essential to strike a balance between technological advancement and responsible innovation, ensuring that the benefits of advanced control are harnessed for the greater good of society.。
基于虚拟矢量的FCS-MPC在单相PWM整流器中的应用
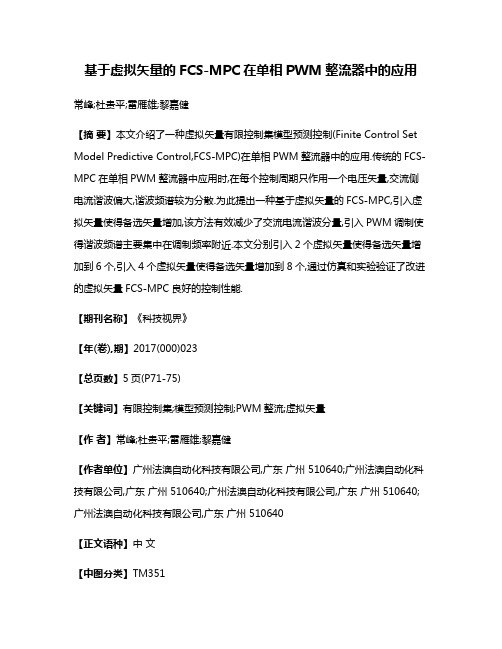
基于虚拟矢量的FCS-MPC在单相PWM整流器中的应用常峰;杜贵平;雷雁雄;黎嘉健【摘要】本文介绍了一种虚拟矢量有限控制集模型预测控制(Finite Control Set Model Predictive Control,FCS-MPC)在单相PWM整流器中的应用.传统的FCS-MPC在单相PWM整流器中应用时,在每个控制周期只作用一个电压矢量,交流侧电流谐波偏大,谐波频谱较为分散.为此提出一种基于虚拟矢量的FCS-MPC,引入虚拟矢量使得备选矢量增加,该方法有效减少了交流电流谐波分量,引入PWM调制使得谐波频谱主要集中在调制频率附近.本文分别引入2个虚拟矢量使得备选矢量增加到6个,引入4个虚拟矢量使得备选矢量增加到8个,通过仿真和实验验证了改进的虚拟矢量FCS-MPC良好的控制性能.【期刊名称】《科技视界》【年(卷),期】2017(000)023【总页数】5页(P71-75)【关键词】有限控制集;模型预测控制;PWM整流;虚拟矢量【作者】常峰;杜贵平;雷雁雄;黎嘉健【作者单位】广州法澳自动化科技有限公司,广东广州 510640;广州法澳自动化科技有限公司,广东广州 510640;广州法澳自动化科技有限公司,广东广州 510640;广州法澳自动化科技有限公司,广东广州 510640【正文语种】中文【中图分类】TM351PWM(Pulse Width Modulation)整流器可以实现quot;绿色电能变换quot;:交流侧电流可以正弦化;运行功率因数可控,能实现单位功率因数;能四象限运行,可以实现能量双向流动.这些优良的特性让其在工业、民生、电力系统、电气传动及军事领域中都得到了较为广泛应用[1],单相PWM整流器在交流传动、UPS电源、柔性交流电传输、光伏及风能并网发电等领域得到广泛应用,使其研究得到越来越多的关注[2].随着自动控制的发展,多种基于PWM整流的控制方案相继被提出,目前最常用的主要有这两类:滞环控制[3],这类控制方法原理简单、无需复杂控制参数设计、不易受电路参数变化的影响,但主要的缺点是开关频率不固定、交流侧电流谐波含量大、控制性能受环宽影响大;线性控制[4],这类控制方法动静态性能好、可以实现无静差,但主要的缺点是控制性能易受系统运行参数变化的影响、需要将系统线性化进行多组PI参数的设计.随着微处理器的发展,模型预测控制(Model Predictive Control,MPC)[5][6]逐渐被结合到电力电子变换器中.而MPC在电力电子系统中的应用主要分为两类:连续状态的模型预测控制(Continuous Control Set MPC,CCS-MPC)[7]和离散状态的有限控制集模型预测控制(Finite Control Set MPC,FCS-MPC)[8].其中FCS-MPC具有无需相关控制参数的调节、无需调制单元、动态响应快等优点逐渐成为电力电子系统模型预测控制研究领域的主要方向.自智利学者Jose Rodriguez等在文献[9]中首次提出了FCSMPC的控制思想以来,在近10年中FCS-MPC在电力电子变换器和相关领域中得到广泛的应用和发展[10][11].但传统的FCS-MPC在一个控制周期只作用一个电压矢量,在开关频率不是非常高的情况下交流侧电流或电压谐波偏大;而且由于没有调制单元,谐波频谱较为分散,给滤波器的设计的带来困难.所以一些学者结合虚拟矢量和调制的思想进行FCS-MPC 的研究[12][13].为此,本文提出一种基于虚拟矢量的FCS-MPC,将其应用到单相电压型PWM整流器中.通过仿真和实验表明所述的虚拟矢量FCS-MPC相比于传统的FCS-MPC交流电流谐波得到有效减少,谐波频谱主要集中在PWM调制频率附近.单相电压型PWM整流器的主电路结构如图1所示,其中e为单相交流电压;L、R 分别为交流侧电感及其等效电阻;V为整流器交流侧电压;i为整流器交流侧电流;C 为直流侧电容;Vdc为直流侧输出电压;RL为纯阻性负载.单相PWM整流器交流测的数学模型可以表述为:假设系统采样频率为Ts,可将式(1)改写为离散形式为:式中:i(k)表示k采样时刻电流的采样值;e(k)表示k采样时刻交流电压的采样值;i(k+1)表示在k采样时刻预测的k+1时刻电流值;V(k)表示k采样时刻整流器交流侧的电压值.定义每个桥臂的开关状态:则整流器交流侧电压可以表示为:可知单相PWM整流共有4种输出电压矢量V0~V3,如图2所示.1)预测模型传统的FCS-MPC利用式(2)在当前采样时刻k预测下一采样时刻k+1的交流侧的电流值,基于k采样时刻的测量值分别将图2所示的4个电压矢量带入(2)得到4个预测值.2)目标函数为了使PWM整流器交流侧的实际运行电流较好地跟踪参考电流,通过定义目标函数J让k+1时刻的预测电流与参考电流的误差最小,J考虑了未来的电流误差,可表示为:式中:i(k+1)*为参考电流.一般认为k采样时刻的参考电流等于k+1采样时刻的参考电流,即i*(k+1)=i*(k).3)参考电流参考电流由电压外环PI控制得到,直流母线电压给定值与反馈直流电压的偏差经过PI控制器得到参考电流的幅值,将幅值与电网电压相位相乘的参考电流.传统FCS-MPC在单相电压型PWM整流器中应用时,每个控制周期从备选的4个电压矢量中选取使得目标函数J最小的最优电压矢量,每个控制周期只作用这一个最优电压矢量,所以在控制频率较低的情况下很难获得较好的控制效果,交流侧电流谐波偏大.由于没有调制单元,单相PWM整流器的开关频率不固定,谐波频谱分散,不利于滤波电感的设计.为了解决传统FCS-MPC在实际应用中存在的问题,我们引入虚拟矢量和调制单元,改善谐波偏大和谐波频谱分散的问题,本文提出两种虚拟矢量FCS-MPC,一种是增加2个虚拟矢量,另一种是增加4个虚拟矢量,下面进行分别阐述.1)6矢量FCS-MPC增加两个虚拟矢量如图3所示.根据图 3可知,Sa共有 0和 1两种值,Sb有 0、1、0.5三种值,对应的电压矢量V=Vdc*(Sa-Sb)共有 5种不同的值.在每个控制周期内这6个电压矢量被分别带入目标函数J中,使得目标函数J最小的电压矢量被送到PWM调制单元,经调制后输出开关状态.其中PWM调制示意图如图4所示.2)8矢量FCS-MPC增加四个虚拟矢量如图5所示.根据图 5可知,Sa共有0和 1两种值,Sb有 0、1/3、2/3、1四种值,同理电压矢量V有7种不同的值.最优矢量的选取和PWM调制与6矢量的FCS-MPC相同.基于虚拟矢量的FCS-MPC算法流程图如图6所示.为了进一步验证本文所述的基于虚拟矢量的FCS-MPC的控制性能,搭建了一套功率为250W的单相电压型PWM整流器实验平台,如图7所示.系统采用TI公司的TMS320F28069作为控制核心板,实验参数如表1所示.A.稳态实验结果图8为传统FCS-MPC稳态实验结果,可以看出传统的FCS-MPC开关频率不固定,电流谐波主要集中在低频处,电流i的 THD约为4.36%.图9为改进的6矢量FCS-MPC的稳态实验结果,可以看出,交流侧电流i能够很好地跟踪电压e,可以实现单位功率因数.另外一部分谐波集中到了调制频率处,低频谐波有效减少,基本实现了定频控制.电流i的 THD明显减少,约为 2.87%.图10为改进的8矢量FCS-MPC稳态实验结果图,相比传统FCS-MPC和改进的6矢量FCS-MPC,电流i的THD进一步减少到2.32%,低频谐波进一步减少.综合上述实验结果和分析可知,改进的虚拟矢量FCS-MPC相比传统的FCS-MPC 的稳态控制性能得到显著改善,电流THD有效减少,低频谐波减少,并主要集中到调制频率附近,基本实现定频控制.可以得出:改进的虚拟矢量FCS-MPC具有较好的稳态性能.B.动态实验结果图 11、12、13分别为传统 FCS-MPC、改进 6矢量FCS-MPC和改进8矢量FCS-MPC的动态试验结果图.为了验证电流的动态响应时间,本文将参考电流的幅值由4.5A切换至7.5A,视为小电流切换为大电流;将参考电流的幅值由7.5A切换为4.5A,视为大电流切换为小电流.当小电流切换为大电流时,从图11(a)、12(a)、13(a)分别可以得出三种控制的动态响应时间分别为101μs、113μs 和117μs; 当大电流切换为小电流是,从图 11(b)、12(b)、13(b)分别可以得出三种控制的动态响应时间分别为114μs、121μs和126μs.根据实验结果和分析可知,改进的虚拟矢量FCSMPC的动态性能相比传统的FCS-MPC受到一点影响,但影响较小,可以忽略.可以得出:改进的虚拟矢量具备较好的动态性能.传统的FCS-MPC由于其优良的动态特性得到广泛的研究,但在控制频率不是很高的情况下很难获得较好的控制效果.本文提出一种基于虚拟矢量的FCSMPC,在单相电压型PWM整流器原有4个电压矢量的基础上分别增加2个和4个虚拟矢量得到6矢量FCS-MPC和8矢量FCS-MPC,并引入PWM调制.通过仿真和实验验证,在常10kHZ的控制频率下,改进的FCSMPC具有较好的稳态控制性能,电流谐波大幅度下降,而且使得电流谐波的频谱基本集中在调制频率附近.在一些应用单相整流或者单相逆变的场合,在要求不太高控制频率和较快动态响应的情况下,本文的研究具有一定的意义.【相关文献】[1]张兴,张崇巍.PWM整流器及其控制[M].北京:机械工业出版社,2012:1-11.[2]罗永捷,李耀华,李子欣,王平.多端柔性直流输电系统直流故障保护策略[J].电工电能新技术,2015,34(12):1-6转13.[3]李欣哲,林明耀,付兴贺,韩愚拙.基于空间矢量的定频直接功率控制系统研究[J].电工电能新技术,2014,33(11):20-23转60.[4]MalinowskiM,KazmierkowskiM P,TrzynadowskiA M.A comparative study of control techniques for PWM rectifiers in AC adjustable speed drives [J].IEEE Trans.on Power Electronic,2003,18(6):1390-1396.[5]谷鑫,姜勃,耿强,刘涛.双永磁同步电机五桥臂变换器模型预测控制[J].电工电能新技术,2015,34(12):25-30.[6]Samir Kouro,MarceloA.Perez,Jose Rodriguez,et al.Model Predictive Control:MPC's Role in the Evolution of Power Electronics[J].IndustrialElectronics Magazine,IEEE,2015,9(4):8-21.[7]CarlosBordons,CarlosMontero.Basic PrinciplesofMPC for Power Converters:Bridging the Gap between Theory and Practice[J].Industrial Electronics Magazine,IEEE,2015,9(3):31-43.[8]Niklas Panten,Nils Hoffmann,and Friedrich Wilhelm Fuchs.Finite ControlSetModelPredictive CurrentControlforGrid-Connected Voltage-SourceConverterswith LCL Filters:A Study Based on DifferentState Feedbacks [J].IEEE Transactions on Power Electronics,2016,31(7):5189-5200.[9]Jose Rodriguez,Jorge Pontt,Cesar Silva,et al.Predictive controlofthree-phase inverter[J].ElectronicsLetters,2004,40(9):561-562.[10]VazquezS.,Leon J.I,Franquelo L.G.,Rodriguez J.,Young H.A.,Marquez A.,Zanchetta P.,quot;ModelPredictive Control:A Review of Its Applications in PowerElectronics,quot;Industrial Electronics Magazine,IEEE.,2014,8(1):16-31.[11]Jose Rodriguez,Marian P.Kazmierkowski,JoséR.Espinoza,Pe ricle Zanchetta,Haitham Abu -Rub,Héctor A.Young,and Christian A.Rojas.State of the Art of Finite ControlSet Model Predictive Control in Power Electronics[J].IEEE Transactions on Industrial Informatics,2013,9(2):1003-1016.[12]Vazquez S,Leon J I,Franquelo L G,et al.Model predictive control with constant switching frequency using a discrete space vector modulation with virtualstate vectors[C].IEEE International Conference on Industrial Technology.IEEE,2009:1-6. [13]曹晓冬,谭国俊,王从刚,李浩.三相 PWM 整流器模型预测虚拟电压矢量控制[J].中国电机工程学报,2014,34(18):2926-2935.。
双三相永磁同步电机模型预测电流控制研究
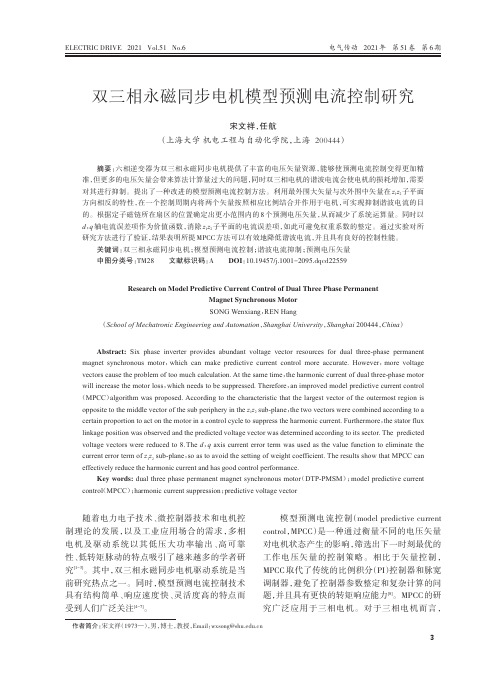
电气传动2021年第51卷第6期摘要:六相逆变器为双三相永磁同步电机提供了丰富的电压矢量资源,能够使预测电流控制变得更加精准,但更多的电压矢量会带来算法计算量过大的问题,同时双三相电机的谐波电流会使电机的损耗增加,需要对其进行抑制。
提出了一种改进的模型预测电流控制方法。
利用最外围大矢量与次外围中矢量在z 1z 2子平面方向相反的特性,在一个控制周期内将两个矢量按照相应比例结合并作用于电机,可实现抑制谐波电流的目的。
根据定子磁链所在扇区的位置确定出更小范围内的8个预测电压矢量,从而减少了系统运算量。
同时以d ,q 轴电流误差项作为价值函数,消除z 1z 2子平面的电流误差项,如此可避免权重系数的整定。
通过实验对所研究方法进行了验证,结果表明所提MPCC 方法可以有效地降低谐波电流,并且具有良好的控制性能。
关键词:双三相永磁同步电机;模型预测电流控制;谐波电流抑制;预测电压矢量中图分类号:TM28文献标识码:ADOI :10.19457/j.1001-2095.dqcd22559Research on Model Predictive Current Control of Dual Three Phase PermanentMagnet Synchronous Motor SONG Wenxiang ,REN Hang(School of Mechatronic Engineering and Automation ,Shanghai University ,Shanghai 200444,China )Abstract:Six phase inverter provides abundant voltage vector resources for dual three-phase permanent magnet synchronous motor ,which can make predictive current control more accurate.However ,more voltage vectors cause the problem of too much calculation.At the same time ,the harmonic current of dual three-phase motor will increase the motor loss ,which needs to be suppressed.Therefore ,an improved model predictive current control (MPCC )algorithm was proposed.According to the characteristic that the largest vector of the outermost region is opposite to the middle vector of the sub periphery in the z 1z 2sub-plane ,the two vectors were combined according to a certain proportion to act on the motor in a control cycle to suppress the harmonic current.Furthermore ,the stator flux linkage position was observed and the predicted voltage vector was determined according to its sector.The predicted voltage vectors were reduced to 8.The d ,q axis current error term was used as the value function to eliminate the current error term of z 1z 2sub-plane ,so as to avoid the setting of weight coefficient.The results show that MPCC can effectively reduce the harmonic current and has good control performance.Key words:dual three phase permanent magnet synchronous motor (DTP-PMSM );model predictive current control (MPCC );harmonic current suppression ;predictive voltage vector双三相永磁同步电机模型预测电流控制研究宋文祥,任航(上海大学机电工程与自动化学院,上海200444)作者简介:宋文祥(1973—),男,博士,教授,Email :**************.cn随着电力电子技术、微控制器技术和电机控制理论的发展,以及工业应用场合的需求,多相电机及驱动系统以其低压大功率输出、高可靠性、低转矩脉动的特点吸引了越来越多的学者研究[1-3]。
电力电子电路容错控制研究

电力电子电路容错控制研究俞保平;俞佳【摘要】与电力电子电路的传统开关函数模型相比,由于混合逻辑动态(MLD)模型同时包含电路的控制变迁和条件变迁,因而MLD模型更能精确的反应电路的变化过程。
这里建立了电力电子电路的MLD模型,考虑到MLD模型中包含离散变量,传统控制方法不再适用,因而将辅助逻辑变量和辅助连续变量引入模型预测控制(MPC),研究了基于MLD模型和MPC的电力电子电路容错控制及其实现步骤。
该方法具有实现简单、容错性能良好、通用性较强的优点。
以三相四桥臂逆变电路的容错控制为例验证了该方法的可行性和有效性。
%Compared with traditional switching function model of power electronic circuit,mixed logic dynamic (MLD) model can accurately describe the changing process of circuit because the mixed logic dynamic model contains the control change and condition change of circuit. The MLD model of power electronic circuit is established in this paper. Considering that the MLD model contains the discrete variables,the traditional control method is no longer applicable. Therefore,the auxiliary logical variables and assisted continuous variable were introduced into the model predictive control (MPC). The fault tolerant control and realization steps of power electronic circuit based on MLD model and MPC were researched. The method has the ad-vantages of simple realization,good fault tolerance error performance,strong versatility. The feasibility and effectiveness of this method were verified by the example of fault-tolerant control of a three-phase four-leg inverter circuit.【期刊名称】《现代电子技术》【年(卷),期】2014(000)020【总页数】3页(P154-156)【关键词】电力电子电路;容错控制;混杂系统;模型预测控制【作者】俞保平;俞佳【作者单位】西安创联电气工程有限公司,陕西西安 710065;中航工业西安富士达科技有限责任公司,陕西西安 710077【正文语种】中文【中图分类】TN710-34;TM464微电子技术、计算机技术、控制技术的发展带动了电力电子技术的快速进步[1],近年来,电力电子电路的应用遍布工业、军事、航空航天等重要领域,主要用于电能的处理与变换,电路的可靠性关乎到整个系统的健康运行,而容错控制(Fault Tolerant Control,FTC)是提高系统可靠性的一个重要手段,容错控制的目的在于通过控制器的调节使故障系统仍能保持满意的性能或至少达到可以接受的性能指标[2]。
基于Matlab的直流无刷电机IP控制的设计与仿真
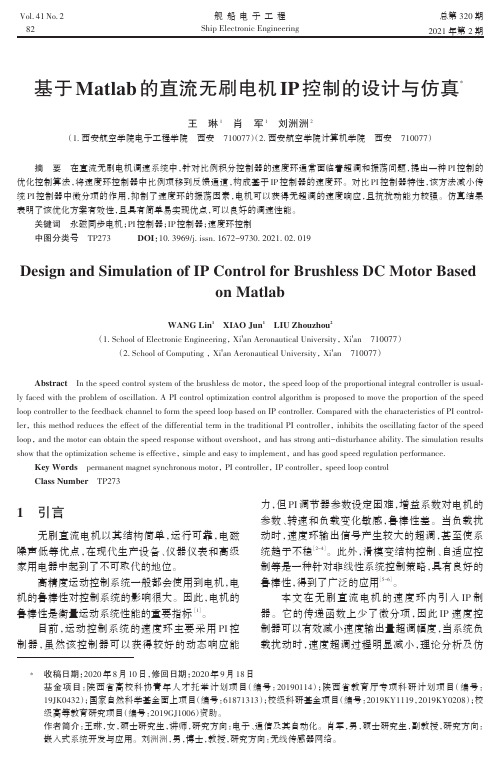
0.643
-0.512
处理方法以满足更多的数据处理需求,将是下一步
0.352
-0.309
-0.317
0.339
0.477
发提高了实验的工作效率,促进了实验室的发展,
两相绕组工作。
(
)
JLs + J ( R s + G c ( s ) β ) s + 1.5K t α + G c ( s ) K p s + 1.5K t G c ( s ) K p
3
2
(2)
相 对 于 PI 控 制 策 略 的 无 刷 直 流 电 机 调 速 系
统,IP 控制策略的闭环传递函数具有相同的特征方
真结果表明该系统速度环的抗干扰能力提高了。
2
83
舰 船 电 子 工 程
无刷直流电动机控制
节器的速度环控制系统,如图 3 所示。电流环作为
普通的无刷直流电机采用三相电压型逆变器
为,β(s) 为电流环反馈回路传递函数。
速度环的内环,其中 G c (s) 为控制器传递函数表示
供电,其定子绕组为星型接法,如图 1 所示,其中
响应能力增强。根据幅频响应曲线可知增大 IP 控
制器比例增益可以提高系统响应带宽,而稳定裕度
变换很小,保留了原系统的鲁棒性。
5
结语
[5]黎永华,皮佑国. 基于磁定位原理的永磁同步电机转子
初始位置定位研究[J]. 电气传动,2010,40(3):28-31.
[6]陆华才,徐月同,杨伟民,等. 表面式永磁直线同步电机
loop,and the motor can obtain the speed response without overshoot,and has strong anti-disturbance ability. The simulation results
摄影专业英语
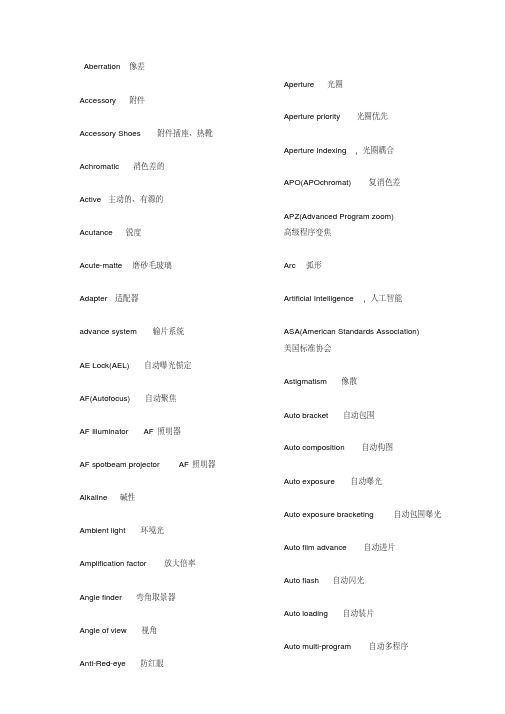
Aberration 像差Accessory 附件Accessory Shoes 附件插座、热靴Achromatic 消色差的Active 主动的、有源的Acutance 锐度Acute-matte 磨砂毛玻璃Adapter 适配器advance system 输片系统AE Lock(AEL) 自动曝光锁定AF(Autofocus) 自动聚焦AF Illuminator AF照明器AF spotbeam projector AF照明器Alkaline 碱性Ambient light 环境光Amplification factor 放大倍率Angle finder 弯角取景器Angle of view 视角Anti-Red-eye 防红眼Aperture 光圈Aperture priority 光圈优先Aperture Indexing,光圈耦合APO(APOchromat) 复消色差APZ(Advanced Program zoom)高级程序变焦Arc 弧形Artificial Intelligence,人工智能ASA(American Standards Association)美国标准协会Astigmatism 像散Auto bracket 自动包围Auto composition 自动构图Auto exposure 自动曝光Auto exposure bracketing 自动包围曝光Auto film advance 自动进片Auto flash 自动闪光Auto loading 自动装片Auto multi-program 自动多程序Auto rewind 自动退片Auto wind 自动卷片Auto zoom 自动变焦Automatic exposure(AE) 自动曝光Automation 自动化Auxiliary 辅助的Back 机背Back light 逆光、背光Back light compensation 逆光补偿Background 背景Balance contrast 反差平衡Bar code system 条形码系统Barrel distortion 桶形畸变BAse-Stored Image Sensor (BASIS) 基存储影像传感器Battery check 电池检测Battery holder 电池手柄Bayonet 卡口Bellows 皮腔Blue filter 蓝色滤光镜Body-integral 机身一体化Bokeh,焦外成像Bridge camera 桥梁相机Brightness control 亮度控制Built in 内置Bulb B 门Button 按钮CCable release 快门线Camera 照相机Camera shake 相机抖动Cap 盖子Caption 贺辞、祝辞、字幕Card 卡Cartridges 暗盒Case 机套CCD(Charge Coupled Device) 电荷耦合器件CdS cell 硫化镉元件Center spot 中空滤光镜Center weighted averaging中央重点加权平均Chromatic Aberration 色差Circle of confusion 弥散圆Close-up 近摄Coated 镀膜coating,镀膜Compact camera 袖珍相机Composition 构图Compound lens 复合透镜Computer 计算机Contact 触点Continuous advance 连续进片Continuous autofocus 连续自动聚焦Contrast 反差、对比Convetor 转换器Coreless 无线圈Correction 校正Coupler 耦合器Coverage 覆盖范围CPU(Central Processing Unit) 中央处理器Creative expansion card 艺术创作软件卡Cross 交叉Curtain 帘幕Customized function 用户自选功能DData back 数据机背Data panel 数据面板Dedicated flash 专用闪光灯Defocus 焦外,散焦Definition 清晰度Delay 延迟、延时Depth of field 景深Depth of field preview 景深预测Detection 检测Developer,显影剂Diaphragm 光阑Diffuse 柔光Diffusers 柔光镜DIN (Deutsche Industrische Normen)德国工业标准Diopter 屈光度Dispersion 色散Display 显示Distortion 畸变Double exposure 双重曝光Double ring zoom 双环式变焦镜头Dreams filter 梦幻滤光镜Drive mode 驱动方式Duration of flash 闪光持续时间Dual Focus,双聚焦,缩写为DFDX-code DX编码Dynamic AF,动态聚焦ED(Extra low Dispersion) 超低色散Electro selective pattern(ESP)电子选择模式Elmar,Leica Elmar结构EOS(Electronic Optical System)电子光学系统Ergonomic 人体工程学EV(Exposure Value) 曝光值Evaluative metering 综合评价测光Expert 专家、专业Exposure 曝光Exposure adjustment 曝光调整Exposure compensation 曝光补偿Exposure memory 曝光记忆Exposure mode 曝光方式Exposure value(EV) 曝光值Extension tube 近摄接圈Extension ring 近摄接圈External metering 外测光Extra wide angle lens 超广角镜头Eye-level fixed 眼平固定Eye-start 眼启动Eyepiece 目镜Eyesight correction lenses 视力校正镜FFiber Paper,纤维纸Field curvature 像场弯曲Fill in 填充(式)Film 胶卷(片)Film speed 胶卷感光度Film transport 输片、过片Filter 滤光镜Finder 取景器First curtain 前帘、第一帘幕Fish eye lens 鱼眼镜头fixer,定影剂Flare 耀斑、眩光Flash 闪光灯、闪光Flash range 闪光范围Flash ready 闪光灯充电完毕Flexible program 柔性程序Fluorite 萤石Focal length 焦距Focal plane 焦点平面Focus 焦点Focus area 聚焦区域Focus hold 焦点锁定Focus lock 焦点锁定Focus prediction 焦点预测Focus priority 焦点优先Focus screen 聚焦屏Focus tracking 焦点跟踪Focusing 聚焦、对焦、调焦Focusing stages 聚焦级数Fog filter 雾化滤光镜Foreground 前景FP Sync,FP同步Frame 张数、帧Freeze 冻结、凝固Fresnel lens 菲涅尔透镜、环状透镜Frontground 前景Fuzzy logic 模糊逻辑GGauss Type,高斯结构Glare 眩光Glossy,光面相纸GN(Guide Number) 闪光指数GPD(Gallium Photo Diode) 稼光电二极管Graduated 渐变HHalf frame 半幅Halfway 半程Hand grip 手柄High eye point 远视点、高眼点High key 高调Highlight 高光、高亮Highlight control 高光控制High speed 高速Honeycomb metering 蜂巢式测光Hologon, Zeiss的超广角镜头Horizontal 水平Hot shoe 热靴、附件插座Hybrid camera 混合相机Hyper manual 超手动Hyper program 超程序Hyperfocal 超焦距IIC(Integrated Circuit) 集成电路Illumination angle 照明角度Illuminator 照明器Image control 影像控制Image size lock 影像放大倍率锁定index of refraction 折射率Infinity 无限远、无穷远Infra-red(IR) 红外线Instant return 瞬回式Integrated 集成Intelligence 智能化Intelligent power zoom 智能化电动变焦Interactive function 交互式功能Interchangeable 可更换nternal focusing 内调焦Interval shooting 间隔拍摄ISO(International Standard Association) 国际标准化组织JJIS(Japanese Industrial Standards)日本工业标准LLandscape 风景Latitude 宽容度LCD data panel LCD数据面板LCD(Liquid Crystal Display) 液晶显示LED(Light Emitting Diode) 发光二极管Lens 镜头、透镜Lens cap 镜头盖Lens hood 镜头遮光罩Lens release 镜头释放钮Lithium battery 锂电池Lock 闭锁、锁定Low key 低调Low light 低亮度、低光LSI(Large Scale Integrated) 大规模集成MMacro 微距、巨像Magnification 放大倍率Main switch 主开关Manual 手动Manual exposure 手动曝光Manual focusing 手动聚焦Matrix metering 矩阵式测光Maximum 最大Metering Coupling,测光耦合Metered manual 测光手动Metering 测光Micro prism 微棱Minimum 最小Mirage 倒影镜Mirror 反光镜Mirror box 反光镜箱Mirror lens 折反射镜头Module 模块Monitor 监视、监视器Monopod 独脚架Motor 电动机、马达Mount 卡口MTF (Modulation Transfer Function调制传递函数Multi beam 多束Multi-layer Caoting Multi-coated,多层镀膜Multi control 多重控制Multi-dimensional 多维Multi-exposure 多重曝光Multi-image 多重影Multi-mode 多模式Multi-pattern 多区、多分区、多模式Multi-program 多程序Multi sensor 多传感器、多感光元件Multi spot metering 多点测光Multi task 多任务Negative 负片Neutral 中性Neutral density filter 中灰密度滤光镜Ni-Cd battery 镍铬(可充电)电池Noctilux,Leica消彗差镜头OOff camera 离机Off center 偏离中心OTF(Off The Film) 偏离胶卷平面One ring zoom 单环式变焦镜头One touch 单环式Orange filter 橙色滤光镜Over exposure 曝光过度Panning 摇拍Panorama 全景Parallel 平行Parallax 平行视差Partial metering 局部测光Passive 被动的、无源的Pastels filter 水粉滤光镜PC(Perspective Control) 透视控制Pearl,珠面相纸Pentaprism 五棱镜Perspective 透视的Phase detection 相位检测Photography 摄影Pincushion distortion 枕形畸变Plane of focus 焦点平面Point of view 视点polarisation 偏振polariser偏振镜Polarizing 偏振、偏光Polarizer 偏振镜Portrait 人像、肖像Power 电源、功率、电动Power focus 电动聚焦Power zoom 电动变焦Predictive 预测Predictive focus control 预测焦点控制Preflash 预闪Professional 专业的Program 程序Program back 程序机背Program flash 程序闪光Program reset 程序复位Program shift 程序偏移Programmed Image Control (PIC)程序化影像控制QQuartz data back 石英数据机背RRainbows filter 彩虹滤光镜Range finder 测距取景器Release priority 释放优先Resin Coated,涂塑相纸Rear curtain 后帘Reciprocity failure 倒易律失效Reciprocity Law 倒易律Recompose 重新构图Red eye 红眼Red eye reduction 红眼减少Reflector 反射器、反光板Reflex 反光Remote control terminal 快门线插孔Remote cord 遥控线、快门线Resolution 分辨率Reversal films 反转胶片Rewind 退卷Ring flash 环形闪光灯ROM(Read Only Memory) 只读存储器Rotating zoom 旋转式变焦镜头RTF(Retractable TTL Flash)可收缩TTL闪光灯Second curtain 后帘、第二帘幕Secondary Imaged Registration(SIR)辅助影像重合Segment 段、区Selection 选择Self-timer 自拍机Sensitivity 灵敏度Sensitivity range 灵敏度范围Sensor 传感器servo 伺服Separator lens 分离镜片Sepia filter 褐色滤光镜Sequence zoom shooting 顺序变焦拍摄Sequential shoot 顺序拍摄Servo autofocus 伺服自动聚焦Setting 设置Shadow 阴影、暗位Shadow control 阴影控制Sharpness 清晰度Shift 偏移、移动Shutter 快门Shutter curtain 快门帘幕Shutter priority 快门优先Shutter release 快门释放Shutter speed 快门速度Shutter speed priority 快门速度优先Silhouette 剪影Single frame advance 单张进片Single shot autofocus 单次自动聚焦Skylight filter 天光滤光镜Slide film 幻灯胶片Slow speed synchronization 慢速同步SLD(Super Lower Dispersion) 超低色散SLR(Single Lens Reflex)单镜头反光照相机SMC(Super Multi Coated) 超级多层镀膜Soft focus 柔焦、柔光Softar,Rodenstock的柔焦镜头SP(Super Performance) 超级性能SPC(Silicon Photo Cell) 硅光电池SPD(Silicon Photo Dioxide) 硅光电二极管Speedlight 闪光灯、闪光管Split image 裂像Sport 体育、运动Spot metering 点测光Standard 标准stepless shutter,无级快门Standard lens 标准镜头Starburst 星光镜Stop 档Synchronization 同步Tele converter 增距镜、望远变换器Telyt, Leica望远镜头Telephoto lens 长焦距镜头Trailing-shutter curtain 后帘同步tone,影调Trap focus 陷阱聚焦Tripod 三脚架TS(Tilt and Shift) 倾斜及偏移TTL flash TTL闪光TTL flash metering TTL闪光测光TTL(Through The Lens) 通过镜头、镜后Two touch 双环UUD(Ultra-low Dispersion) 超低色散Ultra wide 超阔、超广Ultrasonic 超声波UV(Ultra-Violet) 紫外线Under exposure 曝光不足VVario-,[前缀]变Vari-colour 变色Var-program 变程序Variable speed 变速vegnetting,晕映Vertical 垂直Vertical traverse 纵走式View finder 取景器WWarm tone 暖色调White Balance, 白平衡. Wide angle lens 广角镜头Wide view 广角预视、宽区预视Wildlife 野生动物Wireless remote 无线遥控XX-sync X-同步ZZoom 变焦Zoom lens 变焦镜头Zoom clip 变焦剪裁Zoom effect 变焦效果。
- 1、下载文档前请自行甄别文档内容的完整性,平台不提供额外的编辑、内容补充、找答案等附加服务。
- 2、"仅部分预览"的文档,不可在线预览部分如存在完整性等问题,可反馈申请退款(可完整预览的文档不适用该条件!)。
- 3、如文档侵犯您的权益,请联系客服反馈,我们会尽快为您处理(人工客服工作时间:9:00-18:30)。
Predictive Control in Power Electronics and Drives Patricio Cortés,Member,IEEE,Marian P.Kazmierkowski,Fellow,IEEE,Ralph M.Kennel,Senior Member,IEEE, Daniel E.Quevedo,Member,IEEE,and JoséRodríguez,Senior Member,IEEEAbstract—Predictive control is a very wide class of controllers that have found rather recent application in the control of power converters.Research on this topic has been increased in the last years due to the possibilities of today’s microprocessors used for the control.This paper presents the application of different pre-dictive control methods to power electronics and drives.A simple classification of the most important types of predictive control is introduced,and each one of them is explained including some application examples.Predictive control presents several advan-tages that make it suitable for the control of power converters and drives.The different control schemes and applications presented in this paper illustrate the effectiveness andflexibility of predictive control.Index Terms—Drives,power electronics,predictive control.I.I NTRODUCTIONT HE USE of power converters has become very popular in the recent decades for a wide range of applications,in-cluding drives,energy conversion,traction,and distributed gen-eration.The control of power converters has been extensively studied,and new control schemes are presented every year. Several control schemes have been proposed for the control of power converters and drives.Some of them are shown in Fig.1.From these,hysteresis and linear controls with pulse-width modulation(PWM)are the most established in the lit-erature[1]–[3].However,with the development of faster and more powerful microprocessors,the implementation of new and more complex control schemes is possible.Some of these new control schemes for power converters include fuzzy logic, sliding mode control,and predictive control.Fuzzy logic is suitable for applications where the controlled system or some of its parameters are unknown.Sliding mode presents robustness and takes into account the switching nature of the power converters.Other control schemes found in the literature in-clude neural networks,neuro–fuzzy,and other advanced control techniques.Manuscript received August20,2008;revised September18,2008.First published October31,2008;current version published December2,2008. This work was supported in part by the Chilean National Fund of Scientific and Technological Development under Grant1080443and in part by the Universidad Técnica Federico Santa María.P.Cortés and J.Rodríguez are with the Electronics Engineering Department, Universidad Técnica Federico Santa María,Valparaíso110-V,Chile(e-mail: patricio.cortes@usm.cl).M.P.Kazmierkowski is with the Institute of Control and Industrial Electro-nics,Warsaw University of Technology,00-661Warsaw,Poland.R.M.Kennel is with the Technical University of Munich,80333Munich, Germany.D.E.Quevedo is with the School of Electrical Engineering and Computer Science,The University of Newcastle,Callaghan,NSW2308,Australia. Color versions of one or more of thefigures in this paper are available online at .Digital Object Identifier10.1109/TIE.2008.2007480Fig.1.Basic methods of converter control.Predictive control presents several advantages that make itsuitable for the control of power converters:Concepts areintuitive and easy to understand,it can be applied to a varietyof systems,constraints and nonlinearities can be easily in-cluded,multivariable case can be considered,and the resultingcontroller is easy to implement.It requires a high amount ofcalculations,compared to a classic control scheme;however,the fast microprocessors available today make possible theimplementation of predictive control.Generally,the quality ofthe controller depends on the quality of the model.This paper presents a survey of the most important typesof predictive control applied in power electronics and drives.A classification of them is proposed in Section II,and eachtype of predictive control is explained in the following sec-tions,including some application examples.Hysteresis-basedpredictive control is presented in Section III,trajectory-basedpredictive control in Section IV,deadbeat control in Section V,model predictive control(MPC)in Section VI,MPC withfinitecontrol set in Section VII,andfinally,some conclusions arepresented.II.P REDICTIVE C ONTROL M ETHODSPredictive control is a very wide class of controllers thathave found rather recent application in power converters.Theclassification proposed in this paper for different predictivecontrol methods is shown in Fig.2.The main characteristic of predictive control is the use of themodel of the system for the prediction of the future behaviorof the controlled variables.This information is used by thecontroller in order to obtain the optimal actuation,accordingto a predefined optimization criterion.The optimization criterion in the hysteresis-based predictivecontrol is to keep the controlled variable within the boundariesof a hysteresis area,while in the trajectory based,the variablesare forced to follow a predefined trajectory.In deadbeat control,the optimal actuation is the one that makes the error equal tozero in the next sampling instant.A moreflexible criterion isused in MPC,expressed as a cost function to be minimized.The difference between these groups of controllers is thatdeadbeat control and MPC with continuous control set needa modulator,in order to generate the required voltage.Thiswill result in having afixed switching frequency.The other 0278-0046/$25.00©2008IEEEFig.2.Classification of predictive control methods used in power electronics.controllers directly generate the switching signals for the converter,do not need a modulator,and present a variable switching frequency.One advantage of predictive control is that concepts are very simple and intuitive.Depending on the type of predictive control,implementation can also be simple,as with deadbeat control andfinite control set MPC(FS-MPC)(particularly for a two-level converter with horizon N=1).However,in general,some implementations of MPC can be more complex. Variations of the basic deadbeat control,in order to make it more robust,can also become very complex and difficult to understand.Using predictive control,it is possible to avoid the cascaded structure,which is typically used in a linear control scheme, obtaining very fast transient responses.An example of this is the speed control using trajectory-based predictive control. Nonlinearities of the system can be included in the model, avoiding the need of linearizing the model for a given operating point and improving the operation of the system for all condi-tions.It is also possible to include restrictions to some variables when designing the controller.These advantages can be very easily implemented in some control schemes as MPC,but it is very difficult in schemes as deadbeat control.A more detailed description of each type of predictive control is shown in the next sections.III.H YSTERESIS-B ASED P REDICTIVE C ONTROL Hysteresis-based predictive control strategies try to keep the controlled system variables between the boundaries of a hysteresis area or space.The most simple form of this principle is the so-called“bang–bang controller.”Although bang–bang controllers usually are not considered as predictive controllers in literature,they clearly show the characteristics of a typical predictive controller.An improved form of a bang–bang con-troller is the predictive current controller proposed by Holtz and Stadtfeld[4].The block diagram of the hysteresis-based predictive control is shown in Fig.3.Using predictive current control,the switching instants are determined by suitable error boundaries.As an example,Fig.4 shows a circular boundary,the location of which is controlled by the current reference vector i∗s.When the current vector is Fig.3.Hysteresis-based predictive control[4].Fig.4.Predictive current control,boundary circle,and space vector[4]. touches the boundary line,the next switching state vector is determined by prediction and optimization.The trajectories of the current vector for each possible switching state are computed,and predictions are made of the respective time intervals required to reach the error boundary again.These events also depend on the location of the error boundary,which is considered moving in the complex plane as commanded by the predicted current reference.The movement is indicated by the dotted circle in Fig.4.The predictions of the switching instants are based on mathematical equations of the machine.The switching state vector that produces the maximum on-time isfinally selected.This corresponds to min-imizing the switching frequency.The maximum possible switching frequency is limited by the computing time of the algorithms which determine the optimal switching state vector.Higher frequencies can be handled by employing the double prediction method:Well before the boundary is reached,the actual current trajectory is predicted in order to identify the time instant at which the boundary transition is likely to occur.The back electromotiveFig.5.DSPC.force(EMF)vector at this time instant is predicted then.It is used for the optimal selection of the future switching state vector using the earlier described procedure.A further reduction of the switching frequency,which may be needed in very high-power applications,can be achieved by defining a current error boundary of rectangular shape,having the rectangle aligned with the rotorflux vector of the machine. Usingfield-oriented predictive current control,the switching frequency can be reduced more than with a circular boundary area in stator coordinates[5].Holtz and Stadtfeld optimized their predictive controller for minimum switching frequency.Today,different optimizing criteria are considered,e.g.,low current distortion or low elec-tromagnetic inferences(EMIs).Modifications of the predictive current control are consequently under consideration.IV.T RAJECTORY-B ASED P REDICTIVE C ONTROLThe principle of trajectory-based predictive control strategies is to force the system’s variables onto precalculated trajectories. Control algorithms according to this strategy are direct self control by Depenbrock[6]or direct mean torque control by Flach et al.[7].Some additional methods like sliding mode control[8]or direct torque control[9]are a combination of hysteresis-and trajectory-based strategies,whereas direct speed control(DSPC)by Mutschler[10]can be identified as a trajectory-based control system,although it also has a few hysteresis-based aspects.DSPC will be further explained as an example of trajectory-based predictive controllers.Unlike cascade controllers,predictive control algorithms of-fer the possibility to directly control the desired system values. Most predictive control methods published so far only deal with stator currents,torque,orflux(linear)directly;the drive speed is controlled by a superimposed control loop.DSPC,shown in Fig.5,in contrast,has no control loop of this type;the switching events in the inverter are calculated in a way where speed is directly controlled in a time-optimal manner.Similar to the methods of Depenbrock[6]and Takahashi and Nogushi[9],the switching states of the inverter are classified as“torque increasing,”“slowly torque decreasing,”or“rapidly torque decreasing.”For small time intervals,the inertia of the system and the derivatives of machine and load torques are assumed as constant values.The behavior of the system leads to a set of parabolas in the speed error versus acceleration area as shown in Fig.6.Fig.6.DSPC:Trajectories in the e/a-state plane.The initial state of the system is assumed to be e k/a k.Inthis state,a torque increasing voltage vector has to be producedby the inverter,and therefore,the switching state S k is chosen.The state now travels along the dotted parabola until the pointe k+1/a k+1is reached.This is the intersection with anotherparabola for a“torque decreasing”switching state S k+1,whichwill pass through the point“+Hy.”The intersection e k+1/a k+1has been precalculated as the optimal switching instant to reachthe desired state point“+Hy”as fast as possible.Therefore,in e k+1/a k+1,the inverter is commutated into the switchingstate S k+1.Then,the state of the system travels along the newparabola until the point e k+2/a k+2is reached.At this point,theinverter is switched again into a torque increasing state S k+2.The corresponding trajectory passes the point“−Hy.”In steadystate,the state moves along the path+Hy−e k+2/a k+2−Hy−e k+3/a k+3−+Hy.Hence,the speed error e is kept in the hysteresis band between−Hy and+Hy.This is thehysteresis aspect of this strategy aforementioned.Of course,theoptimal steady-state point would be the point of origin.Sincethe switching frequency of the inverter is limited,the drive statecannot befixed to that point.Therefore,the hysteresis band isdefined to keep the switching frequency in an acceptable range.The algorithm of DSPC clearly shows the main principleof predictive control that foreknowledge of the drive systemis used to precalculate the optimal switching states instead oftrying to linearize the nonlinear parts of the system and thencontrol them by PI controllers.The speed can be controlleddirectly without a cascade structure.V.D EADBEAT-B ASED P REDICTIVE C ONTROLA well-known type of predictive controller is the deadbeatcontroller.This approach uses the model of the system tocalculate,once every sampling period,the required referencevoltage in order to reach the reference value in the next sam-pling instant.Then,this voltage is applied using a modulator.It has been applied for current control in three-phase inverters[11]–[21],rectifiers[22],[23],activefilters[24],[25],powerfactor correctors[26],power factor preregulators[27],[28],un-interruptible power supplies[29]–[31],dc–dc converters[32],and torque control of induction machines[33].While thismethod has been used when a fast dynamic response is required,being deadbeat-based,it is often fragile.Indeed,errors in theparameter values of the model,unmodeled delays and otherFig.7.Deadbeat currentcontrol.Fig.8.Illustration of deadbeat current controller operation.errors in the model often deteriorate system performance and may even give rise to instability.Another disadvantage of these deadbeat control schemes is that non-linearities and constraints of the system variables are difficult to incorporate.A.Deadbeat Current ControlA typical deadbeat current control scheme is shown in Fig.7.It can be noted that,compared to a classic current control scheme,the PI controller has been replaced by the deadbeat controller.The reference voltage is applied using a modulator.The load model for a generic RLE load is described by the following space vector equationv =R i +Ld i dt+e (1)where v is the voltage space vector,i is the current space vector,and e is the EMF voltage space vector.The following discrete-time equation can be obtained from (1)for a sampling time T s :1δi (k +1)−χδi (k )=v (k )−e (k )(2)where δ=e −T s R/L and χ=1/R (1−e −T s R/L ).Based on the discrete-time model (2),the reference voltage vector is obtained asv ∗(k )=1δ[i ∗(k +1)−χi (k )]+e (k ).(3)Reference voltage v ∗is applied in the converter using a modulator.The basic operating principle of deadbeat current control is shown in Fig.8.Here,the load current i at time k is different to the reference current i ∗.This error is used for calculation of the reference voltage v ∗,which is applied to the loadatFig.9.Experimental results for the deadbeat current control.(a)Conventional deadbeat current controller implemented in a full digital system (without any compensation).(b)Modified deadbeat current controller proposed in [34].time k .Ideally,at time k +1,the load current will be equal to the reference current.B.Modifications to Basic AlgorithmWhen implemented in a real system,several problems may appear and deteriorate the performance of a deadbeat controller.One of them is the delay introduced by calculation time and modulation.This problem has been solved in [17],[18],and [20]by considering this delay in the model.Another important issue is the sensitivity to plant uncertain-ties and errors in the model parameter values.This problem has been studied,and several solutions have been proposed,includ-ing the use of an adaptive self-tuning scheme [34],a predictive internal model [35],and neural networks [36].Some results comparing the implementation of the conventional deadbeat current controller,i.e.,using (3),implemented in a full digital system without any compensation of the calculation delay,and the modified deadbeat controller proposed in [34]are shown in Fig.9.In some applications,information about the disturbances is needed by the controller,and these include variables which are not measured.In these cases,the use of disturbance observers has been proposed [28],[30].Other specific applications can require a modified algorithm for reduced switching frequency,as proposed in [37].VI.MPCMPC,also referred to as receding horizon control ,is the only one among the so-called advanced control techniques (usually understood as techniques more advanced than a standard PID control)which has been extremely successful in practical appli-cations in recent decades,exerting a great influence on researchand development directions of industrial control systems.Ap-plications and theoretical results abound;see,e.g.,the books [38]–[40]and survey papers[41]–[44].An attractive feature of MPC is that it can handle general constrained nonlinear systems with multiple inputs and outputs in a unified and clear manner. In this section,we will focus on MPC formulations where a continuous control set is considered and controller outputs arefirst passed through modulators,which then provide the switch positions.A survey on FS-MPC formulations which control the states of the converter switches directly,i.e., without any intermediate modulators,will be given later in Section VII.Although the problem setting is common for bothfinite and infinite control set MPC,for sake of practical implications we will discuss it separately.A.System ModelMost MPC strategies are formulated in a discrete-time set-ting with afixed sampling interval,for example,h>0.Here, system inputs are restricted to change their values only at the discrete sampling instants,i.e.,at times t=kh,where k∈{0,1,2,...}denotes the sampling instants.Since power electronics applications are often governed by nonlinear dynamic relations,it is convenient to represent the system to be controlled in discrete-time state space form via x(k+1)=f(x(k),u(k)),k∈{0,1,2,...}(4) where x(k)denotes the state value at time k,whereas u(k)is the plant input.As aforementioned,in this section,we will study configura-tions where the controller output feeds into a modulator stage which then provides the switch positions.Consequently,we will restrict the system inputs{u(k)}in(4)according tou(k)∈U⊆R p,k∈{0,1,2,...}(5) where U is a polytope and p denotes the number of switches. For example,the components of u(k)could correspond to duty cycles or PWM reference signals,in which case,U is formed of intervals,namely,U=[0,1]p.Clearly,the aforementioned model can only approximate switching effects,see also[45]. Nevertheless,as we will see,several interesting proposals for power electronics and drives have been developed by using this simple setting.In addition to the constraints on the system inputs,MPC also allows one to incorporate state constraints,for examplex(k)∈X⊆R n,k∈{0,1,2,...}.(6) State constraints can,for example,correspond to constraints on capacitor voltages inflying capacitor converters or neutral point clamped converters.Constraints on inductive load currents can also be modeled as state constraints.B.Cost FunctionIn MPC,at each time instant k and for a given(measured or estimated)plant state x(k),a cost function over afinite horizon of length N is minimized.The following choice encompasses many alternatives documented in the literature:V(x(k), u (k))Δ=F(x (k+N))+k+N−1=kL(x ( ),u ( )).(7) Here,L(·,·)and F(·)are weighting functions which serve to penalize predicted system behavior,e.g.,differences between voltage references and predicted values;see Section VI-D. Predicted plant state values are formed according tox ( +1)=f(x ( ),u ( )), ∈{k,k+1,...,k+N−1}(8) whereu ( )∈U, ∈{k,k+1,...,k+N−1}(9) refers to tentative plant inputs.The recursion(8)is initialized with the current plant state measurement,i.e.,x (k)←−x(k).(10) Thus,(8)refers to the predictions of the plant states which would result if the plant input at the time instants{k,k+1,..., k+N−1}was set equal to the corresponding values in1u (k)Δ={u (k),u (k+1),...,u (k+N−1)}.(11) Both the predicted plant state trajectory and the plant inputs are constrained in accordance with(5),i.e.,we haveu ( )∈U x ( )∈X∀ ∈{k,k+1,...,k+N−1}.(12) In addition,x (k+N)is typically required to satisfy a given terminal state constraint,for examplex (k+N)∈X f⊆X.(13) Note that,generally,the selection of the terminal state con-straint is related to the stability issues[38],[39].The constrained minimization of V(·,·)as in(7)gives the optimizing control sequence at time k and for state x(k)u(k)Δ={u(k;k),u(k+1;k),...,u(k+N−1;k)}.(14)C.Moving Horizon OptimizationDespite the fact that u(k)contains feasible plant inputs over the entire horizon,in standard MPC,only thefirst element is used,i.e.,the system input is set tou(k)←−u(k;k).(15) At the next sampling step,i.e.,at time k+1,the system state x(k+1)is measured(or estimated),the horizon is shifted by 1It is worth emphasizing here that,in general,plant state predictions will differ from actual plant state trajectories.Fig.10.Moving horizon principle N=3.one step,and another optimization is carried out.This yields u(k+1)and u(k+1)=u(k+1;k+1),etc.As shown in Fig.10for a horizon length N=3,the horizon taken into account in the minimization of V slides forward as k increases. Thus,MPC amounts to an open-loop-optimal feedback control method;see,e.g.,[46].Note that the past is propagated forward in time via the plant state sequence{x(k)}.D.Design ParametersAs seen previously,MPC allows one to treat multivariable nonlinear systems in an,at least,conceptually simple way.In addition to choosing the sampling interval h[which determines the system model(4)],the MPC design essentially amounts to selecting the cost function,i.e.,the weighting functions F(·)and L(·,·),the horizon length N,and,possibly,the state constraint sets X and X f.1)Weighting Functions:The design of the weighting func-tions F(·)and L(·,·)is related to the actual control objectives.2 For example,the tracking of the desired output and internal voltages and currents can be accommodated into the MPC framework by choosing weights which penalize a measure of the difference between predicted and reference values.In[47],it was shown how spectral characteristics of voltage and current tracking errors can be controlled through the use of frequency selective weighting.More details on this approach will be given in Section VII.2)Horizon Length:For a given sampling frequency1/h and,particularly for a system with nonminimum phase dynam-ics,larger values for the horizon length N will,in general, provide better performance,as quantified by the weighting functions F(·)and L(·,·).Indeed,one can expect that,for an N 2Note that the weighting functions should be chosen such that V(·,·) depends on the decision variables contained in u (k);see(11).that is large enough,the effect of u(k)on x ( )for >k+N will be negligible,and consequently,MPC will approximate the performance of an infinite horizon optimal controller.On the other hand,the constrained optimization problem which needs to be solved online tofind the controller output,has computational complexity which,in general,increases with the horizon length.As a consequence,the optimization horizon parameter N allows the designer to tradeoff performance versus online computational effort.Fortunately,excellent performance can often be achieved with relatively small horizons. Interestingly,in some situations,the stability of the closed loop model x(k+1)=f(x(k),u(k;k))can be ensured through the choice of the MPC design parameters,see,e.g., [40],[42],and[48].For power electronics applications con-trolled via MPC,establishing stability results remains,to date, an open problem.However,there is a significant repository of tools in the control community that merit an investigation of their applicability in power electronics applications(see[49] and[50]).E.Linear Quadratic CaseA particularly simple case of(4)–(7)arises when the system model is linear and the cost function is quadratic,i.e.,x(k+1)=Ax(k)+Bu(k)V(x(k), u (k))=x (k+N)T P x (k+N)+k+N−1=kx ( )T Qx ( )+u ( )T Ru ( )(16) where A,B,P,Q,and R are given matrices of appropriate dimensions.If the system inputs and states in(16)are unconstrained, then the moving horizon optimization of the aforementioned cost function leads to a linear time invariant controller.This simple approach was investigated in[51]and[52]for use in drive applications.On the other hand,if the inputs and states are constrained to belong to polytopes,then minimizing the cost function amounts to solving a convex quadratic program.In this case,semiexplicit solutions to the MPC optimization problem can be found.In the associated Explicit MPC schemes,some of the computations can be carried out offline,thus alleviating online computational burden;see[53]and[54].Several works,including[44]and [51],have studied constrained linear quadratic MPC in the context of power electronics and drive applications.We will next revise the approach taken in[44].F.Explicit MPC for Drive ControlThe work[44]illustrates the advantages of replacing the PI current controller in thefield-oriented drive control scheme in Fig.11by Explicit MPC.The dynamics of an induction machine are governed by nonlinear differential equations.To obtain a model suitable for Explicit MPC,see(16),in[44],the time-continuous ma-chine model was discretized,and the nonlinear cross-couplingFig.11.Typicalfield-oriented control of an induction machine[44].Fig.12.Experimental tracking performance of current control:(a)With PI controllers designed according to symmetrical optimum and(b)with model predictive controller[44].between the stator current components i sd and i sq was ne-glected.The state variable x(k)in(16)was chosen asx(k)=[i sd(k)i sq(k)]T.Despite the fact that the model used is only approximate, it turns out that the Explicit MPC formulation adopted can outperform standard PI control without requiring significant additional computation times.Fig.12(a)and(b)shows experi-mental results.In thisfigure,i∗sq is the reference of the stator current component,whereas u∗sq refers to the stator voltage component,i.e.,the control input,which is fed to the PWM module,see Fig.11.mentsThe results of[44]are certainly promising.However,we feel that MPC has significantly more to offer than replacing individual modules within a cascaded control structure.Indeed, the main advantage of MPC,with respect to other control approaches,lies in the possibility to control nonlinear and constrained systems.In addition,various objectives,such as reference tracking and disturbance compensation,can be incor-porated.Thus,it is worth studying the feasibility of develop-ing MPC architectures which govern the entire drive control architecture without using any additional PI loops or PWM modules.For that purpose,nonlinearities need to be taken into account,and thefinite set nature of switching states should be respected.In the following section,we will revise some MPC formula-tions where the switches are controlled directly,without using PWM modules.VII.FS-MPCConsidering the discrete nature of power converters,it is pos-sible to simplify the optimization problem of MPC by avoiding the use of modulators.Taking into account thefinite set of possible switching states of the power converter,which depends on the possible combinations of the on/off switching states of the power switches,the optimization problem is reduced to the evaluation of all possible states and the selection of the one which minimizes the given cost function.In addition to this,if the horizon length is set to N=1,the calculation of the optimal actuation is very simple and easy to implement experimentally, as will be shown later in this section.A.System ModelWhen modeling a converter,the basic element is the power switch,which can be an insulated-gate bipolar transistor,a thyristor,a gate turn-off thyristor,or others.The simplest model of these power switches considers an ideal switch with only two states:on and off.Therefore,the total number of switching states of a power converter is equal to the number of the different combinations of the two switching states of each switch.However,some combinations are not possible,for example,those combinations that short-circuit the dc link.Let us consider the example of an H-bridge single-phase inverter.It has four switches;therefore,the total number of combinations is24=16.However,two switches in the same leg of the inverter cannot be on at the same time;therefore,it is usual to drive them as complementary switches.This way,the number of possible states is reduced,and each leg has only two states. Therefore,the number of possible states is22=4.As a general rule,the number of possible switching states can be calculated as x y,where x is the number of possible states of each leg of the converter and y is the number of phases(or legs) of the converter.This way,a three-phase two-level converter has23=8possible switching states,a three-phase three-level converter has33=27switching states,and afive-phase two-level converter has25=32switching states.Some examples of different converter topologies and their corresponding number of possible switching states are shown in Table I.For some other converter topologies,the way of calculating the possible switching states may be different.。