换热英文参考哦
换热器英语

impingement plate 防冲板
tie rod and spacer 拉杆和定距管
transverse baffle (横向)折流板
floating head backing device 浮头钩圈
floating tube sheet 浮头管板
outside packed floating head exchanger (外)填料函式浮头换热器
kettle type reboiler 釜式重沸器
bayonet type exchanger 内插管式换热器
stacked exchanger 重叠式换热器
tank suction heater 贮罐抽吸加热器
U-tube bundle U形管束
coil heater 盘管式加热器
spiral tube exchanger 螺旋管式换热器
spiral coil 螺旋盘管
hairpin U形盘管
plate exchanger 板式换热器
air cooled heat exchanger 空冷器
channel cover 管箱盖板,平盖
stationary head flange 管箱法兰
instrument connection 仪表接口
stationary head nozzle 管箱接管
pass partition 分程隔板
stationary tubesheet port saddle 固定鞍式支座
sliding support saddle 活动鞍式支座
shell side 壳程
tube side 管程
607换热器外文翻译
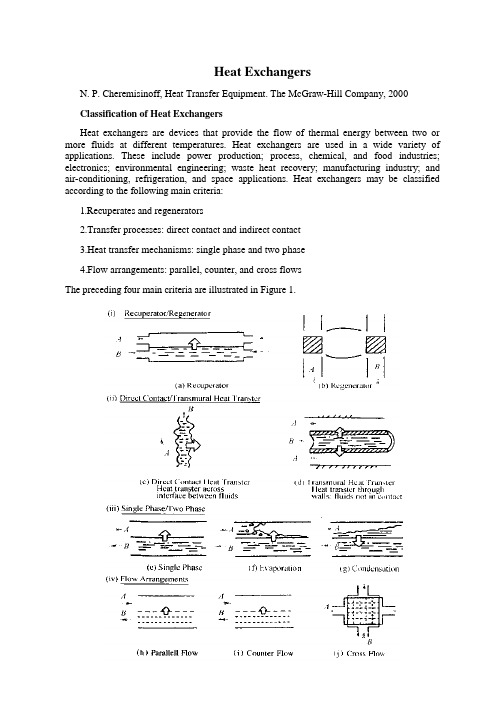
Heat ExchangersN. P. Cheremisinoff, Heat Transfer Equipment. The McGraw-Hill Company, 2000Classification of Heat ExchangersHeat exchangers are devices that provide the flow of thermal energy between two or more fluids at different temperatures. Heat exchangers are used in a wide variety of applications. These include power production; process, chemical, and food industries; electronics; environmental engineering; waste heat recovery; manufacturing industry; and air-conditioning, refrigeration, and space applications. Heat exchangers may be classified according to the following main criteria:1.Recuperates and regenerators2.Transfer processes: direct contact and indirect contact3.Heat transfer mechanisms: single phase and two phase4.Flow arrangements: parallel, counter, and cross flowsThe preceding four main criteria are illustrated in Figure 1.Recuperation and RegenerationThe conventional heat exchangers shown diagrammatically in Figure la with heat transfer between two fluids is called a recuperate, because the hot stream A recovers (recuperates) some of the heat from stream B. The heat transfer is through a separating wall or through the interface between the streams as in the case of direct contact type of heat exchangers (Figure lc).In regenerators or in storage-type heat exchangers, the same flow passage (matrix) is alternately occupied by one of the two fluids. The hot fluid stores the thermal energy in the matrix; during the cold-fluid flow through the same passage later, energy stored will be extracted from the matrix. Therefore, thermal energy is not transferred through the wall as in a direct transfer type of heat exchanger. This cyclic principle is illustrated in Figure lb. While the solid is in the cold stream A it loses heat; while it is in the hot stream B it gains heat (i. e., it is regenerated). Some examples of storage-type heat exchangers are rotary regenerator for preheating the air in a large coal-fired steam power plant, gas turbine rotary regenerator, and fixed-matrix air presenters for blast furnace stoves, steel furnaces, open-hearth steel melting furnaces, and glass furnaces.Criteria used in the classification of heat exchangers, Regenerators can be classified as follows:1.Rotary regenerator2.Fixed-matrix regeneratorRotary regenerators can be further subclassified as:1.Disk type2.Drum typeIn a disk-type regenerator, the heat transfer surface is in a disk form and fluids flow axially. In a drum type, the matrix is in a hollow drum form and fluids flow radially.These regenerators are periodic flow heat exchangers. In rotary regenerators, the operation is continuous. To have this, the matrix moves periodically in and out of the fixed stream of gases. A rotary regenerator can be used for air heating. There are two kinds of regenerative air presenters used in convectional power plants: the rotating-plate type and the stationary-plate type. The rotor of the rotating-plate air heater is mounted within box housing and is installed with the heating surface in the form of plates. As the rotor rotates slowly, the heating surface is exposed alternately to flue gases and to the entering air. When the heating surface is placed in the flue gas stream, the heating surface is heated; and then when it is rotated by mechanical devices into the air stream, the stored heat is released to the air flow. Thus, the air stream is heated. In the stationary-plate air heater, the heating plates are stationary, while cold-air hoods-both top and bottom-are rotated across the heating plates; the heat transfer principles are the same as those of the rotating-plate regenerative air heater. In a fixed-matrix regenerator, the gas flows must be diverted to and from the fixed matrices. Regenerators are compact heat exchangers and they are designed for surface area density of up to approximately 6 600 m2 /m3.Transfer ProcessesAccording to transfer processes, heat exchangers are classified as direct contact type and indirect contact type.In direct contact type heat exchangers, heat is transferred between the cold and hot fluids through a direct contact between these fluids. There is no wall between hot and cold streams, and the heat transfer occurs through the interface between two streams as illustrated in Figure lc. In direct contact-type heat exchangers the streams are two immiscible liquids, a gas-liquid pair, or a solid particle-fluid combination. Spray and tray condensers and cooling towers are good examples of such heat exchangers.In an indirect contact type heat exchanger, the heat energy is exchanged between hot and cold fluids through a heat transfer surface (i.e. a wall separating the fluids). The cold and hot fluids flow simultaneously while heat energy is transferred through a separating wall as illustrated in Figure 16. Id. The fluids are not mixed.Indirect contact- and direct transfer-type heat exchangers are also called recuperates cooling towers; and tray condensers are examples of recuperates.Heat Transfer MechanismsHeat exchanger equipment can also be classified according to the heat transfer mechanisms as:1.Single-phase convection on both sides2.Single-phase convection on one side, rwo-phase convection on other side3.Two-phase convection on both sidesIn heat exchangers like economizers and air heaters in boilers, compressor intercoolers, automotive radiators, regenerators, oil coolers, space heaters, etc., single-phase convection occurs on both sides.Condensers, boilers and steam generators used in pressurized water reactors, power plants, evaporators, and radiators used in air-conditioning and space heating include the mechanisms of condensation, boiling, and radiation on one of the surfaces of the heat exchanger. Two-phase heat transfer could also occur on each side of the heat exchanger such as condensing on one side and boiling on the other side of the heat transfer surface. However, without phase change, we may also have a two-phase flow heat transfer mode as in the case of fluidized beds where a mixture of gas and solid particles transports heat to or from a heat transfer surface.Flow ArrangementsHeat exchangers may be classified according to the fluid-flow path through the heat exchanger. The three basic configurations are1.Parallel flow2.Counter flow3.Cross flowIn parallel flow (concurrent) heat exchangers, the two fluid streams enter together at one end, flow through in the same direction, and leave together at the other end. In counterflow (countercurrent) heat exchangers, two fluid streams flow in opposite directions. In single-crossflow heat exchangers, one fluid flows through the heat transfer surface at right angles to the flow path of the other fluid. Multipass crossflow configurations can also be arranged by having the basic arrangements in series. For example, in a U-baffled tube single-pass shell-and-tube heat exchanger, one fluid flows through the U-tube while the otherfluid flows first downward and then upward, crossing the flow path of the other fluid stream, which is also referred to as crosscounter, cross-parallel flow arrangements.The multipass flow arrangements are frequently used in heat exchanger designs, especially in shell-and-tube heat exchangers with baffles. The main difference between the flow arrangements lies in the temperature distribution along the length of the heat exchanger, and the relative amounts of heat transfer under given temperature specifications for specified heat exchanger surfaces (i.e., for given flow and specified temperatures, a counterflow heat exchanger requires a minimum area, a parallel flow heat exchanger requires a maximum area, while a crossflow heat exchanger requires an area in between).In the crossflow arrangement, the flow may be called mixed or unmixed, depending on the design. If both hot and cold fluids flow through individual flow channels with no fluid mixing between adjacent flow channels, each fluid stream is said to be unmixed. If one fluid flows inside the tubes, thus is not free to move in the transverse direction, and therefore is considered unmixed; on the other hand if another fluid is free to move in the transverse direction and mix itself, and therefore is called unmixed-mixed crossflow heat exchanger.General Design TerminoJogyThe rate of heat transfer from one fluid to another through a metal wall is proportional to the overall heat transfer coefficient, to the area of the metal wall, and to the temperature difference between the hot and cold fluid:Q = U o A MTD eWhen specifying a heat exchanger, the designer nearly always knows or can readily calculate the Q and MTDe terms for the process conditions. It is necessary only to evaluate the coefficient Uo in order to arrive at a proper value of the necessary heat transfer area. Unfortunately, Uo is a function of the actual exchanger design as well as of the fluid properties and fouling rates. For this reason, the design of a heat exchanger requires a trial-and-error calculation.The general procedure used in heat-exchanger design is as follows:1.Establish Q from process considerations.2.Establish MTD e from process considerations. Exchanger type and tube arrange ment will have some effect on MTD? as explained subsequently.3.Assume Uo is the overall duty coefficient.4.Calculate an assumed A from the assumed Uo.5.D etermine the physical dimensions of the applicable type heat exchanger from the calculated A.6.Calculate the fluid pressure drops through the exchanger and modify internals if required to obtain a reasonable balance between pressure drop and exchanger size.7.Calculate Uo from physical properties of the fluids, fouling factors, and theexchanger layout.8.Calculate A based on Q and the calculated valued of U o and MTD e.pare A calculated with A assumed and repeat the calculations until they are equal. (For almost any value of U0there is an exchanger design that satisfies the criterion that Acalculated equals A assumed. However, only a few of these designs are reasonable. ) When heat flows from a fluid on one side of a tube to a fluid on the other side of a tube, it must overcome the following resistances:1.R i0 is the resistance of the fluid laminar "film" on the inside of the tube,2.r io, is the resistance (fouling factor) of foreign material deposited on the inside of the tube,3.r m is the resistance of the metal wall,4.r o is the resistance (fouling factor) of foreign material deposited on the outside of the tube,5.R o is the resistance of the fluid laminar film on the outside of the tube.The sum of these five resistances is R,, the total resistance; andU o=l/R t,The fouling factors r io and r o are estimated based on experience or taken from typical values listed in steam tables. The term r w is calculated from the thickness and thermal conductivity of the metal wall. Rio and R o are functions of mass velocity and physical properties of the fluid and are evaluated from the applicable correlations in temrs of h io and h where 1 /R o = h o, and 1 /R io = h o. The h terms are known as the film coefficients.The resistance terms contain an area dimension, m2, which usually refers to the square meters of surface area at which the resistance occurs. Since the resistance terms must be added to obtain the overall resistance, the area dimensions of each term must refer to the same surface area rather than to its own area. This rationalizes the terms and makes them additive. Standard practice is to use the outside tube area as the basis for calculation and specification of exchangers. As shown earlier, the usual nomenclature indicates this by the subscript io. For example, h io is the inside coefficient based on the outside tube area. For a tube or pipe, h io = h i ( d i/ d o), where hi is the inside coefficient based on the inside tube area. This factor is already included in the correlations presented.The commercially clean coefficient is the overall coefficient which can be expected when a new exchanger is first placed into service and before process fouling of the tubes occurs. It is calculated according to the following:l/U c = Rc = R io + R o + r w+0. 00025The 0.00025 term is an estimated resistance to heat transfer to allow for fouling in a new exchanger due to tube roller lubricants, mild corrosion from hydrostatic testing water, and so on. It is assumed that this allowance is split evenly between the shell-side and the tube-side surfaces.The operating temperatures of the exchanger are usually set by process conditions. However, in certain cases, the exchanger designer will establish the operating temperatures.Temperature of Streams to StorageThe maximum temperature of a stream going to atmospheric storage generally is set by safety, economics, or special process considerations.A stream going to an atmospheric tank at sea level should not exceed that temperature at which its true vapor pressure is 89.6 kPa abs. This value is reduced 11.3 kPa for each 1, 000 m elevation. For heavy streams whose true vapor pressure is difficult to determine, the maximum temperature to tankage should be the lower value of either 28t below the ASTM initial boiling point or 8t below the minimum flash point.Streams should not be sent to tankage at temperatures above 90 to 120℃. Operation in or above this temperature range could cause the tank water heel to flash to steam, resulting in a boll over.The selection of the optimum temperature of a stream going to cone-roof tankage is generally based on an economic balance between the cost of incremental cooler surface and cooling water and the savings due to reduced vapor losses.Opportunity for optimizing the temperature of a stream going to storage is greatest for intermediate products. However, special considerations are required for the following cases;1.Streams that are stored prior to a process requiring refrigeration of the feed.2.Steams whose properties are permanently degraded by high storage temperature.3.Streams those are stored prior to blending. The storage temperatures for such streams should be chosen after considering the properties and temperature of the blend, assuming no heat loss in intermediate tankage.The maximum allowable cooling-water outlet temperatures, set by fouling considerations, for coolers other than box coolers are:•Salt water, 48℃•Brackish water, 51℃•F resh water, 54℃The maximum temperature used for a project should be checked with the affiliate, since this has an important bearing on the economics of surface selection.An equally, if not more, important criteria is the maximum allowable cooling-water temperature. This is the average film temperature at the water outlet. These limits are: • Salt water, 60℃• Fresh water, 65℃For box coolers, the maximum cooling-water outlet temperature is 65℃for both salt and fresh water. If water film temperatures are allowed to exceed the preceding values, catastrophic fouling could result.In those cases where the hot stream outlet temperature is equal to or lower than the maximum allowable cooling-water outlet temperature, a brief economic study may be required to set the optimum cooling-water outlet temperature. The cooling-water outlet temperature is normally determined by designing the exchanger so that the log mean temperature difference correction factor ( Fn) is equal to the minimum allowable value (0. 8). Keep in mind the possibility of using a two-shell pass unit or two shells in series in this situation.At times, a condenser or cooler might be designed using a large amount of cooling water with a relatively low outlet temperature. Consideration should be given to reuse of this water in other coolers where the water outlet temperature may be the maximum permissible. Box coolers invariably operate with reused water.The selection of the optimum arrangement of a series of exchangers (exchanger train) requires a more complex economic study because of the greater number of variables. In many cases, not only must the total heat requirement be distributed between exchangers and a furnace (or steam}, but also several streams at different heat levels may be available to supply the heat. Investment costs must be considered for the exchangers, for the related coolers, and for the furnace (or steam heater). Operating costs for the preceding units must also be included.It is possible to transfer too much heat at the cold end of an exchanger train and thus “pinch out” or require excessive surface at a point farther along when the stream being heated has increased in temperature. Also, in an effort to squeeze out as much heat as possible from a source, the approach temperature (temperature difference between the stream outlet temperatures) used may be too close to be economical. Obviously, for cases where the cost of fuel is high there is more incentive to take a critical look at the economics.The driving force of the heat transfer mechanism is the effective temperature difference, MTD e, between hot and cold fluids. This temperature difference is calculated from the counter-current log mean temperature difference with correction factors applied to account for the actual flow arrangement.In the case of condensation or vaporization, the relationship between Q and the temperature of the fluid usually deviates appreciably from a straight line. In this case, it is necessary to divide the exchanger into zones so that Q is approximately linear with the temperatures for each zone. Based on the logarithmic mean temperature differences and heat duties for the individual zones, a weighted MTD e is obtained for the entire exchanger.Applications of Heat ExchangersMost common heat exchangers are two-fluid heat exchangers. Three-fluid heat I exchangers are widely used in cryogenics. They are also used in various heat exchangers that are used in chemical and process industries, such as air separation systems, purification and liquefaction of hydrogen, and ammonia gas synthesis. Three and multicomponent heat exchangers are very complex to design. They may also include multicomponent two-phase convection as in condensation of mixed vapors in the distillation of hydrocarbons.Heat exchangers are used in a wide variety of applications as in the process, power, air-conditioning, refrigeration, cryogenics, heat recovery, and manufacturing industries. In the power industry, various kinds of fossil boilers, nuclear steam generators, steam condensers, regenerators, and cooling towers are used. In the process industry, two-phase flow heat exchangers are used for vaporizing, condensing, and freezing in crystallization, and for fluidized beds with catalytic reaction. The air-conditioning and refrigeration industries need condensers and evaporators.Energy can be saved by direct contact condensation. By direct contact condensation of a vapor in liquid of the same substance under high pressure, thermal energy can be stored in a storage tank. When the energy is needed again, the liquid is depressurized and flashing occurs that results in producing vapor. The vapor can then be used for heating or as a working fluid for an engine.There have been abrupt developments in heat exchanger applications. One of the mainsteps for the early development of boilers was the introduction of the water-tube boilers. The demand for more powerful engines created a need for boilers that operated at higher pressures, and as a result, individual boilers were built larger and larger. The boiler units used in modern power plants for steam pressures above 1200 lb /in. (80 bar) consist of furnace water-wall tubes, superheaters, and such heat recovery accessories as economizers and air heaters. The development of modem boilers and of more efficient condensers for the power industry has represented a major milestone in engineering. In the pressurized water reactors (PWR), large-sized, inverted U-tube types of steam generators, each providing steam 300 to 400 MW of electrical power, are constructed. In the process industry, engineers are concerned with designing equipment to vaporize a liquid. In the chemical industry, the function of an evaporator is to vaporize a liquid (vaporizer) or to concentrate a solution by vaporizing part of the solvent. Evaporizers may also be used in the crystallization process. Often, the solvent is water, but in many cases the solvent is valuable and recovered for reuse. The vaporizers used in the process chemical industry cover a wide range of sizes and applications.换热器换热器的类型换热器是在两种或多种温度不同的流体间传递热量的一种设备;它有着广泛的应用范围,如动力生产,加工、化学工业及食品行业,电子工业,环境工程,废热回收,制造业,空调、制冷以及空间应用。
(译文)换热器英文参考文献
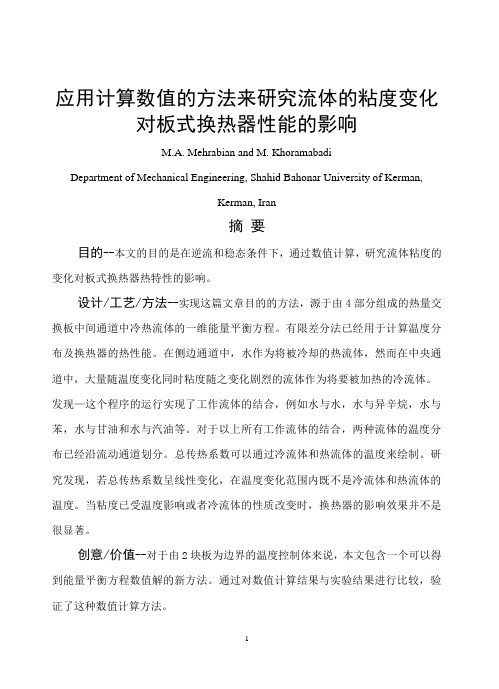
应用计算数值的方法来研究流体的粘度变化对板式换热器性能的影响M.A. Mehrabian and M. KhoramabadiDepartment of Mechanical Engineering, Shahid Bahonar University of Kerman,Kerman, Iran摘要目的--本文的目的是在逆流和稳态条件下,通过数值计算,研究流体粘度的变化对板式换热器热特性的影响。
设计/工艺/方法--实现这篇文章目的的方法,源于由4部分组成的热量交换板中间通道中冷热流体的一维能量平衡方程。
有限差分法已经用于计算温度分布及换热器的热性能。
在侧边通道中,水作为将被冷却的热流体,然而在中央通道中,大量随温度变化同时粘度随之变化剧烈的流体作为将要被加热的冷流体。
发现—这个程序的运行实现了工作流体的结合,例如水与水,水与异辛烷,水与苯,水与甘油和水与汽油等。
对于以上所有工作流体的结合,两种流体的温度分布已经沿流动通道划分。
总传热系数可以通过冷流体和热流体的温度来绘制。
研究发现,若总传热系数呈线性变化,在温度变化范围内既不是冷流体和热流体的温度。
当粘度已受温度影响或者冷流体的性质改变时,换热器的影响效果并不是很显著。
创意/价值--对于由2块板为边界的温度控制体来说,本文包含一个可以得到能量平衡方程数值解的新方法。
通过对数值计算结果与实验结果进行比较,验证了这种数值计算方法。
关键词:热交换器、热传递、数值分析、有限差分法研究类型:研究性论文。
术 语2:m A 板传热面积,m b 板间距,:等式常数:CC ︒W/:C 热容,C kg J C p ︒⋅/:定压比热容,m D e 当量直径,:Cm W h ︒⋅2/:对流传热系数, 指定轴截面:jC m W k ︒⋅/:板传导率,m L 板长度,:粘度修正系数:ms kg m /:质量流量,•之间的斜率与e r R NuP n 31:-NTU: 传热单元数Nu: 努塞尔数Pr: 普朗特数Q: 传热速率, WRe: 雷诺数r: 方程指数 (8)t: 时间, sT: 温度, ℃u: 流速, m/sC m W U ︒⋅2/:总传热系数,C m W U ︒-⋅2/:平均传热系数,3:m V 通道体积,w: 流动宽度, mx: 横向坐标y: 轴向坐标sm kg m ⋅/:流体动粘度系数, 3/:m kg r 流体密度,l: 换热器有效性d: 板厚度, mf: 板投影面积的比值下标c : 冷流体Cv: 控制体h : 热流体m : 平均值min:最小值w : 板壁介绍板式换热器在不同产业发展进程中的贡献日益增加。
供热英语术语知识介绍
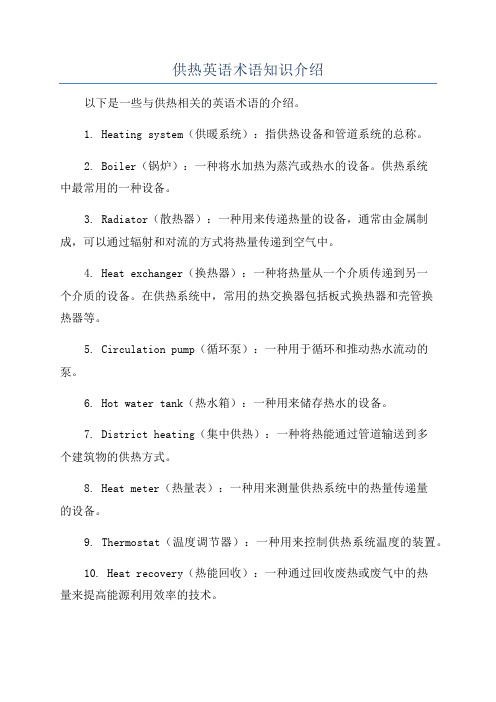
供热英语术语知识介绍以下是一些与供热相关的英语术语的介绍。
1. Heating system(供暖系统):指供热设备和管道系统的总称。
2. Boiler(锅炉):一种将水加热为蒸汽或热水的设备。
供热系统中最常用的一种设备。
3. Radiator(散热器):一种用来传递热量的设备,通常由金属制成,可以通过辐射和对流的方式将热量传递到空气中。
4. Heat exchanger(换热器):一种将热量从一个介质传递到另一个介质的设备。
在供热系统中,常用的热交换器包括板式换热器和壳管换热器等。
5. Circulation pump(循环泵):一种用于循环和推动热水流动的泵。
6. Hot water tank(热水箱):一种用来储存热水的设备。
7. District heating(集中供热):一种将热能通过管道输送到多个建筑物的供热方式。
8. Heat meter(热量表):一种用来测量供热系统中的热量传递量的设备。
9. Thermostat(温度调节器):一种用来控制供热系统温度的装置。
10. Heat recovery(热能回收):一种通过回收废热或废气中的热量来提高能源利用效率的技术。
11. Heat insulation(隔热):一种通过使用隔热材料来减少热量传递的技术。
12. Heat loss(热损失):指供热系统中未被有效利用的热量。
13. Heat supply(供热):指向建筑或设备提供热量。
14. Boiler room(锅炉房):一种专门用于放置和操作锅炉设备的房间。
16. Flue gas(烟气):一种由燃料燃烧产生的含有废气和烟尘的气体。
17. Heat pump(热泵):一种利用环境中的低温热源来提供热量的设备。
18. Boiler efficiency(锅炉效率):指锅炉在将燃料转化为热能时的能源利用效率。
19. Pressure drop(压力降低):指流体通过管道或设备时的压力下降。
传热学课程英语词汇小集

《传热学》课程专业英语词汇1. heat transfer 传热学2. heat conduction 导热3. convection heat transfer 对流换热4. thermal radiation 热辐射5. condensation heat transfer 凝结换热6. boiling heat transfer 沸腾换热7. number of heat transfer unit 传热单元数8. heat exchanger 换热器9. temperature field 温度场10. Fourier’s law 傅里叶定律11. Isothermal surface 等温面12. temperature gradient 温度梯度13. unsteady heat conduction 非稳态导热14. Isotherms 等温线15. lumped method 集总参数法16. thermal conductivity 导热系数17. heat flux 热流密度18. thermal resistance 热阻19. Newton’s law of cooling 牛顿冷却公式20. boundary layer 边界层21. thermal boundary layer 热边界层22. continuity equation 连续性方程23. laminar flow in tube 管内层流24. turbulent flow in tube 管内湍流25. in-tube boiling 管内沸腾26. dimensional analysis 量纲分析27. flow boundary layer 流动边界层28. fin 肋片29. fin efficiency 热效率30. Reynolds number 雷诺数31. Nusselt number 努谢尔数32. Prandtl number 普朗克数33. Planck’slaw 普朗克定律34. boundary layer integral equation 边界层积分方程35.boundary layer differential equation 边界层微分方程36.boundary condition 边界条件37.finite difference 差分38.initial condition 初始条件39.transmissivity 穿透比40.mass transfer process 传质过程41.natural convection in infinite space 大空间自然对流42.poor boiling 大容器沸腾43.partial differential equation of heat conduction 导热微分方程44.numerical solution of heat conduction 导热问题数值解45.directional radiation intensity 定向辐射强度46.log-mean temperature difference 对数平均温差47.multidimensional steady state heat conduction 多维稳态导热48.emissivity 发射率49.analytical solution of transient heat conduction 非稳态导热问题分析解50.Fourier number 傅里叶数work method of radiation heat exchange 辐射换热的网络法52.emissive power 辐射力53.Grashof number 格拉晓夫数54.insulating material 隔热材料(保温材料,绝热材料)55.spectral emissive power 光谱辐射力56.excess temperature 过余温度57.nucleate boiling 核态沸腾58.black body 黑体(绝对黑体)59.flow across single tube 横掠单管60.flow across non-circular cylinder 横掠非圆形截面柱体61.flow across tube bundles 横掠管束62.gray body 灰体63.effectiveness of heat exchanger 换热器的效能64.mixed convection 混合对流65.Kirchhoff’s law 基尔霍夫定律66.cross strings method 交叉线法67.view factor ,angle factor 角系数68.heat conduction with internal heat source 具有内热源的导热erning equation 控制方程place equation 拉普拉斯方程mbert’s law 兰贝特定律72.discretized equation 离散方程73.critical insulation radius 临界绝缘直径74 diffuse surface 漫射表面75.film-wise condensation 膜状凝结76.internal flow 内部流动77.counter-flow 逆流78.gaseous radiation 气体辐射79.enhancement of heat transfer 强化传热80.forced convection 强制对流81.heat pipe 热管82.heat transfer rate 热流量83.time constant 时间常数84.numerical solution 数值解85.Stefan-Boltzmann’s law 斯特潘-波耳兹曼定律86.velocity boundary layer 速度边界层87.solar radiation 太阳辐射88.characteristic length 特征长度89.characteristic number 特征数(准则数)90.irradiation 投入辐射91.turbulent flow 湍流92.external flow 外部流动93.flow along a flat plate 外掠平板94.Wien’s displacement law 维恩位移定律95.green house effect 温室效应96.steady –state heat conduction 稳态导热97.thermal resistance of fouling 污垢热阻98.absorptivity 吸收比99.back difference 向后差分100.forward difference 向前差分101.similarity principle 相似原理102.shape factor 形状因子103.1-dimensional steady state heat conduction 一维稳态导热。
换热器外文翻译 (2)

Heat ExchangersKey Terms Baffles—evenly spaced partitions in a shell and tube heat exchanger that support the tubes, prevent vibration, control fluid velocity and direction, increase turbulent flow, and reduce hot spots. Channel head—a device mounted on the inlet side of a shell-and-tube heat exchanger that is used to channel tube-side flow in a multipass heat exchanger.Condenser—a shell-and-tube heat exchanger used to cool and condense hot vapors.Conduction—the means of heat transfer through a solid, nonporous material resulting from molecular vibration. Conduction can also occur between closely packed molecules.Convection—the means of heat transfer in fluids resulting from currents. Counterflow—refers to the movement of two flow streams in opposite directions; also called countercurrent flow.Crossflow—refers to the movement of two flow streams perpendicular to each other.Differential pressure—the difference between inlet and outlet pressures; represented as ΔP, or delta p.Differential temperature—the difference between inlet and outlet temperature; represented as ΔT, or delta t.Fixed head—a term applied to a shell-and-tube heat exchanger that has the tube sheet firmly attached to the shell.Floating head—a term applied to a tube sheet on a heat exchanger that is not firmly attached to the shell on the return head and is designed to expand (float) inside the shell as temperature rises. Fouling—buildup on the internal surfaces of devices such as cooling towers and heat exchangers, resulting in reduced heat transfer and plugging.Kettle reboiler—a shell-and-tube heat exchanger with a vapor disengaging cavity, used to supply heat for separation of lighter and heavier components in a distillation system and to maintain heat balance. Laminar flow—streamline flow that is more or less unbroken; layers of liquid flowing in a parallel path.Multipass heat exchanger—a type of shell-and-tube heat exchanger that channels the tubeside flow across the tube bundle (heating source) more than once.Parallel flow—refers to the movement of two flow streams in the same direction; for example, tube-side flow and shell-side flow in a heat exchanger; also called concurrent.Radiant heat transfer—conveyance of heat by electromagnetic waves from a source to receivers.Reboiler—a heat exchanger used to add heat to a liquid that was onceboiling until the liquid boils again.Sensible heat—heat that can be measured or sensed by a change in temperature.Shell-and-tube heat exchanger—a heat exchanger that has a cylindrical shell surrounding a tube bundle.Shell side—refers to flow around the outside of the tubes of ashell-and-tube heat exchanger. See also Tube side.Thermosyphon reboiler—a type of heat exchanger that generates natural circulation as a static liquid is heated to its boiling point.Tube sheet—a flat plate to which the ends of the tubes in a heat exchanger are fixed by rolling, welding, or both.Tube side—refers to flow through the tubes of a shell-and-tube heat exchanger; see Shell side.Turbulent flow—random movement or mixing in swirls and eddies of a fluid. Types of Heat Exchangers换热器的类型Heat transfer is an important function of many industrial processes. Heat exchangers are widely used to transfer heat from one process to another.A heat exchanger allows a hot fluid to transfer heat energy to a cooler fluid through conduction and convection. A heat exchanger provides heating or cooling to a process. A wide array of heat exchangers has been designed and manufactured for use in the chemical processing industry. In pipe coil exchangers, pipe coils are submerged in water or sprayed with water to transfer heat. This type of operation has a low heat transfer coefficient and requires a lot of space. It is best suited for condensing vapors with low heat loads.The double-pipe heat exchanger incorporates a tube-within-a-tube design. It can be found with plain or externally finned tubes. Double-pipe heat exchangers are typically used in series-flow operations in high-pressure applications up to 500 psig shell side and 5,000 psig tube side.A shell-and-tube heat exchanger has a cylindrical shell that surrounds a tube bundle. Fluid flow through the exchanger is referred to as tubeside flow or shell-side flow. A series of baffles support the tubes, direct fluid flow, increase velocity, decrease tube vibration, protect tubing, and create pressure drops.Shell-and-tube heat exchangers can be classified as fixed head, single pass; fixed head, multipass; floating head, multipass; or U-tube.On a fixed head heat exchanger (Figure 7.1), tube sheets are attached to the shell. Fixed head heat exchangers are designed to handle temperature differentials up to 200°F (93.33°C). Thermal expansion prevents a fixed head heat exchanger from exceeding this differential temperature. It is best suited for condenser or heater operations.Floating head heat exchangers are designed for high temperature differentia is above 200°F (93.33°C).During operation, one tube sheet is fixed and the other “floats” inside the shell.The floatingend is not attached to the shell and is free toexpand.Figure 7.1 Fixed Head Heat ExchangerReboilers are heat exchangers that are used to add heat to a liquid that was once boiling until the liquid boils again. Types commonly used in industry are kettle reboilers and thermosyphon reboilers.Plate-and-frame heat exchangers are composed of thin, alternating metal plates that are designed for hot and cold service. Each plate has an outer gasket that seals each compartment. Plate-and-frame heat exchangers have a cold and hot fluid inlet and outlet. Cold and hot fluid headers are formed inside the plate pack, allowing access from every other plate on the hot and cold sides. This device is best suited for viscous or corrosive fluid slurries. It provides excellent high heat transfer. Plate-and-frame heat exchangers are compact and easy to clean. Operating limits of 350 to 500°F (176.66°C to 260°C) are designed to protect the internal gasket. Because of the design specification, plate-and-frame heat exchangers are not suited for boiling and condensing. Most industrial processes use this design in liquid-liquid service.Air-cooled heat exchangers do not require the use of a shell in operation. Process tubes are connected to an inlet and a return header box. The tubes can be finned or plain. A fan is used to push or pull outside air over the exposed tubes. Air-cooled heat exchangers are primarily used in condensing operations where a high level of heat transfer is required.Spiral heat exchangers are characterized by a compact concentric design that generates high fluid turbulence in the process medium. As do otherexchangers, the spiral heat exchanger has cold-medium inlet and outlet and a hot-medium inlet and outlet. Internal surface area provides the conductive transfer element. Spiral heat exchangers have two internal chambers.The Tubular Exchanger Manufacturers Association (TEMA) classifies heat exchangers by a variety of design specifications including American Society of Mechanical Engineers (ASME) construction code, tolerances, and mechanical design:●Class B, Designed for general-purpose operation (economy and compactdesign)●Class C. Designed for moderate service and general-purpose operation(economy and compact design)●Class R. Designed for severe conditions (safety and durability) Heat Transfer and Fluid FlowThe methods of heat transfer are conduction, convection, and radiant heat transfer (Figure 7.2). In the petrochemical, refinery, and laboratory environments, these methods need to be understood well. A combination of conduction and convection heat transfer processes can be found in all heat exchangers. The best conditions for heat transfer are large temperature differences between the products being heated and cooled (the higher the temperature difference, the greater the heat transfer), high heating or coolant flow rates, and a large cross-sectional area of the exchanger.ConductionHeat energy is transferred through solid objects such as tubes, heads,baffles, plates, fins, and shell, by conduction. This process occurs when the molecules that make up the solid matrix begin to absorb heat energy from a hotter source. Since the molecules are in a fixed matrix and cannot move, they begin to vibrate and, in so doing, transfer the energy from the hot side to the cooler side.ConvectionConvection occurs in fluids when warmer molecules move toward cooler molecules. The movement of the molecules sets up currents in the fluid that redistribute heat energy. This process will continue until the energy is distributed equally. In a heat exchanger, this process occurs in the moving fluid media as they pass by each other in the exchanger. Baffle arrangements and flow direction will determine how this convective process will occur in the various sections of the exchanger.Radiant Heat TransferThe best example of radiant heat is the sun’s warming of the earth. The sun’s heat is conveyed by electromagnetic waves. Radiant heat transfer is a line-of-sight process, so the position of the source and that of the receiver are important. Radiant heat transfer is not used in a heat exchanger.Laminar and Turbulent FlowTwo major classifications of fluid flow are laminar and turbulent (Figure 7.3). Laminar—or streamline—flow moves through a system in thin cylindrical layers of liquid flowing in parallel fashion. This type of flow will have little if any turbulence (swirling or eddying) in it. Laminar flow usually exists atlow flow rates. As flow rates increase, the laminar flow pattern changes into a turbulent flow pattern. Turbulent flow is the random movement or mixing of fluids. Once the turbulent flow is initiated, molecular activity speeds up until the fluid is uniformly turbulent.Turbulent flow allows molecules of fluid to mix and absorb heat more readily than does laminar flow. Laminar flow promotes the development of static film, which acts as an insulator. Turbulent flow decreases the thickness of static film, increasing the rate of heat transfer. Parallel and Series FlowHeat exchangers can be connected in a variety of ways. The two most common are series and parallel (Figure 7.4). In series flow (Figure 7.5), the tube-side flow in a multipass heat exchanger is discharged into the tubeside flow of the second exchanger. This discharge route could be switched to shell side or tube side depending on how the exchanger is in service. The guiding principle is that the flow passes through one exchanger before it goes to another. In parallel flow, the process flow goes through multiple exchangers at the same time.Figure 7.5 Series Flow Heat ExchangersHeat Exchanger EffectivenessThe design of an exchanger usually dictates how effectively it can transfer heat energy. Fouling is one problem that stops an exchanger’s ability to transfer heat. During continual service, heat exchangers do not remain clean. Dirt, scale, and process deposits combine with heat to form restrictions inside an exchanger. These deposits on the walls of the exchanger resist the flow that tends to remove heat and stop heat conduction by i nsulating the inner walls. An exchanger’s fouling resistance depends on the type of fluid being handled, the amount and type of suspended solids in the system, the exchanger’s susceptibility to thermal decomposition, and the velocity and temperature of the fluid stream. Fouling can be reduced by increasing fluid velocity and lowering the temperature. Fouling is often tracked and identified usingcheck-lists that collect tube inlet and outlet pressures, and shell inlet and outlet pressures. This data can be used to calculate the pressure differential or Δp. Differential pressure is the difference between inlet and outlet pressures; represented as ΔP, or delta p. Corrosion and erosion are other problems found in exchangers. Chemical products, heat, fluid flow, and time tend to wear down the inner components of an exchanger. Chemical inhibitors are added to avoid corrosion and fouling. These inhibitors are designed to minimize corrosion, algae growth, and mineral deposits.Double-Pipe Heat ExchangerA simple design for heat transfer is found in a double-pipe heat exchanger.A double-pipe exchanger has a pipe inside a pipe (Figure 7.6). The outside pipe provides the shell, and the inner pipe provides the tube. The warm and cool fluids can run in the same direction (parallel flow) or in opposite directions (counterflow or countercurrent).Flow direction is usually countercurrent because it is more efficient. This efficiency comes from the turbulent, against-the-grain, stripping effect of the opposing currents. Even though the two liquid streams never come into physical contact with each other, the two heat energy streams (cold and hot) do encounter each other. Energy-laced, convective currents mix within each pipe, distributing the heat.In a parallel flow exchanger, the exit temperature of one fluid can only approach the exit temperature of the other fluid. In a countercurrent flowexchanger, the exit temperature of one fluid can approach the inlet temperature of the other fluid. Less heat will be transferred in a parallel flow exchanger because of this reduction in temperature difference. Static films produced against the piping limit heat transfer by acting like insulating barriers.The liquid close to the pipe is hot, and the liquid farthest away from the pipe is cooler. Any type of turbulent effect would tend to break up the static film and transfer heat energy by swirling it around the chamber. Parallel flow is not conducive to the creation of turbulent eddies. One of the system limitations of double-pipe heat exchangers is the flow rate they can handle. Typically, flow rates are very low in a double-pipe heat exchanger, and low flow rates are conducive to laminar flow. Hairpin Heat ExchangersThe chemical processing industry commonly uses hairpin heat exchangers (Figure 7.7). Hairpin exchangers use two basic modes: double-pipe and multipipe design. Hairpins are typically rated at 500 psig shell side and 5,000 psig tube side. The exchanger takes its name from its unusual hairpin shape. The double-pipe design consists of a pipe within a pipe. Fins can be added to the internal tube’s external wall to increase heat transfer. The multipipe hairpin resembles a typical shell-and-tube heat exchanger, stretched and bent into a hairpin.The hairpin design has several advantages and disadvantages. Among its advantages are its excellent capacity for thermal expansion because of its U-tube type shape; its finned design, which works well with fluids that have a low heat transfer coefficient; and its high pressure on the tube side. In addition, it is easy to install and clean; its modular design makes it easy to add new sections; and replacement parts are inexpensive and always in supply. Among its disadvantages are the facts that it is not as cost effective as most shell-and-tube exchangers and it requires special gaskets.Shell-and-Tube Heat ExchangersThe shell-and-tube heat exchanger is the most common style found inindustry. Shell-and-tube heat exchangers are designed to handle high flow rates in continuous operations. Tube arrangement can vary, depending on the process and the amount of heat transfer required. As the tube-side flow enters the exchanger—or “head”—flow is directed into tubes that run parallel to each other. These tubes run through a shell that has a fluid passing through it. Heat energy is transferred through the tube wall into the cooler fluid. Heat transfer occurs primarily through conduction (first) and convection (second). Figure 7.8 shows a fixed head,single-pass heat exchanger.Fluid flow into and out of the heat exchanger is designed for specific liquid–vapor services. Liquids move from the bottom of the device to the top to remove or reduce trapped vapor in the system. Gases move from top to bottom to remove trapped or accumulated liquids. This standard applies to both tube-side and shell-side flow.Plate-and-Frame Heat ExchangersPlate-and-frame heat exchangers are high heat transfer and high pressure drop devices. They consist of a series of gasketed plates, sandwiched together by two end plates and compression bolts (Figures 7.20 and 7.21). The channels between the plates are designed to create pressure drop and turbulent flow so high heat transfer coefficients can be achieved.The openings on the plate exchanger are located typically on one of the fixed-end covers.As hot fluid enters the hot inlet port on the fixed-end cover, it is directed into alternating plate sections by a common discharge header. The header runs the entire length of the upper plates. As cold fluid enters the countercurrent cold inlet port on the fixed-end cover, it is directed into alternating plate sections. Cold fluid moves up the plates while hot fluid drops down across the plates. The thin plates separate the hot and cold liquids, preventing leakage. Fluid flow passes across the plates one time before entering the collection header. The plates are designed with an alternating series of chambers. Heat energy is transferred through the walls of the plates by conduction and into the liquid by convection. The hot and cold inlet lines run the entire length of the plate heater and function like a distribution header. The hot and cold collection headers run parallel and on the opposite side of the plates from each other. The hot fluid header that passes through the gasketed plate heat exchanger is located in the top. This arrangement accounts for the pressure drop and turbulent flow as fluid drops over the plates and into the collection header. Cold fluid enters the bottom of the gasketed plate heat exchanger and travels countercurrent to the hot fluid. The cold fluid collection header is located in the upper section of the exchanger.Plate-and-frame heat exchangers have several advantages and disadvantages. They are easy to disassemble and clean and distribute heat evenly so there are no hot spots. Plates can easily be added or removed. Other advantages of plate-and-frame heat exchangers are their low fluid resistance time, low fouling, and high heat transfer coefficient. In addition, if gaskets leak, they leak to the outside, and gaskets are easy to replace.The plates prevent cross-contamination of products. Plate-and-frame heat exchangers provide high turbulence and a large pressure drop and are small compared with shell-and-tube heat exchangers.Disadvantages of plate-and-frame heat exchangers are that they have high-pressure and high-temperature limitations. Gaskets are easily damaged and may not be compatible with process fluids.Spiral Heat ExchangersSpiral heat exchangers are characterized by a compact concentric design that generates high fluid turbulence in the process medium (Figure 7.22). This type of heat exchanger comes in two basic types: (1) spiral flow on both sides and (2) spiral flow–crossflow. Type 1 spiral exchangers are used in liquid-liquid, condenser, and gas cooler service. Fluid flow into the exchanger is designed for full counterflow operation. The horizontal axial installation provides excellent self-cleaning of suspended solids.Type 2 spiral heat exchangers are designed for use as condensers, gas coolers, heaters, and reboilers. The vertical installation makes it an excellent choice for combining high liquid velocity and low pressure drop on the vapor-mixture side. Type 2 spirals can be used in liquid-liquid systems where high flow rates on one side are offset by low flow rates on the other.Air-Cooled Heat ExchangersA different approach to heat transfer occurs in the fin fan or air-cooled heat exchanger. Air-cooled heat exchangers provide a structured matrix of plain or finned tubes connected to an inlet and return header (Figure 7.23). Air is used as the outside medium to transfer heat away from the tubes. Fans are used in a variety of arrangements to apply forced convection for heattransfer coefficients. Fans can be mounted above or below the tubes in forced-draft or induced-draft arrangements. Tubes can be installed vertically or horizontally.The headers on an air-cooled heat exchanger can be classified as cast box, welded box, cover plate, or manifold. Cast box and welded box types have plugs on the end plate for each tube. This design provides access for cleaning individual tubes, plugging them if a leak is found, and rerolling to tighten tube joints. Cover plate designs provide easy access to all of the tubes. A gasket is used between the cover plate and head. The manifold type is designed for high-pressure applications.Mechanical fans use a variety of drivers. Common drivers found in service with air-cooled heat exchangers include electric motor and reduction gears, steam turbine or gas engine, belt drives, and hydraulic motors. The fan blades are composed of aluminum or plastic. Aluminum blades are d esigned to operate in temperatures up to 300°F (148.88°C), whereas plastic blades are limited to air temperatures between 160°F and 180°F(71.11°C, 82.22°C).Air-cooled heat exchangers can be found in service on air compressors, in recirculation systems, and in condensing operations. This type of heat transfer device provides a 40°F (4.44°C) temperature differential between the ambient air and the exiting process fluid.Air-cooled heat exchangers have none of the problems associated with water such as fouling or corrosion. They are simple to construct and cheaper to maintain than water-cooled exchangers. They have low operating costs and superior high temperature removal (above 200°F or 93.33°C). Their disadvantages are that they are limited to liquid or condensing service and have a high outlet fluid temperature and high initial cost of equipment. In addition, they are susceptible to fire or explosion in cases of loss of containment.。
换热器英文
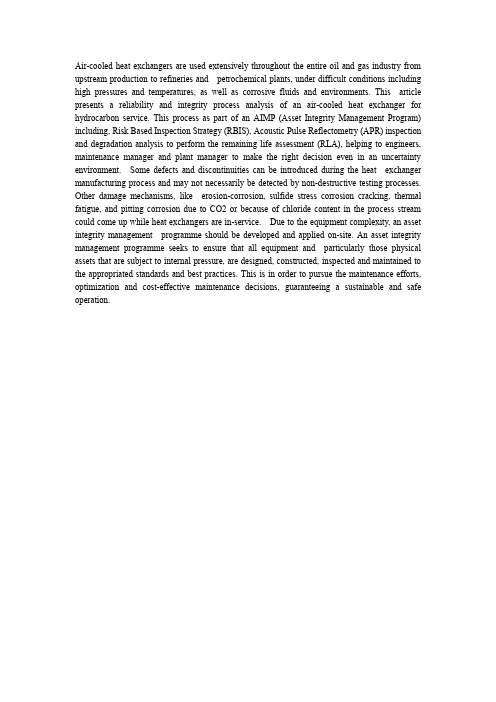
Air-cooled heat exchangers are used extensively throughout the entire oil and gas industry from upstream production to refineries and petrochemical plants, under difficult conditions including high pressures and temperatures, as well as corrosive fluids and environments. This article presents a reliability and integrity process analysis of an air-cooled heat exchanger for hydrocarbon service. This process as part of an AIMP (Asset Integrity Management Program) including, Risk Based Inspection Strategy (RBIS), Acoustic Pulse Reflectometry (APR) inspection and degradation analysis to perform the remaining life assessment (RLA), helping to engineers, maintenance manager and plant manager to make the right decision even in an uncertainty environment. Some defects and discontinuities can be introduced during the heat exchanger manufacturing process and may not necessarily be detected by non-destructive testing processes. Other damage mechanisms, like erosion-corrosion, sulfide stress corrosion cracking, thermal fatigue, and pitting corrosion due to CO2 or because of chloride content in the process stream could come up while heat exchangers are in-service. Due to the equipment complexity, an asset integrity management programme should be developed and applied on-site. An asset integrity management programme seeks to ensure that all equipment and particularly those physical assets that are subject to internal pressure, are designed, constructed, inspected and maintained to the appropriated standards and best practices. This is in order to pursue the maintenance efforts, optimization and cost-effective maintenance decisions, guaranteeing a sustainable and safe operation.。
换热器文献翻译之英文部分
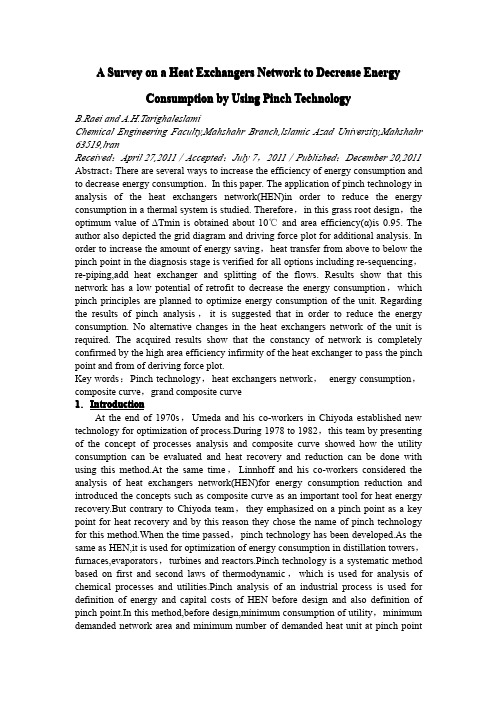
A Survey on a Heat Exchangers Network to Decrease EnergyConsumption by Using Pinch TechnologyB.Raei and A.H.TarighaleslamiChemical Engineering Faculty,Mahshahr Branch,lslamic Azad University,Mahshahr 63519,lranReceived:April27,2011/Accepted:July7,2011/Published:December20,2011 Abstract:There are several ways to increase the efficiency of energy consumption and to decrease energy consumption.In this paper.The application of pinch technology in analysis of the heat exchangers network(HEN)in order to reduce the energy consumption in a thermal system is studied.Therefore,in this grass root design,the optimum value ofΔTmin is obtained about10℃and area efficiency(α)is0.95.The author also depicted the grid diagram and driving force plot for additional analysis.In order to increase the amount of energy saving,heat transfer from above to below the pinch point in the diagnosis stage is verified for all options including re-sequencing,re-piping,add heat exchanger and splitting of the flows.Results show that this network has a low potential of retrofit to decrease the energy consumption,which pinch principles are planned to optimize energy consumption of the unit.Regarding the results of pinch analysis,it is suggested that in order to reduce the energy consumption.No alternative changes in the heat exchangers network of the unit is required.The acquired results show that the constancy of network is completely confirmed by the high area efficiency infirmity of the heat exchanger to pass the pinch point and from of deriving force plot.Key words:Pinch technology,heat exchangers network,energy consumption,composite curve,grand composite curve1.At the end of1970s,Umeda and his co-workers in Chiyoda established new technology for optimization of process.During1978to1982,this team by presenting of the concept of processes analysis and composite curve showed how the utility consumption can be evaluated and heat recovery and reduction can be done with using this method.At the same time,Linnhoff and his co-workers considered the analysis of heat exchangers network(HEN)for energy consumption reduction and introduced the concepts such as composite curve as an important tool for heat energy recovery.But contrary to Chiyoda team,they emphasized on a pinch point as a key point for heat recovery and by this reason they chose the name of pinch technology for this method.When the time passed,pinch technology has been developed.As the same as HEN,it is used for optimization of energy consumption in distillation towers,furnaces,evaporators,turbines and reactors.Pinch technology is a systematic method based on first and second laws of thermodynamic,which is used for analysis of chemical processes and utilities.Pinch analysis of an industrial process is used for definition of energy and capital costs of HEN before design and also definition of pinch point.In this method,before design,minimum consumption of utility,minimum demanded network area and minimum number of demanded heat unit at pinch pointare targeted for given process.At next stage,design of HEN will be done to satisfy performed target.Finally,minimum annual cost is obtained with comparison between energy cost and capital cost and trade of them.Therefore,the main goal of pinch analysis is the optimization of process heat integration,increase the process-process heat recovery,and decrease the amount of utility consumption.For analysis,at first,shifted temperature is obtained then temperature and enthalpy plot draw(half of amount of minimum temperature are deducted from hot stream and added to cold stream).Fig.1shows the composite curve and grand composite curve as tools for pinch Analysis.The composite curves(CCs)present the relationship between cumulative enthalpy flow rate and temperature for the HEN hot and cold streams.In practice,CCs are generated by a cumulative process over a temperature range,and the resulting hot and cold CCs are labelled CCh and CCc,respectively.2.Methods and Data2.1Presentation of a Heat Exchanger NetworkIn a heat exchanger network,arrangement of exchangers in the network is important.For representing such arrangement,the concept of“stage"is used.In every stage,the input and output heat of the stage is equal for the entire exchangers that settled on special stream,whereas the number of stages is not too many in an optimal network.In this part,stages of heat exchanger networks analysis for reduction of energy consumption using pinch technology were explained.Since targeting and design is based on extracted data any mistake and careless in data assembling can lead to completely unreal results.In pinch analysis,design data such as supply and target temperature of streams,flow and heat capacity of stream was used and on the other hand,heat exchangers design was related to heat transfer coefficient directly.In Table1,the necessary extracted information and a sample network is represented.In this research,Aspen pinch software has been used.Fig.1Tools for pinch analysis:composite curve(CC)and grand composite curve(Gee).Table1Extracted data.2.2Economical DataCorrect economic data including operation time ,interest rate and equipment life have an important role on successful execution ofretrofit and preparation .The values are shown in Table 2.The condition of utilities which includes steam and cooling water is shown in Table 3.Capital cost and energy cost of network can be calculated with respect to the shells number and the cost of any exchanger calculates with using Eq .(1):c Area b a t CapitalCos )(+=(1)In this equation ,a ,b and C are constant .So that ,“a”is function of pressure intensity .“b”is function of exchanger material and “c”is function of type of exchanger that is different for various exchangers ;SO 0<C <1.Types of exchanger are defined by designer based on nature of chemical materials ,pressure of flows ,pressure condition and ability of corrosion .For carbon-still exchanger ,cost equation is as follow :81.0)(75030800Area t CapitalCos +=。
化工装置常用英语词汇对照

化工装置常用英语词汇对照1. 反应釜(Reactort) Reactor2. 蒸馏塔(Distillation Tower) Distillation Column3. 冷凝器(Condenser) Condenser4. 换热器(Heat Exchanger) Heat Exchanger5. 压缩机(Compressor) Compressor6. 泵(Pump) Pump7. 阀门(Valve) Valve8. 管道(Pipeline) Pipeline9. 传感器(Sensor) Sensor10. 控制系统(Control System) Control System11. 进料(Feed) Feed12. 产品(Product) Product13. 副产品(Byproduct) Byproduct14. 废料(Waste) Waste15. 物料(Material) Material16. 流量(Flow Rate) Flow Rate17. 压力(Pressure) Pressure18. 温度(Temperature) Temperature19. 浓度(Concentration) Concentration20. 比重(Specific Gravity) Specific Gravity21. 开车(Startup) Startup22. 停车(Shutdown) Shutdown23. 维修(Maintenance) Maintenance24. 检修(Overhaul) Overhaul25. 调试(Commissioning) Commissioning26. 操作规程(Operating Procedure) Operating Procedure27. 安全规程(Safety Procedure) Safety Procedure28. 紧急停车(Emergency Shutdown) Emergency Shutdown29. 报警系统(Alarm System) Alarm System30. 防爆区域(Explosionproof Area) Explosionproof Area 化工装置常用英语词汇对照(续)31. 化学反应(Chemical Reaction) Chemical Reaction32. 反应速率(Reaction Rate) Reaction Rate33. 溶解度(Solubility) Solubility34. 酸碱度(pH Value) pH Value35. 悬浮物(Suspension) Suspension36. 沉淀(Precipitation) Precipitation37. 搅拌(Agitation) Agitation38. 过滤(Filtration) Filtration39. 萃取(Extraction) Extraction40. 吸附(Adsorption) Adsorption41. 蒸发(Evaporation) Evaporation42. 结晶(Crystallization) Crystallization43. 干燥(Drying) Drying44. 焙烧(Calcination) Calcination45. 熔融(Melting) Melting46. 铸造(Casting) Casting47. 冷却(Cooling) Cooling48. 加热(Heating) Heating49. 真空(Vacuum) Vacuum50. 压缩空气(Compressed Air) Compressed Air51. 工艺流程(Process Flow) Process Flow52. 设备布局(Equipment Layout) Equipment Layout53. 流程图(Piping and Instrumentation Diagram, P&ID)Piping and Instrumentation Diagram54. 设计规范(Design Specification) Design Specification55. 操作手册(Operation Manual) Operation Manual56. 安全手册(Safety Manual) Safety Manual57. 环保要求(Environmental Requirements) Environmental Requirements58. 能耗(Energy Consumption) Energy Consumption59. 自动化(Automation) Automation60. 信息化(Informatization) Informatization这些词汇在化工装置的日常操作、维护和管理中扮演着重要角色。
焊接,换热器的专业英文 英翻中词汇 呕血翻译的总结

unfired shell and tube type heat exchangers. 未燃烧壳管式热交换器EXCHANGER UNIT 热交换器机组Purchaser's identification number 买方识别号thermal design specification sheet热设计规格单U- tube bundles U型管束U-bend portion U型弯管部分shell nozzle location 壳体接管位置GENERAL总则SCOPE 范围Specification 规范material/purchase requisition 材料或请购单and/or 和/或Equipment data sheet/DWG's 设备数据表/图纸Licensers standards/specifications and drawings 许可标准/规范和图纸Tubular Exchanger 管式换热器Boiler and Pressure Vessel Code 锅炉和压力容器规范Welding Electrodes 电焊条Spherical and Cylindrical Shells 球形和圆柱壳External Loading 外部负载Local load stress 局部荷载应力Uniform Building Code 统一建筑规范Steel Pipe Flanges 钢制管法兰Flanged Fittings 法兰配件Forged Steel Fittings锻钢配件Socket-welding and Threaded承插焊和螺纹Ring Joint环缘接合Spiral Wound and jacketed螺旋状伤用金属垫片Large Diameter Carbon Steel Flanges大直径碳钢法兰Expansion Joint 伸缩接头Nozzles 喷嘴tapered pipe threads锥管螺纹pipe fittings管件bolts 螺栓nuts 螺帽gasket 垫圈design, fabrication and testing 设计、制造和测试specifications, standards, drawings, provisions and codes 规格、标准、图纸、规定和规范longitudinal seam 纵向接缝circumferential seamspecific applicable item 具体适用项weld joint 焊缝automatic submerged arc process 自动埋弧过程integral cladding 整体电镀overlay 覆盖面、层weld metal 焊缝金属filler metal 填充金属,焊料filler rod 焊条eposited weld metal 堆积焊接金属joining base metal 接缝基底金属base metal 基底金属nominal composition 标定成分,标称化学成分dissimilar joint 异种接头austenitic stainless steel 奥氏体不锈钢check analysis 校检分析成品分析Production overlay weld composition 生产覆盖焊接成分Clad equipment 堆焊设备Lined equipment 线性设备Overlay equipment 覆面设备inert gas tungsten n arc process 惰性气体钨电弧焊过程pressure containing weldsweld overlaid vessels or vessel component 焊接覆盖容器或容器组件weld bead 焊缝Shell and head joint 壳头接头double-welded butt joints 双焊对接接头cladding 电镀,包层Back-up ring 支撑环back-up bar 备份栏fusion 熔接bevel 斜面。
换热温差 英文
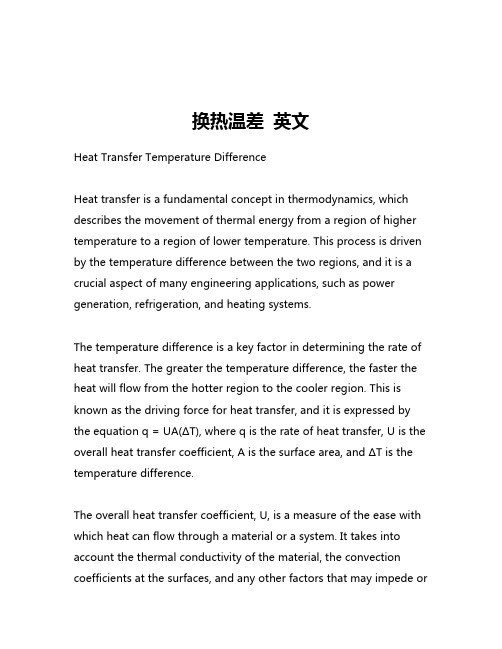
换热温差英文Heat Transfer Temperature DifferenceHeat transfer is a fundamental concept in thermodynamics, which describes the movement of thermal energy from a region of higher temperature to a region of lower temperature. This process is driven by the temperature difference between the two regions, and it is a crucial aspect of many engineering applications, such as power generation, refrigeration, and heating systems.The temperature difference is a key factor in determining the rate of heat transfer. The greater the temperature difference, the faster the heat will flow from the hotter region to the cooler region. This is known as the driving force for heat transfer, and it is expressed by the equation q = UA(ΔT), where q is the rate of heat transfer, U is the overall heat transfer coefficient, A is the surface area, and ΔT is the temperature difference.The overall heat transfer coefficient, U, is a measure of the ease with which heat can flow through a material or a system. It takes into account the thermal conductivity of the material, the convection coefficients at the surfaces, and any other factors that may impede orfacilitate the heat transfer process. The surface area, A, is the area through which the heat is being transferred, and it can have a significant impact on the rate of heat transfer.The temperature difference, ΔT, is the difference between the temperature of the hotter region and the temperature of the cooler region. This difference is the driving force that causes the heat to flow from the hotter region to the cooler region. The greater the temperature difference, the faster the heat will flow, and the greater the rate of heat transfer will be.One common application of heat transfer temperature difference is in the design of heat exchangers, which are devices used to transfer heat from one fluid to another. Heat exchangers are used in a wide range of applications, including power plants, refrigeration systems, and industrial processes. In a heat exchanger, the temperature difference between the two fluids is the driving force that causes the heat to flow from the hotter fluid to the cooler fluid.Another application of heat transfer temperature difference is in the design of insulation systems. Insulation is used to reduce the rate of heat transfer between two regions with different temperatures, such as the inside and outside of a building. The effectiveness of an insulation system is determined by the temperature difference across the insulation and the thermal resistance of the insulation material.In addition to these practical applications, the concept of heat transfer temperature difference is also important in the study of thermodynamics and the behavior of heat engines. In a heat engine, the temperature difference between the hot and cold reservoirs is the driving force that allows the engine to perform work and generate power.Overall, the heat transfer temperature difference is a fundamental concept in thermodynamics and engineering, and it has a wide range of practical applications in fields such as power generation, refrigeration, and heating systems. Understanding the principles of heat transfer temperature difference is essential for designing and optimizing a wide range of thermal systems and devices.。
常见换热器形式 英文缩写
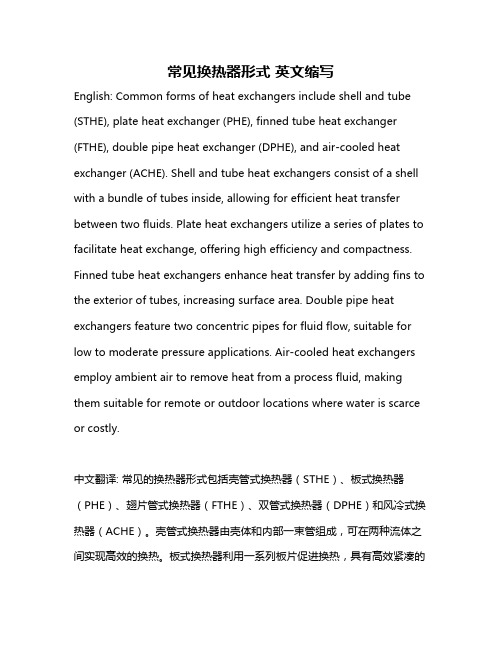
常见换热器形式英文缩写English: Common forms of heat exchangers include shell and tube (STHE), plate heat exchanger (PHE), finned tube heat exchanger (FTHE), double pipe heat exchanger (DPHE), and air-cooled heat exchanger (ACHE). Shell and tube heat exchangers consist of a shell with a bundle of tubes inside, allowing for efficient heat transfer between two fluids. Plate heat exchangers utilize a series of plates to facilitate heat exchange, offering high efficiency and compactness. Finned tube heat exchangers enhance heat transfer by adding fins to the exterior of tubes, increasing surface area. Double pipe heat exchangers feature two concentric pipes for fluid flow, suitable for low to moderate pressure applications. Air-cooled heat exchangers employ ambient air to remove heat from a process fluid, making them suitable for remote or outdoor locations where water is scarce or costly.中文翻译: 常见的换热器形式包括壳管式换热器(STHE)、板式换热器(PHE)、翅片管式换热器(FTHE)、双管式换热器(DPHE)和风冷式换热器(ACHE)。
全热交换英文缩写
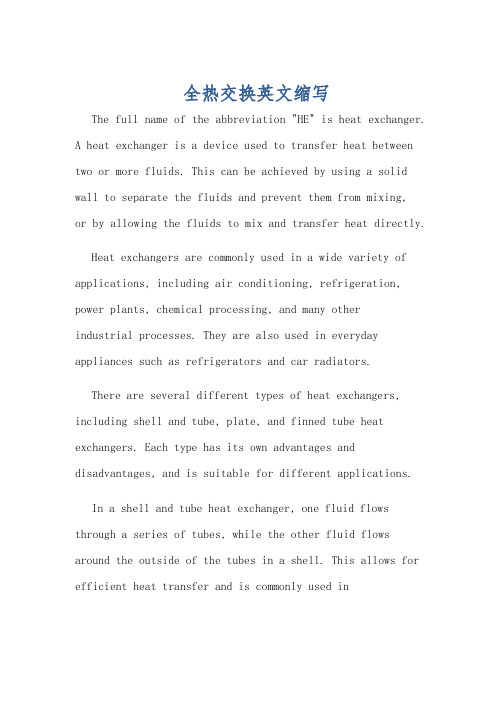
全热交换英文缩写The full name of the abbreviation "HE" is heat exchanger.A heat exchanger is a device used to transfer heat between two or more fluids. This can be achieved by using a solid wall to separate the fluids and prevent them from mixing,or by allowing the fluids to mix and transfer heat directly.Heat exchangers are commonly used in a wide variety of applications, including air conditioning, refrigeration, power plants, chemical processing, and many otherindustrial processes. They are also used in everyday appliances such as refrigerators and car radiators.There are several different types of heat exchangers, including shell and tube, plate, and finned tube heat exchangers. Each type has its own advantages and disadvantages, and is suitable for different applications.In a shell and tube heat exchanger, one fluid flows through a series of tubes, while the other fluid flows around the outside of the tubes in a shell. This allows for efficient heat transfer and is commonly used inapplications where one of the fluids is highly viscous or contains solid particles.Plate heat exchangers consist of a series of metal plates with narrow gaps between them. The fluids flow through the gaps, allowing for efficient heat transfer. Plate heat exchangers are compact and lightweight, making them suitable for applications where space is limited.Finned tube heat exchangers are similar to shell and tube heat exchangers, but with the addition of fins on the outside of the tubes. This increases the surface area available for heat transfer, making them suitable for applications where high heat transfer rates are required.Overall, heat exchangers play a crucial role in many industrial processes and everyday appliances, allowing for efficient heat transfer and temperature control."HE"的全称是热交换器。
板式换热英文简写

板式换热英文简写Plate heat exchangers, also known as plate-and-frame heat exchangers, are widely used in various industries for their high efficiency and compact size. These devices consist of a series of thin, corrugated plates that are stacked together with gaskets in between to create multiple channels for the fluid to flow through.板式换热器,也被称为板框式换热器,在各个行业被广泛使用,因其高效和紧凑的尺寸而备受青睐。
这种设备由一系列薄的波纹板组成,板与板之间夹有垫片,形成多个通道,供流体通过。
One of the main advantages of plate heat exchangers is their high heat transfer efficiency. The large surface area of the plates allows for a greater heat exchange between the two fluids, resulting in a more efficient transfer of heat. This makes plate heat exchangers ideal for applications where space is limited but high performance is required.板式换热器的主要优势之一是其高热传递效率。
板的大表面积使两种流体之间能够进行更大的热交换,从而实现更高效的热传递。
专业英语
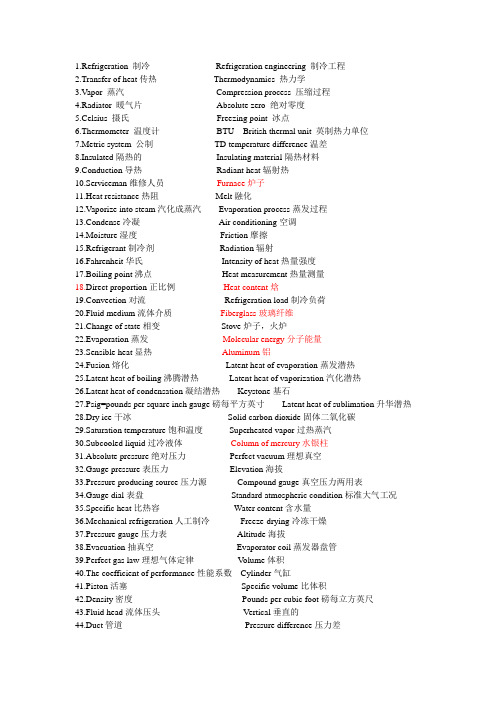
1.Refrigeration 制冷Refrigeration engineering 制冷工程2.Transfer of heat传热Thermodynamics 热力学3.Vapor 蒸汽Compression process 压缩过程4.Radiator 暖气片Absolute zero 绝对零度5.Celsius 摄氏Freezing point 冰点6.Thermometer 温度计BTU British thermal unit 英制热力单位7.Metric system 公制TD temperature difference温差8.Insulated隔热的Insulating material隔热材料9.Conduction导热Radiant heat辐射热10.Serviceman维修人员Furnace炉子11.Heat resistance热阻Melt融化12.Vaporize into steam汽化成蒸汽Evaporation process蒸发过程13.Condense冷凝Air conditioning空调14.Moisture湿度Friction摩擦15.Refrigerant制冷剂Radiation辐射16.Fahrenheit华氏Intensity of heat热量强度17.Boiling point沸点Heat measurement热量测量18.Direct proportion正比例Heat content焓19.Convection对流Refrigeration load制冷负荷20.Fluid medium流体介质Fiberglass玻璃纤维21.Change of state相变Stove炉子,火炉22.Evaporation蒸发Molecular energy分子能量23.Sensible heat显热Aluminum铝24.Fusion熔化Latent heat of evaporation蒸发潜热tent heat of boiling沸腾潜热Latent heat of vaporization汽化潜热tent heat of condensation凝结潜热Keystone基石27.Psig=pounds per square inch gauge磅每平方英寸Latent heat of sublimation升华潜热28.Dry ice干冰Solid carbon dioxide固体二氧化碳29.Saturation temperature饱和温度Superheated vapor过热蒸汽30.Subcooled liquid过冷液体Column of mercury水银柱31.Absolute pressure绝对压力Perfect vacuum理想真空32.Gauge pressure表压力Elevation海拔33.Pressure producing source压力源Compound gauge真空压力两用表34.Gauge dial表盘Standard atmospheric condition标准大气工况35.Specific heat比热容Water content含水量36.Mechanical refrigeration人工制冷Freeze-drying冷冻干燥37.Pressure gauge压力表Altitude海拔38.Evacuation抽真空Evaporator coil蒸发器盘管39.Perfect gas law理想气体定律V olume体积40.The coefficient of performance性能系数Cylinder气缸41.Piston活塞Specific volume比体积42.Density密度Pounds per cubic foot磅每立方英尺43.Fluid head流体压头Vertical垂直的44.Duct管道Pressure difference压力差45.Pipe管子,管道faucet水龙头46.Viscosity粘度Velocity速度47.Flow rate流量Pressure loss压力损失48.Connecting line连接管道Metal fins金属翘片minar flow层流Refrigeration process制冷过程pressor压缩机Performance性能51.Stroke行程Non-compressible不可压缩的52.Reciprocal倒数Naked eye裸视53.General term通用术语Fluid flow流体流动54.Tubing管件Water main水总管55.Sharp bends急转弯Micron微米56.Pressure drop压力降Manual手册57.Turbulent flow紊流Insulating film边界层58.Fittings配件Valves阀59.The walls of tube管壁Terminology术语60.Transport properties输送特性Pure fluid单组份流体61.Mixture and blend混合物和混合料Azeotropic refigeration mixtures共沸制冷剂62.Near-ARMs近共沸制冷剂Zeotropes非共沸制冷剂63.Subset子集Deviaton from偏离64.Bubble line泡点线Compression ratio压缩比65.Constant pressure phase state等压相变Propane丙烷66.Materials compatibility材料兼容性Thermodynamic performance热力学性能67.Flammability燃烧性Stability稳定性68.Environmental properties环境特性Proportion比例69.Sealed密封的Meter计量70.Guideline操作规矩Characteristics规格参数表71.Horsepower马力Working fluid工作流体72.Azeotropic point共沸点Dew line露点线73.Concentration浓度Lubricant润滑性74.Toxcity毒性Manufacturer制造商75.Leakage泄流Fractionation分馏76.Accumulator气液分离器Suction吸气ponent成分Refrigeration cycle制冷循环78.Receiver储液器Vapor compression system蒸汽压缩式系统79.Absorption system吸收式系统Centrifugal system离心式系统80.Simple compression refrigeration cycle单级压缩制冷循环Water cooled system水冷系统81.Cooling water冷却水Metering device节流装置82.TEV thermostatic expansion valve热力膨胀阀Power element bulb感温包83.Suction line吸气管Air cooled system空冷系统84.Fin-type condenser翘片式冷凝器Heat of compression压缩热pressor body压缩机机体Reciprocating compressor往复式压缩机86.Motor inefficiency电机损耗Accessible hermetic motor-compressor半封闭压缩机87.Volumetric efficiency容积效率Compression ratio压缩比88.Absolute discharge pressure绝对排气压力Absolute suction pressure绝对吸气压力pressor efficiency压缩机效率Clearance volume余隙容积90.Spring tension弹簧张力Discharge port排气孔91.Filter-drier干燥过滤器Orifice节流孔92.Discharge valve排气阀Valve plate阀板pressor capacity压缩机制冷能力Residual vapor余隙气体94.Discharge stroke排气行程Suction stroke吸气行程95.Reed簧片Two stage compressor两级压缩机96.Scroll compressor涡旋式压缩机compressor's pumping capacity压缩机输气量97.Displacement排量EER energy efficiency ratio能效比98.Condensing pressure冷凝压力Discharge pressure排气压力99.TXV热力膨胀阀Instantaneous boiling瞬间沸腾100.Flash gas闪发气体 A suction gas to liquid refrigerant heat exchanger回热器101.Offset补偿Crankcase曲轴箱pressor power consumption压缩机功耗Square root平方根103.Low stage cylinder低压级气缸High stage cylinder高压级气缸104.Interstage pressure中间压力Inter cooler 中间冷却器105.Clearance space余隙容积Re-expand再膨胀106.Curve曲线Liquid subcooler液体过冷剂107.Piston compressor活塞式压缩机Head pressure高压108.Flashing闪发Operating cost运行费t109.Solid liquid纯液体V ertical lift垂直提升110.COP=Coefficient of Performance性能系数screw 螺杆式111.Sight glass 视镜solenoid valve电磁阀112.A V AC= deep vacuum 真空度113.Oxygen and nitrogen 氧气和氮气hermetic motor-compressor 全封闭压缩机114.CFH=cubic foot per hour mechanical energy 机械能115.Guidelines for charging procedures冲注过程的操作手册116.Vapor-containing region包含气体的区域droplet 液滴117.Vapor phase 气相ternary三重的118.Non-ozone depleting 无臭氧消耗的glide 滑动。
传热专业英语对照表
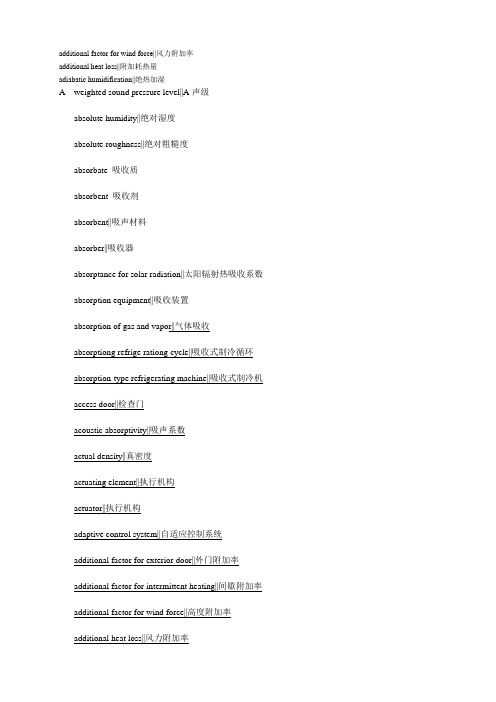
additional factor for wind force||风力附加率additional heat loss||附加耗热量adiabatic humidification||绝热加湿A-weighted sound pressure level||A声级absolute humidity||绝对湿度absolute roughness||绝对粗糙度absorbate 吸收质absorbent 吸收剂absorbent||吸声材料absorber||吸收器absorptance for solar radiation||太阳辐射热吸收系数absorption equipment||吸收装置absorption of gas and vapor||气体吸收absorptiong refrige rationg cycle||吸收式制冷循环absorption-type refrigerating machine||吸收式制冷机access door||检查门acoustic absorptivity||吸声系数actual density||真密度actuating element||执行机构actuator||执行机构adaptive control system||自适应控制系统additional factor for exterior door||外门附加率additional factor for intermittent heating||间歇附加率additional factor for wind force||高度附加率additional heat loss||风力附加率adiabatic humidification||附加耗热量adiabatic humidiflcation||绝热加湿adsorbate||吸附质adsorbent||吸附剂adsorber||吸附装置adsorption equipment||吸附装置adsorption of gas and vapor||气体吸附aerodynamic noise||空气动力噪声aerosol||气溶胶air balance||风量平衡air changes||换气次数air channel||风道air cleanliness||空气洁净度air collector||集气罐air conditioning||空气调节air conditioning condition||空调工况air conditioning equipment||空气调节设备air conditioning machine room||空气调节机房air conditioning system||空气调节系统air conditioning system cooling load||空气调节系统冷负荷air contaminant||空气污染物air-cooled condenser||风冷式冷凝器air cooler||空气冷却器air curtain||空气幕air cushion shock absorber||空气弹簧隔振器air distribution||气流组织air distributor||空气分布器air-douche unit with water atomization||喷雾风扇air duct||风管、风道air filter||空气过滤器air handling equipment||空气调节设备air handling unit room||空气调节机房air header||集合管air humidity||空气湿度air inlet||风口air intake||进风口air manifold||集合管air opening||风口air pollutant||空气污染物air pollution||大气污染air preheater||空气预热器air return method||回风方式air return mode||回风方式air return through corridor||走廊回风air space||空气间层air supply method||送风方式air supply mode||送风方式||air supply (suction) opening with slide plate||插板式送(吸)风口||air supply volume per unit area||单位面积送风量||air temperature||空气温度air through tunnel||地道风||air-to-air total heat exchanger||全热换热器air-to-cloth ratio||气布比air velocity at work area||作业地带空气流速air velocity at work place||工作地点空气流速air vent||放气阀air-water systen||空气—水系统airborne particles||大气尘air hater||空气加热器airspace||空气间层alarm signal||报警信号ail-air system||全空气系统all-water system||全水系统allowed indoor fluctuation of temperature and relative humidity||室内温湿度允许波动范围ambient noise||环境噪声ammonia||氨amplification factor of centrolled plant||调节对象放大系数amplitude||振幅anergy||@||angle of repose||安息角ange of slide||滑动角angle scale||热湿比angle valve||角阀annual [value]||历年值annual coldest month||历年最冷月annual hottest month||历年最热月anticorrosive||缓蚀剂antifreeze agent||防冻剂antifreeze agent||防冻剂apparatus dew point||机器露点apparent density||堆积密度aqua-ammonia absorptiontype-refrigerating machine||氨—水吸收式制冷机aspiation psychrometer||通风温湿度计Assmann aspiration psychrometer||通风温湿度计atmospheric condenser||淋激式冷凝器atmospheric diffusion||大气扩散atmospheric dust||大气尘atmospheric pollution||大气污染atmospheric pressure||大气压力(atmospheric stability||大气稳定度atmospheric transparency||大气透明度atmospheric turblence||大气湍流automatic control||自动控制automatic roll filter||自动卷绕式过滤器automatic vent||自动放气阀available pressure||资用压力average daily sol-air temperature||日平均综合温度axial fan||轴流式通风机azeotropic mixture refrigerant||共沸溶液制冷剂Bback-flow preventer||防回流装置back pressure of steam trap||凝结水背压力back pressure return余压回水background noise||背景噪声back plate||挡风板bag filler||袋式除尘器baghouse||袋式除尘器barometric pressure||大气压力basic heat loss||基本耗热量hend muffler||消声弯头bimetallic thermometer||双金属温度计black globe temperature||黑球温度blow off pipe||排污管blowdown||排污管boiler||锅炉boiller house||锅炉房boiler plant||锅炉房boiler room||锅炉房booster||加压泵branch||支管branch duct||(通风) 支管branch pipe||支管building envelope||围护结构building flow zones||建筑气流区building heating entry||热力入口bulk density||堆积密度bushing||补心butterfly damper||蝶阀by-pass damper||空气加热器〕旁通阀by-pass pipe||旁通管Ccanopy hood ||伞形罩capillary tube||毛细管capture velocity||控制风速capture velocity||外部吸气罩capturing hood ||卡诺循环Carnot cycle||串级调节系统cascade control system||铸铁散热器cast iron radiator||催化燃烧catalytic oxidation ||催化燃烧ceilling fan||吊扇ceiling panelheating||顶棚辐射采暖center frequency||中心频率central air conditionint system ||集中式空气调节系统central heating||集中采暖central ventilation system||新风系统centralized control||集中控制centrifugal compressor||离心式压缩机entrifugal fan||离心式通风机||check damper||(通风〕止回阀||check valve||止回阀||chilled water||冷水chilled water system with primary-secondary pumps||一、二次泵冷水系统chimney||(排气〕烟囱circuit||环路circulating fan||风扇circulating pipe||循环管circulating pump||循环泵clean room||洁净室cleaning hole||清扫孔cleaning vacuum plant||真空吸尘装置cleanout opening||清扫孔clogging capacity||容尘量close nipple||长丝closed booth||大容积密闭罩closed full flow return||闭式满管回水。
科技标准术语词典--换热
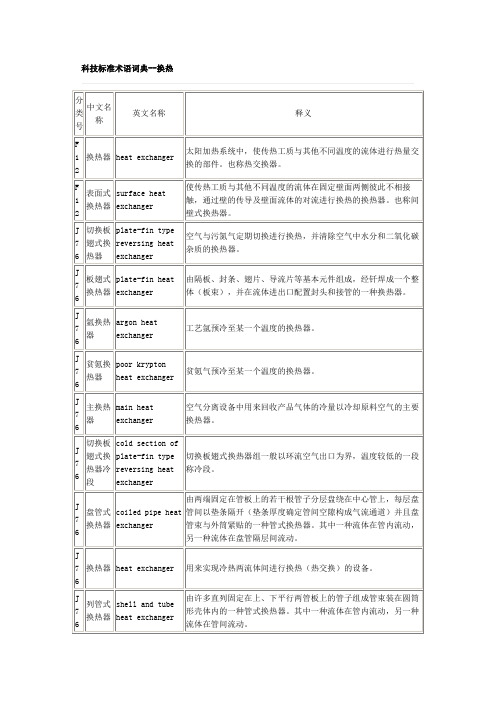
切换板翅式换热器
plate-fin type reversing heat exchanger
空气与污氮气定期切换进行换热,并清除空气中水分和二氧化碳杂质的换热器。
J76
板翅式换热器
plate-fin heat exchanger
由隔板、封条、翅片、导流片等基本元件组成,经钎焊成一个整体(板束),并在流体进出口配置9
夹套式换热器
jacket heat exchanger
板式热交换器的一种。在反应器或贮槽等容器外壁加一夹套,所形成的空间供载热体流通以进行加热和冷却。
X99
表面喷淋式换热器
surface jetting heat exchanger
管内热流体通过蛇管壁与管外喷淋的冷流体进行热交换的换热设备。
J76
切换板翅式换热器冷段
cold section of plate-fin type reversing heat exchanger
切换板翅式换热器组一般以环流空气出口为界,温度较低的一段称冷段。
J76
盘管式换热器
coiled pipe heat exchanger
由两端固定在管板上的若干根管子分层盘绕在中心管上,每层盘管间以垫条隔开(垫条厚度确定管间空隙构成气流通道)并且盘管束与外筒紧贴的一种管式换热器。其中一种流体在管内流动,另一种流体在盘管隔层间流动。
Y22
换热式熔炉
recuperative furnace
通过各种形式的换热器来预热助燃空气以回收烟气余热的熔炉。
Y22
辐射式换热器
radiation recuperator
warm section of plate-fin type reversing heat exchanger
换热器科技名词概念
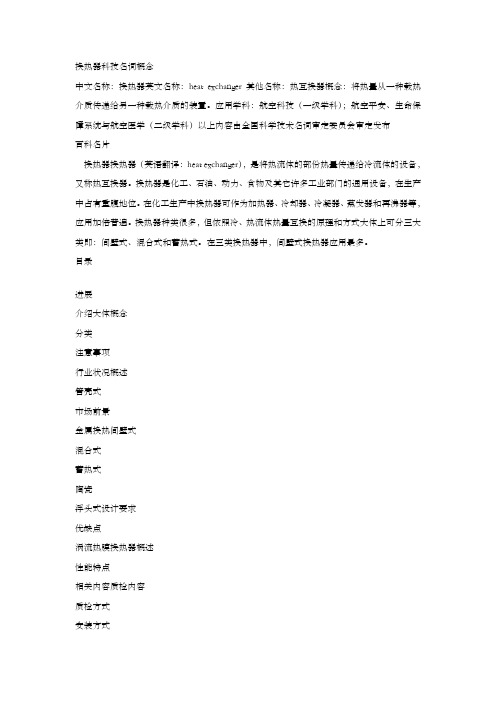
换热器科技名词概念中文名称:换热器英文名称:heat exchanger其他名称:热互换器概念:将热量从一种载热介质传递给另一种载热介质的装置。
应用学科:航空科技(一级学科);航空平安、生命保障系统与航空医学(二级学科)以上内容由全国科学技术名词审定委员会审定发布百科名片换热器换热器(英语翻译:heat exchanger),是将热流体的部份热量传递给冷流体的设备,又称热互换器。
换热器是化工、石油、动力、食物及其它许多工业部门的通用设备,在生产中占有重腹地位。
在化工生产中换热器可作为加热器、冷却器、冷凝器、蒸发器和再沸器等,应用加倍普遍。
换热器种类很多,但依照冷、热流体热量互换的原理和方式大体上可分三大类即:间壁式、混合式和蓄热式。
在三类换热器中,间壁式换热器应用最多。
目录进展介绍大体概念分类注意事项行业状况概述管壳式市场前景金属换热间壁式混合式蓄热式陶瓷浮头式设计要求优缺点涡流热膜换热器概述性能特点相关内容质检内容质检方式安装方式进展历史机组构造常见问题新型气动喷涂螺旋折流麻花管螺旋管式螺旋板式变声速压侵蚀防护侵蚀防护清洗注意事项系统查验防除垢管网清洁展开进展介绍大体概念分类注意事项行业状况概述管壳式市场前景金属换热间壁式混合式蓄热式陶瓷浮头式设计要求优缺点涡流热膜换热器概述性能特点相关内容质检内容质检方式安装方式进展历史机组构造常见问题新型气动喷涂螺旋折流麻花管螺旋管式螺旋板式变声速压侵蚀防护侵蚀防护清洗注意事项系统查验防除垢管网清洁展开编辑本段进展换热器是一种在不同温度的两种或两种以上流体间实现物料之间热量传递的节能设备,是使热量由较高的流体传递给温度较低的流体,使流体温度达到流程规定的指标,以知足进程工艺条件的需要,同时也提高能源利用率的要紧设备之一。
换热器行业涉及暖通、压力容器、中水处置设备等近30多种产业,彼此形成产业链条。
据《2021-2017年中国换热器行业进展前景预测与转型升级分析报告》[1]数据显示2020年中国换热器产业市场规模在500亿元左右,要紧集中于石油、化工、冶金、电力、船舶、集中供暖、制冷空调、机械、食物、制药等领域。
- 1、下载文档前请自行甄别文档内容的完整性,平台不提供额外的编辑、内容补充、找答案等附加服务。
- 2、"仅部分预览"的文档,不可在线预览部分如存在完整性等问题,可反馈申请退款(可完整预览的文档不适用该条件!)。
- 3、如文档侵犯您的权益,请联系客服反馈,我们会尽快为您处理(人工客服工作时间:9:00-18:30)。
examples of passive augmentation are surface roughness, displaced promoters, and vortex generators. Surface roughness--introduced through knurling or threading or formed by repeated r i b s - - p r o m o t e s augmentation through the disturbance of the sublayer that is close to the surface. These methods are used to improve heat-transfer coefficients inside tubes [2] or outside tubes or rods [3]. Displaced promoters include inserts that alter flow mechanics near the surface by disturbing the core flow. Examples are baffles [4] and mixing elements [5]. Vortex flow can be created through coiled wires [6], stationary propellers [7], or twisted tapes [8]. Active augmentation, which has also been studied extensively, requires the addition of external
Address correspondence to Professor T. S. Ravigururajan, Department of Mechanical Engineering, Wichita State University, Wichita, KS 67260-0035. E-mail: ravi@
INTRODUCTION Thermal system performance and efficiency can be vastly improved, thereby reducing the size of heat exchangers. The augmentation (or enhancement) of heat transfer has become an important factor in achieving these goals [1]. The performance of heat exchangers, for single-phase flows in particular, can be improved by many active and passive augmentation techniques. The former uses surface modification or an additional device incorporated into the equipment. The existing boundary layer is disturbed and the heat-transfer performance is improved, usually with an increase in the flow friction and pressure drop. Typical
Experimental Thermal and Fluid Science 1996; 13:55-70
© Elsevier Science Inc., 1996 655 Avenue of the Americas, New York, NY 10010
0894-1777/96/$15.00 PII S0894-1777(96)00014-3
Department of Mechanical Engineering Wichita State University Wichita, Kansas 67260 USA
• General correlations are developed and verified for friction factor and heat-transfer coefficient for single-phase turbulent flow in internally augmented tubes. Data from frequently referred investigations were gathered for a wide range of tube parameters with e/d, 0.01 to 0.2; p / d , 0.1 to 7.0; a/90, 0.3 to 1.0, and flow parameters Re, 5000 to 250,000 and Pr, 0.66 to 37.6. The data were applied to a linear model to obtain normalized correlations that were then modified to approach smooth tube correlation as the roughness variables became very small. A shape function was included in the friction correlation to account for different rib profiles. These correlations were then verified by independent experiments. Different types of commercial fibbed tubes were tested with water as working fluid under heating conditions. Five wire coil inserts were tested in another apparatus using air under cooling conditions. The parameters tested were Re = 5000-60,000, e / d = 0.023-0.127, p / d = 0.17-1.12, and a/90 = 0.34-0.91. The results proved that the correlations can be successfully applied to a wide range of roughness types and Prandtl number. The present correlations are superior, for this large database, to those presented by other investigators. The friction factor correlation predicts 96% of the database to within + 50% and 77% of the database to within + 20%. Corresponding prediction figures for the heat-transfer correlation are 99 and 69%. Keywords: heat-transfer enhancement, correlations, augmented tubes,
A. E. Bergles
Department of Mechanical Engineering Aeronautical Engineering and Mechanics Renssalaer Polytechnic Institute Troy, New York 12180, USA
wire coils
56 T.S. Ravigururajan and A. E. Bergles power to bring about the desired flow modification. Examples include heat-transfer surface vibration [9], fluid vibration [10], and electrostatic field introduction [11]. Of the several methods discussed above, the most popular and successful technique has been augmentation through surface roughness. This is mainly because of its effectiveness in enhancing the heat transfer and its simplicity in application. Transverse or helical repeated ribs* are an especially attractive way of creating the surface roughness. Ribs are found in several configurations, depending on the manufacturing technique (machining, forming, or insert). Figure 1 presents sketches of the various types of ribbed tubes along with the important geometrical parameters involved. Tubes with transverse ribs of rectangular profile shape are fabricated in segments, each of which is machined from a smooth pipe on a lathe. These segments are fitted over a long mandrel and brazed together to obtain any desired length. But this method of fabrication is suitable only for laboratory testing. Production on a large scale may be carried out through rolling or indenting, which produces ribs having a smooth profile. High production rates usually dictate helical ribs; a feed is given to the tube to form the desired helix angle. Here the roughness height, pitch, helix angle, and to a large extent, the profile shapes are controlled by the roller design, the feed rate, and the pressure applied * Ribbed tubes are also commonly referred to as "spirally fluted," "convoluted," "corrugated," "spiral," "helically fluted," "spirally grooved," "spirally indented," "roped," or "enhanced." during the process. Another simple method of introducing roughness in a tube is to insert wire coils, in which case the roughness height equals the wire diameter. The various investigations carried out on these augmented tubes are listed in Ref. [1], and patents describing the fabrication methods are included in [12]. Ribbed tubes are sometimes considered in the design of shell-and-tube heat exchangers. The applications include single-phase tubeside augmentation of flooded chillers for air conditioners [13], surface condensers for desalting plants [14], and surface condensers for power plants [15]. The size of these heat exchangers can be reduced considerably by use of augmented tubes instead of smooth tubes; alternatively, the heat duty of a fixed area heat exchanger can be increased. This has important potential applications in large systems such as those used in thermal, geothermal, and ocean thermal energy conversion (OTEC) power plants, which require very large heat exchangers. BACKGROUND In spite of the increasing use of these augmented tubes, there are no truly general correlations available for quantitative assessment of pressure drop and heat transfer. In one of the early studies on ribbed tubes, Kalinin et al. [16] proposed correlations for pressure drop and heat transfer. However, the correlations are valid only for transverse ribs within specific ranges of e/d and p/d. Many of the studies found in the literature have tried to extend the studies of Nikuradse [17] on friction with sand-grain roughness and the heat-transfer-momentum-