NAC4分子机制图-对比谷胱甘肽及相关巯基化合物对药物性肝损伤的保护作用
还原型谷胱甘肽治疗药物性肝损伤的疗效观察
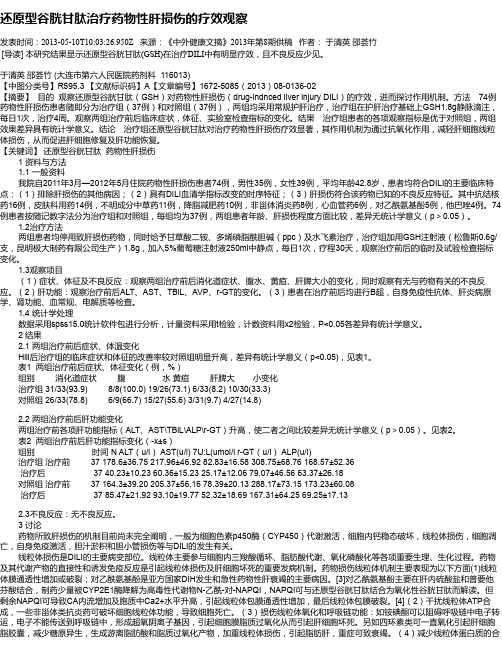
还原型谷胱甘肽治疗药物性肝损伤的疗效观察发表时间:2013-05-10T10:03:26.950Z 来源:《中外健康文摘》2013年第8期供稿作者:于清英邵荟竹[导读] 本研究结果显示还原型谷胱甘肽(GSH)在治疗DILI中有明显疗效,且不良反应少见。
于清英邵荟竹 (大连市第六人民医院药剂科 116013)【中图分类号】R595.3 【文献标识码】A【文章编号】1672-5085(2013)08-0136-02【摘要】目的观察还原型谷胱甘肽(GSH)对药物性肝损伤(drug-indnced liver injury DILI)的疗效,进而探讨作用机制。
方法74例药物性肝损伤患者随即分为治疗组(37例)和对照组(37例),两组均采用常规护肝治疗,治疗组在护肝治疗基础上GSH1.8g静脉滴注,每日1次,治疗4周。
观察两组治疗前后临床症状,体征、实验室检查指标的变化。
结果治疗组患者的各项观察指标是优于对照组,两组效果差异具有统计学意义。
结论治疗组还原型谷胱甘肽对治疗药物性肝损伤疗效显著,其作用机制为通过抗氧化作用,减轻肝细胞线粒体损伤,从而促进肝细胞修复及肝功能恢复。
【关键词】还原型谷胱甘肽药物性肝损伤1 资料与方法1.1 一般资料我院自2011年3月—2012年5月住院药物性肝损伤患者74例,男性35例,女性39例,平均年龄42.8岁,患者均符合DILI的主要临床特点:(1)排除肝损伤的其他病因;(2)具有DILI血清学指标改变的时序特征;(3)肝损伤符合该药物已知的不良反应特征。
其中抗结核药16例,皮肤科用药14例,不明成分中草药11例,降脂减肥药10例,非甾体消炎药8例,心血管药6例,对乙酰氨基酚5例,他巴唑4例。
74例患者按随记数字法分为治疗组和对照组,每组均为37例,两组患者年龄、肝损伤程度方面比较,差异无统计学意义(p>0.05)。
1.2治疗方法两组患者均停用致肝损伤药物,同时给予甘草酸二铵、多烯磷脂酰胆碱(ppc)及水飞素治疗,治疗组加用GSH注射液(松鲁斯0.6g/支,昆明极大制药有限公司生产)1.8g,加入5%葡萄糖注射液250ml中静点,每日1次,疗程30天,观察治疗前后的临时及试验检查指标变化。
常用的护肝药有哪些
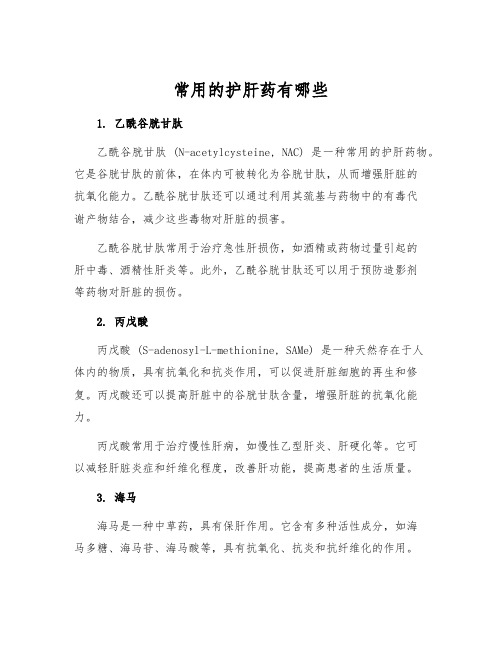
常用的护肝药有哪些1. 乙酰谷胱甘肽乙酰谷胱甘肽 (N-acetylcysteine, NAC) 是一种常用的护肝药物。
它是谷胱甘肽的前体,在体内可被转化为谷胱甘肽,从而增强肝脏的抗氧化能力。
乙酰谷胱甘肽还可以通过利用其巯基与药物中的有毒代谢产物结合,减少这些毒物对肝脏的损害。
乙酰谷胱甘肽常用于治疗急性肝损伤,如酒精或药物过量引起的肝中毒、酒精性肝炎等。
此外,乙酰谷胱甘肽还可以用于预防造影剂等药物对肝脏的损伤。
2. 丙戊酸丙戊酸 (S-adenosyl-L-methionine, SAMe) 是一种天然存在于人体内的物质,具有抗氧化和抗炎作用,可以促进肝脏细胞的再生和修复。
丙戊酸还可以提高肝脏中的谷胱甘肽含量,增强肝脏的抗氧化能力。
丙戊酸常用于治疗慢性肝病,如慢性乙型肝炎、肝硬化等。
它可以减轻肝脏炎症和纤维化程度,改善肝功能,提高患者的生活质量。
3. 海马海马是一种中草药,具有保肝作用。
它含有多种活性成分,如海马多糖、海马苷、海马酸等,具有抗氧化、抗炎和抗纤维化的作用。
海马常用于治疗肝炎、肝硬化等肝脏疾病。
研究表明,海马可以减轻肝脏损伤,促进肝脏细胞的再生,改善肝功能。
4. 氨基酸氨基酸是构成蛋白质的基本单位。
在肝脏中,氨基酸起着重要的营养和代谢作用,可以促进肝脏细胞的修复和再生。
常用的护肝氨基酸包括谷氨酰胺、赖氨酸、精氨酸等。
它们可以提供充足的营养物质,促进肝功能的恢复。
5. 维生素E维生素E是一种脂溶性维生素,具有抗氧化作用。
它可以清除体内的自由基,减轻肝脏细胞的氧化损伤,保护肝脏免受损害。
维生素E常用于治疗脂肪肝、酒精性肝炎等肝脏疾病。
研究表明,维生素E可以减少脂肪在肝脏中的积累,改善肝脏功能。
6. 丹参丹参是一种中草药,被广泛用于中医治疗肝脏疾病。
它含有多种活性成分,如丹参酮、丹酚酸等,具有抗氧化、抗炎和抗纤维化的作用。
丹参常用于治疗慢性肝炎、肝硬化等肝脏疾病。
研究表明,丹参可以减轻肝脏损伤,改善肝功能,促进肝脏细胞的修复和再生。
谷胱甘肽的保护机制及其修复细胞受损功能
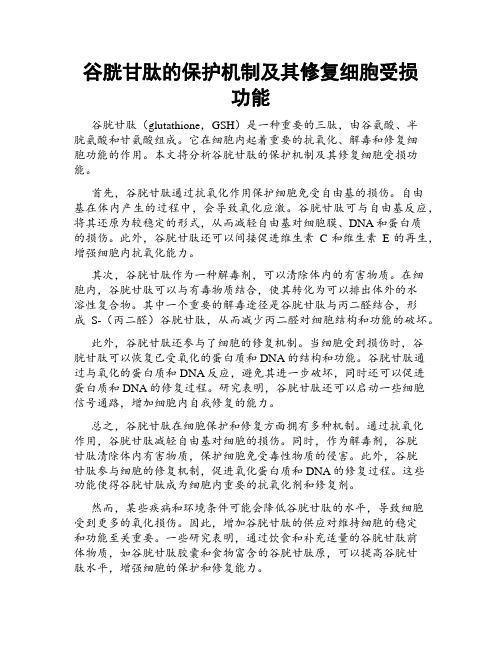
谷胱甘肽的保护机制及其修复细胞受损功能谷胱甘肽(glutathione,GSH)是一种重要的三肽,由谷氨酸、半胱氨酸和甘氨酸组成。
它在细胞内起着重要的抗氧化、解毒和修复细胞功能的作用。
本文将分析谷胱甘肽的保护机制及其修复细胞受损功能。
首先,谷胱甘肽通过抗氧化作用保护细胞免受自由基的损伤。
自由基在体内产生的过程中,会导致氧化应激。
谷胱甘肽可与自由基反应,将其还原为较稳定的形式,从而减轻自由基对细胞膜、DNA和蛋白质的损伤。
此外,谷胱甘肽还可以间接促进维生素C和维生素E的再生,增强细胞内抗氧化能力。
其次,谷胱甘肽作为一种解毒剂,可以清除体内的有害物质。
在细胞内,谷胱甘肽可以与有毒物质结合,使其转化为可以排出体外的水溶性复合物。
其中一个重要的解毒途径是谷胱甘肽与丙二醛结合,形成S-(丙二醛)谷胱甘肽,从而减少丙二醛对细胞结构和功能的破坏。
此外,谷胱甘肽还参与了细胞的修复机制。
当细胞受到损伤时,谷胱甘肽可以恢复已受氧化的蛋白质和DNA的结构和功能。
谷胱甘肽通过与氧化的蛋白质和DNA反应,避免其进一步破坏,同时还可以促进蛋白质和DNA的修复过程。
研究表明,谷胱甘肽还可以启动一些细胞信号通路,增加细胞内自我修复的能力。
总之,谷胱甘肽在细胞保护和修复方面拥有多种机制。
通过抗氧化作用,谷胱甘肽减轻自由基对细胞的损伤。
同时,作为解毒剂,谷胱甘肽清除体内有害物质,保护细胞免受毒性物质的侵害。
此外,谷胱甘肽参与细胞的修复机制,促进氧化蛋白质和DNA的修复过程。
这些功能使得谷胱甘肽成为细胞内重要的抗氧化剂和修复剂。
然而,某些疾病和环境条件可能会降低谷胱甘肽的水平,导致细胞受到更多的氧化损伤。
因此,增加谷胱甘肽的供应对维持细胞的稳定和功能至关重要。
一些研究表明,通过饮食和补充适量的谷胱甘肽前体物质,如谷胱甘肽胶囊和食物富含的谷胱甘肽原,可以提高谷胱甘肽水平,增强细胞的保护和修复能力。
此外,还有一些通过其他途径提高谷胱甘肽水平的方法。
谷胱甘肽反应性代谢物引起药物性肝损伤的机制研究进展

谷胱甘肽反应性代谢物引起药物性肝损伤的机制研究进展Δ
# 何亮伟 1,2*, 耿 婷 1, 李艳静 1, 黄文哲 1, 王振中 1, 齐炼文 2, 萧 伟 1( 1.江苏康缘药业股份有限公司, 江苏 连云港 222001; 2.中国药科大学中药学院, 南京 211198)
中图分类号 DOI 摘
R932
药物性肝损伤是指药物本身的作用、 药物的相互作 用及药物代谢物的作用引起肝毒性或功能损害, 进而引 起各种临床异常症状, 临床表现为肝功能异常、 肝硬化, 抗结核药 最终可能导致肝性死亡 [1- 2]。 抗生素类药物 、 物、 心血管药物、 消化道用药、 抗真菌药物、 抗癫痫药物、 抗肿瘤药物以及一些中药如补骨脂、 首乌片、 马钱子等, [3] 都会引起肝轻度或重度损害 。临床药物的不良反应中 有 10%~15%为肝损伤, 老年人更高达 20%以上[4]。 由于药物性肝损伤的临床表现和病理变化较为复 杂, 传统的临床生化指标不足以揭示其发病机制。近年 来, 基因组学、 蛋白质组学、 代谢组学等系统生物学技术 逐渐被运用到药物性肝损伤的研究中 。 如 Kiyosawa N 以谷胱甘肽 (GSH) 生物标记物 等 [5] 运用代谢组学技术 , 评价化学品诱导的 GSH 耗竭在肝中的潜在风险。笔者 以 “ 谷胱甘肽 ” “ 反应性代谢物 ” “ 药物性肝损伤 ” “Reactive metabolites” “Glutathione conjugate” “Liver injury” 等 为关键词, 组 合 查 询 1993 年 1 月 - 2016 年 4 月 在 PubMed、 ScienceDirect、 Web of Science、 中国知网、 万方、 维普等数据库中的相关文献。结果, 共检索到相关文献 112 篇, 其中有效文献 37 篇。现对引起药物性肝损伤的 可能机制 、 化学药物和中药的肝损伤研究 、 GSH 反应性
保肝药物思美泰作用和机制探讨ppt课件

➢ 医疗保健研究和质量机构支持SAMe用于:
➢ 减轻瘙痒症; ➢ 妊娠期胆汁淤积; ➢ 其它原因的高胆红素血症。
为深入学习习近平新时代中国特色社 会主义 思想和 党的十 九大精 神,贯彻 全国教 育大会 精神,充 分发挥 中小学 图书室 育人功 能
SAMe代谢障碍导致肝病发生
基侧膜 √ √ √
√ √ √ √
肝细胞内 √ √ √
√
毛细胆管膜 √ √ √ √ √ √ √ √
Stieger B, et al. Curr Opinion Biol 1998;10:462-467
诱导 肝细胞
B2)
目标 诱导 基因拼接
Bcl-xs
促细胞 凋亡
抑制
甜菜碱甲 基转移酶
PP1 分解亚基
mRNA
SR蛋白 去磷酸化
甲硫氨酸
为深入学习习近平新时代中国特色社 会主义 思想和 党的十 九大精 神,贯彻 全国教 育大会 精神,充 分发挥 中小学 图书室 育人功 能
SAMe用于肝脏疾病的治疗
➢ 动物试验证明-SAMe可用于:
➢ 治疗酒精性肝病,减少GSH的损耗及肝损伤; ➢ 改善半乳糖、对乙酰氨基酚以及硫代乙酰胺诱导
的肝脏毒性和以及缺血再灌注诱导的肝损伤; ➢ 减少四氯化碳所造成的肝纤维化; ➢ 减少动物肝细胞癌模型肝细胞增生。
为深入学习习近平新时代中国特色社 会主义 思想和 党的十 九大精 神,贯彻 全国教 育大会 精神,充 分发挥 中小学 图书室 育人功 能
HGF
P
肝细胞生长 因子代谢
AMP
HGF:肝细胞生长因子 AMP:腺苷一磷酸 AMPK:AMP激活蛋白激酶
AMPK SAMe
谷胱甘肽的生物合成及其作用机制

谷胱甘肽的生物合成及其作用机制谷胱甘肽(Glutathione)是一种非常重要的小分子抗氧化剂,广泛存在于各种生物体中,尤其是细胞内。
其作用在于保护细胞免受氧化损伤和维持氧化还原平衡,是机体抵御各种疾病和病理过程的重要保障。
本文将介绍谷胱甘肽的生物合成及其作用机制,为大家揭开一个神奇的分子的神秘面纱。
一、谷胱甘肽的生物合成谷胱甘肽由三种氨基酸谷氨酸、半胱氨酸和甘氨酸通过谷胱甘肽还原酶(GR)催化反应所合成。
整个合成过程可以分为两个步骤:1、谷胱甘肽合成第一步谷胱甘肽合成的第一步是在谷氨酸的羧基上添加半胱氨酸,形成γ-谷胱氨酸(γ-Glu-Cys)。
这个反应是通过γ-谷胱氨酸合成酶(GCL)催化的。
γ-Glu-Cys在生物体内是一种非常不稳定的中间物质,容易被分解成半胱氨酸和谷氨酸。
因此,这个反应在体内受到好几种因素的调节,如细胞内Nrf2等转录因子在接受一些刺激后可上调GCL的表达水平,从而促进谷胱甘肽的合成。
2、谷胱甘肽合成第二步谷胱甘肽合成的第二步是在γ-谷胱氨酸的羧基上添加一个甘氨酸残基,形成谷胱甘肽(GSH)。
这个反应是通过谷胱甘肽合成酶(GS)催化的。
实验发现,GSH的合成过程同样受到很多调节因素的影响。
例如,通过增加GS的活性和GSH合成前体的供应可以提高GSH的合成。
二、谷胱甘肽的作用机制谷胱甘肽的作用机制涉及到多个领域,包括氧化还原状态的调节、解毒、细胞的增殖和凋亡、蛋白质和DNA的修复等。
以下分别介绍其主要作用机制。
1、谷胱甘肽的抗氧化作用谷胱甘肽作为一个还原型分子,具有代谢过程中的还原能力。
体内的氧化还原平衡常常受到氧化应激的影响,即存在氧自由基等有害物质,会造成氧化失衡。
当造成氧自由基的生成时,谷胱甘肽被氧化成了谷胱甘肽二硫化物(GSSG),但在还原支持下会再次回到GSH形成还原状态。
因此,谷胱甘肽通过消除氧自由基的产生和抑制细胞内的氧化应激反应,从而保护细胞不受到氧化损伤。
谷胱甘肽在肝脏疾病中的作用及应用
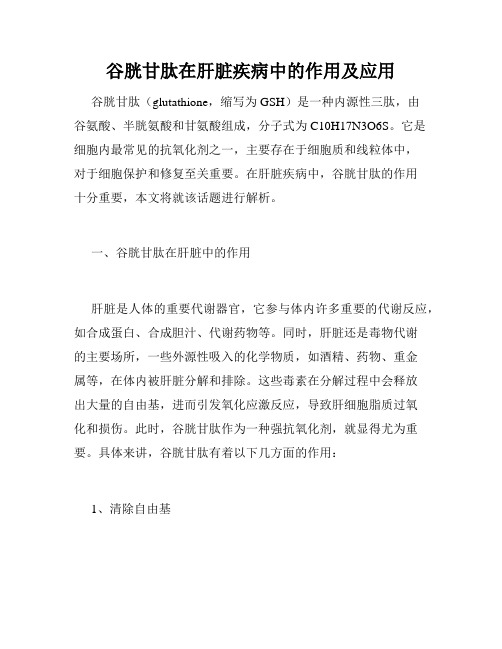
谷胱甘肽在肝脏疾病中的作用及应用谷胱甘肽(glutathione,缩写为GSH)是一种内源性三肽,由谷氨酸、半胱氨酸和甘氨酸组成,分子式为C10H17N3O6S。
它是细胞内最常见的抗氧化剂之一,主要存在于细胞质和线粒体中,对于细胞保护和修复至关重要。
在肝脏疾病中,谷胱甘肽的作用十分重要,本文将就该话题进行解析。
一、谷胱甘肽在肝脏中的作用肝脏是人体的重要代谢器官,它参与体内许多重要的代谢反应,如合成蛋白、合成胆汁、代谢药物等。
同时,肝脏还是毒物代谢的主要场所,一些外源性吸入的化学物质,如酒精、药物、重金属等,在体内被肝脏分解和排除。
这些毒素在分解过程中会释放出大量的自由基,进而引发氧化应激反应,导致肝细胞脂质过氧化和损伤。
此时,谷胱甘肽作为一种强抗氧化剂,就显得尤为重要。
具体来讲,谷胱甘肽有着以下几方面的作用:1、清除自由基自由基是氧化应激反应产生的一种中间产物,它们具有极强的氧化性,能与生物分子发生反应,导致细胞损伤或死亡。
而谷胱甘肽具有强烈的清除自由基的能力,通过捕捉自由基上的过多电子,中和自由基的氧化能力,减少氧化应激反应的程度。
2、保护细胞膜肝细胞膜是肝细胞的一个重要部分,它与细胞内某些功能有着密切的关系。
谷胱甘肽可以通过清除自由基、减少氧化应激反应等途径,保护细胞膜的完整性和功能。
3、促进肝解毒酶的活性肝细胞是毒物代谢的主要场所,其中的解毒酶对于对抗外源性的有害物质具有重要作用。
而谷胱甘肽可以促进解毒酶的活性,帮助肝脏更加有效地分解和清除体内有害物质。
4、促进肝细胞再生谷胱甘肽具有促进肝细胞再生的作用。
当肝细胞受到损伤或死亡时,谷胱甘肽能够帮助细胞恢复正常的状态并促进细胞修复和再生,从而保护肝脏功能。
二、谷胱甘肽在肝疾病中的应用谷胱甘肽在肝疾病治疗中具有广泛的应用价值。
目前相关研究表明,谷胱甘肽对于肝硬化、乙型肝炎、脂肪肝、毒性肝病等肝脏疾病具有较好的治疗效果。
谷胱甘肽可以通过多种途径进行使用,如口服、注射等。
谷胱甘肽的生理功能和医学用途-病理学论文-基础医学论文-医学论文
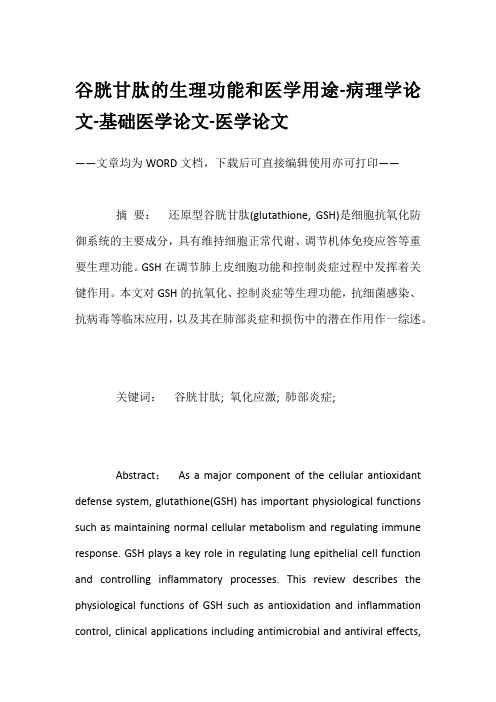
谷胱甘肽的生理功能和医学用途-病理学论文-基础医学论文-医学论文——文章均为WORD文档,下载后可直接编辑使用亦可打印——摘要:还原型谷胱甘肽(glutathione, GSH)是细胞抗氧化防御系统的主要成分,具有维持细胞正常代谢、调节机体免疫应答等重要生理功能。
GSH在调节肺上皮细胞功能和控制炎症过程中发挥着关键作用。
本文对GSH的抗氧化、控制炎症等生理功能,抗细菌感染、抗病毒等临床应用,以及其在肺部炎症和损伤中的潜在作用作一综述。
关键词:谷胱甘肽; 氧化应激; 肺部炎症;Abstract:As a major component of the cellular antioxidant defense system, glutathione(GSH) has important physiological functions such as maintaining normal cellular metabolism and regulating immune response. GSH plays a key role in regulating lung epithelial cell function and controlling inflammatory processes. This review describes the physiological functions of GSH such as antioxidation and inflammation control, clinical applications including antimicrobial and antiviral effects,as well as its potential role in lung inflammation and injury.Keyword:glutathione; oxidative stress; lung inflammation;由获得者、英国皇家学会会长Hopkins在1921年发现的谷胱甘肽(glutathione),是一种由谷氨酸、半胱氨酸和甘氨酸组成的三肽,所有器官均可合成。
谷胱甘肽的生物学功能及药理学应用
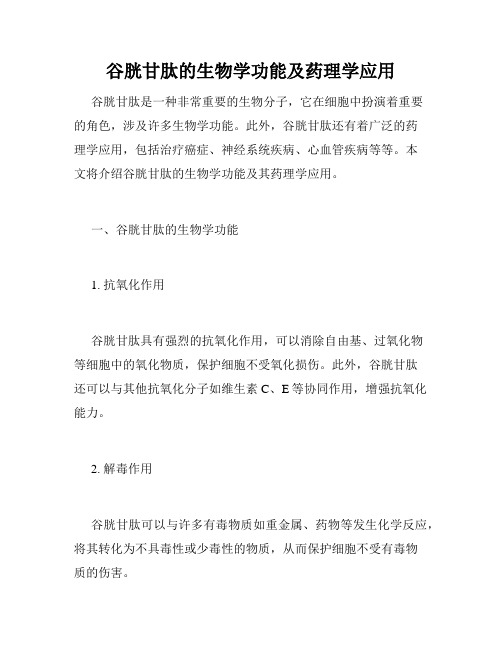
谷胱甘肽的生物学功能及药理学应用谷胱甘肽是一种非常重要的生物分子,它在细胞中扮演着重要的角色,涉及许多生物学功能。
此外,谷胱甘肽还有着广泛的药理学应用,包括治疗癌症、神经系统疾病、心血管疾病等等。
本文将介绍谷胱甘肽的生物学功能及其药理学应用。
一、谷胱甘肽的生物学功能1. 抗氧化作用谷胱甘肽具有强烈的抗氧化作用,可以消除自由基、过氧化物等细胞中的氧化物质,保护细胞不受氧化损伤。
此外,谷胱甘肽还可以与其他抗氧化分子如维生素C、E等协同作用,增强抗氧化能力。
2. 解毒作用谷胱甘肽可以与许多有毒物质如重金属、药物等发生化学反应,将其转化为不具毒性或少毒性的物质,从而保护细胞不受有毒物质的伤害。
3. 免疫调节作用谷胱甘肽可以调节免疫系统功能,促进细胞免疫、体液免疫等免疫反应,增强细胞的抵抗力,保护细胞免受病毒、细菌等病原体的感染。
4. 维持蛋白质结构稳定性谷胱甘肽可以与蛋白质发生反应,形成可稳定蛋白质的二硫键,从而维持蛋白质的结构稳定性,保护蛋白质不受蛋白质氧化、降解等损伤。
二、谷胱甘肽的药理学应用1. 抗癌作用谷胱甘肽可以通过抑制癌细胞的生长、调节肿瘤免疫反应等多种机制发挥抗癌作用。
目前已经有许多研究表明,谷胱甘肽可以用于治疗肿瘤,尤其是与化疗、放疗联合应用可以增强治疗效果。
2. 神经系统疾病治疗谷胱甘肽可以通过调节神经系统氧化状态、抑制神经元死亡等机制,对神经系统疾病如帕金森病、阿尔兹海默病等有一定的治疗效果。
3. 心血管疾病治疗谷胱甘肽可以通过改善血管内皮细胞功能、抑制血小板凝聚等机制,具有一定的治疗心血管疾病的效果。
例如,研究表明谷胱甘肽可以降低血压、预防心血管疾病等。
4. 其他应用谷胱甘肽还可以应用于肝病、糖尿病、防辐射等领域,具有较好的临床应用效果。
三、结论总之,作为一种生物分子,谷胱甘肽具有多种生物学功能,涉及抗氧化、解毒、免疫调节等多种生物学作用。
同时,谷胱甘肽还具有广泛的药理学应用,包括治疗癌症、神经系统疾病、心血管疾病等方面。
谷胱甘肽 二硫键

谷胱甘肽二硫键谷胱甘肽(Glutathione)是一种由谷氨酸、半胱氨酸和甘氨酸组成的三肽,是人体内一种重要的抗氧化剂。
谷胱甘肽具有非常强的还原能力,可以帮助清除体内的自由基和有害物质,保护细胞免受氧化损伤。
在人体内,谷胱甘肽还参与调节免疫系统、蛋白质合成和DNA 修复等重要生理活动。
同时,它还能影响人体内许多重要酶和蛋白的活性,对抗细胞凋亡和老化,维护人体健康。
为了更好地了解谷胱甘肽的作用机制,有必要深入探讨其分子结构和生物学功能。
由于谷胱甘肽中含有两个半胱氨酸残基,使其具有很强的还原性。
这两个半胱氨酸残基之间通过二硫键(S-S键)相连,形成谷胱甘肽的三维结构。
这个二硫键不仅赋予了谷胱甘肽其特殊的生化性质,也为其在生物体内的功能发挥提供了重要的基础支持。
谷胱甘肽的分子结构和二硫键谷胱甘肽的分子式为C10H17N3O6S,分子量为307.33 g/mol。
其分子结构中含有一个谷氨酸残基、一个半胱氨酸残基和一个甘氨酸残基,它们通过肽键相互连接而成。
其中,半胱氨酸残基含有巯基(-SH基),在细胞内可以发生氧化反应而形成二硫键,使得两个半胱氨酸残基之间形成S-S键,从而构成谷胱甘肽的分子结构。
二硫键是指两个硫原子通过共价键连接在一起的结构,对于蛋白质的空间构象和生物活性具有非常重要的影响。
在谷胱甘肽中,二硫键的存在使得其分子呈现出特殊的立体构象,从而决定了它的生物学功能。
此外,二硫键的形成还使谷胱甘肽在生物体内能够稳定存在,不易受到氧化反应的影响。
谷胱甘肽的生物学功能谷胱甘肽作为一种抗氧化剂,具有重要的生物学功能。
在细胞内,它可以与氧化物质发生还原反应,将其转化为无害的物质,减少氧化应激对细胞的损害。
同时,谷胱甘肽还可以与其他抗氧化物质如维生素C和维生素E等相互作用,增强它们的抗氧化能力。
这些功能使得谷胱甘肽在减少细胞氧化损伤、预防衰老和抵抗疾病方面发挥着重要作用。
此外,谷胱甘肽还参与调节细胞内的氧化还原平衡,维持细胞内环境的稳定。
肝脏损伤机制PPT课件

1
肝对化学物的摄取和浓缩
2
化学物代谢活化与解毒
3
破坏细胞骨架
4
线粒体损伤
5
胆汁淤积
6
肝血窦细胞活化
7
炎症和免疫反应
肝对化学物的摄取和浓缩
❖首过性效应 ❖亲脂化学物易于扩散进入肝细胞 ❖肝脏主动摄取和贮存的生理过程可使毒物
在肝脏蓄积 ❖肝脏对毒物的易感性较高
化学物代谢活化与解毒
❖ 肝是外源化学物最主要的代谢器官
举例: 对乙酰氨基酚 四氯化碳
对乙酰氨基酚代谢活化和肝毒性发生过程中的关键事件
❖ 对乙酰氨基酚
激活剂 [如维生素A,内毒素]
肝细胞
CYP2E1 CYP3A
NAPA1形成 GSH耗竭
信号(??)
枯否细胞
“预激”肝细 胞
细胞死亡
细胞因子
活性枯否细胞
影响CCl4肝损伤程度的因素
保护作用因素包括
❖ CYP450活性低的幼小动物 ❖ CYP450抑制剂处理 ❖ 用小剂量同一毒物预处理降低肝CYP450水平
微囊藻素
胆盐
有机阴离子 微管蛋白
激酶
磷酸酶
大剂量
微管 蛋白 微囊藻素
肝细胞 变形
磷酸化 细胞骨架 微管蛋白
磷酸化微管蛋白
小剂量
微管动蛋白微囊藻素
分泌和 输出减少
磷酸化微管蛋白
胆汁淤积
六种致胆汁淤积的潜在机制
摄取障碍
胆小管伸缩性下降
转胞吞作用减低
细胞连接泄漏
分泌障碍
活性物质富集
线粒体损伤
❖ 代谢失衡和/或氧化应激反应
增加毒性的因素包括
❖ 缺氧 ❖ 糖尿病 ❖ 膳食维生素E缺乏 ❖ 其他化学物预处理(如乙醇、丙酮)
nac预处理细胞原理
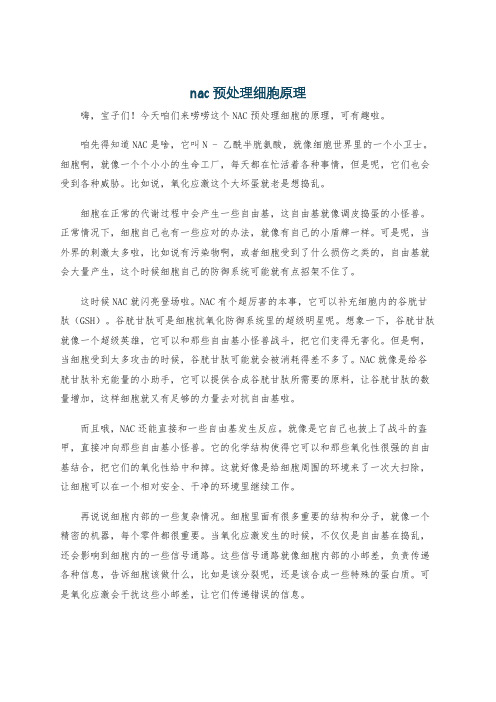
nac预处理细胞原理嗨,宝子们!今天咱们来唠唠这个NAC预处理细胞的原理,可有趣啦。
咱先得知道NAC是啥,它叫N - 乙酰半胱氨酸,就像细胞世界里的一个小卫士。
细胞啊,就像一个个小小的生命工厂,每天都在忙活着各种事情,但是呢,它们也会受到各种威胁。
比如说,氧化应激这个大坏蛋就老是想捣乱。
细胞在正常的代谢过程中会产生一些自由基,这自由基就像调皮捣蛋的小怪兽。
正常情况下,细胞自己也有一些应对的办法,就像有自己的小盾牌一样。
可是呢,当外界的刺激太多啦,比如说有污染物啊,或者细胞受到了什么损伤之类的,自由基就会大量产生,这个时候细胞自己的防御系统可能就有点招架不住了。
这时候NAC就闪亮登场啦。
NAC有个超厉害的本事,它可以补充细胞内的谷胱甘肽(GSH)。
谷胱甘肽可是细胞抗氧化防御系统里的超级明星呢。
想象一下,谷胱甘肽就像一个超级英雄,它可以和那些自由基小怪兽战斗,把它们变得无害化。
但是啊,当细胞受到太多攻击的时候,谷胱甘肽可能就会被消耗得差不多了。
NAC就像是给谷胱甘肽补充能量的小助手,它可以提供合成谷胱甘肽所需要的原料,让谷胱甘肽的数量增加,这样细胞就又有足够的力量去对抗自由基啦。
而且哦,NAC还能直接和一些自由基发生反应。
就像是它自己也披上了战斗的盔甲,直接冲向那些自由基小怪兽。
它的化学结构使得它可以和那些氧化性很强的自由基结合,把它们的氧化性给中和掉。
这就好像是给细胞周围的环境来了一次大扫除,让细胞可以在一个相对安全、干净的环境里继续工作。
再说说细胞内部的一些复杂情况。
细胞里面有很多重要的结构和分子,就像一个精密的机器,每个零件都很重要。
当氧化应激发生的时候,不仅仅是自由基在捣乱,还会影响到细胞内的一些信号通路。
这些信号通路就像细胞内部的小邮差,负责传递各种信息,告诉细胞该做什么,比如是该分裂呢,还是该合成一些特殊的蛋白质。
可是氧化应激会干扰这些小邮差,让它们传递错误的信息。
NAC预处理细胞呢,就像是给这些小邮差保驾护航。
谷胱甘肽对大鼠急性肝损伤的保护作用

N C L H
1 2 3 3
8.730 61.112 30.556 8.730
33.09 36.62 33.84 34.94
0.068 0.561 0.390 0.157
7
论文要点
•CCl4使得大鼠产生氧化应激,即体内高活性分子如活性
氧自由基(ROS)和活性氮自由基(RNS)产生过多,氧化程度 超出抗氧化物的清除能力,氧化系统和抗氧化系统失衡,从 而导致组织损伤。
8
论文要点
•还原型谷胱甘肽(GSH),
能参与体内氧化还原过程,清除 自由基,因此外源性GSH的补充对于肝损伤理论上是有保 护作用的。实验中高浓度GSH组和低浓度GSH组的大鼠的血 清中ALT含量较模型组均有不同程度的降低,肝匀浆MDA活 性也有所减小,表明预防性地给予外源性的GSH在一定程 度上起到抗自由基所导致的质膜损伤和脂质过氧化作用, 这与理论相符合。
还原型谷胱甘肽对四氯化碳致大 鼠急性肝损伤的保护作用
1
背景
在国内,已有研究表明还原型-谷胱甘肽对肝损伤有保护作 用,但缺少不同剂量的还原型-谷胱甘肽对肝损伤的保护 效果差异的研究。 故本实验给大鼠注射高浓度和低浓度的还原型-谷胱甘肽, 通过测其血清谷丙转氨酶活性和肝组织中丙二醛的含量来 比较两个浓度下对肝保护的效果。
4
结果
表1 各组大鼠大体肝脏外观比较
组别
肝脏外观
N
正常红棕 色,表面 光滑
C
土黄色 (++), 表面有出 血点
L
土黄色 (++), 表面有出 血点
H
土黄色 (++),表 面出血点较 少
5
6
表2 各组大鼠血清ALT活性、肝指数 以及肝组织匀浆MDA活性
nac抗氧化剂机制
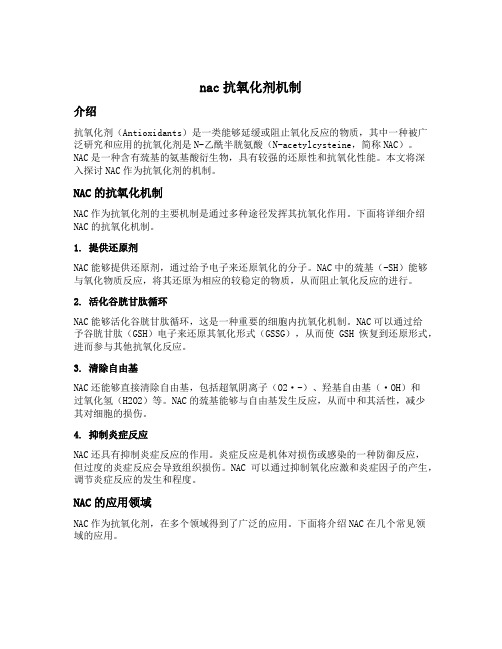
nac抗氧化剂机制介绍抗氧化剂(Antioxidants)是一类能够延缓或阻止氧化反应的物质,其中一种被广泛研究和应用的抗氧化剂是N-乙酰半胱氨酸(N-acetylcysteine,简称NAC)。
NAC是一种含有巯基的氨基酸衍生物,具有较强的还原性和抗氧化性能。
本文将深入探讨NAC作为抗氧化剂的机制。
NAC的抗氧化机制NAC作为抗氧化剂的主要机制是通过多种途径发挥其抗氧化作用。
下面将详细介绍NAC的抗氧化机制。
1. 提供还原剂NAC能够提供还原剂,通过给予电子来还原氧化的分子。
NAC中的巯基(-SH)能够与氧化物质反应,将其还原为相应的较稳定的物质,从而阻止氧化反应的进行。
2. 活化谷胱甘肽循环NAC能够活化谷胱甘肽循环,这是一种重要的细胞内抗氧化机制。
NAC可以通过给予谷胱甘肽(GSH)电子来还原其氧化形式(GSSG),从而使GSH恢复到还原形式,进而参与其他抗氧化反应。
3. 清除自由基NAC还能够直接清除自由基,包括超氧阴离子(O2·-)、羟基自由基(·OH)和过氧化氢(H2O2)等。
NAC的巯基能够与自由基发生反应,从而中和其活性,减少其对细胞的损伤。
4. 抑制炎症反应NAC还具有抑制炎症反应的作用。
炎症反应是机体对损伤或感染的一种防御反应,但过度的炎症反应会导致组织损伤。
NAC可以通过抑制氧化应激和炎症因子的产生,调节炎症反应的发生和程度。
NAC的应用领域NAC作为抗氧化剂,在多个领域得到了广泛的应用。
下面将介绍NAC在几个常见领域的应用。
1. 肝脏保护NAC被广泛应用于肝脏保护。
肝脏是体内重要的代谢器官,容易受到氧化损伤的影响。
NAC能够通过抗氧化作用和解毒作用,保护肝细胞免受氧化损伤和毒性物质的伤害。
2. 心脑血管疾病NAC在心脑血管疾病的预防和治疗中也有一定的应用。
心脑血管疾病的发生与氧化应激密切相关,NAC作为抗氧化剂能够减轻氧化损伤,保护心脑血管系统的健康。
谷胱甘肽的功效与作用

谷胱甘肽的功效与作用谷胱甘肽(Glutathione)是一种由谷氨酸、半胱氨酸和甘氨酸组成的三肽,是细胞内最重要的抗氧化物质之一。
它存在于细胞核、线粒体和细胞质中,广泛存在于动、植物及微生物体内。
谷胱甘肽在生物体内具有多种重要的生理功能和药理作用,对人体健康具有重要的保护作用。
首先,谷胱甘肽可以作为细胞内抗氧化剂,对细胞内的氧化应激具有重要的保护作用。
氧化应激是指细胞的抗氧化系统不能有效清除自由基,导致自由基与生物大分子产生连锁反应,破坏细胞膜、DNA、蛋白质等生物大分子的稳定性,从而引发氧化损伤。
氧化应激可导致多种疾病的发生,如心脑血管病、糖尿病、癌症等。
而谷胱甘肽能够中和自由基,同时参与还原型谷胱甘肽还原酶的活化,进一步增强细胞内的抗氧化能力,保护细胞膜、DNA、蛋白质等生物大分子的稳定性,抵御氧化应激的损害,维持细胞内环境的稳定。
其次,谷胱甘肽对细胞内的解毒作用也至关重要。
细胞内的毒性物质包括化学物质、药物、肿瘤化疗药物等,它们通过活性氧、自由基等机制损伤细胞内的生物大分子。
而谷胱甘肽作为一种解毒剂,能够与这些毒性物质结合形成稳定的无毒物质,进而通过胆汁、尿液等排出体外,减少对细胞的损害。
此外,谷胱甘肽还能够通过调节多种解毒酶的活性,进一步增强细胞内的解毒能力,保护细胞免受损害。
此外,谷胱甘肽还可以促进机体免疫功能的增强,抑制炎症反应的发生。
免疫功能是机体抵御病原微生物入侵、维持正常组织结构和功能的重要保障。
而谷胱甘肽作为一种调节因子,可调节多种免疫细胞如T细胞、B细胞、NK细胞等免疫细胞的功能,增强其对病原微生物和肿瘤细胞的识别、杀伤和清除能力。
同时,谷胱甘肽还可以抑制炎症因子的合成和释放,降低炎症反应的强度,减少炎症对组织结构和功能的破坏。
此外,谷胱甘肽还具有调节细胞生长和凋亡的作用。
细胞的生长和凋亡是机体维持正常组织结构和功能的基本保障。
谷胱甘肽可以通过调节细胞内氧化还原态的平衡,影响多种蛋白质的活性和表达,从而调控细胞的生长和凋亡。
nac作用机制

NAC(N-乙酰半胱氨酸)的作用机制主要有以下几个方面:1.直接清除自由基:NAC可以通过给予自由基氢(-SH)供体,直接清除多种自由基,如超氧阴离子、羟自由基和过氧自由基,从而减少自由基对细胞的损害。
2.促进谷胱甘肽(GSH)合成:NAC可以进入细胞并通过授予GSH碱基中的半胱氨酸,促进GSH的合成。
GSH是体内最重要的细胞内抗氧化剂,可以清除氧自由基、过氧化物和其他有害物质,维护细胞的氧化还原平衡。
3.补充谷胱甘肽:NAC能够直接为细胞提供GSH前体分子,从而增加GSH的水平。
GSH的充足水平可增强细胞对氧化应激的抵抗能力,保护细胞免受氧自由基的伤害。
4.改善呼吸功能:NAC可刺激肺泡上皮Ⅱ型细胞分泌及形成表面活性物质,使肺泡有效扩张,增加气体的交换面积。
同时通过在激活抗蛋白酶,抑制多形核中性粒细胞释放的弹性酶对肺泡弹性蛋白的破坏作用,从而保护肺泡弹性。
5.抗氧化作用:氧化作用派生的自由基在肺部的病理生理学中起重要作用,吸烟或从炎性细胞(嗜中性白细胞或巨嗜细胞)中释放的内源性物质均可导致自由基的增加。
NAC的抗氧化作用可以帮助减少由这些因素引发的自由基的产生。
6.增强机体的免疫功能:NAC能抑制病毒的复制、刺激T—淋巴细胞的复制应答,增加T—淋巴细胞亚群的数量及活性、增加巨嗜细胞的黏附和吞噬作用,保护细胞免受病毒感染引起的相关损害,从而起到预防及减少呼吸道感染的作用。
7.降低气道内细菌的数量:慢性支气管炎患者恶化率的增加可能与患者支气管内菌群类型的改变或免疫缺陷有关。
NAC具有三种抗氧化作用,包括直接作用于自由基(无酶参加反应)、抑制病毒的复制以及刺激T淋巴细胞的复制应答。
8.保护肝细胞:NAC可以减轻对乙酰氨基酚引起的肝毒性,对于慢阻肺病的治疗也有一定效果。
9.其他应用:部分研究显示,NAC可能有助于预防和改善帕金森症、阿尔茨海默氏症、自闭症、严重肾脏病和女性多囊卵巢不孕等症状。
以上信息仅供参考,如有需要,建议查阅相关文献或咨询专业医生。
nac抗氧化剂机制

nac抗氧化剂机制
摘要:
1.NAC 的介绍
2.NAC 的抗氧化机制
3.NAC 的应用
正文:
【1.NAC 的介绍】
-乙酰半胱氨酸(NAC)是一种广泛应用于医药和食品领域的抗氧化剂。
它是半胱氨酸的衍生物,具有较强的还原性,能有效地对抗自由基,保护细胞免受氧化损伤。
【2.NAC 的抗氧化机制】
AC 的抗氧化机制主要体现在以下几个方面:
(1)直接还原自由基:NAC 分子中含有活性的硫基,可以与自由基发生反应,直接将其还原为相对稳定的分子,从而终止自由基链式反应。
(2)促进谷胱甘肽的合成:NAC 可以增加谷胱甘肽合成酶的活性,提高谷胱甘肽的合成水平。
谷胱甘肽是一种重要的抗氧化物质,能与自由基结合,保护细胞免受损伤。
(3)增强其他抗氧化酶的活性:NAC 可以刺激其他抗氧化酶的活性,如过氧化氢酶、超氧化物歧化酶等,增强细胞整体的抗氧化能力。
【3.NAC 的应用】
由于NAC 具有强大的抗氧化作用,因此在多个领域都有广泛应用:
(1)医疗领域:NAC 被用于治疗各种氧化应激相关的疾病,如肝损伤、肺部感染等。
此外,NAC 还可以预防心血管疾病,降低胆固醇,减缓动脉硬化进程。
(2)食品添加剂:NAC 作为食品抗氧化剂,可延长食品的保质期,防止油脂氧化,提高食品的营养价值。
(3)保健品:NAC 作为保健品,可以提高人体抗氧化能力,预防疾病,延缓衰老。
总之,NAC 作为一种重要的抗氧化剂,在多个领域都发挥着重要作用。
谷胱甘肽的作用与功效
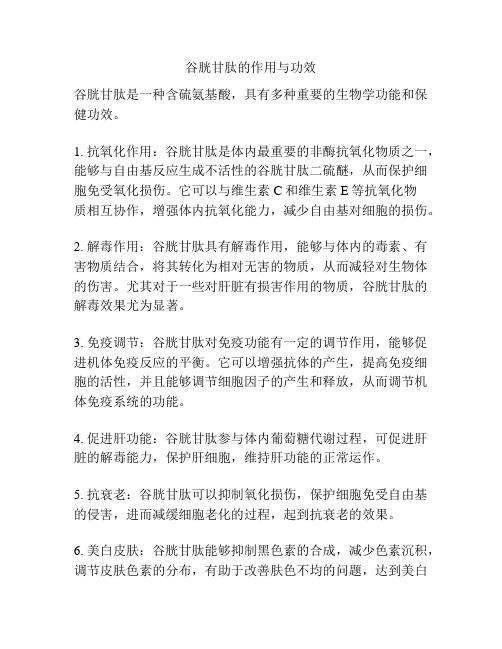
谷胱甘肽的作用与功效
谷胱甘肽是一种含硫氨基酸,具有多种重要的生物学功能和保健功效。
1. 抗氧化作用:谷胱甘肽是体内最重要的非酶抗氧化物质之一,能够与自由基反应生成不活性的谷胱甘肽二硫醚,从而保护细胞免受氧化损伤。
它可以与维生素C和维生素E等抗氧化物
质相互协作,增强体内抗氧化能力,减少自由基对细胞的损伤。
2. 解毒作用:谷胱甘肽具有解毒作用,能够与体内的毒素、有害物质结合,将其转化为相对无害的物质,从而减轻对生物体的伤害。
尤其对于一些对肝脏有损害作用的物质,谷胱甘肽的解毒效果尤为显著。
3. 免疫调节:谷胱甘肽对免疫功能有一定的调节作用,能够促进机体免疫反应的平衡。
它可以增强抗体的产生,提高免疫细胞的活性,并且能够调节细胞因子的产生和释放,从而调节机体免疫系统的功能。
4. 促进肝功能:谷胱甘肽参与体内葡萄糖代谢过程,可促进肝脏的解毒能力,保护肝细胞,维持肝功能的正常运作。
5. 抗衰老:谷胱甘肽可以抑制氧化损伤,保护细胞免受自由基的侵害,进而减缓细胞老化的过程,起到抗衰老的效果。
6. 美白皮肤:谷胱甘肽能够抑制黑色素的合成,减少色素沉积,调节皮肤色素的分布,有助于改善肤色不均的问题,达到美白
肌肤的效果。
总之,谷胱甘肽具有重要的抗氧化、解毒、免疫调节等多种功效,对于维护身体健康、预防衰老以及改善皮肤问题都是有益的。
N-乙酰半胱氨酸防治内毒素所致肝损伤的实验研究

N-乙酰半胱氨酸防治内毒素所致肝损伤的实验研究吴虎山;许瑞龄【期刊名称】《山西医药杂志》【年(卷),期】2005(034)001【摘要】目的探讨N-乙酰半胱氨酸(NAC)可否对内毒素性肝损伤进行抑制及相应的细胞、分子机制.方法健康雄性昆明种小鼠30只随机分为肝损伤组、NAC组和对照组,不同给药后检测肝匀浆肿瘤坏死因子(TNF)-α、丙二醛(MDA)和还原型谷胱甘肽(GSH)含量变化,并行聚丙烯酰胺凝胶电泳,分析NAC对核因子(NF)-κB p65、IκBα的影响.结果 NAC不但使肝组织TNF-α、MDA含量降低,GSH含量升高,且抑制了内毒素诱导胞质IκBα降解和NF-κB p65的表达.结论 NAC可能通过调整库普弗细胞氧化还原平衡,影响NF-κB p65活化(核易位),从而抑制了TNF-α等炎性因子基因的表达,减轻肝损伤.【总页数】2页(P27-28)【作者】吴虎山;许瑞龄【作者单位】山西医科大学,030001;山西医科大学,030001【正文语种】中文【中图分类】R3【相关文献】1.N-乙酰半胱氨酸和地塞米松对脂多糖所致大鼠急性肺损伤防治作用的比较 [J], 陈美云;邓志雄2.N-乙酰半胱氨酸在肠缺血再灌注肝损伤中保护作用的实验研究 [J], 刘维明;顾志成3.N-乙酰半胱氨酸对急性梗阻性化脓性胆管炎时内毒素性急性肝损伤的保护作用[J], 薛强;刘作金;杨慷;涂兵;丁雄4.N-乙酰半胱氨酸联合双环醇治疗抗结核药物所致的药物性肝损伤患者疗效研究[J], 杜云飞;刘景瑞;叶飞翔5.N-乙酰半胱氨酸对二甲基甲酰胺所致急性肝损伤的保护作用 [J], 郭凤华;杨文福;穆进军;姚汝琳;李美丽因版权原因,仅展示原文概要,查看原文内容请购买。
- 1、下载文档前请自行甄别文档内容的完整性,平台不提供额外的编辑、内容补充、找答案等附加服务。
- 2、"仅部分预览"的文档,不可在线预览部分如存在完整性等问题,可反馈申请退款(可完整预览的文档不适用该条件!)。
- 3、如文档侵犯您的权益,请联系客服反馈,我们会尽快为您处理(人工客服工作时间:9:00-18:30)。
Acetaminophen (AP AP), a commonly used analgesic, is usually safe when administered at therapeutic doses. How-ever, AP AP overdose causes liver injury both in experimental animals and humans. AP AP has been used extensively to de-velop an animal model of drug-induced liver injury. The toxi-city is initiated by cytochrome P450 (CYP) metabolism into N -acetyl-p -benzoquinone imine (NAPQI), and the high reac-tivity of NAPQI with sulfhydryl groups results in depletion of reduced glutathione (GSH) in hepatocytes, followed by covalent binding to intracellular proteins.1—3)Therefore, he-patic GSH levels are important in protecting against AP AP hepatotoxicity, and enhancement of hepatic GSH is a reason-able strategy for the treatment of AP AP intoxication.N -Acetylcysteine (NAC) is clinically used as an antidote for AP AP intoxication.4)It is thought that NAC provides cys-teine (CYS) as a precursor of GSH which traps NAPQI, lead-ing to a decrease in toxicity (Fig. 1).5)However, NAC was shown to improve patient outcome after late administration 6);this could not be explained by trapping NAPQI. In fact, treat-ment of mice with NAC after AP AP administration protected against hepatic necrosis without reducing covalent binding,suggesting that mechanisms other than GSH replenishment are involved in the hepatoprotective effects of NAC.7—9)In contrast to NAC, GSH itself is not used as an antidote for AP AP intoxication. It is believed that intraperitoneal ad-ministration of GSH is less effective in restoring hepatic GSH, probably because exogenous GSH can not be taken up by hepatocytes, whereas ethyl esters of GSH are proposed as GSH precursors that are taken up and hydrolyzed by hepato-cytes into GSH (Fig. 1).10)On the other hand, intravenously administered GSH was found to protect against AP AP hepa-totoxicity.11)I t is considered that intravenous GSH rapidlydegrades in the kidney, is absorbed as amino acids, and is used to synthesize GSH in the liver, which could led to he-patoprotection. These studies suggest the potential impor-tance of restoring hepatic GSH levels in the protection against APAP hepatotoxicity by exogenous thiol compounds.The objective of this study was to clarify the role of GSH supplementation in the hepatoprotective action of NAC and other thiol compounds, which could lead to the development of a new hepatoprotective agent. We found that a relatively low dose of thiol compounds, GSH, NAC, CYS, and glu-tathione-monoethyl ester (GSH-EE), protected mice against AP AP hepatotoxicity together with different abilities to re-366V ol. 34, No. 3Regular Article Protective Effects of Exogenous Glutathione and Related Thiol Compounds against Drug-Induced Liver InjuryY asuhiro M ASUBUCHI ,* Junpei N AKA Y AMA , and Yuka S ADAKATALaboratory of Clinical Pharmacy, Faculty of Pharmace utical Scie nce s, Chiba Institute of Scie nce ; 15–8 Shiomi-cho,Choshi, Chiba 288–0025, Japan.Received October 27, 2010; accepted December 15, 2010; published online December 15, 2010An overdose of acetaminophen (AP AP) causes liver injury both in experimental animals and humans. N -Acetylcysteine (NAC) is clinically used as an antidote for AP AP intoxication, and it is thought to act by providing cysteine as a precursor of glutathione, which traps a reactive metabolite of AP AP . Other hepatoprotective mecha-nisms of NAC have also been suggested. Here, we examined the effects of thiol compounds with different abilities to restore hepatic glutathione, on hepatotoxicity of AP AP and furosemide in mice. Overnight-fasted male CD-1mice were given AP AP or furosemide intraperitoneally. NAC, cysteine, glutathione, or glutathione-monoethyl ester was administered concomitantly with AP AP or furosemide. All thiol compounds used in this study effec-tively protected mice against AP AP-induced liver injury. Only glutathione-monoethyl ester completely prevented AP AP-induced early hepatic glutathione depletion. Cysteine also significantly restored hepatic glutathione levels.NAC partially restored glutathione levels. Exogenous glutathione had no effect on hepatic glutathione loss. NAC and glutathione highly stimulated the hepatic expression of cytokines, particularly interleukin-6, which might be involved in the alleviation of AP AP hepatotoxicity. Furosemide-induced liver injury, which does not accompany hepatic glutathione depletion, was also attenuated by NAC and exogenous glutathione, supporting their protec-tive mechanisms other than replenishment of glutathione. In conclusion, exogenous thiols could alleviate drug-induced liver injury. NAC and glutathione might exert their effects, at least partially, via mechanisms that are in-dependent of increasing hepatic glutathione, but probably act through cytokine-mediated and anti-inflammatory mechanisms.Key wordshepatotoxicity; glutathione; N -acetylcysteine; acetaminophen; furosemide; interleukin-6Biol. Pharm. Bull.34(3) 366—370 (2011)© 2011 Pharmaceutical Society of Japan∗To whom correspondence should be addressed.e-mail: ymasubuchi@cis.ac.jpFig.1.Pathways for Biosynthesis of GSH and Chemical Structures of Thiol Compounds Used in This Studystore hepatic GSH. We also examined the effects of these thiol compounds on hepatic cytokine expression, which is in-volved in pathogenesis and prevention of AP AP hepatotoxic-ity. Furthermore, the effects of thiol compounds were exam-ined using furosemide (FS) as a hepatotoxic agent which does not deplete GSH prior to the development of hepatotox-icity.MATERIALS AND METHODSChemicals AP AP was purchased from Sigma-Aldrich (St. Louis, MO, U.S.A.). GSH, NAC, CYS, FS, diethyldithio-carbamic acid, and ketoconazole were purchased from Wako Pure Chemical Ind. (Osaka, Japan). GSH-EE was purchased from Bachem AG (Bubendorf, Switzerland). All chemicals and solvents used in this study were of analytical grade.Animals and in Vivo Treatment Male and female CD-1mice were purchased from Japan SLC, I nc. (Shizuoka,Japan). The mice were acclimatized for at least 1 week in a climate-controlled room on a 12-h light–dark cycle and were fed ad libitum . The mice were used in our experiment at 9—10 weeks of age. All procedures and care techniques were performed according to the National I nstitutes of Health ‘Guide for the Care and Use of Laboratory Animals.’ The mice were fasted for 16h before experimentation to sensitize them to AP AP hepatotoxicity by decreasing basal levels of liver GSH. The mice received either 300mg/kg AP AP or 400mg/kg FS in 10ml/kg saline intraperitoneally (i.p.). Con-trol mice received only 10ml/kg saline vehicle. NAC (250mg/kg, i.p.) or an equimolar (1.5mmol/kg) dose of another thiol compound (CYS, GSH, or GSH-EE) was coadminis-tered with AP AP or FS. In some experiments, diethyldithio-carbamic acid (200mg/kg, i.p.), or ketoconazole (50mg/kg,i.p.) was coadministered with AP AP or FS. The mice were then sacrificed to obtain their blood and livers at 3 or 24h after the administration of AP AP or FS. Blood samples were allowed to coagulate and then were centrifuged to obtain serum.Assessment of Hepatotoxicity Serum alanine amino-transferase (ALT) activity was assayed as a marker of drug-induced hepatotoxicity. Assays were run on a test kit (Sigma Diagnostics, St. Louis, MO, U.S.A.).Assay of Hepatic GS H Levels Liver tissues (100mg)were homogenized in 1.0ml 0.5 % sulfosalicylic acid. GSH concentrations in liver homogenates were determined by the dithionitrobenzoic acid-glutathione disulfide reductase recy-cling assay.12)Assays were run on a GSH test kit (Dojindo Laboratories, Kumamoto, Japan).RNA Isolation from Liver Liver tissues (approximately 60mg) were homogenized in 1.2ml RLT buffer (Qiagen Inc.,Valencia, CA, U.S.A.) including 1% mercaptoethanol, and total cellular RNA was extracted according to the manufac-turer’s instructions. The isolated RNA was quantitated by spectrophotometric analysis at 260nm.mRNA Quantification of Tumor Necrosis Factor-a (TNF-a ) and Interleukin-6 (IL-6) by Reverse Transcrip-tion-Polymerase Chain Reaction (RT-PCR)Real-time RT -PCR was performed to quantify the mRNA expression of TNF-a and L-6 genes with modifications of described methods.13)Total RNA (0.8m g) from each sample was tran-scribed into complementary DNA (cDNA) using high-capac-ity cDNA reverse transcription kits (Applied Biosystems,Foster City, CA, U.S.A.). Real-time RT -PCR was performed using TaqMan Gene Expression Assays (Applied Biosys-tems) for mouse TNF-a (Mm00443258_m1), mouse I L-6(Mm99999064_m1), and mouse glyceraldehyde 3-phosphate dehydrogenase (G3PDH, Mm99999915_g1). The reaction mixture contained one of these probes, TaqMan Universal PCR Master Mix (Applied Biosystems), and cDNA obtained as described above. Amplification and further analysis were performed by the ABI 7500 Real-Time PCR System (Ap-plied Biosystems), which were initiated at 50°C for 2min and 95°C for 10min, followed by 40 cycles of denaturation at 95°C for 15s and annealing/extension at 60°C for 1min.The intensity for mRNA expression of TNF-a and IL-6 thus obtained were normalized relative to G3PDH expression for each sample.Statistical Analysis Statistical comparisons between two groups were performed using Student’s t -test. Analyses in-volving more than two groups were compared using analysis of variance, followed by Dunnett’s multiple comparison test to determine significant differences between group means.RESULTSProtective Effects of GS H and Its Related Thiols against AP AP Hepatotoxicity and AP AP-Induced Hepatic GS H Depletion Overnight-fasted male CD-1 mice were administered 300mg/kg AP AP . NAC, CYS, GSH, or GSH-EE were administered concomitantly with AP AP , and hepato-toxicity was assessed at 3 and 24h after AP AP administration (Fig. 2). Treatment of mice with AP AP caused liver injury as assessed by increases in serum ALT levels, and all thiols used in this study protected mice against AP AP-induced liver injury almost completely. Hepatic GSH rapidly declined but recovered thereafter, as assessed at 3 and 24h after AP AP ad-ministration (Fig. 3). Only GSH-EE completely prevented AP AP-induced early hepatic GSH depletion. Cysteine also significantly but not completely restored hepatic glutathione levels. NAC produced partial glutathione restoration. How-ever, GSH administration was ineffective in protectingMarch 2011367Fig.2.Protection against AP AP-Induced Liver Injury by Thiol DerivativesMice were administered AP AP 300mg/kg alone or with various thiol derivatives, and were sacrificed 3 or 24h after administration for assay of serum ALT. Results are means ϮS.E. Numbers of mice used are 6 (AP AP) and 3 (other groups). ∗p Ͻ0.05 vs.control; †p Ͻ0.05 vs.AP AP alone.against hepatic GSH loss by AP AP treatment, which is con-sistent with previous observations that exogenous GSH is less effective in restoring hepatic GSH than GSH-EE.10)Altered Ratio of Hepatic Cytokine Expression Follow-ing Administration of GS H Liver TNF-a and I L-6mRNA expression in mice administered AP AP with or with-out a thiol compound was measured by real-time RT -PCR.TNF-a is a typical proinflammatory cytokine, and some studies suggest its involvement in the progression of AP AP hepatotoxicity.14,15)On the other hand, I L-6 is a pleiotropic cytokine, which may behave as an anti-inflammatory cy-tokine and protect mice against AP AP hepatotoxicity.16)He-patic mRNA expression of these two cytokines was measured 3h after AP AP administration (Fig. 4). Both TNF-a and IL-6were modestly but significantly induced by administration of AP AP alone. Coadministration of every thiol with AP AP po-tentiated the induction of hepatic TNF-a and IL-6. Notably,coadministration of NAC and GSH markedly potentiated the induction of hepatic IL-6. Accordingly, a ratio of TNF-a to IL-6, a postulated proinflammatory/anti-inflammatory index,was decreased in those mice administered AP AP with GSH (0.166) or NAC (0.327), compared with AP AP administra-tion alone (0.512). None of the thiol compounds affected the hepatic expressions of TNF-a or IL-6 in the mice not given AP AP (data not shown).Protective Effects of NAC and GSH against FS Hepato-toxicity FS, a loop diuretic, is converted into a reactive metabolite and causes hepatic necrosis in mice as well as AP AP .17)In the present study, FS at a dose of 400mg/kg was subjected to induce hepatotoxicity. Diethyldithiocarbamate or ketoconazole, inhibitors of mouse CYP2E118)and CYP3A11,19)respectively, protected mice against FS- as well as AP AP-induced hepatotoxicity (Fig. 5A). Despite a poten-tial role of the P450-mediated reactive metabolite in FS he-patotoxicity, hepatic GSH was unchanged in those mice treated with FS (Fig. 5B), which agrees with previous obser-vations.20,21)We examined the protective effects of thiol com-pounds on FS hepatotoxicity, which does not require hepatic368Vol. 34, No. 3Fig.3.Protection against AP AP-Induced Hepatic GSH Depletion by Thiol DerivativesMice were administered AP AP 300mg/kg alone or with various thiol derivatives, and were sacrificed 3 and 24h after administration for assay of liver GSH. Results are means ϮS.E. Numbers of mice used are 4 (AP AP) and 3 (other groups). ∗p Ͻ0.05 vs.control; †p Ͻ0.05 vs.APAP alone.Fig.4.Hepatic TNF-a and I L-6 Expression in AP AP-Treated Mice and Additional Effects of Thiol CompoundsMice were administered AP AP 300mg/kg alone or with various thiol compounds,and were sacrificed 3h after administration. Hepatic mRNA expression of TNF-a (A)and IL-6 (B) are presented as ratios to GAPDH. Results are means ϮS.E. Numbers of mice used are 4 (AP AP) and 3 (other groups). ∗p Ͻ0.05 vs.control; †p Ͻ0.05 vs.AP APalone.Fig.5.Roles of Reactive Metabolites in AP AP- and FS-Induced Liver In-juryMice were administered AP AP 300mg/kg alone or FS 400mg/kg alone, or with di-ethyldithiocarbamate (ϩDDC) or ketoconazole (ϩKCZ), and were sacrificed 3 or 24h after administration. (A) Serum ALT 24h after administration. Results are means ϮS.E.Numbers of mice used are 5 (AP AP and FS) and 3 (other groups). ∗p Ͻ0.05 vs.control;†p Ͻ0.05 vs.no inhibitor. (B) Liver GSH levels 3 or 24h after administration. Results are means ϮS.E. of 3 mice. ∗p Ͻ0.05 vs.control (C).Fig.6.Protection against FS-Induced Liver Injury by Thiol CompoundsMice were administered FS alone or with various thiol derivatives, and were sacri-ficed 24h after administration for assay of serum ALT. Results are means ϮS.E. Num-bers of mice used are 6 (FS) and 3 (other groups). ∗p Ͻ0.05 vs.control; †p Ͻ0.05 vs.FS alone.GSH depletion prior to the development of hepatotoxicity. Among the four thiols, NAC and GSH alleviated FS hepato-toxicity, whereas no significant effect of GSH-EE or CYS was observed (Fig. 6). This also supports the notion that he-patoprotection by NAC and GSH could be mediated by a mechanism other than GSH supplementation.DISCUSSIONI n the present study, coadministration of a relatively low dose (1.5mmol/kg) of NAC, CYS, GSH, and GSH-EE effec-tively protected mice against AP AP-induced liver injury. By contrast, their effects on an AP AP-induced early decrease in hepatic GSH were distinctly different from each other. Only GSH-EE completely prevented AP AP-induced early hepatic GSH depletion. CYS was also effective in preventing an AP AP-induced decrease in hepatic GSH, while NAC showed a partial GSH restoration. Unlike the other thiols, exogenous GSH had no effect on hepatic GSH loss in AP AP-treated mice. Therefore, the protective action of GSH-EE and CYS can be mainly explained by GSH replenishment. NAC exerts its effects not only via GSH replenishment but also other mechanisms. Exogenous GSH could protect mice against AP AP hepatotoxicity without replenishing hepatic GSH lev-els.The major mechanism underlying protection against AP AP hepatotoxicity by NAC is thought to provide CYS as a pre-cursor of GSH, which traps NAPQI.5)However, treatment of mice with NAC 1—2h after AP AP protected against hepatic necrosis without preventing covalent binding, suggesting that mechanisms other than GSH replenishment are involved in the hepatoprotective effects of NAC.7—9)These include in-hibiting the production of reactive nitrogen species and/or proinflammatory cytokines,7)which are pathogenic events in AP AP hepatotoxicity.14,15)In the present study, coadministra-tion of NAC with AP AP highly potentiated the early induc-tion of hepatic TNF-a and I L-6; the latter was more pro-nounced, leading to a decreased ratio of TNF-a/I L-6, an index of the Th1/Th2 cytokine balance. Disruption of this balance is responsible for the pathogenesis of AP AP hepato-toxicity,13)and I L-6 is one of the protective factors against it,16)Therefore, NAC could exert its hepatoprotective effects by increasing IL-6 expression and improving the Th1/Th2 re-sponse. The induction of cytokines, including IL-6, in AP AP-treated mice and their suppression by NAC at relatively later time points might be due to the response to hepatotoxicity and its attenuation by NAC.7)Intravenous injections of GSH have been reported to pro-tect mice against AP AP hepatotoxicity by restoring hepatic GSH levels.11)The mechanisms underlying exogenous GSH are explained as follows: GSH is rapidly degraded after intra-venous administration, and is taken up by the liver as amino acids where it is regenerated in hepatocytes. On the other hand, the present study shows the lack of restoration of he-patic GSH after intraperitoneal administration of GSH. This may be due to differences in the route of administration, be-cause the absence of any effect following the intraperitoneal injection of GSH on hepatic GSH levels is consistent with a previous report, which suggested that GSH could not be taken up by hepatocytes.10)It is thus suggested that GSH acts from outside of the hepatocyte membrane, and/or GSH-induced hepatoprotection is mediated by other endogenous components. GSH administration induced IL-6, and this se-lectivity (ratio to TNF-a) is more pronounced than NAC ad-ministration. Therefore, GSH could exert its effects mainly via an increased production of the anti-inflammatory cy-tokine. The present results provide novel insight into the he-patoprotection mechanisms of thiol compounds, particularly GSH, which are independent of supplementation of hepatic GSH.To verify further these findings, we next evaluated effects of the thiol compounds on a non-AP AP model of hepatotoxi-city, which is not accompanied by depletion of hepatic GSH. FS is a loop diuretic frequently used in the treatment of car-diovascular and renal diseases. I t was reported to be con-verted into a reactive metabolite and shown to cause hepatic necrosis as well as AP AP in mice.17)In the present study, we confirmed that hepatotoxicity requires metabolic activation, possibly by CYP2E1 and CYP3A11, because of its protec-tion by diethyldithiocarbamate and ketoconazole. Unlike AP AP, FS hepatotoxicity did not accompany changes in he-patic GSH levels as previously observed.20,21)Interestingly, only NAC and GSH, both of which could protect mice against AP AP hepatotoxicity via GSH-independent mecha-nisms as described above, attenuated FS hepatotoxicity. Thus, the FS model supports the idea that a protective mech-anism of NAC and GSH involves factors other than GSH re-plenishment, such as cytokine modulation.In summary, exogenous thiols alleviate AP AP-induced he-patotoxicity, some of which mainly mediate GSH replenish-ment, and others of which mediate without increasing he-patic GSH, probably through cytokine-mediated and anti-inflammatory mechanisms. A simple and reasonable theory classifying AP AP-induced liver injury has been proposed to involve two stages22): stage I includes metabolic activation, covalent binding, and mitochondrial dysfunction, while stage I I involves subsequent processes of adaptation or failure of the response in modifying essential cellular processes, which may include cytokine modulation. The latter mechanism could provide the rationale for late administration of NAC as an antidote of AP A hepatotoxicity. Furthermore, because NAC is associated with various adverse effects,23)GSH could also be considered as an antidote for AP AP intoxication. Acknowledgements This study was supported in part by a Grant-in-Aid for Scientific Research from the Ministry of Education, Culture, Sports, Science and Technology of Japan.REFERENCES1)Mitchell J. R., Jollow D. J., Potter W. Z., Davis D. C., Gillette J. R.,Brodie B. B., J. Pharmacol. Exp. Ther., 187, 185—194 (1973).2)Jollow D. J., Mitchell J. R., Potter W. Z., Davis D. C., Gillette J. R.,Brodie B. B., J. Pharmacol. Exp. Ther., 187, 195—202 (1973).3)Dahlin D. C., Miwa G. T., Lu A. Y., Nelson S. D., Proc. Natl. Acad.Sci. U.S.A., 81, 1327—1331 (1984).4)Prescott L. F., Park J., Ballantyne A., Adriaenssens P., Proudfoot A. T.,Lancet, 2, 432—434 (1977).5)Corcoran G. B., Wong B. K., J. Pharmacol. Exp. Ther., 238, 54—61(1986).6)Harrison P. M., Keays R., Bray G. P., Alexander G. J., Williams R.,Lancet, 335, 1572—1573 (1990).7)James L. P., McCullough S. S., Lamps L. W., Hinson J. A., Toxicol.Sci., 75, 458—467 (2003).March 20113698)Salminen W. F. Jr., V oellmy R., Roberts S. M., J. Pharmacol. Exp.Ther., 286, 519—524 (1998).9)Dambach D. M., Durham S. K., Laskin J. D., Laskin D. L., Toxicol.Appl. Pharmacol., 211, 157—165 (2006).10)Puri R. N., Meister A., Proc. Natl. Acad. Sci. U.S.A., 80, 5258—5260(1983).11)Knight T. R., Ho Y. S., Farhood A., Jaeschke H., J. Pharmacol. Exp.Ther., 303, 468—475 (2002).12)Anderson M. E., Methods Enzymol., 113, 548—555 (1985).13)Masubuchi Y., Sugiyama S., Horie T., Che m. Biol. Inte ract., 179,273—279 (2009).14)Blazka M. E., Wilmer J. L., Holladay S. D., Wilson R. E., Luster M. I.,Toxicol. Appl. Pharmacol., 133, 43—52 (1995).15)Ishida Y., Kondo T., Tsuneyama K., Lu P., Takayasu T., Mukaida N., J.Leukoc. Biol., 75, 59—67 (2004).16)Masubuchi Y., Bourdi M., Reilly T. P., Graf M. L., George J. W., PohlL. R., Biochem. Biophys. Res. Commun., 304, 207—212 (2003).17)Mitchell J. R., Nelson W. L., Potter W. Z., Sasame H. A., Jollow D. J.,J. Pharmacol. Exp. Ther., 199, 41—52 (1976).18)Carlson G., J. Toxicol. Environ. Health A, 67, 905—909 (2004).19)Martignoni M., Groothuis G., de Kanter R., Drug Metab. Dispos., 34,1047—1054 (2006).20)Wong S. G., Card J. W., Racz W. J., Toxicol. Le tt., 116, 171—181(2000).21)Randle L. E., Goldring C. E., Benson C. A., Metcalfe P. N., Kittering-ham N. R., Park B. K., Williams D. P., Toxicology, 243, 249—260 (2008).22)Bessems J. G., Vermeulen N. P., Crit. Re v. Toxicol., 31, 55—138(2001).23)Kao L. W., Kirk M. A., Furbee R. B., Mehta N. H., Skinner J. R.,Brizendine E. J., Ann. Emerg. Med., 42, 741—750 (2003).370V ol. 34, No. 3。