钾通道和盐关系
植物耐盐性和耐旱性的机制和应用价值
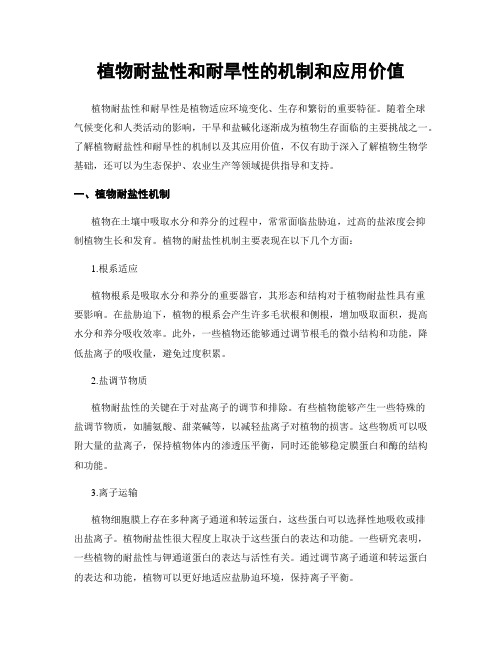
植物耐盐性和耐旱性的机制和应用价值植物耐盐性和耐旱性是植物适应环境变化、生存和繁衍的重要特征。
随着全球气候变化和人类活动的影响,干旱和盐碱化逐渐成为植物生存面临的主要挑战之一。
了解植物耐盐性和耐旱性的机制以及其应用价值,不仅有助于深入了解植物生物学基础,还可以为生态保护、农业生产等领域提供指导和支持。
一、植物耐盐性机制植物在土壤中吸取水分和养分的过程中,常常面临盐胁迫,过高的盐浓度会抑制植物生长和发育。
植物的耐盐性机制主要表现在以下几个方面:1.根系适应植物根系是吸取水分和养分的重要器官,其形态和结构对于植物耐盐性具有重要影响。
在盐胁迫下,植物的根系会产生许多毛状根和侧根,增加吸取面积,提高水分和养分吸收效率。
此外,一些植物还能够通过调节根毛的微小结构和功能,降低盐离子的吸收量,避免过度积累。
2.盐调节物质植物耐盐性的关键在于对盐离子的调节和排除。
有些植物能够产生一些特殊的盐调节物质,如脯氨酸、甜菜碱等,以减轻盐离子对植物的损害。
这些物质可以吸附大量的盐离子,保持植物体内的渗透压平衡,同时还能够稳定膜蛋白和酶的结构和功能。
3.离子运输植物细胞膜上存在多种离子通道和转运蛋白,这些蛋白可以选择性地吸收或排出盐离子。
植物耐盐性很大程度上取决于这些蛋白的表达和功能。
一些研究表明,一些植物的耐盐性与钾通道蛋白的表达与活性有关。
通过调节离子通道和转运蛋白的表达和功能,植物可以更好地适应盐胁迫环境,保持离子平衡。
二、植物耐旱性机制随着全球气候变化以及人类对水资源的过度开发,干旱逐渐成为植物面临的重要环境压力。
植物维持生命活动的同时还需要适应干旱环境,植物耐旱性的机制主要表现在以下几方面:1.保护细胞膜结构在干旱胁迫下,植物细胞膜的双层脂质会发生一系列调整,以减少失水。
植物细胞膜中富含脂质分子,而随着失水的增多,细胞膜的脂质分子会发生氧化,脂质过氧化反应等,破坏细胞膜结构。
植物通过表达一些相关基因,如LEA(late embryogenesis abundant)基因家族,保护改变细胞膜结构的过程,以适应干旱环境的压力。
植物对盐胁迫的生理学和分子生物学响应研究
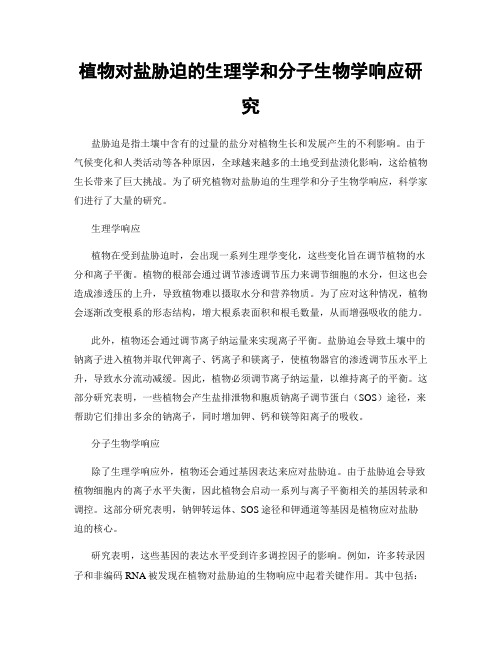
植物对盐胁迫的生理学和分子生物学响应研究盐胁迫是指土壤中含有的过量的盐分对植物生长和发展产生的不利影响。
由于气候变化和人类活动等各种原因,全球越来越多的土地受到盐渍化影响,这给植物生长带来了巨大挑战。
为了研究植物对盐胁迫的生理学和分子生物学响应,科学家们进行了大量的研究。
生理学响应植物在受到盐胁迫时,会出现一系列生理学变化,这些变化旨在调节植物的水分和离子平衡。
植物的根部会通过调节渗透调节压力来调节细胞的水分,但这也会造成渗透压的上升,导致植物难以摄取水分和营养物质。
为了应对这种情况,植物会逐渐改变根系的形态结构,增大根系表面积和根毛数量,从而增强吸收的能力。
此外,植物还会通过调节离子纳运量来实现离子平衡。
盐胁迫会导致土壤中的钠离子进入植物并取代钾离子、钙离子和镁离子,使植物器官的渗透调节压水平上升,导致水分流动减缓。
因此,植物必须调节离子纳运量,以维持离子的平衡。
这部分研究表明,一些植物会产生盐排泄物和胞质钠离子调节蛋白(SOS)途径,来帮助它们排出多余的钠离子,同时增加钾、钙和镁等阳离子的吸收。
分子生物学响应除了生理学响应外,植物还会通过基因表达来应对盐胁迫。
由于盐胁迫会导致植物细胞内的离子水平失衡,因此植物会启动一系列与离子平衡相关的基因转录和调控。
这部分研究表明,钠钾转运体、SOS途径和钾通道等基因是植物应对盐胁迫的核心。
研究表明,这些基因的表达水平受到许多调控因子的影响。
例如,许多转录因子和非编码RNA被发现在植物对盐胁迫的生物响应中起着关键作用。
其中包括:抗氧化反应、脱水诱导因子(DREB)和乙烯反应途径等。
这些因子通过调节与离子平衡相关的基因表达来维持植物生长和发展的正常状态。
未来展望现在,研究人员越来越关注植物对盐胁迫的生理学和分子生物学响应。
未来的研究可能会导致对抗盐渍化的新策略和技术,例如:转录因子的筛选和定向培育抗盐胁迫的新物种。
其次,将进一步掌握植物对盐胁迫的分子机制,建立正反馈机制,从而实现更好的调控效果。
钾代谢的基本原理与机制

01
02
03
04
排泄主要通过肾脏完成
排泄受多因素影响
排遗主要通过粪便完成
代谢平衡对人体健康至关重要
钾的排泄主要通过肾脏,约有90%以上的钾由肾脏排泄,其余10%通过肠道和皮肤排出。
钾的排泄受到多种因素的影响,如肾功能、饮食中钾的摄入量、药物的摄入等。
人体排遗的钾主要通过粪便排出,这是人体排除多余钾的主要方式。
钾平衡
代谢紊乱
肌肉功能
高钾血症
神经系统
防治高钾血症的方法
内分泌系统
钾离子
排钾
肾脏
离子通道
排钠
酸碱平衡
激素调节
高钾血症
钾代谢异常的诊断
保持身体健康的核心要素
废物排泄
呼吸系统
体温调节
神经系统
异常的诊断
病理
症状
病因
诊断
异常治疗需根据病因制定个性化方案
康复训练
病因
手术治疗
营养疗法
2.离子参与生物电的传导钾和钠是细胞膜电位的关键因素,它们在参与生物电传导中起到重要作用。当钾离子浓度在细胞膜内外差异较大时,形成电位差,从而形成生物电。钠离子在动作电位的产生和神经冲动的传导中起到关键作用。
3.代谢与心血管健康的关系钾和钠的代谢与心血管健康密切相关。高钠摄入会增加高血压和心血管疾病的风险,而高钾摄入则有助于降低血压和维护心血管健康。
02
失衡与心血管疾病的关系
钾代谢失衡可能导致心律失常、心肌肥厚等心血管问题。低钾血症或高钾血症都可能增加心血管疾病的风险。
钾与心血管健康的关系
THANK YOU
钾代谢的基本原理与机制
分享人-xxx
2024/2/26
无机盐的作用高中生物的题

无机盐的作用高中生物的题无机盐是指不含有机物质的化合物,由金属离子和非金属离子组成。
在生物体内,无机盐扮演着重要的角色,它们参与了多种生物化学反应和维持了生理功能的正常运转。
本文将从无机盐在维持水平衡、参与酶反应、传递神经信号、维持酸碱平衡、构建生物组织等方面详细介绍无机盐的作用。
首先,无机盐在维持水平衡方面扮演着重要角色。
在体内,无机盐通过维持渗透压的平衡来调节细胞的水分含量。
例如,钠离子和氯离子在体液中的浓度决定了细胞内外的渗透压,进而影响细胞对水的吸收和排泄。
如果水分摄入不足或排出过多,体内的无机盐浓度会发生改变,导致水平衡失调,造成脱水或水中毒的情况。
其次,无机盐参与了多种酶反应的进行。
酶是生物体内的催化剂,它们可以加速和调节生物化学反应的速率。
无机盐作为酶的辅助因子,可以影响酶活性并参与酶反应。
例如,锌离子是许多酶的活化剂,它与酶的活性中心结合,促进酶的催化作用。
另外,镁离子在DNA合成和核酸代谢过程中起到了重要的催化作用。
同时,无机盐在神经传递中也发挥着重要的作用。
神经系统通过神经元之间的化学和电信号传递来控制和调节身体的各种功能。
钠离子和钾离子是神经元细胞膜上的电解质,它们参与了神经传递过程中的电信号传导。
当神经兴奋时,钠离子通过神经元细胞膜的通道进入细胞内,从而使细胞内产生电位差,并导致神经信号的传递。
而钾离子则通过神经元细胞膜的通道从细胞内流出,恢复细胞的静息状态。
此外,无机盐还参与了维持酸碱平衡的过程。
生物体内的酶活性和代谢过程对于细胞内外环境的酸碱度非常敏感。
无机盐可以与氢离子或氢氧根离子结合,调节细胞外液和细胞内液的pH值。
例如,碳酸氢根离子和碳酸根离子在血液中维持了酸碱平衡的稳定状态。
最后,无机盐在构建生物组织中发挥了重要作用。
例如,钙离子是骨骼和牙齿的主要成分,在骨骼的构建和维持中起到了关键作用。
此外,磷酸盐也是DNA和RNA的主要组分,并参与能量分子ATP的合成和储存。
植物细胞无机盐的作用
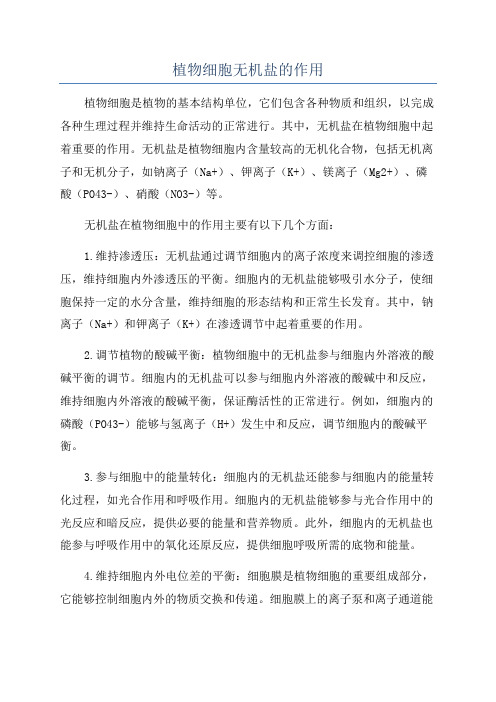
植物细胞无机盐的作用植物细胞是植物的基本结构单位,它们包含各种物质和组织,以完成各种生理过程并维持生命活动的正常进行。
其中,无机盐在植物细胞中起着重要的作用。
无机盐是植物细胞内含量较高的无机化合物,包括无机离子和无机分子,如钠离子(Na+)、钾离子(K+)、镁离子(Mg2+)、磷酸(PO43-)、硝酸(NO3-)等。
无机盐在植物细胞中的作用主要有以下几个方面:1.维持渗透压:无机盐通过调节细胞内的离子浓度来调控细胞的渗透压,维持细胞内外渗透压的平衡。
细胞内的无机盐能够吸引水分子,使细胞保持一定的水分含量,维持细胞的形态结构和正常生长发育。
其中,钠离子(Na+)和钾离子(K+)在渗透调节中起着重要的作用。
2.调节植物的酸碱平衡:植物细胞中的无机盐参与细胞内外溶液的酸碱平衡的调节。
细胞内的无机盐可以参与细胞内外溶液的酸碱中和反应,维持细胞内外溶液的酸碱平衡,保证酶活性的正常进行。
例如,细胞内的磷酸(PO43-)能够与氢离子(H+)发生中和反应,调节细胞内的酸碱平衡。
3.参与细胞中的能量转化:细胞内的无机盐还能参与细胞内的能量转化过程,如光合作用和呼吸作用。
细胞内的无机盐能够参与光合作用中的光反应和暗反应,提供必要的能量和营养物质。
此外,细胞内的无机盐也能参与呼吸作用中的氧化还原反应,提供细胞呼吸所需的底物和能量。
4.维持细胞内外电位差的平衡:细胞膜是植物细胞的重要组成部分,它能够控制细胞内外的物质交换和传递。
细胞膜上的离子泵和离子通道能够调控细胞内外离子的平衡,维持正常的细胞内外电位差。
细胞内的无机盐通过离子泵和离子通道的调节,参与细胞内外电位差的平衡。
5.参与细胞分裂和生长发育:细胞内的无机盐在植物的细胞分裂和生长发育过程中发挥重要的作用。
例如,钙离子(Ca2+)能够调节细胞的分裂和生长发育,参与细胞壁的合成和细胞的延伸。
综上所述,植物细胞中的无机盐在维持细胞的渗透压、调节酸碱平衡、参与能量转化、维持电位差平衡以及参与细胞分裂和生长发育等方面起着重要的作用。
无机盐分子的吸收原理
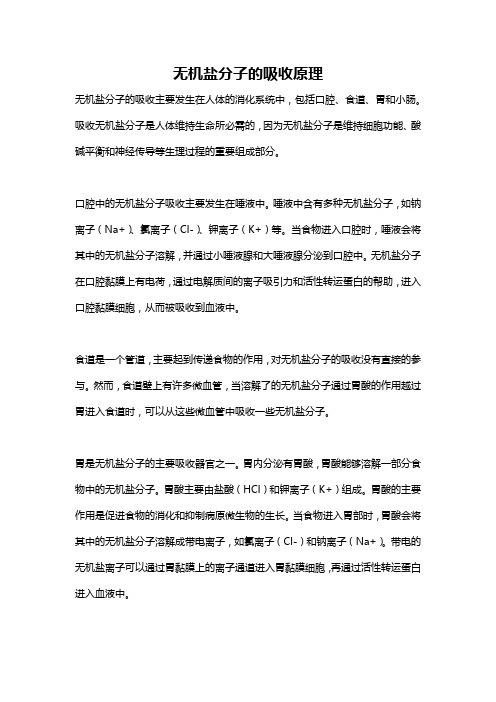
无机盐分子的吸收原理无机盐分子的吸收主要发生在人体的消化系统中,包括口腔、食道、胃和小肠。
吸收无机盐分子是人体维持生命所必需的,因为无机盐分子是维持细胞功能、酸碱平衡和神经传导等生理过程的重要组成部分。
口腔中的无机盐分子吸收主要发生在唾液中。
唾液中含有多种无机盐分子,如钠离子(Na+)、氯离子(Cl-)、钾离子(K+)等。
当食物进入口腔时,唾液会将其中的无机盐分子溶解,并通过小唾液腺和大唾液腺分泌到口腔中。
无机盐分子在口腔黏膜上有电荷,通过电解质间的离子吸引力和活性转运蛋白的帮助,进入口腔黏膜细胞,从而被吸收到血液中。
食道是一个管道,主要起到传递食物的作用,对无机盐分子的吸收没有直接的参与。
然而,食道壁上有许多微血管,当溶解了的无机盐分子通过胃酸的作用越过胃进入食道时,可以从这些微血管中吸收一些无机盐分子。
胃是无机盐分子的主要吸收器官之一。
胃内分泌有胃酸,胃酸能够溶解一部分食物中的无机盐分子。
胃酸主要由盐酸(HCl)和钾离子(K+)组成。
胃酸的主要作用是促进食物的消化和抑制病原微生物的生长。
当食物进入胃部时,胃酸会将其中的无机盐分子溶解成带电离子,如氯离子(Cl-)和钠离子(Na+)。
带电的无机盐离子可以通过胃黏膜上的离子通道进入胃黏膜细胞,再通过活性转运蛋白进入血液中。
小肠是无机盐分子吸收的最主要地方。
小肠内壁有大量的绒毛和肠道上皮细胞,这些细胞上有丰富的微绒毛。
微绒毛上有许多离子通道和活性转运蛋白,帮助无机盐分子的吸收。
消化食物通过胃经过幽门进入小肠时,胃酸会被胰液中的碳酸氢钠中和,使小肠内的pH值升高。
这种碱性环境有利于无机盐分子的吸收。
而且,小肠上皮细胞表面有许多绒毛,提供了巨大的表面积,以便于无机盐分子的吸收。
当食物经过小肠时,无机盐分子可以通过运输蛋白、离子通道或被动扩散等方式进入小肠细胞内,再通过细胞内的运输蛋白进入血液和淋巴系统。
主要涉及的无机盐分子包括钠、钾、钙、镁和氯等。
这些离子通过被动扩散和活性转运蛋白的作用,进入细胞内,并由胞内离子泵将多余的离子排泄出去,以保持体内的离子平衡。
离子通道的生理功能
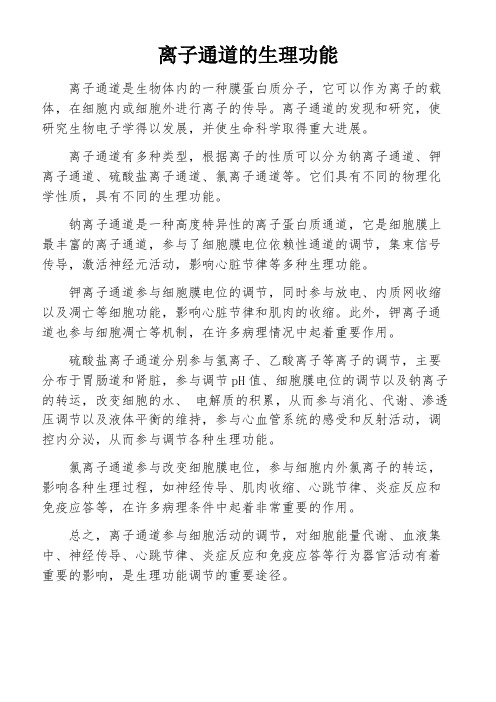
离子通道的生理功能
离子通道是生物体内的一种膜蛋白质分子,它可以作为离子的载体,在细胞内或细胞外进行离子的传导。
离子通道的发现和研究,使研究生物电子学得以发展,并使生命科学取得重大进展。
离子通道有多种类型,根据离子的性质可以分为钠离子通道、钾离子通道、硫酸盐离子通道、氯离子通道等。
它们具有不同的物理化学性质,具有不同的生理功能。
钠离子通道是一种高度特异性的离子蛋白质通道,它是细胞膜上最丰富的离子通道,参与了细胞膜电位依赖性通道的调节,集束信号传导,激活神经元活动,影响心脏节律等多种生理功能。
钾离子通道参与细胞膜电位的调节,同时参与放电、内质网收缩以及凋亡等细胞功能,影响心脏节律和肌肉的收缩。
此外,钾离子通道也参与细胞凋亡等机制,在许多病理情况中起着重要作用。
硫酸盐离子通道分别参与氢离子、乙酸离子等离子的调节,主要分布于胃肠道和肾脏,参与调节pH值、细胞膜电位的调节以及钠离子的转运,改变细胞的水、电解质的积累,从而参与消化、代谢、渗透压调节以及液体平衡的维持,参与心血管系统的感受和反射活动,调控内分泌,从而参与调节各种生理功能。
氯离子通道参与改变细胞膜电位,参与细胞内外氯离子的转运,影响各种生理过程,如神经传导、肌肉收缩、心跳节律、炎症反应和免疫应答等,在许多病理条件中起着非常重要的作用。
总之,离子通道参与细胞活动的调节,对细胞能量代谢、血液集中、神经传导、心跳节律、炎症反应和免疫应答等行为器官活动有着重要的影响,是生理功能调节的重要途径。
长期高盐所致高血压大鼠主动脉eNOS蛋白表达及主动脉血管平滑肌细胞BKCa电流的变化
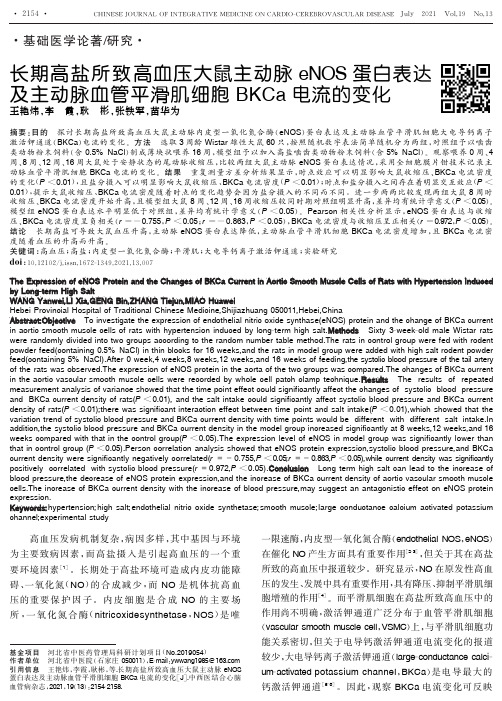
中 西 医 结 合 心 脑 血 管 病 杂 志 2021 年 7 月 第 19 卷 第 13 期
· 2155 ·
血管平滑肌细胞在高盐所致高血压中的作用。本研究 通 过 构 建 动 物 模 型 ,探 讨 长 期高盐所致高血压大 鼠 主 动 脉 eNOS 蛋白表达及主动脉血管平滑肌细胞 BKCa 电流 的变化。 1 材料与方法 1.1 动 物 及 分 组 无 特 定 病 原 体 (specific pathogen free,SPF)级 3 周龄 Wistar 雄性大鼠 60 只,体重 50~ 70 g,由上 海 杰 思 捷 实 验 动 物 有 限 公 司 提 供,许 可 证 号:SCXK(沪)2012-0002。将 60 只大鼠随机分为模型 组和对照组,每 组 30 只,对 照 组 予 以 含 0.5% NaCl 的 啮齿类饲料喂养,模 型 组 予 以 含 5% NaCl 的 啮 齿 类 饲 料喂养。饲养环境:温度 20~25 ℃,相对湿度 40% ~ 70% ,饮用 水 为 自 来 水。 分 别 于 0 周、4 周、8 周、12 周、16 周,采用 动 脉 测 压 仪 测 量 大 鼠 处 于 安 静 状 态 鼠 尾动脉 压,测 量 时 间 为 08:00~ 14:00,每 次 测 量 6 个 数值的平均值为当天收缩压。16 周后模型组收缩压≥ 115 mmHg(1 mmHg=0.133 kPa),且比同时点对照组 收缩压均值≥20 mmHg,则 判 定 为 造 模 成 功。 本 试 验 中,模型组 12 周后收缩压为(138.24±5.33)mmHg,对 照 组 收 缩 压 为 (108.12± 4.18)mmHg,模 型 组 高 于 对 照 组收缩压均值 20 mmHg 以上,确认造模成功。 1.2 主要实验仪器 动物恒温系 统(上 海 奥 尔 科 特 生 物科技有限公司),无创血压测量仪(BP-600A 型,成都 泰盟科技),倒置显微镜系 统(XDS-1,重 庆 光 电 仪 器 有 限 公 司 ),膜 片 钳 放 大 器 (Axo-patch 200B 型 ), pCLAMP 9.0 软件程序和 DigiData 1200B 型数/模转换 器(Axon 公司,美 国),微 电 极 拉 制 仪 (PC-10 型,玉 研 仪器 公 司 ),电 泳 仪 (DYCZ-24KS 型,北 京 六 一 仪 器 厂),凝胶成像系统(美国 Alpha Innotech 公司)。兔抗 鼠微管蛋 白 β-tubulin 抗 体,兔 抗 鼠 eNOS 抗 体、羊 抗 兔 IgG 抗体(辣 根 过 氧 化 物 酶 标 记)、β-tubulin 内 参 均 购自北京中杉金 桥 生 物 技 术 有 限 公 司,磷 酸 缓 冲 盐 溶 液(phosphate buffer saline,PBS)、蛋 白 裂 解 缓 冲 液、 硝酸纤维素膜(PVDF)及 其 余 试 剂 均 购 自 上 海 生 物 工 程技术公司。 1.3 大鼠尾 动 脉 无 创 性 血 压 测 量 开 启 无 创 血 压 测 量仪,开启前预 热 20 min 左 右,压 力 信 号 定 标 后 将 清 醒 大 鼠 装 入 固 定 盒 内 固 定 ,然 后 放 入 固 定 架 ,固 定 完 成 后将加压套插入 大 鼠 尾 根 部,使 鼠 尾 穿 过 脉 搏 传 感 器 插入尾部加热管内,并 刚 好 处 于 “脉 搏 信 号 传 感 片”上 方,调节脉搏 传 感 器,使 传 感 片 紧 贴 鼠 尾 下 方 的 尾 动 脉 ,大 鼠 脉 搏 稳 定 后 ,测 量 血 压 。 1.4 主动脉 eNOS 蛋白表达水平的测定 1.4.1 主动脉 采 集 喂 养 16 周 结 束 后,大 鼠 禁 食 10
食物消化 盐和矿物质的吸收
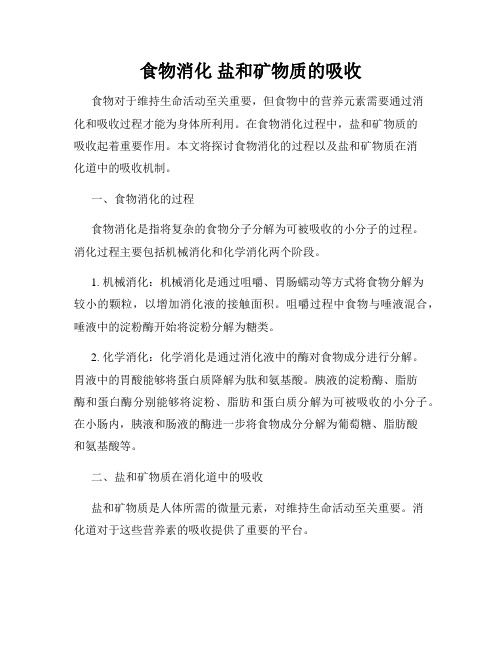
食物消化盐和矿物质的吸收食物对于维持生命活动至关重要,但食物中的营养元素需要通过消化和吸收过程才能为身体所利用。
在食物消化过程中,盐和矿物质的吸收起着重要作用。
本文将探讨食物消化的过程以及盐和矿物质在消化道中的吸收机制。
一、食物消化的过程食物消化是指将复杂的食物分子分解为可被吸收的小分子的过程。
消化过程主要包括机械消化和化学消化两个阶段。
1. 机械消化:机械消化是通过咀嚼、胃肠蠕动等方式将食物分解为较小的颗粒,以增加消化液的接触面积。
咀嚼过程中食物与唾液混合,唾液中的淀粉酶开始将淀粉分解为糖类。
2. 化学消化:化学消化是通过消化液中的酶对食物成分进行分解。
胃液中的胃酸能够将蛋白质降解为肽和氨基酸。
胰液的淀粉酶、脂肪酶和蛋白酶分别能够将淀粉、脂肪和蛋白质分解为可被吸收的小分子。
在小肠内,胰液和肠液的酶进一步将食物成分分解为葡萄糖、脂肪酸和氨基酸等。
二、盐和矿物质在消化道中的吸收盐和矿物质是人体所需的微量元素,对维持生命活动至关重要。
消化道对于这些营养素的吸收提供了重要的平台。
1. 胃内的吸收:一些可溶解于水的矿物质如钠、钾、铁等,可以在胃内通过被胃酸促使其转化为可被吸收的离子形式。
其中,钠离子主要通过胃内和十二指肠摄入,并在小肠内进一步吸收。
其他矿物质如钙、镁等在胃内仅有少量吸收。
2. 小肠内的吸收:大部分盐和矿物质的吸收发生在小肠内。
小肠壁上有大量的微绒毛,微绒毛上分布着许多绒毛膜,形成进一步增加吸收面积的结构。
吸收主要发生在空肠和回肠。
- 钠和钾盐的吸收:钠和钾是人体内含量最高的矿物质之一。
它们通过细胞膜上的离子通道主动进入肠细胞。
钠通过钠-葡萄糖共转运体进入细胞,而钾则通过钾通道进入细胞。
- 钙和镁的吸收:钙和镁的吸收主要发生在空肠。
它们通过细胞膜上的不同通道蛋白进入肠细胞,再通过细胞膜上的钙离子泵和镁离子泵进入血液。
- 铁的吸收:铁是体内血红蛋白和肌红蛋白的重要组成部分,也是许多酶的催化剂。
植物对盐胁迫的分子响应机制
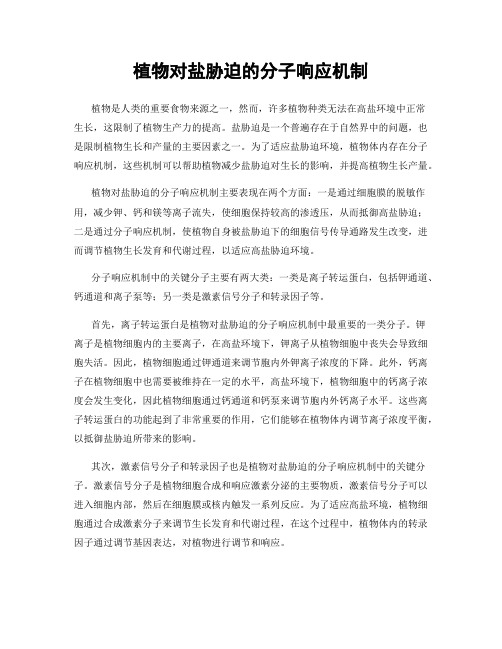
植物对盐胁迫的分子响应机制植物是人类的重要食物来源之一,然而,许多植物种类无法在高盐环境中正常生长,这限制了植物生产力的提高。
盐胁迫是一个普遍存在于自然界中的问题,也是限制植物生长和产量的主要因素之一。
为了适应盐胁迫环境,植物体内存在分子响应机制,这些机制可以帮助植物减少盐胁迫对生长的影响,并提高植物生长产量。
植物对盐胁迫的分子响应机制主要表现在两个方面:一是通过细胞膜的脱敏作用,减少钾、钙和镁等离子流失,使细胞保持较高的渗透压,从而抵御高盐胁迫;二是通过分子响应机制,使植物自身被盐胁迫下的细胞信号传导通路发生改变,进而调节植物生长发育和代谢过程,以适应高盐胁迫环境。
分子响应机制中的关键分子主要有两大类:一类是离子转运蛋白,包括钾通道、钙通道和离子泵等;另一类是激素信号分子和转录因子等。
首先,离子转运蛋白是植物对盐胁迫的分子响应机制中最重要的一类分子。
钾离子是植物细胞内的主要离子,在高盐环境下,钾离子从植物细胞中丧失会导致细胞失活。
因此,植物细胞通过钾通道来调节胞内外钾离子浓度的下降。
此外,钙离子在植物细胞中也需要被维持在一定的水平,高盐环境下,植物细胞中的钙离子浓度会发生变化,因此植物细胞通过钙通道和钙泵来调节胞内外钙离子水平。
这些离子转运蛋白的功能起到了非常重要的作用,它们能够在植物体内调节离子浓度平衡,以抵御盐胁迫所带来的影响。
其次,激素信号分子和转录因子也是植物对盐胁迫的分子响应机制中的关键分子。
激素信号分子是植物细胞合成和响应激素分泌的主要物质,激素信号分子可以进入细胞内部,然后在细胞膜或核内触发一系列反应。
为了适应高盐环境,植物细胞通过合成激素分子来调节生长发育和代谢过程,在这个过程中,植物体内的转录因子通过调节基因表达,对植物进行调节和响应。
上述两类关键分子对植物对盐胁迫的分子响应机制发挥着非常重要的作用。
植物通过调节离子转运蛋白和激素信号分子的表达和调节,来控制植物体内的钾、钙离子和激素等物质的水平,进而适应高盐环境的生长和代谢。
钾离子是一种对于植物生长和发育必需的元素
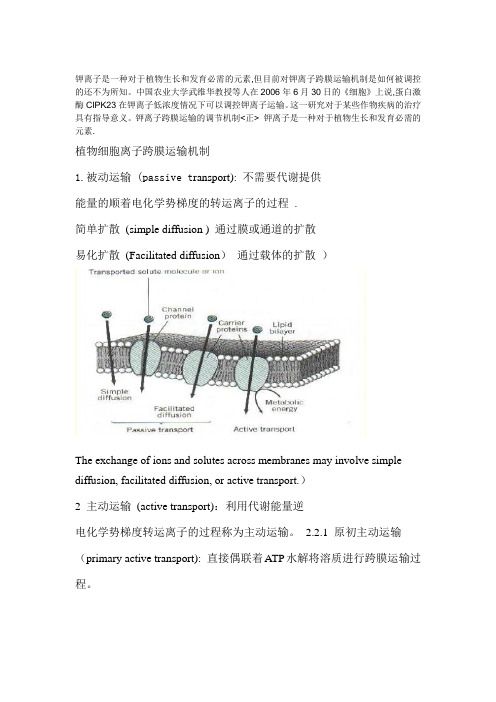
钾离子是一种对于植物生长和发育必需的元素,但目前对钾离子跨膜运输机制是如何被调控的还不为所知。
中国农业大学武维华教授等人在2006年6月30日的《细胞》上说,蛋白激酶CIPK23在钾离子低浓度情况下可以调控钾离子运输。
这一研究对于某些作物疾病的治疗具有指导意义。
钾离子跨膜运输的调节机制<正> 钾离子是一种对于植物生长和发育必需的元素.植物细胞离子跨膜运输机制1.被动运输 (passive t ransport): 不需要代谢提供能量的顺着电化学势梯度的转运离子的过程.简单扩散(simple diffusion ) 通过膜或通道的扩散易化扩散(Facilitated diffusion)通过载体的扩散)The exchange of ions and solutes across membranes may involve simple diffusion, facilitated diffusion, or active transport.)2 主动运输(active transport):利用代谢能量逆电化学势梯度转运离子的过程称为主动运输。
2.2.1 原初主动运输(primary active transport): 直接偶联着ATP水解将溶质进行跨膜运输过程。
Hypothetical steps in the transportof a cation against its chemicalgradient by a electrogenic pump.质膜+-ATPase (P-ATPase): 它是质膜上的一种插入蛋白,其水解ATP 部分在质膜细胞质一侧质膜H+-ATPase建立的跨膜的+ 部分在质膜细胞质一侧, 质膜建立的跨膜的H 部分在质膜细胞质一侧建立的跨膜的浓度梯度和电势梯度,为其它离子或分子的跨膜运输提供动力。
浓度梯度和电势梯度,为其它离子或分子的跨膜运输提供动力。
生物体中无机盐的重要作用与补充
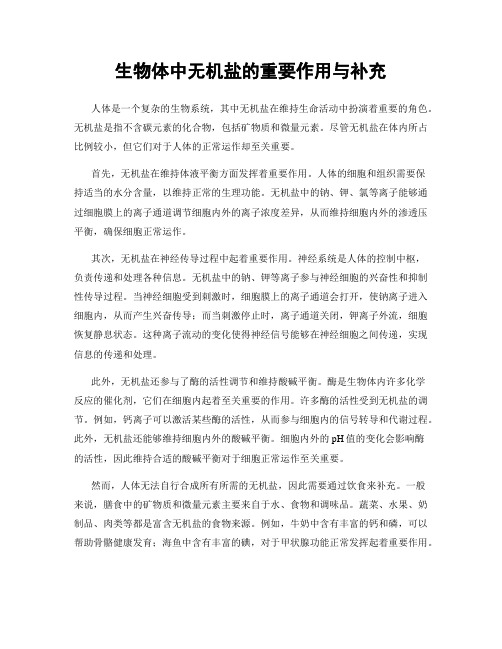
生物体中无机盐的重要作用与补充人体是一个复杂的生物系统,其中无机盐在维持生命活动中扮演着重要的角色。
无机盐是指不含碳元素的化合物,包括矿物质和微量元素。
尽管无机盐在体内所占比例较小,但它们对于人体的正常运作却至关重要。
首先,无机盐在维持体液平衡方面发挥着重要作用。
人体的细胞和组织需要保持适当的水分含量,以维持正常的生理功能。
无机盐中的钠、钾、氯等离子能够通过细胞膜上的离子通道调节细胞内外的离子浓度差异,从而维持细胞内外的渗透压平衡,确保细胞正常运作。
其次,无机盐在神经传导过程中起着重要作用。
神经系统是人体的控制中枢,负责传递和处理各种信息。
无机盐中的钠、钾等离子参与神经细胞的兴奋性和抑制性传导过程。
当神经细胞受到刺激时,细胞膜上的离子通道会打开,使钠离子进入细胞内,从而产生兴奋传导;而当刺激停止时,离子通道关闭,钾离子外流,细胞恢复静息状态。
这种离子流动的变化使得神经信号能够在神经细胞之间传递,实现信息的传递和处理。
此外,无机盐还参与了酶的活性调节和维持酸碱平衡。
酶是生物体内许多化学反应的催化剂,它们在细胞内起着至关重要的作用。
许多酶的活性受到无机盐的调节。
例如,钙离子可以激活某些酶的活性,从而参与细胞内的信号转导和代谢过程。
此外,无机盐还能够维持细胞内外的酸碱平衡。
细胞内外的pH值的变化会影响酶的活性,因此维持合适的酸碱平衡对于细胞正常运作至关重要。
然而,人体无法自行合成所有所需的无机盐,因此需要通过饮食来补充。
一般来说,膳食中的矿物质和微量元素主要来自于水、食物和调味品。
蔬菜、水果、奶制品、肉类等都是富含无机盐的食物来源。
例如,牛奶中含有丰富的钙和磷,可以帮助骨骼健康发育;海鱼中含有丰富的碘,对于甲状腺功能正常发挥起着重要作用。
然而,需要注意的是,无机盐的过量摄入也会对人体健康造成负面影响。
例如,过量的钠摄入会导致血压升高,增加心血管疾病的风险;过量的铅摄入会对神经系统和肾脏造成损害。
因此,在补充无机盐时,应该根据个人的需求和特殊情况进行合理搭配,避免过量摄入。
盐为什么咸
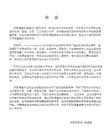
味觉有甜、酸、苦、咸和鲜味五种,已经发现咸味和酸味的受体是离子通道,其他的味觉受体被认为是G蛋白偶联受体GPCRs(G-protein-coupled receptors)。有味物质刺激口腔内的味觉感受体,通过一个收集和传递信息的神经感觉系统传导到大脑的味觉中枢,最后通过大脑的综合神经中枢系统的分析,从而产生味觉。这些味觉受体主要分布在舌背和舌缘的和口咽部粘膜味蕾细胞上。NaCl在水中离子化后主要是Na+刺激感觉咸味的钠离子通道受体产生咸觉。
钠离子和钾离子通过在溶液中的渗透作者用产生的。
决定食盐味道的离子是Na+ ,其理由是其他相应的含有Na+的盐的味道,如NaNO2也是咸的,所以它易和食盐相混淆,而Cl-是无味道的,对应的Mg2+呈苦味,所以在食盐中,若有其他盐类,其味道就受到一定影响,如海盐和井盐,若含MgCl2多的就有苦味,当然,不同阴、阳离子的组合,对物质的味道特征有影响,最典型的就是食盐,既Na+和Cl-的组合,其咸味特征最为人接受,这似乎和人类的起源于海洋有关。另外,钾离子也可以产生,但稍苦涩.常见的亚硝酸钠也是典型的咸味物质,硫酸钠等等也是咸的.至于烧碱由于氢氧根可以溶解角质蛋白,可以产生特殊的苦涩味,掩盖了钠离子的咸味. 还有碳酸钠水解呈碱性,原理同氢氧化钠.
水稻抗旱与耐盐的分子机理研究
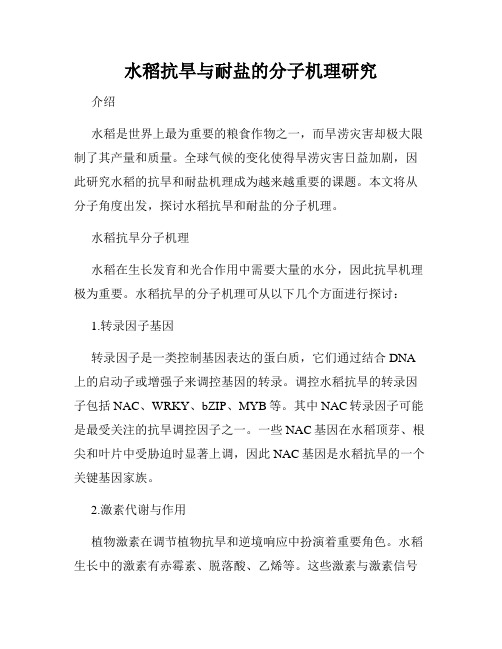
水稻抗旱与耐盐的分子机理研究介绍水稻是世界上最为重要的粮食作物之一,而旱涝灾害却极大限制了其产量和质量。
全球气候的变化使得旱涝灾害日益加剧,因此研究水稻的抗旱和耐盐机理成为越来越重要的课题。
本文将从分子角度出发,探讨水稻抗旱和耐盐的分子机理。
水稻抗旱分子机理水稻在生长发育和光合作用中需要大量的水分,因此抗旱机理极为重要。
水稻抗旱的分子机理可从以下几个方面进行探讨:1.转录因子基因转录因子是一类控制基因表达的蛋白质,它们通过结合DNA 上的启动子或增强子来调控基因的转录。
调控水稻抗旱的转录因子包括NAC、WRKY、bZIP、MYB等。
其中NAC转录因子可能是最受关注的抗旱调控因子之一。
一些NAC基因在水稻顶芽、根尖和叶片中受胁迫时显著上调,因此NAC基因是水稻抗旱的一个关键基因家族。
2.激素代谢与作用植物激素在调节植物抗旱和逆境响应中扮演着重要角色。
水稻生长中的激素有赤霉素、脱落酸、乙烯等。
这些激素与激素信号通路调控了水稻根系生长、叶片水分利用和开花生长等过程。
研究发现,在水稻响应缺水和盐胁迫中,激素如ABA(脱落酸)、GA(赤霉素)和IAA(生长素)会产生变化,它们的量变化可以在一定程度上调控植物休眠、保护细胞膜、调节水分平衡、增加根系活力、保持光合作用强度。
3.抗氧化系统氧化应激指的是由于有害自由基产生增加或抗氧化物光合作用不足,引起氧化状态不平衡的一种生理现象。
植物第一道抗氧化防线是超氧化物歧化酶、过氧化物酶等酶类。
其次,植物还可以合成类黄酮醇、雌激素和谷胱甘肽来降低氧化应激。
水稻在受到干旱、盐胁迫时,抗氧化酶系统被激活,抗氧化物质如抗坏血酸、谷胱甘肽累积增加,抑制自由基的生成和损伤细胞的生境。
水稻耐盐分子机理水稻生长所需要的离子和营养元素会通过根系吸收,同时较高的盐浓度会导致吸收速率降低甚至离子有害晶体沉积在植株部分器官,影响正常代谢。
如何避免水稻受到盐胁迫的影响,其耐盐分子机理也是一个热门话题。
最新钠离子钾离子通道阻滞剂

胺碘酮的发现
1960s,临床上用于治疗心绞痛
发现它对钾通道有阻滞作用 对钠、钙通道有一定阻滞作用 对α、β受体有非竟争性阻滞作用
1970s,作为抗心律失常药正式用于临床
具有广谱抗心律失常作用 可用于其他药物治疗无效的严重心律失常
吸收与代谢特点
本品口服吸收慢,一周左右才起效 半衰期长达9.33~44天 分布广泛,可蓄积在多种器官和组织内 主要代谢物为氮上去乙基产物,该代谢物亦具有
美西律的合成路线
同类药物
利多卡因 妥卡胺 钠通道阻滞剂(Ib)
--治疗各种室性心律失常 局部麻醉药
--作用机制相似、作用部位不同
苯妥英
洋地黄中毒而致心律失常的首选药物 --抑制 出现的触发活动 --改善 伴发的传导阻滞
抗癫痫药物
1.3 Ic类钠通道阻滞剂
普罗帕酮
氟卡尼
均能有效地抑制心肌的自律性、传导性,延 长有效不应期,在消除折返传导和冲动形成 异常方面均有作用,亦属广谱抗心律失常药。
NO在体内的合成及作用
一氧化氮合酶存在于血管内皮细胞中 在一定条件下,如乙酰胆碱作用下,将L-
精氨酸分解产生NO和L-瓜氨酸。 NO也称为内皮舒张因子(EDRF) 有效扩张血管(静脉血管)降低血压
NO供体药作用机制
鸟苷酸环化酶
NO
NO供体
GTP
cGMP 激活
cGMP蛋白激酶
肌凝蛋白
去磷酸轻链肌凝蛋白
钠离子钾离子通道阻滞剂
一、钠通道阻滞剂
钠通道:选择性允许钠离子跨膜通过的离子通道, 在维持细胞兴奋性及正常生理功能上十分重要。
钠通道阻滞剂:是一类能够抑制钠离子内流,从 而抑制心肌细胞动作电位振幅及超射幅度,减慢传 导,延长有效不应期的药物,因而具有良好的抗心 律失常作用。
维持机体渗透压的无机盐
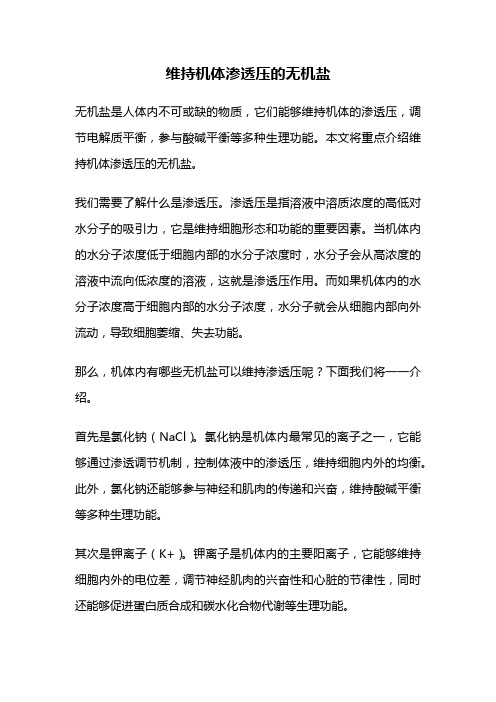
维持机体渗透压的无机盐无机盐是人体内不可或缺的物质,它们能够维持机体的渗透压,调节电解质平衡,参与酸碱平衡等多种生理功能。
本文将重点介绍维持机体渗透压的无机盐。
我们需要了解什么是渗透压。
渗透压是指溶液中溶质浓度的高低对水分子的吸引力,它是维持细胞形态和功能的重要因素。
当机体内的水分子浓度低于细胞内部的水分子浓度时,水分子会从高浓度的溶液中流向低浓度的溶液,这就是渗透压作用。
而如果机体内的水分子浓度高于细胞内部的水分子浓度,水分子就会从细胞内部向外流动,导致细胞萎缩、失去功能。
那么,机体内有哪些无机盐可以维持渗透压呢?下面我们将一一介绍。
首先是氯化钠(NaCl)。
氯化钠是机体内最常见的离子之一,它能够通过渗透调节机制,控制体液中的渗透压,维持细胞内外的均衡。
此外,氯化钠还能够参与神经和肌肉的传递和兴奋,维持酸碱平衡等多种生理功能。
其次是钾离子(K+)。
钾离子是机体内的主要阳离子,它能够维持细胞内外的电位差,调节神经肌肉的兴奋性和心脏的节律性,同时还能够促进蛋白质合成和碳水化合物代谢等生理功能。
还有钙离子(Ca2+)。
钙离子在机体内广泛存在,它能够影响心肌收缩和松弛、骨骼形态的维持和生长等重要生理过程。
此外,钙离子还能够影响细胞内的酶活性、离子通道的打开和关闭等多种生理功能。
还有氢离子(H+)。
氢离子是影响人体酸碱平衡的重要因素,它能够影响细胞内外的pH值,进而影响细胞内外的代谢和功能。
机体内还存在着多种无机盐,如镁离子、磷酸盐、铁离子等,它们都在不同程度上参与了维持机体的渗透压、电解质平衡和酸碱平衡等生理功能。
无机盐在人体内发挥着重要的生理作用,它们能够维持机体的渗透压、调节电解质平衡和酸碱平衡等多种生理功能。
因此,我们应该注重摄入各种无机盐,保证机体内的正常代谢和功能。
植物钾离子通道的分子生物学研究进展
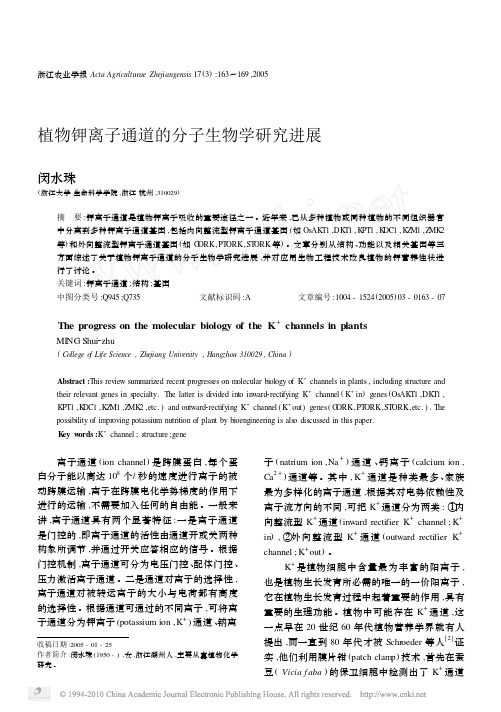
浙江农业学报Acta Agriculturae Zhejiangensis17(3):163~169,2005植物钾离子通道的分子生物学研究进展闵水珠(浙江大学生命科学学院,浙江杭州,310029)摘 要:钾离子通道是植物钾离子吸收的重要途径之一。
近年来,已从多种植物或同种植物的不同组织器官中分离到多种钾离子通道基因,包括内向整流型钾离子通道基因(如OsAK T1,DK T1,K PT1,K DC1,K Z M1,Z MK2等)和外向整流型钾离子通道基因(如G ORK,PT ORK,ST ORK等)。
文章分别从结构、功能以及相关基因等三方面综述了关于植物钾离子通道的分子生物学研究进展,并对应用生物工程技术改良植物的钾营养性状进行了讨论。
关键词:钾离子通道;结构;基因中图分类号:Q945;Q735 文献标识码:A文章编号:1004-1524(2005)03-0163-07The progress on the molecular biology of the K+channels in plantsMI NG Shui2zhu(College o f Life Science,Zhejiang Univer sity,Hangzhou310029,China)Abstract:This review summarized recent progresses on m olecular biology of K+channels in plants,including structure and their relevant genes in specialty.The latter is divided into inward2rectifying K+channel(K+in)genes(OsAK T1,DK T1, K PT1,K DC1,K Z M1,Z MK2,etc.)and outward2rectifying K+channel(K+out)genes(G ORK,PT ORK,ST ORK,etc.).The possibility of im proving potassium nutrition of plant by bioengineering is als o discussed in this paper.K ey w ords:K+channel;structure;gene 离子通道(ion channel)是跨膜蛋白,每个蛋白分子能以高达108个/秒的速度进行离子的被动跨膜运输,离子在跨膜电化学势梯度的作用下进行的运输,不需要加入任何的自由能。
ENaC通道,激素及高血压之间的关系
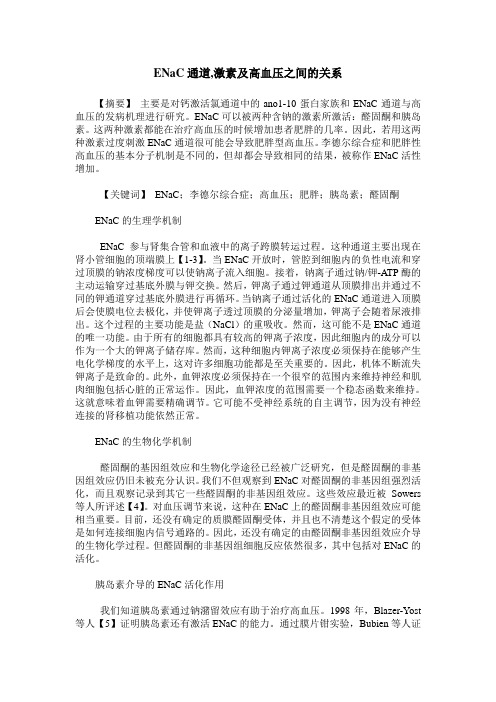
ENaC通道,激素及高血压之间的关系【摘要】主要是对钙激活氯通道中的ano1-10蛋白家族和ENaC通道与高血压的发病机理进行研究。
ENaC可以被两种含钠的激素所激活:醛固酮和胰岛素。
这两种激素都能在治疗高血压的时候增加患者肥胖的几率。
因此,若用这两种激素过度刺激ENaC通道很可能会导致肥胖型高血压。
李德尔综合症和肥胖性高血压的基本分子机制是不同的,但却都会导致相同的结果,被称作ENaC活性增加。
【关键词】ENaC;李德尔综合症;高血压;肥胖;胰岛素;醛固酮ENaC的生理学机制ENaC参与肾集合管和血液中的离子跨膜转运过程。
这种通道主要出现在肾小管细胞的顶端膜上【1-3】。
当ENaC开放时,管腔到细胞内的负性电流和穿过顶膜的钠浓度梯度可以使钠离子流入细胞。
接着,钠离子通过钠/钾-ATP酶的主动运输穿过基底外膜与钾交换。
然后,钾离子通过钾通道从顶膜排出并通过不同的钾通道穿过基底外膜进行再循环。
当钠离子通过活化的ENaC通道进入顶膜后会使膜电位去极化,并使钾离子透过顶膜的分泌量增加,钾离子会随着尿液排出。
这个过程的主要功能是盐(NaCl)的重吸收。
然而,这可能不是ENaC通道的唯一功能。
由于所有的细胞都具有较高的钾离子浓度,因此细胞内的成分可以作为一个大的钾离子储存库。
然而,这种细胞内钾离子浓度必须保持在能够产生电化学梯度的水平上,这对许多细胞功能都是至关重要的。
因此,机体不断流失钾离子是致命的。
此外,血钾浓度必须保持在一个很窄的范围内来维持神经和肌肉细胞包括心脏的正常运作。
因此,血钾浓度的范围需要一个稳态函数来维持。
这就意味着血钾需要精确调节。
它可能不受神经系统的自主调节,因为没有神经连接的肾移植功能依然正常。
ENaC的生物化学机制醛固酮的基因组效应和生物化学途径已经被广泛研究,但是醛固酮的非基因组效应仍旧未被充分认识。
我们不但观察到ENaC对醛固酮的非基因组强烈活化,而且观察记录到其它一些醛固酮的非基因组效应。
动作电位的生理名词解释

动作电位的生理名词解释动作电位是细胞膜发生的一种快速、短暂的电活动,是神经、肌肉和其他可兴奋组织的基本电活动单位。
它是一种电流产生和传导的过程,使得神经元能够传递信息、控制肌肉运动和产生感觉。
本文将深入探讨动作电位的产生、传导和相关的生理过程。
一、动作电位的产生动作电位的产生与神经元的细胞膜上存在的离子通道和离子平衡有关。
在静息状态下,神经元的细胞膜内外存在着电位差,称为静息膜电位。
细胞内带负电荷的大量蛋白质和细胞外的离子导致了负向的静息膜电位。
当神经元受到足够强度的刺激时,细胞膜上的特定离子通道会打开,导致离子的流动。
其中最重要的是钠离子通道和钾离子通道。
刺激引起钠离子通道打开,钠离子由细胞外流入细胞内,使得细胞内变得正电荷更多,进而产生电位上升,称为去极化。
这个阶段被称为上升阶段。
随着时间的推移,钠离子通道关闭,钾离子通道开放。
钾离子从细胞内流出,使细胞内回到负电荷状态,称为复极化。
这个阶段被称为下降阶段。
二、动作电位的传导动作电位在神经元内的传导方式分为两种:盐atory conduction(盐性传导)和continuous conduction(连续传导)。
盐atory conduction指的是动作电位以跳跃的方式在轴突上传导,即只在轴突上产生和传导动作电位,而在轴突之间的距离则通过离子的扩散来传递信号。
这种传导方式的特点是速度较快,传导效率高。
非髓鞘轴突纤维和非髓鞘纤维属于这种类型。
连续传导指的是动作电位连续地在轴突上产生和传导,细胞膜沿整个轴突上都发生了去极化和复极化。
这种传导方式主要出现在髓鞘轴突纤维中,髓鞘是一种由格兰氏细胞产生的脂质层,能够增加电信号的传导速度。
三、动作电位与反应潜伏期动作电位的产生和传导过程需要一定的时间,这个时间称为反应潜伏期。
反应潜伏期是指从刺激开始到产生反应之间的时间间隔。
它与感受器的刺激强度、神经元的兴奋状态和传导距离等因素有关。
在感官系统中,动作电位可以帮助我们对刺激作出快速的反应。
- 1、下载文档前请自行甄别文档内容的完整性,平台不提供额外的编辑、内容补充、找答案等附加服务。
- 2、"仅部分预览"的文档,不可在线预览部分如存在完整性等问题,可反馈申请退款(可完整预览的文档不适用该条件!)。
- 3、如文档侵犯您的权益,请联系客服反馈,我们会尽快为您处理(人工客服工作时间:9:00-18:30)。
Potassium and Potassium-Permeable Channels in Plant Salt ToleranceSergey Shabala and Igor I.PottosinAbstract Salinity causes billion dollar losses in crop production around the globe and has also a significant social impact on rural communities.To breed salt tolerant crops,a better understanding of mechanisms mediating plant adaptive responses to salinity is needed.Over the last years,evidence has been accumulated suggesting that plants’ability to maintain a high cytosolic K+/Na+ratio appears to be critical to plant salt tolerance.This paper reviews molecular and ionic mechanisms contributing to potassium homeostasis in salinized plant tissues and discuss prospects for breeding for salt tolerance by targeting this trait.We show that K+channels are instrumental to nearly all aspects of salinity stress signaling and tolerance,and the plant’s ability to control intracellular K+homeostasis is arguably the most important feature of salt-tolerant species.The molecular identity of key genes,mediating plant adaptive responses to salinity,is analyzed,and the modes of their control are discussed.It is suggested that the major focus of plant physiologists and breeders should be on revealing the specificity of K+channel regulation under saline conditions and a“fine tuning”of all mechanisms involved in the regulation of K+homeostasis in plants, including both plasma-and endomembrane channels and transporters. AbbreviationsCAX Cation/H+antiporterCBL Calcineurin B-like proteinsCHX Chloroplast envelope K+/H+exchangerS.Shabala(*)School of Agricultural Science,University of Tasmania,Australiae-mail:Sergey.Shabala@.auI.I.PottosinCentro Universitario de Investigaciones Biome´dicas(CUIB),Universidad de Colima,Colima, Me´xicoe-mail:pottosin@ucol.mxV.Demidchik and F.Maathuis(eds.),Ion Channels and Plant Stress Responses,87 Signaling and Communication in Plants,DOI10.1007/978-3-642-10494-7_5,#Springer-Verlag Berlin Heidelberg201088S.Shabala and I.I.Pottosin CNGC Cyclic nucleotide-gated channelCIPK CBL-interacting protein kinasesFACC Fast activating cation channelFV Fast vacuolar channelGLR Glutamate receptorKIR Inward-rectifying K+channelKOR Outward-rectifying K+channelKUP/HAK/KT H+/K+symporterNHX Vacuolar Na+/H+exchangerNSCC Non-selective cation channelPCD Programmed cell deathPM Plasma membraneROS Reactive oxygen speciesSV Slow vacuolar channelTEA Tetraethylammonium chlorideTPK/KCO Two-pore K+channelTrk/HKT Na+/K+symporter1Introduction1.1Salinity as an IssueGlobal food production will need to increase by approximately50%by2050to match the projected population growth(Flowers2004;Rengasamy2006).As most suitable land has already been cultivated,this implies a need for expansion into new areas to meet the above target.Most of these areas are either severely affected by salinity,or require extensive irrigation and,hence,are at risk to become saline (Flowers2004).Over800million hectares of land worldwide are affected by salinity(Munns2005),comprising nearly7%of the world’s total land area.Irriga-tion systems are particularly prone to salinization,with nearly one-third of irrigated land being severely affected(Munns2002).The economic penalties are in the billion dollar range.Salt tolerant crops,or plant species able to remove excessive salt from the soil while lowering the water table,may contribute significantly to managing this problem.The key to engineering plants for salt tolerance lies in a better understanding of the key physiological mechanisms underlying the adaptive responses of plants to salinity.Numerous strategies are used by different species to deal with excessive NaCl content in the soil.Most of them are attributed to better regulation of Na+uptake and compartmentation(e.g.,Na+exclusion from uptake and sequestration in vacuoles,regulation of Na+transport to the shoot at the xylem/ root parenchyma boundary,retranslocation in phloem,or compartmentation within the shoot;reviewed by Tester and Davenport2003).In addition,plant’s ability to retain K+in its tissues under saline conditions appears to be central to salinity tolerance(Shabala and Cuin2008).This chapter addresses the latter mechanism.Potassium and Potassium-Permeable Channels in Plant Salt Tolerance89 1.2Physiological Constraints Imposed by SalinityTraditionally,growth inhibition and poor plant performance under saline conditions are attributed to osmotic stress imposed by salinity and to specific ion(Na+in most cases)toxicity.As will be shown below,detrimental effects of each of these factors are crucially dependent on a plant’s ability to maintain K+homeostasis and control K+transport across cellular membranes.To deal with osmotic stress problem and maintain sufficient turgor pressure required to drive extension growth in roots and shoots,plants must increase their cell sap osmolality in a process called osmotic adjustment.The traditional view is that plants are doing this by increased de novo synthesis of a variety of organic osmolytes(so-called“compatible solutes”)(Bohnert and Jensen1996;Sakamoto and Murata2000).However,being an energetically expensive process(Raven 1985),such de novo synthesis draws on a substantial portion of the ATP pool, thus making it less available for other metabolic functions and imposing growth penalties.Alternatively,plants can also achieve osmotic adjustment by increased accumulation of inorganic ions(mainly,Na+,ClÀ,and K+)in the cell.However, being accumulated in high quantities,both Na+and ClÀare toxic to cell metabo-lism.Such specific ion(particularly Na+)toxicity is often considered as a primary reason for detrimental effects of salinity(Tester and Davenport2003).In this review,we show that an optimal Na+management by plants crucially depends on K+transport and Na+compartmentation.Recently,a third component,an ROS-induced damage to key macromolecules and proteins,has been added to the list of detrimental effects of salinity(Zhu2003; Tester and Davenport2003).Moreover,several papers have shown that salinity-induced increases in ROS production may often lead to programmed cell death (PCD),and that the cytosolic K+“status”is critical in triggering salinity-induced PCD(reviewed in Shabala2009).This issue is also discussed briefly in this chapter(see Sect.1.2in Chapter“Cation Channels and the Uptake of Radiocaesium by Plants”).2Potassium Homeostasis in Plants2.1Potassium Essentiality and Functions in PlantsBeing the second(after nitrogen)most abundant mineral nutrient in plants(Marschner 1995),potassium is crucial to cell metabolism.The typical potassium concentration in shoot tissue varies between4and8%of the plant’s dry weight.Importantly,the concentration of free potassium is highest among all essential mineral nutrients, which determines its crucial role in cellular osmo-and turgor regulation.In addition to this,K+activates over50key metabolic enzymes,including those involved in photosynthesis,oxidative metabolism,and protein synthesis(Marschner1995).90S.Shabala and I.I.Pottosin Potassium is also widely used as a charge-balancing ion and is essential for all types of plant movements,including stomatal opening.In addition,K+neutralizes the soluble(e.g.,organic acid anions and inorganic anions)and insoluble macromolec-ular anions and stabilizes cytosolic pH at the level optimal for most enzymatic reactions(pH~7.2)(Cuin and Shabala2006).Thus,cytosolic K+homeostasis is crucial to optimal cell metabolism.To a large extent,detrimental effects of salinity can be explained by the existing competition between Na+and K+,as Na+substitutes K+at major binding sites in key metabolic processes in the cytoplasm(Marschner 1995).It is becoming increasingly evident that it is not the absolute quantity of Na+ per se,but rather the cytosolic K+/Na+ratio that determines cell metabolic compe-tence and ultimately,the ability of a plant to survive in saline environments(Gorham et al.1991;Gaxiola et al.1992;Maathuis and Amtmann1999;Cuin et al.2003; Colmer et al.2006;Shabala and Cuin2008),and the difference in salt sensitivity between some species was attributed to enhanced K+/Na+discrimination(Gorham et al.1991;Dvorˇa´k et al.1994;Dubcovsky et al.1996;Volkov et al.2004).2.2Tissue-and Organelle-Specific Potassium Compartmentation The two major pools of potassium in plant cells are in the vacuole and in the cytosol.Cytosolic K+concentrations are maintained at a constant level of~100mM and do not differ between root and leaf cells(Walker et al.1996;Cuin et al.2003). Such strict cytosolic K+homeostasis is explained by the fact that both activation and protein biosynthesis rely on high and stable K+concentrations within the cytoplasm(Maathuis and Sanders1994;Leigh et al.1999).On the contrary, vacuolar K+content may vary dramatically between different cell types,ranging from~120mM in root cell vacuoles(Walker et al.1996)to~230mM in mesophyll cell vacuoles(Cuin et al.2003).Under K+deficiency conditions,cytosolic K+is maintained at a constant level at the expense of vacuolar potassium(Walker et al. 1996)where it can drop essentially to zero.However,given the important role of vacuolar K+in maintaining cell turgor,other cations(e.g.,Na+,Mg2+,or Ca2+)or organic solutes(e.g.,sugars)must substitute for the osmotic functions of potassium in vacuoles.A failure to do this causes an immediate arrest of plant growth.Salinity stress results in significant membrane depolarization,favoring passive K+efflux from the cytosol.As a result,a rapid decline in cytosolic K+is measured (Shabala et al.2006).This decline,however,is only transient,as shown by direct electrophysiological measurements using impaled K+selective microelectrodes (Cuin et al.2003;Shabala et al.2006).The rapid recovery of cytosolic K+in these acute NaCl stress experiments may be interpreted as evidence for the restoration of the cytosolic K+pool at the expense of the vacuole(see Sect.2in in Chapter“Cation Channels and the Uptake of Radiocaesium by Plants”).It is obvious that such a process can have only a limited time span and,unless further K+leakage across the plasma membrane is prevented,the vacuolar K+pool will eventually become depleted.This is the case for prolonged salinity treatment(Cuin et al.2003).Potassium and Potassium-Permeable Channels in Plant Salt Tolerance91 K+also plays an important role in charge balance in thylakoid membranes (Junge and Jackson1982),as well as in enzymatic control of leaf photochemistry in stroma(Demmig and Gimmler1983;Pier and Berkowitz1987).The impact of salinity of K+transport and homeostasis in chloroplasts is discussed in Sect.3in Chapter“Cation Channels and the Uptake of Radiocaesium by Plants”.2.3Major Potassium Transport Systems:A Brief OverviewOver millions of years,plants have evolved a sophisticated network of potassium transport systems.In Arabidopsis,seven major families of cation transporters are known,comprising75genes in total.These include(Ma¨ser et al.2001;Ve´ry and Sentenac2002,2003;Shabala2003)the following:–Shaker-type family of K+channels(9genes in total);–“Two-pore”potassium channels(TPK;5genes in total);–KUP/HAK/KT transporters(H+/K+symporter,13genes in total);–Trk/HKT transporters(Na+/K+symporter;one gene);–K+/H+antiporter homologs(NHX/CHX;around26genes);–Cyclic-nucleotide-gated channels(CNGC;20genes in total);–Glutamate receptors(GLRs;20genes in total).It is also important to note that not only are these transporters specifically expressed within various cell compartments and tissues,but also their expression patterns are strongly affected by environmental conditions and,specifically,by salinity.Thus,K+ transport activity may be adjusted in various cells,independently in each organ/tissue, to match the plant’s demands in a challenging environment.As a result,cytosolic K+ homeostasis can be maintained to enable optimal plant function.2.4Potassium and Potassium-Permeable ChannelsShaker-type potassium channels include nine members in Arabidopsis and are further subdivided into three major functional groups,based on their voltage dependency(Ve´ry and Sentenac2002,2003):(a)Inward-rectifying channels(AKT1,KAT1,KAT2and SPIK)mediating potas-sium uptake and activated by membrane hyperpolarization;(b)Weakly-inward-rectifying channels(AKT2/3)which mediate both potassiumuptake and release depending on the local potassium electrochemical gradients;(c)Outward-rectifying channels(SKOR and GORK)mediating potassium releasefrom the cell and activated by membrane depolarization.Shaker channels are ubiquitously expressed in various plant tissues,providing a possibility for the rapid redistribution of K+between various plant parts and cellular compartments.92S.Shabala and I.I.Pottosin “Two-pore”TPK/KCO potassium channels havefive members in Arabidopsis (Czempinski et al.2002).Only one of them,AtTPK4,is targeted to the plasma membrane(in pollen tubes)and forms a functional K+channel there.All others encode tonoplast proteins(Voelker et al.2006);see Sect.1in Chapter“Cation Channels and the Uptake of Radiocaesium by Plants”for further details.TPKs play roles in pollen tube growth,stomatal closure and radical development(Becker et al. 2004;Gobert et al.2007).Non-selective cation channels(NSCCs)form a large(40putative members in Arabidopsis)heterogeneous group of channels.As the name suggests,NSCC typically show a high selectivity for cations over anions but differ widely in their ability to conduct mono-and divalent cations(Demidchik et al.2002;Demidchik and Maathuis2007).These channels are ubiquitous at the plasma and tonoplast membranes of plant cells and vary greatly in their voltage dependence and perme-ability ratios.Accordingly,they are further classified as depolarization activated, hyperpolarization activated,voltage insensitive,calcium activated,mechanosensi-tive,cyclic nucleotide-gated,and glutamate-gated(Demidchik et al.2002).So far, no proteins responsible for non-selective cation currents have been identified at the molecular level.Likely candidates for NSCC forming proteins belong to two families,namely CNGCs and GluRs.Cyclic nucleotide-gated channels(CNGCs)are ligand-gated channels that are regulated by cAMP or cGMP(Leng et al.2002).Twenty and sixteen CNGC family members are identified in Arabidopsis(Ko¨hler et al.1999;Ma¨ser et al.2001)and rice(Yuan et al.2003),respectively.In contrast to animal CNGCs,the domains binding cyclic nucleotide and calmodulin overlap in plant CNGCs(Ko¨hler et al. 1999)enabling cross-talk between cyclic nucleotides and calmodulin signaling (Arazi et al.2000).At least some CNGCs show equal permeability for K+and Na+(Balague et al.2003;Bridges et al.2005)and may thus impact on cytosolic K/Na ratios under saline conditions(Maathuis and Sanders2001),and one specific channel(AtCNGC2)is probably highly selective for K+(Hua et al.2003).Ionotropic Glutamate receptors(iGluRs)form NSCCs in animals;whether they form functional channels in planta has yet to be established.In Arabidopsis,20 genes are reported to encode putative glutamate receptor subunits(Lacombe et al. 2001).The high expression levels of all AtGLR genes in Arabidopsis roots imply that they are important in regulating ion(including K+)uptake from the soil(Chiu et al.2002).A possible signaling role has been also postulated(Lam et al.1998). 3Regulation of K+Channel Activity Under Saline Conditions3.1K+Channels and“Osmotic”and“Ionic”Componentsof Salt StressSalinity causes a plethora of physiological responses including a deceleration of the growth of root tips,shoots,and young leaves,as well as stomatal closure (Munns and Tester2008).All these effects may be attributed to the impact ofsalinity on K +transport across the plasma membrane (PM).Not only do high concentrations of Na +in the soil reduce the activity of K +,making it less available for plants,but K +uptake is also significantly reduced as a result of the direct competition between Na +and K +for uptake sites at the PM,including both low-(e.g.,NSCC)and high-(e.g.,KUP and HKT)affinity transporters (Fig.1;see also Shabala and Cuin 2008for more details).Even more important is NaCl-induced PM depolarization.Depending on NaCl concentration and plant species,40–80mV PM depolarization was reported in both root (Shabala et al.2005a ;Chen et al.2007b;Cuin et al.2008)and leaf (Shabala 2000;Shabala et al.2005b )tissues.Such depolarization makes passive K +uptake through inward-rectifying K +channels thermodynamically impossible and,at the same time,dramatically increases K +efflux through depolarization-activated outward-rectifying K +chan-nels (Fig.1).According to the modern view,a major portion of Na +influx in the root occurs via NSCC channels (see Demidchik and Maathuis 2007for a review).This results in a significant PM depolarization.At the same time,changes in solution osmolality are sensed by a putative osmosensor and are translated into increased activity of the electrogenic H +-ATPase (reviewed in Shabala and Cuin 2008),resulting in membrane hyperpolarization.The downstream targets of each of these components are voltage-dependent hyperpolarization-(KIR)and depolar-ization (KOR)-activated Shaker-type K +channels.Depending on the severity of the salt stress,one of components dominates,resulting in either increased K +uptake (at mild salinity levels),or in a massive K +loss (at more severe salt concentrations)from the cell.Increased H +-pump activity might also provide an additional driving force for the high-affinity K +uptake via HAK/KUP transpor-ters.This issue is discussed further in Sect.2in Chapter “Cation Channels and the Uptake of Radiocaesium by Plants”.VacuoleKORDPZ NSCC NaClATPo s m o s e n s o r HPZ KIR HAK/KUPNa +K +K +K +H +H +Fig.1Potassium transporters and cellular mechanisms of perception of ionic and osmotic components of salt stress.See text for explanations.Reproduced from Shabala and Cuin 2008,with permission of Physiologia PlantarumPotassium and Potassium-Permeable Channels in Plant Salt Tolerance 9394S.Shabala and I.I.Pottosin 3.2GORK and AKT Channels as Downstream Targetsof Salinity EffectsThe model shown in Fig.1highlights the importance of voltage gating and impli-cates Shaker-type K+channels as possible downstream targets during salinity stress signaling.This model was validated in direct electrophysiological experiments using a range of Arabidopsis transport mutants(Shabala and Cuin2008)as well as concurrent measurements of net K+fluxes,membrane potential changes,and intracellular cell turgor pressure(Shabala and Lew2002).No significant difference in NaCl-induced K+efflux was found between WT Columbia and the akt1mutant, while gork roots showed a much more attenuated response to salinity treatment (Shabala and Cuin2008).At the same time,hyperosmotic mannitol treatment caused similar K+uptake in WT and gork roots,but had essentially no impact on K+fluxes in akt1roots.Also,clamping the membrane voltage at values positive and negative of E K led to switching of the K+flux from net efflux to influx and vice versa in Arabidopsis root hairs(Shabala and Lew2002).Given the strong correlation between the ability of the root to retain K+and plant salinity tolerance (Chen et al.2005,2007a,2007b;Cuin et al.2008;Smethurst et al.2008),these findings point to the GORK channel as a main downstream target of the detrimental effects of salinity.In addition,GORK activity may impact on salinity tolerance via its modulation by both exogenous and endogenous factors.Four major lines of evidence support the above statement:(1)In addition to NSCC(Demidchik et al.2002),GORK channels appear to be thekey target for ameliorative effects of supplementary Ca2+and some other divalent cations as revealed in patch-clamp(Shabala et al.2006)and pharma-cological(Shabala et al.2003,2005b)studies.(2)NaCl induced K+leak from roots occurs mainly via GORK channels;decreasesin its activity are associated with a lower salt-induced depolarization and thus confers salt tolerance(Chen et al.2005,2007b;Cuin et al.2008).(3)Detrimental effects of salinity may be also ameliorated by either exogenousapplication of compatible solutes(Harinasut et al.1996)or by overexpressing genes responsible for biosynthesis of various compatible solutes(Bohnert et al.1995;Bray1997).The recent work in our laboratory has shown that compatible solutes prevent NaCl-induced K+leakage from the cell,because of both the enhanced activity of the H+-ATPase(Cuin and Shabala2005,2007a)and a decreased induction of TEA+-sensitive K+leak by ROS(Demidchik et al.2003;Cuin and Shabala2007b).The specific mechanisms by which ROS stress activates K+-permeable ion channels in plant membranes are discussed in this volume in chapter11by Demidchik.(4)In animal tissues,caspase activity is significantly increased by a low cytosolicK+content(Hughes and Cidlowski1999),and a decrease in cytosolic K+pool was suggested as a trigger for the PCD in plant cells(Demidchik,personal communication;Shabala2009).Consistent with this notion,expression of thePotassium and Potassium-Permeable Channels in Plant Salt Tolerance95 animal CED-9anti-apoptotic gene significantly increases plant salinity and oxidative stress tolerance by blocking K+efflux via KORs and NSCCs(Shabala et al.2007a).3.3Voltage Gating and the Role of H+-ATPasesSalt stress rapidly stimulates H+pumping by plasma membrane ATPases(Shabala 2000),most likely because of its modulation by14-3-3protein binding(Babakov et al.2000).Such activation will tend to reduce membrane depolarization,attenu-ating or preventing K+efflux.In this context,salt-tolerant barley varieties had2–2.5 fold intrinsically higher rates of H+-ATPase pumping(Chen et al.2007a).As a result,these varieties were capable of maintaining~10mV more negative mem-brane potential in root epidermal cells and displayed~3-fold less NaCl-induced K+ efflux.In addition,stimulation of H+-pumping will acidify the apoplast,thus improving the K+/Na+selectivity of the ion channel mediated transport.Indeed, such acidification will cause opposite effects on K+-selective channels and NSCC, activation and inhibition,respectively(Amtmann et al.2004).It is not quite clear how long the NaCl-induced plasma membrane depolarization lasts and when the potential becomes sufficiently negative to allow the activation of K+uptake via inward rectifier K+channels.It appears that the effect is highly species-specific.It was shown that a salt-tolerant species,Thelluingiella halophila, was able to recuperate the initial very negative potential difference value while its salt-sensitive relative,Arabidopsis thaliana,was not(Volkov and Amtmann2006).Long-term potentiation of the H+-ATPase activity may be achieved via facil-itating of14-3-3protein binding by spermine,accumulated in salinized tissues (Garufiet al.2007).14-3-3proteins may also directly regulate K+channel activity; they are required for KIR activity and could differentially modulate KORs(Bunney et al.2002;Wijngaard et al.2005).Thus,it appears that14-3-3proteins may control intracellular K+homeostasis both directly,by regulating K+permeable channels, and indirectly,by modulating H+-ATPase activity.This is consistent with the idea that14-3-3proteins might mediate cross-talk between the salt stress and potassium signaling pathways in plant roots(Xu and Shi2006).3.4Maintaining the Optimal Cytosolic K+/Na+RatioBeing an overall result of Na+/K+exchange across the plasma membrane,regula-tion of K+efflux is a more complex trait than control of either Na+uptake or exclusion.The extent of NaCl-induced K+efflux depends on both the magnitude of salt-induced membrane depolarization(H+pump activity vs Na+entrance through NSCC)and on the activity of all K+-release channels(e.g.,KOR and NSCC). Also,the initial rapid loss of cytosolic K+is rapidly reversed through repletion by96S.Shabala and I.I.Pottosin K+from the vacuole(Shabala et al.2006).Thus,regulation of K+transport across membranes of other organelles has to be considered.However,the above repletion could only postpone but not abolish the need to reabsorb K+from the salinized soil. As a result,plants with initially better designed PM Na+and K+transport systems (e.g.,with a reduced Na+influx and K+efflux)will have a longer lasting advantage. Comparative analysis of ion currents in Thellungiella and Arabidopsis may serve as an interesting extension of this principle.Both KOR and NSCC in T.halophila root plasma membranes display a higher degree of the K+/Na+selectivity(Volkov et al. 2004).Consequently,Na+influx in T.halophila was reduced as compared to A.thaliana.Moreover,despite a higher(and more energy consuming)Na+export from roots,A.thaliana accumulated more Na+(Wang et al.2006).In addition,leaf epidermal cells of T.halophila also displayed a higher ratio of Na+to K+currents compared with mesophyll cells,which could underlie the preferential accumulation of Na+in the leaf epidermis as compared to the tissues with higher metabolic workloads.Leaf epidermis and mesophyll could possess contrasting concentrations of cytosolic Na+and K+(Karley et al.2000),which argues for a mainly apoplastic way of communication between these cell types.A redistribution of Na+and K+between these cell types in salinized barley leaves seems to be an important strategy for maintaining constant K+activity and a high K+/Na+ratio in the cytosol of mesophyll cells(Cuin et al.2003).3.5Long-term Salinity Exposure and Regulationof K+TransportLong-term salinity exposure adds another dimension to the regulation of K+ transport,causing tissue-and genotype-specific changes in the expression levels of K+channels.In addition to GORK channels,several more Shaker-type channels are involved in plant adaptive responses to salinity.These are briefly summarized in Table1and are commented on below.AKT1channels are most abundant in root epidermis and responsible for K+ uptake by roots.Not being perfectly K+-selective,AKT1may mediate some Na+ influx when the Na+/K+ratio in the external medium is high(Amtmann and Sanders 1999).Therefore,it comes as little surprise that in halophytes(e.g.,ice plant) and relatively tolerant rice cultivars,expression levels of this channel are down-regulated(Table1)whereas this did not occur in salt-sensitive rice and Arabidopsis (Golldack et al.2003).Interestingly,salt tolerant rice cultivars differed from the salt sensitive ones by a slower accumulation of Na+,whereas K+content remained relatively constant.This ability for Na+exclusion was lost,however,at very low external K+(Golldack et al.2003).This not only emphasizes the importance of maintaining a high K+/Na+ratio,but also implies the participation of additional K+import systems.On the other hand,Atakt1mutants displayed a higher salt-sensitivity(Qi and Spalding2004).It appears,therefore,that the expression of thisT a b l e 1E x p r e s s i o n p a t t e r n s o f p l a s m a m e m b r a n e S h a k e r -t y p e p o t a s s i u m c h a n n e l s i n s a l t -s t r e s s e d p l a n t s N a m e (S p e c i e s )T y p e L o c a t i o n E x p r e s s i o n u n d e r s a l t s t r e s s R e f e r e n c e s A K T 1(A r a b i d o p s i s )I R R o o t c o r t e x ,e p i d e r m i s ,e n d o d e r m i s &h a i r s ;l e a f m e s o p h y l l M o s t l y u n c h a n g e d i n r o o t s a n d s h o o t s P i l o t e t a l .2003A K T 1(r i c e )I R R o o t (e p i d e r m i s a n d e n d o d e r m i s m o s t l y );l e a v e s x y l e m p a r e n c h y m a ;p h l o e m m e s o p h y l l M o s t l y u n c h a n g e d i n s e n s i t i v e v a r i e t i e s ;d o w n -r e g u l a t e d i n r o o t s a n d c o l e o p t i l e s o f t o l e r a n t g e n o t y p e s G o l l d a c k e t a l .2003;F u c h s e t a l .2005M K T 1(i c e p l a n t )I R R o o t s S t r o n g l y d o w n r e g u l a t e d S u e t a l .2001A K T 2/3(A r a b i d o p s i s )W I R P h l o e m ,x y l e m ,l e a f m e s o p h y l l ,g u a r d c e l l D o w n -r e g u l a t e d i n s h o o t s P i l o t e t a l .2003U p -r e g u l a t e d i n l e a v e s M K T 2(i c e p l a n t )L e a v e s ,s t e m s ,flo w e r s s e e d c a p s u l e s N o s i g n i fic a n t c h a n g e s S u e t a l .2001K C T 2(B r a s s i c a r a p a )U p r e g u l a t e d Z h a n g e t a l .2006K A T 1(A r a b i d o p s i s )I R G u a r d c e l l S z y r o k i e t a l .2001K A T 1(r i c e )I R I n t e r n o d e s ,r a c h i d e s I m p r o v e s K /N a r a t i o O b a t a e t a l .2007K M T 1(i c e p l a n t )L e a v e s ,s t e m s ,s e e d c a p s u l e s S t r o n g l y u p r e g u l a t e d S u e t a l .2001S K O R (A r a b i d o p s i s )O R R o o t p e r i c y c l e ;s t e l a r p a r e n c h y m a c e l l s N o s i g n i fic a n t c h a n g e sP i l o t e t a l .2003;Q i a n d S p a l d i n g 2004K C 1(A r a b i d o p s i s )R o o t s ,s h o o t s ,l e a v e sS t r o n g u p -r e g u l a t i o n i n s h o o t s a n dl e a v e s ;n o c h a n g e s i n r o o t sR e i n t a n z e t a l .2002;P i l o t e t a l .2003I R i n w a r d r e c t i fie r ,O R o u t w a r d r e c t i fie r ,W I R w e a k l y i n w a r d r e c t i f y i n g c h a n n e lPotassium and Potassium-Permeable Channels in Plant Salt Tolerance97。