-液相烧结(课堂PPT)
无机材料科学基础-第9章-烧结ppt课件

2、颗粒越小,烧结推动力越大;
粉末体紧密堆积以后,颗粒间仍有很多细小气孔通过,在这 些弯曲的表面上由于表面张力的作用而造成的压力差为
其中γ- 粉末体表面张力;r-粉末球形半径. 若为非球形曲面,可用两个主曲率r1和r2表示
以上两个公式表明,弯曲表面上的附加压力与球形颗粒(或曲 面)曲率半径成反比,与粉料表面张力成正比。
烧结与固相反应区别:
相同点:两个过程均在低于材料熔点或熔融温 度之下进行,并且在过程的自始至终都至少有 一相是固态。
不同点:固相反应发生化学反应,固相反应必 须至少有两组元参加如A和B,最后生成化合 物AB,AB结构与性能不同于A与B。 而烧结不发生化学反应,可以只有单组元; 或者两组元参加,但两组元并不发生化学反应, 仅仅是在表面能驱动下,由粉体变成致密体。
四、烧结模型(model)
1、中心距 不变的双球 模型,如图 9—3(A);
四、烧结模型(model)
2、中心距 缩短的双球 模型,如图 9—3(B)。
四、烧结模型(model)
3、球板模 型(中心距 缩短),如 图9—3(C)。
四、烧结模型(model)
以上三个模型对烧结初期一般是 适用的,但随烧结的进行,球形 颗粒逐渐变形,因此在烧结中、 后期应采用其它模型。
两个过程不同之处是固相反应必须至少有两 组元参加如A和 B,并发生化学反应,最后 生成化合物AB。AB结构与性能不同于A与 B。
二、与烧结有关的一些概念
3、 烧结与固相反应。
而烧结可以只有单组元,或者两组元参加, 但两组元并不发生化学反应。仅仅是在表面 能驱动下,由粉体变成致密体。固态物质烧 结时,会同时伴随发生固相反应或局部熔融 出现液相。实际生产中,烧结、固相反应往 往是同时穿插进行的。
第五章 烧结ppt课件

rs n rs0 n kt
式中,rs为在时间t时的晶粒平均半径,为在时间为0时的晶粒平均 半径,k为晶粒生长速率常数。半径(或晶料尺寸)指数n取决于晶粒 生长机理;n=3和n=2分别为扩散控制相界面反应控制。
fes4fes7o上述反应必须完成于釉层封闭之前5008508501050730950350450500800250920600350082气孔率高2540应力缓冲573高温阶段950烧成温度长石瓷烧成温度1350氧化保温期9501020低速升温或保温加速气流与空气过剩系数保证上述氧化分解与脱水进行完全彻底玻化成瓷期1020烧成温度液相的提前出现高岭土长石石英的最低共熔点985由于camg杂质液相会在950就出现液相对成瓷的促进作用1液相促进晶体生长2al92595010502液相促进坯体致密化玻璃相达4050玻化成瓷期内的收缩曲线玻化成瓷期内的两个阶段15105tatb玻化成瓷期内的收缩曲线t开始烧结温度ta烧结温度下限tb烧结温度上限过烧温度tatb烧结温度范围tb玻化成瓷范围一般情况
优点: 1)提高烧结驱动力。 2)可制备具有控制的微观结构和优化性能
的陶瓷复合材料
.
5.4.1 液相烧结的阶段
(a)液相烧结不同阶段的示意图 (O:熔化;Ⅰ:重排;Ⅱ:溶解-沉 淀;及Ⅲ:气孔排除)。
(b)在不同温度下,氧化铝-玻 璃体系中,实际致密化作为烧 结时间的函数所示 意的不同LPS阶段
.
• 氧化反应
✓参与物: 坯体内的碳素、有机物、硫化物
✓氧化反应:
350~450℃
FeS2+O2
FeS+SO2
4FeS +7O2500~800℃ 2Fe2O3+4SO2
S+O2 250~920℃SO2
第三章烧结过程优秀课件
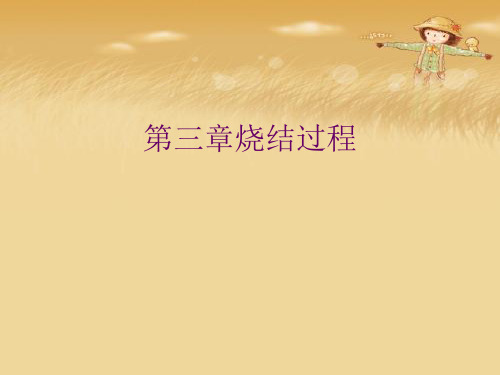
烧结是在远低于固态物质的熔融温度下进行。
泰曼发现烧结温度(TS)和熔融温度(TM)之间 关系有一定规律:
金属粉末:TS(0.3~0.4)TM 盐 类:TS0.57TM 硅 酸 盐:TS(0.8~0.9)TM 熔融时系统中全部组元都转变为液相,烧结时至 少有一种组元处于固态
1.烧结与烧成: 烧成包括多种物理和化学变化,如脱水,坯体 内气体分解,多相反应和熔融,溶解,烧结等 烧结仅仅指粉料经加热而致密化的简单物理过 程,仅仅是烧成过程一个重要组成部分 烧成的含义及包括的范围更广,如普通陶瓷制 备过程中从坯体进入隧道窑到制品离开隧道窑 的整个过程可称为陶瓷的烧成
2.烧结与熔融:
颗粒中心距逼近,逐渐形成晶
电阻
界,气孔形状产生变化,体积 性
缩小,并逐渐从连通的气孔变 成孤立的气孔,气孔逐渐缩小,
质
密度 强度
直至最后大部分甚至全部气孔
从晶体中排除。
➢伴随这些物理变化,坯体中
的气孔率下降,密度、强度增
温度
加,电阻率下降,晶粒据烧结过程是否施加压力分类: ➢不施加外部压力的无压烧结(Pressureless Sintering) ➢施加额外外部压力的加压烧结(Applied Pressure or Pressure-assisted Sintering)
cd.随传质继续,粒界进一步扩大,气孔则逐渐缩 小和变形,最终变成孤立闭气孔。同时,颗粒粒界 开始移动,粒子长大,气孔逐渐迁移到粒界上消失 ,致密度提高
❖烧结过程伴随的主要物理过 程:
➢粉料成型后形成具有一定外
形的坯体,坯体中包含百分之
-液相烧结

液相烧结的早期观点:液相的作用主要是一种物理过程,液相出现时由于毛细管压液相的作用主要是种物理过程液相出现时由于毛细管压力的作用,将引起坯体中粉粒或尖凸部分在液相中溶解,通过扩散而在另一部分的淀析;由于润湿作用而使固-液界面能下降。
Ref: 网络课件液相烧结的早期观点:液相的作用主要是一种物理过程,液相出现时由于毛细管压液相的作用主要是种物理过程液相出现时由于毛细管压力的作用,将引起坯体中粉粒或尖凸部分在液相中溶解,通过扩散而在另一部分的淀析;由于润湿作用而使固-液界面能下降。
¾近期的研究发现:近期的研究发现在绝大多数情况下,液相烧结都伴随着不同形式的化学反应。
这种化学反应的作用力远比毛细管压力大,其所引起的体系自由能的下降,也比表面(包括界面)自由能的下降要大几个数量级,的降也表括自的降大个数故有人将这类有反应作用的烧结称之为活化烧结(Activated sintering)。
§3-6 液相与活化烧结一、液相烧结中的物理作用过程前提条件:¾体系中必须具有一定的液相含量;体系中必须具有一定的液相含量¾固相物质在液相中必须具有明显的溶解度;¾液相必须能较好地润湿固相物质。
产生的物理效应:1.润滑效应——液相对固粒的润滑作用,使粉粒之间的摩擦减小,便于粉粒作相对运动,可使成型时留下的内应力下降,粉粒堆集度也有所改善。
2.毛细管压力与粉粒的初次重排当液相能很好润湿固相时,粉粒间的大多数空隙都将被液相填充,形成毛细管状液膜。
直径为0.1-1微米的毛细管在可在般陶瓷粉粒间产生110Mpa左右的压强,如此大的压可在一般陶瓷粉粒间产生1-10Mpa强加上液相的润滑作用,使胚体成型后粉粒重新排布,达到更紧密的空间堆积。
这被称为烧结过程中的初次重排。
5.熟化与外形适应(Ripening with shape accommodation)——奥氏熟化(Ostwald Ripening)d ti)奥氏熟化(O t ld Ri i)在可传质媒介中的颗粒大现象在可传质媒介中的颗粒长大现象。
液相烧结 ppt课件
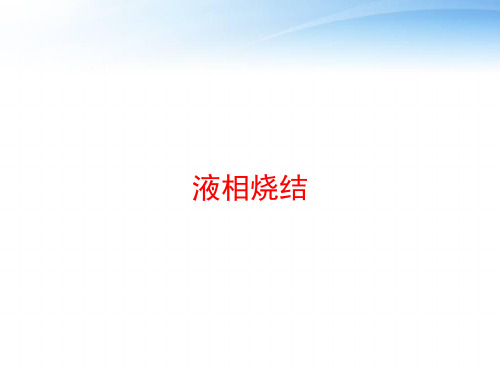
,
又
KTd2 8D*
8D* KTd2
❖ 粘性蠕变产生区域:晶界区域、位错区域
动力学关系:
❖烧结模型:双球模型(中心距缩短)
❖颈部增长公式:
x
(3
1
)2
1
r 2
tHale Waihona Puke 1 2r 2❖体收缩:
V3L 9 t V L 4r
❖麦肯基导出适合粘性流动传质全过程的烧结速率公
式:
d 3 (1)
dt 2 r
θ—相对密度,即体积密度d/理论密度d0
1.颗粒在接触点处溶解到自由表面上沉积
2.小晶粒溶解至大晶粒处沉淀
原理:由于颗粒接触点处(或小晶粒)在液 相中的溶解度大于自由表面(或大晶粒)处 的溶解度
❖线收缩:
1
L L K t 3
4 1
L L r 3 t 3
当T,r一定时,
❖ 4. 影响因素: ❖颗粒起始粒度 ❖粉末特性(溶解度、润湿性) ❖液相数量 ❖烧结温度
产生的传质称为粘性流动传质(或粘性蠕变传质)。
在固态烧结中,晶体内的晶格空位在应力作用下, 由空位的定向流动引起的形变称为粘性蠕变
粘性蠕变与扩散传质的区别:粘性蠕变是在应力 作用下,整排原子沿着应力方向移动,而扩散传 质仅是一个质点的迁移
❖ 粘性蠕变通过粘度系数(η)把粘性蠕变速率(ε)
与应力(σ)联系起来
三. 不同烧结及传质机理比较
❖ 1. 固相烧结与液相烧结的异同点:
⑴ 相同点:
❖烧结的推动力都是表面能
❖烧结过程都包括颗粒接触、聚集、重排,气孔填充、 排出和晶粒生长等阶段
⑵ 不同点:
❖液相烧结比固相烧结传质速率高,因而液相烧结致 密化速率比固相烧结快,可以在更低的温度下使坯 体烧结成致密体
3-6液相烧结教程
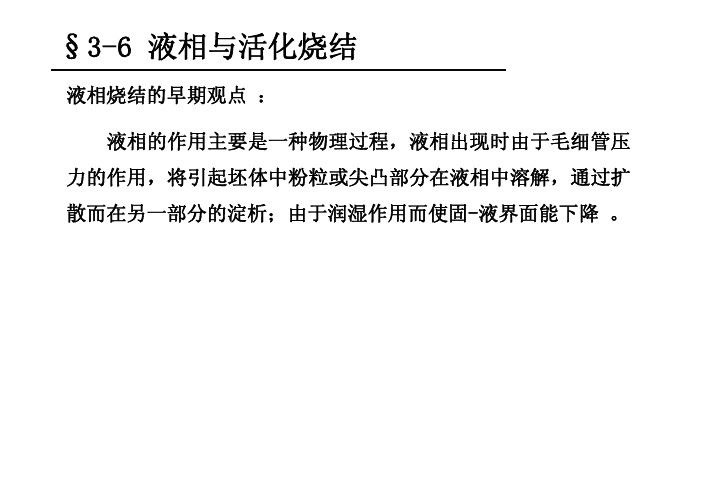
液相烧结的早期观点:液相的作用主要是一种物理过程,液相出现时由于毛细管压液相的作用主要是种物理过程液相出现时由于毛细管压力的作用,将引起坯体中粉粒或尖凸部分在液相中溶解,通过扩散而在另一部分的淀析;由于润湿作用而使固-液界面能下降。
Ref: 网络课件液相烧结的早期观点:液相的作用主要是一种物理过程,液相出现时由于毛细管压液相的作用主要是种物理过程液相出现时由于毛细管压力的作用,将引起坯体中粉粒或尖凸部分在液相中溶解,通过扩散而在另一部分的淀析;由于润湿作用而使固-液界面能下降。
¾近期的研究发现:近期的研究发现在绝大多数情况下,液相烧结都伴随着不同形式的化学反应。
这种化学反应的作用力远比毛细管压力大,其所引起的体系自由能的下降,也比表面(包括界面)自由能的下降要大几个数量级,的降也表括自的降大个数故有人将这类有反应作用的烧结称之为活化烧结(Activated sintering)。
§3-6 液相与活化烧结一、液相烧结中的物理作用过程前提条件:¾体系中必须具有一定的液相含量;体系中必须具有一定的液相含量¾固相物质在液相中必须具有明显的溶解度;¾液相必须能较好地润湿固相物质。
产生的物理效应:1.润滑效应——液相对固粒的润滑作用,使粉粒之间的摩擦减小,便于粉粒作相对运动,可使成型时留下的内应力下降,粉粒堆集度也有所改善。
2.毛细管压力与粉粒的初次重排当液相能很好润湿固相时,粉粒间的大多数空隙都将被液相填充,形成毛细管状液膜。
直径为0.1-1微米的毛细管在可在般陶瓷粉粒间产生110Mpa左右的压强,如此大的压可在一般陶瓷粉粒间产生1-10Mpa强加上液相的润滑作用,使胚体成型后粉粒重新排布,达到更紧密的空间堆积。
这被称为烧结过程中的初次重排。
5.熟化与外形适应(Ripening with shape accommodation)——奥氏熟化(Ostwald Ripening)d ti)奥氏熟化(O t ld Ri i)在可传质媒介中的颗粒大现象在可传质媒介中的颗粒长大现象。
粉末成形与烧结讲义第四部分课件

(3)继续升温到烧结温度及保温阶段 超过共晶温度继续升温,有更多WC 溶解列液相中,液相数量剧增;保温过程中, WC继续溶解到液相中,继续保 温只发生WC通过液相的溶解和再析出过程,WC晶粒逐渐长大,而两相的成分 和比例都维持不变。
热压致密化理论是在粘性或塑性流动烧结理论的基础上建立,并主 要沿着两个方向发展:(1)热压的动力学即致密比方程式,分为理论的 和经验的两类,前者由塑性流动理论和扩散蠕变理论寻出;(2)热压的 致密化机构,包括颗粒相互滑过、颗粒的破碎、塑性变形以及体积扩散 等。
热压烧结的特点:
1. 所需的成型压力仅为冷压法的1/10; 2. 降低烧结温度和缩短烧结时间,抑制了
液相烧结的机构表明,当固相的原子溶解于液相(粘 结相)时致密化速度增加,烧结所需时间缩短,从这个 意义上讲,能在烧结温度下形成液相的就可用作活化 烧结的添加元素。
但是,对于W—Cu—Ni重合金,当Cu与Ni比为1 /2.5时,合金在低于Cu-Ni熔点的温度1050℃烧 结,烧结后可以看到钨颗粒形成明显的卵形结构,并 有明显的体积收缩。
液相烧结过程
液相烧结不同阶段的示意图(O:熔化;Ⅰ:重排;Ⅱ:溶解-沉淀;及Ⅲ:固相烧结)
(1)颗粒重排(Particles Re-arrangement) 在液相烧结过程中,颗粒间的液相膜起润滑作用。颗粒重排向减 少气孔的方向进行,同时减小系统的表面自由能。当坯体的密度 增加时,由于周围颗粒的紧密接触,颗粒进一步重排的阻力增加, 直至形成紧密堆积结构。
四、热压烧结
热压烧结(hot pressing)是在烧结过程中同时对坯料施加压力, 加速了致密化的过程。所以热压烧结的温度更低,烧结时间更短。
第10章 液相烧结
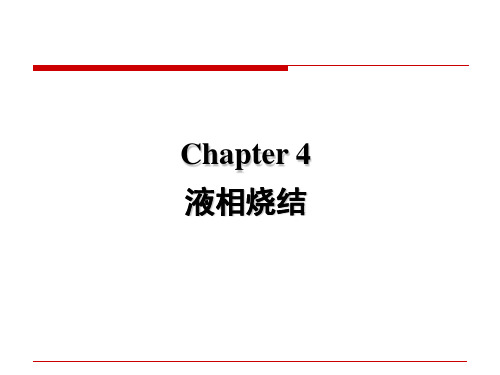
10.5超固相线烧结
10.6熔渗
要想成功地进行溶渗,必须注意以下几点: ①固态骨架与熔渗液体之间不得发生反应形成 化合物,或在熔渗完成之前堵塞孔道。 ②多孔性骨架中的孔洞必须是连通的,因为一 般熔渗不可能浸入到封闭孔隙中。 ③骨架材料在液态熔渗剂中的溶解度应为零或 尽可能低。 ④骨架材料的晶界与液相交汇处的两面角应当 小,但不能太小。
10.1基本概念
1.接触角和两面角
(1)接触角
10.1基本概念
1.接触角和两面角
(2)两面角
2 arccos( SS 2 ) SL
10.1基本概念
1.接触角和两面角
(3)与溶解度的关系
对于液相烧结系统,要获得满意的致密化效果, 固相在液相中的溶解度特别重要。如果烧结的 粉末互不相溶,致密化过程将进行缓慢,甚至 不会达到完全致密。 若希望在液相烧结时实现致密化,则液相在固 相中的溶解度是有害的。另一方面,在生产具 有数量可控的孔隙度的压坯时,溶解度具有重 要的作用。
10.5超固相线烧结
超固相线烧结是另外一种特殊的瞬时液 相烧结。在超固相线烧结中,使用的原料粉 不是元素粉末混合粉,而是水雾化预合金化 粉末,每个粉末颗粒的化学成分相同。将预 合金化粉末压制成形,经烧结制成零件。烧 结温度设定左合金的液相线和固相线之间。 这时,每个颗粒内部都有液相形成。液相出 现时,液相的铺展将导致颗粒破碎、重排, 致使液相均匀分布,压制成形的压坯中的颗 粒间孔隙消失,达到了致密化。
10.3烧结硬质合金的生产工艺
2.硬质合金生产
(3)成形
制得的混合料通常用冷压制成形为生压坯,偶尔也采 用等静压成形。需要大量同一尺寸的压坯时,例如切削刀 具的刀片,可在自动压机上用硬质合金模具压制成形,一 般压制压力较低,为100~200MPa。
四、烧结.ppt
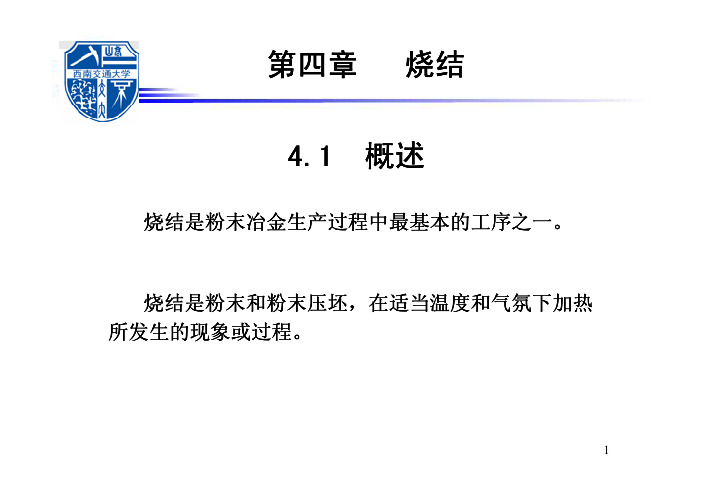
1第四章烧结4.1 4.1 概述概述烧结是粉末冶金生产过程中最基本的工序之一烧结是粉末冶金生产过程中最基本的工序之一。
烧结是粉末和粉末压坯烧结是粉末和粉末压坯,,在适当温度和气氛下加热所发生的现象或过程所发生的现象或过程。
2按烧结过程有无明显的液相出现和烧结系统的组成分为和烧结系统的组成分为::1)单元系烧结2)多元系固相烧结3) 3) 多元系液相烧结多元系液相烧结3粘结阶段颗粒的原始接触点或面转变成晶体结合颗粒的原始接触点或面转变成晶体结合,,即通过成核即通过成核、、结晶长大等原子过程形成烧结颈等原子过程形成烧结颈。
烧结体密度烧结体密度、、烧结体强度烧结体强度、、导电性等的变化烧结颈长大阶段原子向颗粒结合面迁移原子向颗粒结合面迁移,,烧结颈扩大烧结颈扩大,,颗粒间距缩小颗粒间距缩小,,晶粒长大,晶界越过孔隙移动晶界越过孔隙移动。
烧结体密度烧结体密度、、烧结体强度等的变化闭孔隙球化和缩小阶段烧结体致密度达到烧结体致密度达到90%90%90%以上以上以上,,孔隙闭合后孔隙闭合后,,孔隙形状趋于球形并缩小缩小。
4.2 4.2 烧结的基本过程烧结的基本过程41)烧结为什么会发生烧结为什么会发生??2)烧结是怎样进行的烧结是怎样进行的??4.34.3 烧结理论的两个最基本的问题51)烧结为什么会发生烧结为什么会发生??烧结是系统自由能减低的过程。
•由于颗粒结合面的增大和颗粒表面的平直化,粉末体的总表面积和总表面自由能减小•粉末体内孔隙的总体积和总表面积减小•粉末内晶格畸变的消除62)烧结是怎样进行的烧结是怎样进行的??烧结的机构和动力学问题,研究烧结过程中各种物质迁移方式以及速率。
7单元系烧结是指:纯金属或有固定成分的化合物的粉末在固态下的烧结,不会出现新组成物或者新相,也不会出现凝聚状态的改变。
4.4 4.4 单元系烧结单元系烧结8一、烧结温度和时间•单元系的烧结主要机构是扩散和流动构是扩散和流动。
铝合金的液相烧结
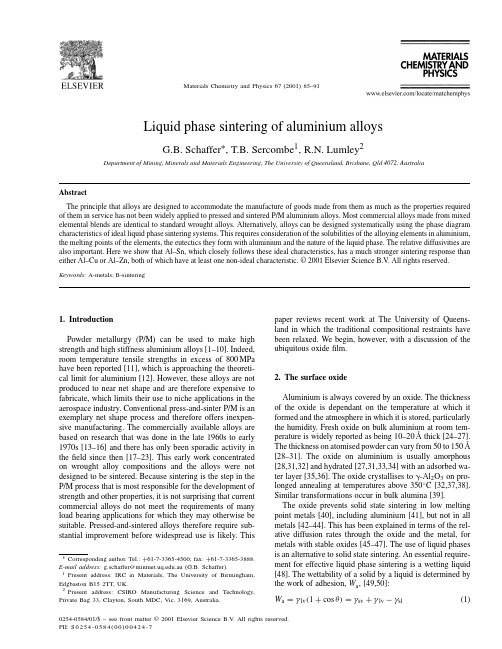
Materials Chemistry and Physics67(2001)85–91Liquid phase sintering of aluminium alloysG.B.Schaffer∗,T.B.Sercombe1,R.N.Lumley2Department of Mining,Minerals and Materials Engineering,The University of Queensland,Brisbane,Qld4072,AustraliaAbstractThe principle that alloys are designed to accommodate the manufacture of goods made from them as much as the properties required of them in service has not been widely applied to pressed and sintered P/M aluminium alloys.Most commercial alloys made from mixed elemental blends are identical to standard wrought alloys.Alternatively,alloys can be designed systematically using the phase diagram characteristics of ideal liquid phase sintering systems.This requires consideration of the solubilities of the alloying elements in aluminium, the melting points of the elements,the eutectics they form with aluminium and the nature of the liquid phase.The relative diffusivities are also important.Here we show that Al–Sn,which closely follows these ideal characteristics,has a much stronger sintering response than either Al–Cu or Al–Zn,both of which have at least one non-ideal characteristic.©2001Elsevier Science B.V.All rights reserved. Keywords:A-metals;B-sintering1.IntroductionPowder metallurgy(P/M)can be used to make high strength and high stiffness aluminium alloys[1–10].Indeed, room temperature tensile strengths in excess of800MPa have been reported[11],which is approaching the theoreti-cal limit for aluminium[12].However,these alloys are not produced to near net shape and are therefore expensive to fabricate,which limits their use to niche applications in the aerospace industry.Conventional press-and-sinter P/M is an exemplary net shape process and therefore offers inexpen-sive manufacturing.The commercially available alloys are based on research that was done in the late1960s to early 1970s[13–16]and there has only been sporadic activity in thefield since then[17–23].This early work concentrated on wrought alloy compositions and the alloys were not designed to be sintered.Because sintering is the step in the P/M process that is most responsible for the development of strength and other properties,it is not surprising that current commercial alloys do not meet the requirements of many load bearing applications for which they may otherwise be suitable.Pressed-and-sintered alloys therefore require sub-stantial improvement before widespread use is likely.This ∗Corresponding author.Tel.:+61-7-3365-4500;fax:+61-7-3365-3888. E-mail address:g.schaffer@.au(G.B.Schaffer).1Present address:IRC in Materials,The University of Birmingham, Edgbaston B152TT,UK.2Present address:CSIRO Manufacturing Science and Technology, Private Bag33,Clayton,South MDC,Vic.3169,Australia.paper reviews recent work at The University of Queens-land in which the traditional compositional restraints have been relaxed.We begin,however,with a discussion of the ubiquitous oxidefilm.2.The surface oxideAluminium is always covered by an oxide.The thickness of the oxide is dependant on the temperature at which it formed and the atmosphere in which it is stored,particularly the humidity.Fresh oxide on bulk aluminium at room tem-perature is widely reported as being10–20Åthick[24–27]. The thickness on atomised powder can vary from50to150Å[28–31].The oxide on aluminium is usually amorphous [28,31,32]and hydrated[27,31,33,34]with an adsorbed wa-ter layer[35,36].The oxide crystallises to␥-Al2O3on pro-longed annealing at temperatures above350◦C[32,37,38]. Similar transformations occur in bulk alumina[39].The oxide prevents solid state sintering in low melting point metals[40],including aluminium[41],but not in all metals[42–44].This has been explained in terms of the rel-ative diffusion rates through the oxide and the metal,for metals with stable oxides[45–47].The use of liquid phases is an alternative to solid state sintering.An essential require-ment for effective liquid phase sintering is a wetting liquid [48].The wettability of a solid by a liquid is determined by the work of adhesion,W a,[49,50]:W a=γlv(1+cosθ)=γsv+γlv−γsl(1)0254-0584/01/$–see front matter©2001Elsevier Science B.V.All rights reserved. PII:S0254-0584(00)00424-786G.B.Schaffer et al./Materials Chemistry and Physics67(2001)85–91 whereγlv is the surface tension of the liquid–vapour inter-face,γsv the surface tension of the solid–vapour interface,γsl the solid–liquid interfacial tension andθthe contactangle.A liquid is said to wet a solid when cosθ>0.Highmelting point metal oxides are generally poorly wetted byliquid metals,except above the wetting threshold,a tem-perature beyond which W a increases sharply[50].Liquidaluminium is not therefore expected to wet alumina nearthe melting point of the metal.Indeed,the contact angle isvariously given as∼103◦at900◦C[51],∼160◦at800◦C[52]or∼162◦at950◦C[53],although this is dependant onthe partial pressure of oxygen and the presence of an oxidefilm on the molten metal[54].It has been suggested that theAl–CuAl2eutectic can wet Al2O3at600◦C[19].However,neither Mg,Ce nor Ca additions to molten Al reduce thecontact angle sufficiently to produce wetting[51,52].Sincethe work of adhesion of liquid metals on oxide surfacesincreases with the free energy of formation of the metaloxide,it is unlikely that Cu will be efficacious.It is there-fore apparent that the oxide on aluminium is a barrier tosintering and needs to be disrupted or otherwise removed.The oxidation of a metal,M,may be represented asM+O2↔MO2(2)The free energy of formation, G,of the oxide is given byG=−R T ln K1(3)where R is the gas constant,T the temperature in kelvin andK1the equilibrium constant given byK1=(P O2)−1(4)where P O2is the partial pressure of oxygen when reaction(1)is at equilibrium.For aluminium at600◦C,a P O2<10−50atm is required to reduce the oxide[55].Atmospheres containing hydrogen are often used in powder metallurgy. Hydrogen can reduce a metal oxide by the reaction:MO+H2↔M+H2O(5) The equilibrium constant for this reaction,K5,is given byK5=P H2OP H2(6)where P H2and P H2Oare the partial pressure of hydrogen andwater vapour,respectively.The ratio of partial pressures can be converted to the dew point,effectively the water vapour content.A dew point of≤−140◦C at600◦C is required to reduce Al2O3[56].Neither a dew point of−140◦C nora P O2of10−50atm is physically attainable and thereforealuminium cannot be sintered in conventional atmospheres. Magnesium is highly reactive and the free energy of for-mation of its oxide is more negative than that of the oxides of aluminium.Magnesium therefore has the potential to act as a solid reducing agent in this system.A possible reaction is3Mg+4Al2O3↔3MgAl2O4+2Al(7)which is a partial reduction reaction.This reaction is ob-served in studies of the oxidation behaviour of Al–Mg alloys[57,58]and at bonding interfaces in metal matrix composites[59–63].Detailed analytical transmission elec-tron microscopy(Fig.1)indicates that spinel crystallites are indeed present in a sintered Al–Mg alloy.The reac-tion may be facilitated during sintering by diffusion of the magnesium through the aluminium matrix and will be ac-companied by a change in volume,creating shear stresses in thefilm,ultimately leading to its break up.This will propitiate diffusion,wetting and therefore sintering.It has been shown that the sintering of aluminium is enhanced in the presence of magnesium[23,64,65].More recently,X-ray photoelectron spectroscopy indicated that the surface oxide can be reduced in the presence of magne-sium,which exposes fresh metal and facilitates the subse-quent formation of AlN on exposed surfaces in a nitrogen atmosphere[66].The effect that magnesium has on sinter-ing can be shown by dilatometry(Fig.2).An addition of >0.15%Mg causes shrinkage.The microstructures of the Al–Sn system show that liquid tin only wets aluminium in the presence of magnesium,when the dihedral angle is very sharp.Without magnesium,the dihedral angle is obtuse and the liquid is exuded during sintering(Fig.3).By promoting sintering,magnesium also affects the mechanical proper-ties(Fig.4).The large increase in strength and ductility at0.15%Mg is a direct consequence of improved inter-particle bonding and densification following oxide rupture. The excess magnesium at concentrations>0.15%remains in solution in the aluminium,causing expansion by the Kirkendall effect and solid solution hardening.It is apparent that the oxide is not the barrier to the sin-tering of aluminium that it is traditionally considered to be. Other factors must therefore be the cause of the poor sinter-ing response.3.Alloy designAlloys are generally designed to accommodate the manu-facture of goods made from them as much as the properties required of them in service.It is for this reason that sintered steels,for example,often contain copper or phosphorous in addition to carbon and nickel.Similarly,cast aluminium al-loys are different to forging alloys which are different again to extrusion alloys.However,with one exception[67],this principle does not appear to have been applied to pressed and sintered P/M aluminium,although Savitskii only exami-ned binary alloys,the oxide phase was not reduced and no allowance was made in the thermal cycle for the transient nature of the sintering liquids.The compositions of the cur-rent commercial alloys are compared to standard wrought material in Table1.It is noteworthy that the compositions of the P/M alloys are essentially identical to those of the wrought material.It is therefore not surprising that their sin-tering response is poor.G.B.Schaffer et al./Materials Chemistry and Physics 67(2001)85–9187Fig.1.(a)Transmission electron micrograph of a sintered Al–2.5%Mg alloy,showing a multitude of spinel crystallites.The inset shows the selected area diffraction pattern from this region;it can be indexed to spinel.(b)EDS spectra from (a)showing that the fine crystallites contain significantly more magnesium and oxygen than does the aluminium matrix (c)[80].Fig.2.Dilatometry curves for Al–x Mg alloys,where x is 0,0.15and 1.5wt.%Mg showing the effect of trace additions of magnesium to aluminium cause shrinkage during sintering [80].Fig.3.Exuded liquid on the surface of an Al–8Sn alloy after sintering at 620◦C.88G.B.Schaffer et al./Materials Chemistry and Physics 67(2001)85–91Fig.4.As sintered tensile properties for Al–Mg alloys sintered 30min at 620◦C [80].Instead of producing sintered alloys which simply mimic existing wrought alloys,it is preferable to develop alloys that are specifically designed to be sintered.Based on an under-standing of fundamental liquid phase sintering phenomena,German and co-workers [68,69]recognised that it is possi-ble to define certain ideal phase diagram characteristics.The key features of an ideal liquid phase sintering system are as follows:•The additive should have a lower melting point than the base.The alternative is a low melting point eutectic which is less advantageous because liquid formation does not occur spontaneously on heating.•The solubility of the additive in the base should be low because this ensures that the additive remains segregated to particle boundaries and maximises the liquid volume.•While the base should be soluble in the liquid,it is not necessary for the base to be soluble in the solid pletely miscible liquids ensures that mass transport is not constrained.In addition,the base should also have a high diffusivity in the liquid.This ensures high rates of mass transport and therefore rapid sintering.3.1.The Al–Cu systemCopper is one of the primary alloying elements for alu-minium,based largely on the substantial age hardening re-sponse of Al–Cu alloys.They are arguably the most widely studied P/M alloys [15,19–22,70,71];they are certainly the most widely used.The binary phase diagram is shown inTable 1The composition and properties of sintered aluminium alloys and the equivalent wrought alloys [79]Alloy TypeComposition Density (%)T6propertiesCuSi Mg UTS (MPa)εf (%)6061Wrought 0.30.6110031012601P/M 0.250.619423222014Wrought 4.40.80.510048313201P/M4.40.80.5933230.5Fig.5.It has two of the ideal features:there is a single liquid phase in which aluminium is continuously soluble and the maximum solid solubility of copper in aluminium is 5.65%at 548.2◦C.However,the melting point of copper is almost double that of aluminium.The liquid phase forms as a eutec-tic between (Al)and Al 2Cu,this is shown in Fig.6.Because there is some solid solubility of copper in aluminium,the liquid is partially transient.The sequence of events during sintering of Al–Cu is:•interdiffusion takes place on heating from room tempe-rature and a series of Al–Cu intermetallics form;•the first liquid forms at 548◦C on Al–Al 2Cu (θ)bound-aries;•Cu is drawn from the liquid into solution in the aluminium and is replaced by dissolution of the intermetallics,which are replenished in turn by solid state diffusion from adjacent Cu particles;•the intermetallics disappear when all the Cu is completely dissolved;•all the liquid is absorbed into the Al particles if the Cu content is low,although most alloys retain some liquid throughoutsintering.Fig.5.The Al–Cu phase diagram [81].G.B.Schaffer et al./Materials Chemistry and Physics67(2001)85–9189Fig.6.Optical micrograph of an Al–5.5Cu alloy quenched from575◦C showing the eutectic liquid forming between the Al matrix and the Al2Cu phase.The major problem in this system is that the diffusivity of copper in aluminium is almost5000times faster than that of aluminium in copper.The diffusivity,D,of Cu in Al at600◦C is5.01×10−9cm2s−1whereas the diffusivity of Al in Cu is1.14×10−12cm2s−1[72].While the faster diffusivity of copper in aluminium enhances the rate of homogenisation, it causes expansion via the Kirkendall effect.Sintering of the Al–Cu system is therefore dependent on the process variables,particularly the copper particle size and the heating rate[73,74].This is non-ideal.3.2.The Al–Zn systemThe Al–Zn system(Fig.7)shows some of the characte-ristics of an ideal liquid phase sintering system in that zinc has a lower melting point than aluminium,no intermediate phases form and there is complete miscibility in the liquid. However,the solid solubility ratio is non-ideal.The maxi-mum solid solubility of zinc in aluminium is83.1%,while the maximum solid solubility of aluminium in zinc is1.2%. The liquid phase during sintering of Al with Zn is therefore highly transient and Al–Zn alloys are extremely process sensitive.Fast heating rates and coarse zinc particle sizes enhance sintering[73].Wherefine zinc particles are used, the zinc dissolves in the aluminium before substantial quan-tities of liquid phase can form.Where coarse zinc particles are used,the aluminium becomes locally saturatedbeforeFig.7.The Al–Zn phase diagram[81]. homogenisation is achieved.Hence the additive forms a liquid which aids sintering.High heating rates also favour liquid formation because the opportunity for diffusion to oc-cur before melting is minimised and the reaction is delayed to higher temperatures where the equilibrium solubility is smaller and therefore local saturation can occur more easily. The7000alloys have the greatest response to age hard-ening of the conventional aluminium alloys and are there-fore used as high strength forgings in the aerospace industry. Because zinc is a poor sintering aid,however,these alloys, which contain3–8%Zn,do not have a good sintering re-sponse either.The high vapour pressure of zinc also gives rise to additional porosity in these alloys,particularly when elemental powders are used[75].It is therefore necessary to use master alloy powders[76]or microalloying additions in order to achieve acceptable sintered properties[77].3.3.The Al–Sn systemAn examination of the binary aluminium phase diagrams indicates that Al–Sn is perhaps the only one which exhibits almost all of the features of an ideal system(Fig.8).The melting point of tin(232◦C)is considerably lower than that of aluminium(660◦C)and there are no intermetallic phases. Tin is sparingly soluble in solid aluminium:the maximum solid solubility is<0.15%.Aluminium is completely soluble in liquid tin and no immiscible liquids form.In addition,the diffusivity of Al in liquid Sn is faster than the diffusivity of either Cu or Zn in liquid Sn and aboutfive times greater than the self diffusivity of liquid Sn[78].In the presence of magnesium,tin is indeed a very ef-fective sintering aid.This is illustrated in Fig.9,which is a densification contour map for the Al–Sn–Mg system.The densification is a function of the green density,sintered den-sity and theoretical density and is a measure of the sintering response:positive values indicate shrinkage,negative values indicate expansion;full density is achieved at a value of1.90G.B.Schaffer et al./Materials Chemistry and Physics 67(2001)85–91Fig.8.The Al–Sn phase diagram [81].The closely spaced,parallel contour lines at low magnesium concentrations indicate that small quantities of magnesium are required to activate the system.The widely spaced,gen-tly sloping contour lines at higher magnesium concentra-tions indicate that the system is relatively insensitive to Sn concentration and Mg levels greater than the critical con-centration.At a tin concentration of 8%,the sintered density approaches 99%of theoretical.The Al–Sn–Mg system,having been designed to be sintered,is also effective for uncompacted powder,i.e.alu-minium can be gravity sintered to near full density.This facilitates free form fabrication and rapid prototyping.By combining the flexibility of free forming and the easy sin-tering of the Al–Sn–Mg system,functionally graded metal matrix composites can also be manufactured (Fig.10).The mechanical properties of the Al–Sn system,however,are low because tin does not provide muchstrengthening.Fig.9.Map showing densification of sintered aluminium as a function of magnesium and tin concentration.Each contour represents a densification of0.2.Fig.10.The macrostructure of a section of one tooth of a functionally graded,freeform fabricated gear.The centre is the Al–8Sn–4Mg alloy;the surface contains a 10wt.%loading of alumina [82].Copper could be incorporated,but sintering of the quater-nary system becomes complicated,partly because of the formation of immiscible liquid phases.4.ConclusionsSintering of aluminium has always been considered to be problematical because of the oxide film present on the sur-face of the powder particles.However,trace additions of magnesium react with the oxide to form spinel.This breaks up the oxide,which facilitates sintering.It is therefore ap-parent that in contradiction to the standard paradigm,the properties of pressed-and-sintered aluminium alloys are not limited by the “oxide problem”.Aluminium P/M alloys can be improved without recourse to hot working or master al-loy powders if their design is based on an understanding of the underlying sintering processes and the characteris-tics of an ideal liquid phase sintering system.The Al–Sn system is close to ideal and Al–Sn–Mg alloys can be sin-tered to 99%of the theoretical density.Once an alloy has been optimised for sintering,a variety of new options can be realised.Free formed,functionally graded,aluminium matrix composites and high strength materials are two such examples.AcknowledgementsThis work has been supported in part by the Australian Research Council,ACL Bearing Company,Comalco Alu-minium Ltd.and Ampal Inc.G.B.Schaffer et al./Materials Chemistry and Physics67(2001)85–9191References[1]W.S.Cebulak,E.W.Johnson,H.Markus,Met.Eng.Quart.(1976)37.[2]J.R.Pickens,J.Mater.Sci.16(1981)1437.[3]P.Gilman,Met.Mater.(1990)504.[4]vernia,J.D.Ayers,T.S.Srivatsan,Int.Mater.Rev.37(1992)1.[5]H.Jones,in:H.M.Flower(Ed.),High Performance Materials inAerospace,Chapman&Hall,London,1995,pp.340–356.[6]I.A.Ibrahim,F.A.Mohamed,vernia,J.Mater.Sci.26(1991)1137.[7]T.S.Srivatsan,I.A.Ibrahim,F.A.Mohamed,vernia,J.Mater.Sci.26(1991)5965.[8]A.L.Geiger,J.A.Walker,JOM(1991)8.[9]D.J.Lloyd,Int.Mater.Rev.39(1994)1.[10]R.Bushby,Mater.World5(1997)79.[11]J.Q.Guo,N.S.Kazama,Mater.Sci.Eng.A232(1997)177.[12]E.Hornbogen,E.A.Starke,Acta Metall.Mater.41(1993)1.[13]J.H.Dudas,W.A.Dean,Int.J.Powder Metall.5(1969)21.[14]J.H.Dudas,C.B.Thompson,Mod.Dev.Powder Metall.5(1971)19.[15]T.Watanabe,K.Yamada,Int.J.Powder Metall.4(1968)37.[16]P.E.Matthews,Int.J.Powder Metall.4(1968)39.[17]I.Amato,S.Corso,E.Sgambetterra,Powder Metall.(1976)171.[18]R.Sundaresan,P.Ramakrishnan,Int.J.Powder Metall.PowderTechnol.14(1978)9.[19]W.Kehl,H.F.Fischmeister,Powder Metall.23(1980)113.[20]W.Kehl,H.F.Fischmeister,Observations on dimensional changesduring sintering of Al–Cu compacts,in:D.Kolar,S.Pejovnik,M.M.Ristic(Eds.),Sintering—Theory and Practice,Proceedings of the Fifth International Round Table Conference on Sintering,Yugoslavia, Elsevier,Amsterdam,1981.[21]W.Kehl,M.Bugajska,H.F.Fischmeister,Powder Metall.26(1983)221.[22]F.Farzin-Nia,B.L.Davies,Powder Metall.25(1982)209.[23]I.A.Shibli,D.E.Davies,Powder Metall.30(1987)97.[24]H.J.Mathieu,M.Datta,ndolt,J.Vac.Sci.Technol.3(1985)331.[25]I.Olefjord,A.Karlsson,Surface analysis of aluminium foil,in:T.Sheppard(Ed.),Aluminium Technology’86,The Institute of Metals, London,1986.[26]P.Marcus,C.Hinnen,I.Olefjord,Surf.Interf.Anal.20(1993)923.[27]A.Nylund,I.Olefjord,Surf.Interf.Anal.21(1994)290.[28]I.E.Anderson,J.C.Foley,J.F.Flumerfelt,Simplified aluminumpowder metallurgy processing routes for automotive applications, in:W.F.Jandeska,R.A.Chernenkoff(Eds.),Powder Metallurgy Aluminum and Light Alloys for Automotive Applications,MPIF, Dearbon,MI,1998.[29]A.Ozbilen,A.Unal,T.Sheppard,Influence of oxygen on morpho-logy and oxide content of gas atomised aluminium powders,in:W.M.Small(Ed.),Physical Chemistry of Powder Metals—Production and Processing,The Minerals,Metals and Materials Society,1989.[30]P.Nielsen,Y.L.Liu,N.Hansen,Manufacturing of aluminiumcomposites with high purity matrix,in:1993Powder Metallurgy World Congress,Japan Society of Powder and Powder Metallurgy, Japan,1993.[31]Y.Kim,W.M.Griffith,F.H.Froes,J.Met.37(1985)27.[32]M.H.Jacobs,J.Microsc.99(1972)165.[33]N.A.Thorne,P.Thuery,A.Frichet,P.Gimenez,A.Sartre,Surf.Interf.Anal.16(1990)236.[34]I.Olefjord,A.Nylund,Surf.Interf.Anal.21(1994)290.[35]J.C.Fuggle,L.M.Watson,D.J.Fabian,S.Affrossman,Surf.Sci.49(1975)61.[36]J.F.Flumerfelt,I.E.Anderson,The surface chemistry of pure alumi-num powders as measured with quadrupole mass spectrometry,in: 1998International Conference on Powder Metallurgy and Particulate Materials,MPIF,Las Vegas,NV,1998.[37]K.Shinohara,T.Seo,H.Kyogoku,Zeitschrift fur Metallkunde73(1982)774.[38]H.J.van Beek,E.J.Mittemeijer,Thin Solid Films122(1984)131.[39]W.H.Gitzen,Alumina as a Ceramic Material,American CeramicSociety,Westerville,OH,Special Publication No.4,1970.[40]R.F.Smart,E.C.Ellwood,Nature181(1958)833.[41]R.Q.Guo,P.K.Rohatgi,D.Nath,J.Mater.Sci.32(1997)3971.[42]P.Ramakrishnan,G.S.Tendolkar,Powder Metall.7(1964)34.[43]P.Ramakrishnan,Powder Metall.9(1966)47.[44]T.Watanabe,Y.Horikosji,Int.J.Powder Metall.Powder Technol.12(1976)209.[45]Z.A.Munir,J.Mater.Sci.17(1979)2733.[46]Z.A.Munir,Powder Metall.24(1981)177.[47]P.K.Higgins,Z.A.Munir,Powder Metall.Int.14(1982)26.[48]W.Kingery,J.Appl.Phys.30(1959)301.[49]F.Delannay,L.Froyen,A.Deruyttere,J.Mater.Sci.22(1987)1.[50]J.V.Naidich,Prog.Surf.Memb.Sci.14(1981)353.[51]S.W.Ip,M.Kucharski,J.M.Toguri,J.Mater.Sci.Lett.12(1993)1699.[52]Y.Liu,Z.He,G.Dong,Q.Li,J.Mater.Sci.Lett.11(1992)896.[53]D.T.Livey,P.Murray,Second Plansee Seminar,1955.[54]J.-G.Li,Ceram.Int.20(1994)391.[55]L.S.Darken,R.W.Gurry,Physical Chemistry of Metals,McGraw-Hill,New York,1953,p.349.[56]ll,Int.J.Powder Metall.27(1991)315.[57]G.M.Scamans,E.P.Butler,Metall.Trans.A6A(1975)2055.[58]D.H.Kim,E.P.Yoon,J.S.Kim,J.Mater.Sci.Lett.15(1996)1429.[59]C.G.Levi,G.J.Abbaschian,R.Mehrabian,Metall.Trans.A9A(1978)697.[60]R.Molins,J.D.Bartout,Y.Bienvenu,Mater.Sci.Eng.A135(1991)111.[61]J.Homeny,M.M.Buckley,Mater.Lett.10(1991)421.[62]A.Munitz,M.Metzger,R.Mehrabian,Metall.Trans.A10A(1979)1491.[63]A.D.McLeod,C.M.Gabryel,Metall.Trans.A23A(1992)1279.[64]S.Miura,Y.Machida,Y.Hirose,R.Yoshimura,The effect of variedalloying methods on the properties of sintered aluminium alloys,in: 1993Powder Metallurgy World Conference,Japan1993.[65]K.Nishiyama,E.Suzuki,Processing and wear properties of P/MAl–Pb alloys,in:1993Powder Metallurgy World Congress,1993.[66]A.Kimura,et al.,Appl.Phys.Lett.70(1997)3615.[67]A.P.Savitskii,Liquid Phase Sintering of the Systems with InteractingComponents,Russian Academy of Sciences,Tomsk,1993.[68]R.M.German,B.H.Rabin,Powder Metall.28(1985)7.[69]R.M.German,Liquid Phase Sintering,Plenum Press,New York,1985.[70]F.J.Esper,G.Leuze,Powder Metall.Int.3(1971)123.[71]T.Watanabe,K.Yamada,Effects of Copper Adding Methods on theStrength of Sintered Aluminium–Copper Alloys,V ol.19,Castings Research Laboratory,Waseda University,1968.[72]H.Mehrer,Landolt Bornstein Numerical Data and FunctionalRelationships in Science and Technology,V ol.III/26,Springer, Berlin,1991.[73]R.Lumley,G.B.Schaffer,Scripta Mater.35(1996)589.[74]R.Lumley,G.B.Schaffer,Scripta Mater.39(1998)1089.[75]R.N.Lumley,G.B.Schaffer,Metall.Mater.Trans.A30A(1999)1682.[76]M.Muhlburger,P.Paschen,Zeitschrift fur Metallkunde84(1993)346.[77]G.B.Schaffer,S.H.Huo,Powder Metall.42(1999)219.[78]C.H.Ma,R.A.Swalin,Acta Metall.8(1960)388.[79]Aluminum and Aluminum Alloys,ASM Specialty Handbook,ASMInternational,Materials Park,OH,1993.[80]R.Lumley,T.B.Sercombe,G.B.Schaffer,Metall.Mater.Trans.A30A(1999)457.[81]Alloy Phase Diagrams,ASM Handbook,V ol.3,ASM International,Materials Park,OH,1992.[82]T.B.Sercombe,G.B.Schaffer,P.Calvert,J.Mater.Sci.34(1999)4245.。
烧结基础知识PPT课件
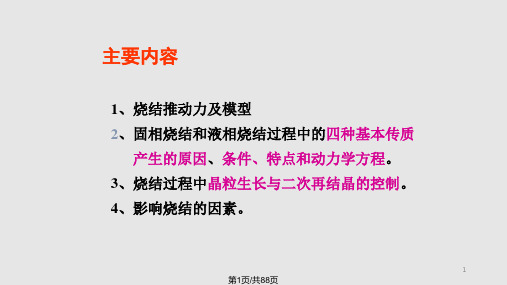
如果表面张力足以使晶体产生位错,这
时质点通过整排原子的运动或晶面的滑移来实
现物质传递,这种过程称塑性流动。可见塑性
流动是位错运动的结果。与粘性流动不同,塑
性流动只有当作用力超过固体屈服点时才能产
生 , 其 流 动 服 从 宾 汉 (Bingham) 型 物 体 的 流
动规律即, F v
S
x
(3-3-9)
3
e KT
1
3
C0
KT
;
C1 C0
C03 KT
自颈部到接触点 浓度差
C1 C2 23 C0 KT
;
C1
C2
2C0 3 KT
自颈部到内部 浓度差
结论: ①由于应力的分布不均匀造成空位浓度梯度,空位将主要从颈部表面扩散到
颈部中心两颗粒接触处;
②空位也从颈部表面扩散到颗粒内无应力区,但其量比前一种扩散量少一半;
物理性质变化:V 、气孔率 、强度 、 致密度……
缺点:只描述宏观变化,未揭示本质。
3
第3页/共88页
定义2:在表面张力作用下的扩散蠕变。
优点:揭示了本质。 缺点:未描述宏观物理性质变化。
4
第4页/共88页
烧结的指标
➢烧结收缩率 ➢强度 ➢实际密度/理论密度 ➢吸水率 ➢气孔率等
5
第5页/共88页
③空位扩散即原子或离子的反向扩散。 这就造成了物质的迁移。而随着这种物质迁移,空隙被填充,致密度提高。
与此同时,颗粒间的接触界面增加,机械强度增加。
28
第28页/共88页
⑶扩散途径 ( 结论: C1>C0>C2 空位扩散:优先由颈表面) 接触点;
1C> 2C
无机非金属材料基础PPT课件第十章 烧结
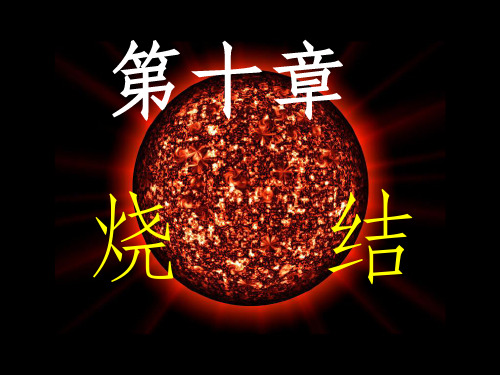
12
烧结与固相反应区别
相同点:
两个过程均在低于材料熔点或熔融温 度之下进行,并且在过程的自始至终都至少 有一相是固态。
整理ppt
13
• 不同点:
固相反应发生化学反应,固相反应必 须至少有两组元参加如A和B,最后生成化 合物AB,AB结构与性能不同于A与B。
•
烧结不发生化学反应,可以只有单组
元;或者两组元参加,但两组元并不发生
烧结不能自发进行,必须对粉体加以高温,才能促 使粉末体转变为烧结体。
整理ppt
18
常用γGB晶界能和γSV表面能之比值来衡量烧结 的难易,γGB/γSV愈小,愈容易烧结,
为了促进烧结,必须使γSV>γGB。
一般Al2O3粉的表面能约为1J/m2,而晶界能为 0.4J/m2,两者之差较大,比较易烧结;
整理ppt
15
近年来,在研制特种结构材料和功能 材料的同时,产生了一些新型烧结方法。
如热压烧结,电火花烧结,热等静压 烧结,微波烧结、反应烧结、活化烧结、 松装烧结等。
整理ppt
16
四、烧结过程推动力
烧结过程推动力是:能量差、压力差、空位差。
1、能量差
近代烧结理论认为,粉状物料的表面能大于多晶
烧结体的晶界能,这就是烧结的推动力,即粉状物料
度增加,因此烧结程度可以用坯体收缩率、气孔 率、吸水率或烧结体密度与理论密度之比(相 对密度)等指标来表示。
同时,粉末压块的性质也随这些物理过程的进 展而出现坯体收缩,气孔率下降、致密、强度增加、 电阻率下降等变化。
随着烧结温度升高,气孔率下降;密度升高;
电阻下降;强度升高;晶粒尺寸增大。
整理ppt
9
整理ppt
- 1、下载文档前请自行甄别文档内容的完整性,平台不提供额外的编辑、内容补充、找答案等附加服务。
- 2、"仅部分预览"的文档,不可在线预览部分如存在完整性等问题,可反馈申请退款(可完整预览的文档不适用该条件!)。
- 3、如文档侵犯您的权益,请联系客服反馈,我们会尽快为您处理(人工客服工作时间:9:00-18:30)。
液相烧结的类型:据液相数量与性 质可分为两类三种情况:
表9-1 液相烧结类型
类 型
条件
Ⅰ
QLS >90° C=0
液相数量 烧结模型
0.01~0.5mol% 双球
传质方式
扩散
QLS <90° 少
Ⅱ
C>0
多
Kingery* LSW* *
溶解-沉淀
3
一. 流动传质:
1. 粘性流动: 机理(原因): 由于高温下粘性液体(熔融体)出现牛顿型流动而
第三节 液相参与的烧结
1
液相烧结:
❖ 凡有液相参加的烧结过程称为液相烧结。 ❖ 液相烧结与固相烧结的异同点: ❖ 相同点:烧结的推动力都是表面能,烧结过程
都是由颗粒重排、气孔充填和晶粒生长等阶段 组成
❖ 不同点:由于流动传质比扩散传质快,因而液 相烧结致密化速率高,可使坯体在比固态烧结 温度低得多的情况下获得致密的烧结体;影响 液相烧结的因素比固相烧结更为复杂,为定量 研究带来困难。
11
三. 不同烧结及传质机理比较
❖ 1. 固相烧结与液相烧结的异同点:
⑴ 相同点:
❖烧结的推动力都是表面能
❖烧结过程都包括颗粒接触、聚集、重排,气孔填充、 排出和晶粒生长等阶段
⑵ 不同点:
❖液相烧结比固相烧结传质速率高,因而液相烧结致 密化速率比固相烧结快,可以在更低的温度下使坯 体烧结成致密体
❖液相烧结的研究比固相烧结复杂。因为液相烧结速 率与液相量、η、γ、对固相润湿程度及固相在液 相中的溶解度等诸多因素有关。
❖ 3. 过程:颗粒重排和溶解-沉淀传质
8
❖ 颗粒重排:
颗粒在毛细管力作用下,通过粘性流动或在一 些颗粒间接触点上由于局部应力的作用而重新 排列,使堆积更致密。
致密化速率与粘性流动相应,线收缩与时间呈 线性关系: L Lt1x
坯体变化:烧结收缩率达60%以上
9
溶解-沉淀传质:
❖据液相量不同分两种情况:
❖决定烧结速率的三个主要参数:颗粒起始粒径r、
粘度η、表面张力。
6
2. 塑性流动
当坯体中液相含量很少时,高温下流动传 质不能看成是纯牛顿型流动,而是塑性流 动
在固态烧结中也存在塑性流动
7
二. 溶解-沉淀传质
❖ 1. 条件:
显著的液相量 固相在液相内有显著的可溶性 液相润湿固相
❖ 2. 原因(推动力):颗粒的表面能,表现 为毛细管力
产生的传质称为粘性流动传质(或粘性蠕变传质)。 在固态烧结中,晶体内的晶格空位在应力作用下,
由空位的定向流动引起的形变称为粘性蠕变 粘性蠕变与扩散传质的区别:粘性蠕变是在应力
作用下,整排原子沿着应力方向移动,而扩散传 质仅是一个质点的迁移
4
❖ 粘性蠕变通过粘度系数(η)把粘性蠕变速率(ε)
与应力(σ)联系起来
1.颗粒在接触点处溶解到自由表面上沉积
2.小晶粒溶解至大晶粒处沉淀
原理:由于颗粒接触点处(或小晶粒)在液 相中的溶解度大于自由表面(或大晶粒)处 的溶解度
❖线收缩:
1
L L K t 3
4 1
L L r 3 t 3
当T,r一定时,
10
Hale Waihona Puke ❖ 4. 影响因素: ❖颗粒起始粒度 ❖粉末特性(溶解度、润湿性) ❖液相数量 ❖烧结温度
3.固-液润湿
特点 公式
1.凸面蒸发凹面凝聚 2. △L/L=0
x Kr2 3t1 3 r
1.空位与结构 基元相对扩散 2.中心距缩短
x Kr3 5t1 5 r LLKr65t25
1.流动同时引 起颗粒重排 2化. 速LL率最t 高致密
1.接触点溶解到平 面上沉积,小晶粒处 溶解到大晶粒处沉 积
,
又
KTd2 8D*
8D* KTd2
❖ 粘性蠕变产生区域:晶界区域、位错区域
5
动力学关系:
❖烧结模型:双球模型(中心距缩短)
❖颈部增长公式:
x
(3
1
)2
1
r 2
t
1 2
r 2
❖体收缩:
V3L 9 t V L 4r
❖麦肯基导出适合粘性流动传质全过程的烧结速率公
式:
d 3 (1)
dt 2 r
θ—相对密度,即体积密度d/理论密度d0
12
❖ 2.各种传质综合比较:
表9-2 各种传质产生原因、条件、特点等综合比较表
传质方式 蒸发-凝聚
扩散
流动
溶解-沉淀
原因 压力差△P 空位浓度差△C 应力-应变
溶解度△C
条件
△P>10~ 1Pa
r<10μm
空位浓度
C n0 N
r<10μm
粘性流动η 小 塑性流动τ >f
1.可观的液相量 2.固相在液相中溶 解度大
2.传质同时又是晶 粒生长过程
L L 3 t 2 r
LLKr43t13
dQ d tK (1Q )/r xrKr23t16
工艺控制
温度(蒸汽压) 粒度
温度(扩散系数) 粒度
粘度 粒度
粒度 温度(溶解1度3 ) 粘度 液相数量