数学分析课件1
数学分析完整版本ppt课件
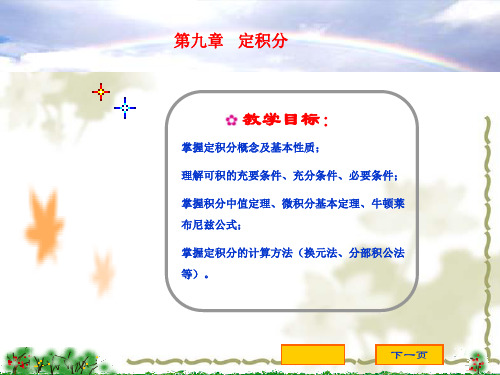
返回
牛 顿(I.Newton 1642.12.25—1727.3.3)
英国数学家和物理学家出生在一个农民家庭,出生前父亲就去世了, 三岁母亲改嫁,由外祖母抚养。1661年入剑桥大学,1665年获学士学位, 1668年获硕士学位。由于他出色的成就,1669年巴鲁(Barrow)把数学 教授的职位让给年仅26岁的牛顿。1703 年被选为英国皇家学会会长。牛 顿一生成就辉煌,堪称科学巨匠。最突出的有四项重大贡献:创立微积 分,为近代数学奠定了基础,推动了整个科学技术的发展。他发现了力 学三大定律,为经典力学奠定了基础;他发现了万有引力为近代天文学 奠定了基础;他对光谱分析的实验,为近代光学奠定了基础 。他的巨著 《自然哲学的数学原理》影响深远,他被公认为历史上伟大的科学家。可 惜他晚年研究神学,走了弯路。
n
n
1
i
2
n
1 n
它的面积
ΔSi
(1
i2 n2
)
1 n
所求的总面积
Sn
n (1 i1
i2 n2
)
1 n
1
1 n3
n
i
2
i 1
1
2n
2 3n 6n 2
1
2 3
我 们 分 别 取 n=10, 50, 100 用 计 算 机 把 它 的 图 象 画 出 来 , 并 计
算出面积的近似值:
clf, n=10; x=0:1/n:1;
四.小结: 学习定积分,不仅要理解、记住定积分的定义,还要学习建立定积分概念
的基本思想,我们以后的学习中还会遇到其它类型的积分,比如勒贝格积分、
斯蒂疌斯积分等,只要理解了定积分的思想,其他类型的积分就很容易理解了。
现在我们再来总结一下定积分建立的的思想和方法:从定积分的实例和概念中
大学数学分析ppt课件
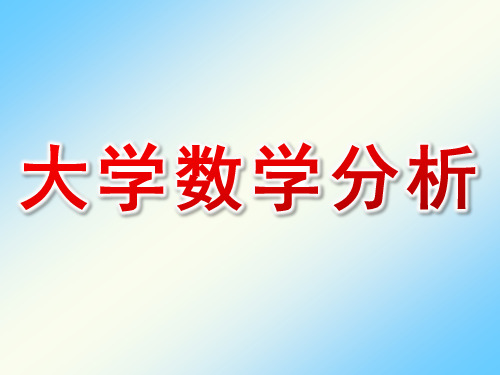
Having a child fall asleep in your arms is one of the most peaceful feeling in the world. 让一个孩子在你的臂弯入睡,你会体会到世间最安宁的感觉.
Being kind is more important than being right. 善良比真理更重要.
§1 微分中值定理 §2 L’Hospital法则 §3 Taylor公式和插值多项式 §4 函数的Taylor公式及其应用 §5 应用举例 §6 方程的近似求解
第六章 不定积分
§1 不定积分的概念和运算法则 §2 换元积分法和分部积分法 §3 有理函数的不定积分及其应用
目 录 (上册)
第七章 定积分
You should never say no to a gift from a child. 永远不要拒绝孩子送给你的礼物.
Sometimes all a person needs is a hand to hold and a heart to understand. 有时候,一个人想要的只是一只可握的手和一颗感知的心.
➢通过严格的训练,具备熟练的运算能力与技巧;
➢注重微积分的应用,掌握数学模型的思想与方法, 提高应用微积分这一有力的数学工具分析问题、解 决问题的能力。
目 录 (上册)
第一章 集合与映射
§1 集合 §2 映射与函数
第二章 数列极限
§1 实数系的连续性 §2 数列极限 §3 无穷大量 §4 收敛准则
Love ,not time,heals all wounds. 治愈一切h,but I'm tougher. 生活是艰苦的,但我应更坚强.
数学分析课件
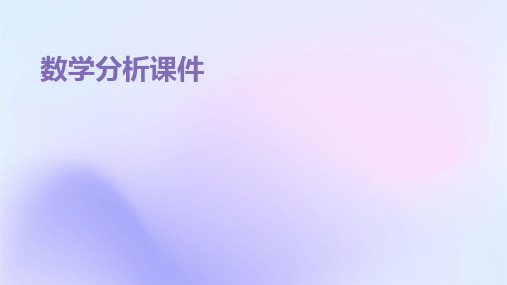
长度的计算
利用定积分可以计算曲线的长度,以及物体的周长。
06
高阶导数与高阶积分
高阶导数的计算与性质
高阶导数的计算方法
定义法:根据导数的定义,对函数进行多次求 导,适用于简单的函数。
莱布尼茨法则:利用已知的导数公式,通过递 推关系计算高阶导数,适用于较复杂的函数。
高阶导数的计算与性质
线性性质:若$f(x)$和$g(x)$的$n$阶导数存在 ,则$(a f+b g)^{(n)}=a f^{(n)}+b g^{(n)}$ 。
数学分析课件
目录
• 数学分析概述 • 数学分析的基本性质 • 极限理论及其应用 • 微分学及其应用 • 定积分及其应用 • 高阶导数与高阶积分 • 数学分析中的重要定理与问题
01
数学分析概述
定义与意义
定义
数学分析是研究函数、序列、极限、 微积分等概念与方法的分支,是数学 的基础学科。
意义
数学分析在数学领域中占据重要地位 ,为其他数学分支提供基础理论和工 具,也是许多实际应用领域的基础。
THANKS。
积分的基本性质
积分具有可加性、可减性、可乘性和可除性 。
积分的基本公式
积分的基本公式包括求导公式、微分公式、 乘积公式、幂函数公式等。
积分的方法
积分的方法包括换元法、分部积分法、有理 函数积分法等。
积分的应用:面积、体积、长度
面积的计算
利用定积分可以计算曲线下面积,以及平面图 形面积。
体积的计算
利用定积分可以计算旋转体的体积,以及立体 的体积。
分区求和法:将积分区间划分为若干小区间,在每个小 区间上应用牛顿-莱布尼茨公式计算积分,再求和得到 总积分值。
《数学分析》课件
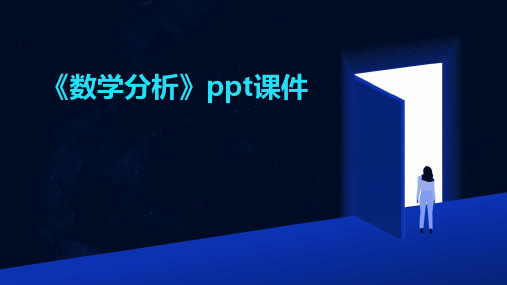
函数与极限
函数
函数是数学分析中的基本概念之一,它是一个从定义域到值域的映射。根据定义域和值域的不同,函数可以分为 不同的类型,如连续函数、可微函数等。
极限
极限是数学分析中描述函数在某一点的行为的工具。极限的定义包括数列的极限和函数的极限,它们都是描述函 数在某一点附近的行为。极限的概念是数学分析中最重要的概念之一,它是研究函数的连续性、可导性、可积性 等性质的基础。
复合函数的导数
复合函数的导数是通过对原函数进行 求导,再乘以中间变量的导数得到的 。
微分及其应用
微分的定义
微分是函数在某一点附近的小变化量 ,可以理解为函数值的近似值。
微分的应用
微分在近似计算、误差估计、求切线 、求极值等方面有着广泛的应用。例 如,在求极值时,可以通过比较一阶 导数在极值点两侧的正负性来确定极 值点。
数列的极限
总结词
数列极限的定义与性质
详细描述
数列极限是数学分析中的一个基本概念,它描述了数列随 着项数的增加而趋近于某个固定值的趋势。极限具有一些 重要的性质,如唯一性、四则运算性质、夹逼定理等。
总结词
数列极限的证明方法
详细描述
证明数列极限的方法有多种,包括定义法、四则运算性质 、夹逼定理、单调有界定理等。这些方法可以帮助我们证 明数列的极限并理解其性质。
含参变量积分的概念与性质
含参变量积分的概念
含参变量积分是指在积分过程中包含一个或多个参数的积分。这种积分在处理一些具有参数的物理问题时非常有 用。
含参变量积分的性质
含参变量积分具有一些重要的性质,如参数可分离性、参数连续性、参数积分区间可变性等。这些性质使得含参 变量积分在解决实际问题时更加灵活和方便。
反常积分与含参变量积分的计算方法
数学分析第一章
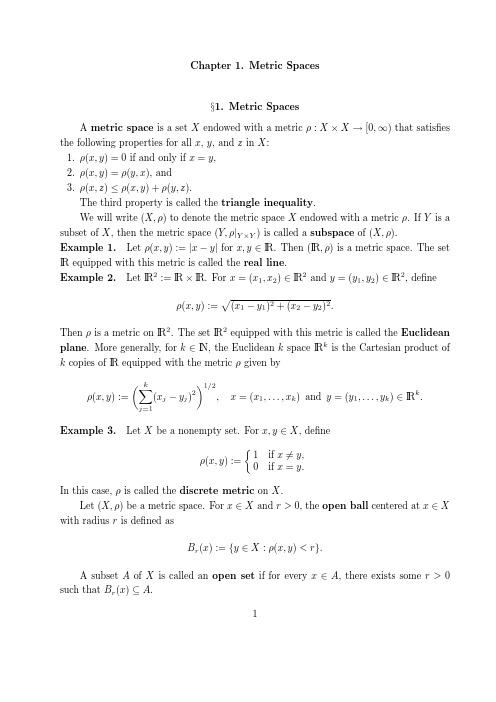
Chapter1.Metric Spaces§1.Metric SpacesA metric space is a set X endowed with a metricρ:X×X→[0,∞)that satisfies the following properties for all x,y,and z in X:1.ρ(x,y)=0if and only if x=y,2.ρ(x,y)=ρ(y,x),and3.ρ(x,z)≤ρ(x,y)+ρ(y,z).The third property is called the triangle inequality.We will write(X,ρ)to denote the metric space X endowed with a metricρ.If Y is a subset of X,then the metric space(Y,ρ|Y×Y)is called a subspace of(X,ρ).Example1.Letρ(x,y):=|x−y|for x,y∈I R.Then(I R,ρ)is a metric space.The set I R equipped with this metric is called the real line.Example2.Let I R2:=I R×I R.For x=(x1,x2)∈I R2and y=(y1,y2)∈I R2,defineρ(x,y):=(x1−y1)+(x2−y2).Thenρis a metric on I R2.The set I R2equipped with this metric is called the Euclidean plane.More generally,for k∈I N,the Euclidean k space I R k is the Cartesian product of k copies of I R equipped with the metricρgiven byρ(x,y):=kj=1(x j−y j)21/2,x=(x1,...,x k)and y=(y1,...,y k)∈I R k.Example3.Let X be a nonempty set.For x,y∈X,defineρ(x,y):=1if x=y, 0if x=y.In this case,ρis called the discrete metric on X.Let(X,ρ)be a metric space.For x∈X and r>0,the open ball centered at x∈X with radius r is defined asB r(x):={y∈X:ρ(x,y)<r}.A subset A of X is called an open set if for every x∈A,there exists some r>0 such thatB r(x)⊆A.1Theorem1.1.For a metric space(X,ρ)the following statements are true.1.X and∅are open sets.2.Arbitrary unions of open sets are open sets.3.Finite intersections of open sets are open sets.Proof.Thefirst statement is obviously true.For the second statement,we let(A i)i∈I be a family of open subsets of X and wish to prove that∪i∈I A i is an open set.Suppose x∈∪i∈I A i.Then x∈A ifor some i0∈I.Since A i0is an open set,there exists some r>0such that B r(x)⊆A i.Consequently,B r(x)⊆∪i∈I A i.This shows that∪i∈I A i is an open set.For the third statement,we let{A1,...,A n}be afinite collection of open subsets of X and wish to prove that∩n i=1A i is an open set.Suppose x∈∩n i=1A i.Then x∈A i for every i∈{1,...,n}.For each i∈{1,...,n},there exists r i>0such that B ri(x)⊆A i. Set r:=min{r1,...,r n}.Then r>0and B r(x)⊆∩n i=1A i.This shows that∩n i=1A i is an open set.Let(X,ρ)be a metric space.A subset B of X is called an closed set if its complement B c:=X\B is an open set.The following theorem is an immediate consequence of Theorem1.1.Theorem1.2.For a metric space(X,ρ)the following statements are true.1.X and∅are closed sets.2.Arbitrary intersections of closed sets are closed sets.3.Finite unions of closed sets are closed sets.Let(X,ρ)be a metric space.Given a subset A of X and a point x in X,there are three possibilities:1.There exists some r>0such that B r(x)⊆A.In this case,x is called an interiorpoint of A.2.For any r>0,B r(x)intersects both A and A c.In this case,x is called a boundarypoint of A.3.There exists some r>0such that B r(x)⊆A c.In this case,x is called an exteriorpoint of A.For example,if A is a subset of the real line I R bounded above,then sup A is a boundary point of A.Also,if A is bounded below,then inf A is a boundary point of A.A point x is called a closure point of A if x is either an interior point or a boundary point of A.We denote by A the set of closure points of A.Then A⊆A.The set A is called the closure of A.2Theorem1.3.If A is a subset of a metric space(X,ρ),then A is the smallest closed set that includes A.Proof.Let A be a subset of a metric space.Wefirst show that A is closed.Suppose x/∈A. Then x is an exterior point of A;hence there exists some r>0such that B r(x)⊆A c.If y∈B r(x),thenρ(x,y)<r.Forδ:=r−ρ(x,y)>0,by the triangle inequality we have Bδ(y)⊆B r(x).It follows that Bδ(y)⊆A c.This shows y/∈A.Consequently,B r(x)⊆A c. Therefore,A c is open.In other words,A is closed.Now assume that B is a closed subset of X such that A⊆B.Let x∈B c.Then there exists r>0such that B r(x)⊆B c⊆A c.This shows x∈A c.Hence,B c⊆A c.It follows that A⊆B.Therefore,A is the smallest closed set that includes A.A subset A of a metric space(X,ρ)is said to be dense in X if A=X.§pletenessLet(x n)n=1,2,...be a sequence of elements in a metric space(X,ρ).We say that (x n)n=1,2,...converges to x in X and write lim n→∞x n=x,ifρ(x n,x)=0.limn→∞From the triangle inequality it follows that a sequence in a metric space has at most one limit.Theorem2.1.Let A be a subset of a metric space(X,ρ).Then a point x∈X belongs to A if and only if there exists a sequence(x n)n=1,2,...in A such that lim n→∞x n=x. Proof.If x∈A,then B1/n(x)∩A=∅for every n∈I N.Choose x n∈B1/n(x)∩A for each n∈I N.Thenρ(x n,x)<1/n,and hence lim n→∞x n=x.Suppose x/∈A.Then there exists some r>0such that B r(x)∩A=∅.Consequently, for any sequence(x n)n=1,2,...in A,we haveρ(x n,x)≥r for all n∈I N.Thus,there is no sequence of elements in A that converges to x.A sequence(x n)n=1,2,...in a metric space(X,ρ)is said to be a Cauchy sequence if for any givenε>0there exists a positive integer N such thatm,n>N impliesρ(x m,x n)<ε.Clearly,every convergent sequence is a Cauchy sequence.If a metric space has the property that every Cauchy sequence converges,then the metric space is said to be complete.For example,the real line is a complete metric space.3The diameter of a set A is defined byd(A):=sup{ρ(x,y):x,y∈A}.If d(A)<∞,then A is called a bounded set.Theorem2.2.Let(X,ρ)be a complete metric space.Suppose that(A n)n=1,2,...is a sequence of closed and nonempty subsets of X such that A n+1⊆A n for every n∈I N and lim n→∞d(A n)=0.Then∩∞n=1A n consists of precisely one element.Proof.If x,y∈∩∞n=1A n,then x,y∈A n for every n∈I N.Hence,ρ(x,y)≤d(A n)for all n∈I N.Since lim n→∞ρ(A n)=0,it follows thatρ(x,y)=0,i.e.,x=y.To show∩∞n=1A n=∅,we proceed as follows.Choose x n∈A n for each n∈I N.Since A m⊆A n for m≥n,we haveρ(x m,x n)≤d(A n)for m≥n.This in connection with the assumption lim n→∞d(A n)=0shows that(x n)n=1,2,...is a Cauchy sequence.Since (X,ρ)is complete,there exists x∈X such that lim n→∞x n=x.We have x m∈A n for all=A n.This is true for all n∈I N.Therefore,x∈∩∞n=1A n.m≥n.Hence,x∈A§pactnessLet(X,ρ)be a metric space.A subset A of X is said to be sequentially compact if every sequence in A has a subsequence that converges to a point in A.For example,afinite subset of a metric space is sequentially compact.The real line I R is not sequentially compact.But a bounded closed interval in the real line is sequentially compact.A subset A of a metric space is called totally bounded if,for every r>0,A can be covered byfinitely many open balls of radius r.For example,a bounded subset of the real line is totally bounded.On the other hand, ifρis the discrete metric on an infinite set X,then X is bounded but not totally bounded. Theorem3.1.Let A be a subset of a metric space(X,ρ).Then A is sequentially compact if and only if A is complete and totally bounded.Proof.Suppose that A is sequentially compact.Wefirst show that A is complete.Let (x n)n=1,2,...be a Cauchy sequence in A.Since A is sequentially compact,there exists a )k=1,2,...that converges to a point x in A.For anyε>0,there exists subsequence(x nka positive integer N such thatρ(x m,x n)<ε/2whenever m,n>N.Moreover,there exists some k∈I N such that n k>N andρ(x n,x)<ε/2.Thus,for n>N we havek4ρ(x n,x)≤ρ(x n,x nk )+ρ(x nk,x)<ε.Hence,lim n→∞x n=x.This shows that A iscomplete.Next,if A is not totally bounded,then there exists some r>0such that A cannot be covered byfinitely many open balls of radius r.Choose x1∈A.Suppose x1,...,x n∈A have been chosen.Let x n+1be a point in the nonempty set A\∪n i=1B r(x i).If m,n∈I N and m=n,thenρ(x m,x n)≥r.Therefore,the sequence(x n)n=1,2,...has no convergent subsequence.Thus,if A is sequentially compact,then A is totally bounded.Conversely,suppose that A is complete and totally bounded.Let(x n)n=1,2,...be a sequence of points in A.We shall construct a subsequence of(x n)n=1,2,...that is a Cauchy sequence,so that the subsequence converges to a point in A,by the completeness of A.For this purpose,we construct open balls B k of radius1/k and corresponding infinite subsets I k of I N for k∈I N recursively.Since A is totally bounded,A can be covered byfinitely many balls of radius1.Hence,we can choose a ball B1of radius1such that the set I1:={n∈I N:x n∈B1}is infinite.Suppose that a ball B k of radius1/k and an infinite subset I k of I N have been constructed.Since A is totally bounded,A can be covered by finitely many balls of radius1/(k+1).Hence,we can choose a ball B k+1of radius1/(k+1) such that the set I k+1:={n∈I k:x n∈B k+1}is infinite.Choose n1∈I1.Given n k,choose n k+1∈I k+1such that n k+1>n k.By our construction,I k+1⊆I k for all k∈I N.Therefore,for all i,j≥k,the points x niandx nj are contained in the ball B k of radius1/k.It follows that(x nk)k=1,2,...is a Cauchysequence,as desired.Theorem3.2.A subset of a Euclidean space is sequentially compact if and only if it is closed and bounded.Proof.Let A be a subset of I R k.If A is sequentially compact,then A is totally bounded and complete.In particular,A is bounded.Moreover,as a complete subset of I R k,A is closed.Conversely,suppose A is bounded and closed in I R k.Since I R k is complete and A is closed,A is complete.It is easily seen that a bounded subset of I R k is totally bounded.Let(A i)i∈I be a family of subsets of X.We say that(A i)i∈I is a cover of a subset A of X,if A⊆∪i∈I A i.If a subfamily of(A i)i∈I also covers A,then it is called a subcover. If,in addition,(X,ρ)is a metric space and each A i is an open set,then(A i)i∈I is said to be an open cover.Let(G i)i∈I be an open cover of A.A real numberδ>0is called a Lebesgue number for the cover(G i)i∈I if,for each subset E of A having diameter less thanδ,E⊆G i for5some i∈I.Theorem3.3.Let A be a subset of a metric space(X,ρ).If A is sequentially compact, then there exists a Lebesgue numberδ>0for any open cover of A.Proof.Let(G i)i∈I be an open cover of A.Suppose that there is no Lebesgue number for the cover(G i)i∈I.Then for each n∈I N there exists a subset E n of A having diameter less than1/n such that E n∩G c i=∅for all i∈I.Choose x n∈E n for n∈I N.Since A is sequentially compact,there exists a subsequence(x nk)k=1,2,...which converges to a point x in A.Since(G i)i∈I is a cover of A,x∈G i for some i∈I.But G i is an open set.Hence, there exists some r>0such that B r(x)⊆G i.We canfind a positive integer k such that1/n k<r/2andρ(x nk ,x)<r/2.Let y be a point in E nk.Since x nkalso lies in the setE nk with diameter less than1/n k,we haveρ(x nk,y)<1/n k.Consequently,ρ(x,y)≤ρ(x,x nk)+ρ(x nk,y)<r2+1n k<r.This shows E nk ⊆B r(x)⊆G i.However,E nkwas so chosen that E nk∩G c i=∅.Thiscontradiction proves the existence of a Lebesgue number for the open cover(Gi)i∈I.A subset A of(X,ρ)is said to be compact if each open cover of A possesses afinite subcover of A.If X itself is compact,then(X,ρ)is called a compact metric space. Theorem3.4.Let A be a subset of a metric space(X,ρ).Then A is compact if and only if it is sequentially compact.Proof.If A is not sequentially compact,then A is an infinite set.Moreover,there exists a sequence(x n)n=1,2,...in A having no convergent subsequence.Consequently,for each x∈A,there exists an open ball B x centered at x such that{n∈I N:x n∈B x}is afinite set.Then(B x)x∈A is an open cover of A which does not possess afinite subcover of A. Thus,A is not compact.Now suppose A is sequentially compact.Let(G i)i∈I be an open cover of A.By Theorem3.3,there exists a Lebesgue numberδ>0for the open cover(G i)i∈I.By Theorem 3.1,A is totally bounded.Hence,A is covered by afinite collection{B1,...,B m}of open balls with radius less thanδ/2.For each k∈{1,...,m},the diameter of B k is less thanδ.Hence,B k⊆G ik for some i k∈I.Thus,{G ik:k=1,...,m}is afinite subcover of A.This shows that A is compact.6§4.Continuous FunctionsLet(X,ρ)and(Y,τ)be two metric spaces.A function f from X to Y is said to be continuous at a point a∈X if for everyε>0there existsδ>0(depending onε)such thatτ(f(x),f(a))<εwheneverρ(x,a)<δ.The function f is said to be continuous on X if f is continuous at every point of X.Theorem4.1.For a function f from a metric space(X,ρ)to a metric space(Y,τ),the following statements are equivalent:1.f is continuous on X.2.f−1(G)is an open subset of X whenever G is an open subset of Y.3.If lim n→∞x n=x holds in X,then lim n→∞f(x n)=f(x)holds in Y.4.f(A)⊆f(A)holds for every subset A of X.5.f−1(F)is a closed subset of X whenever F is a closed subset of Y.Proof.1⇒2:Let G be an open subset of Y and a∈f−1(G).Since f(a)∈G and G is open,there exists someε>0such that Bε(f(a))⊆G.By the continuity of f,there exists someδ>0such thatτ(f(x),f(a))<εwheneverρ(x,a)<δ.This shows Bδ(a)⊆f−1(G). Therefore,f−1(G)is an open set.2⇒3:Assume lim n→∞x n=x in X.Forε>0,let V:=Bε(f(x)).In light of statement2,f−1(V)is an open subset of X.Since x∈f−1(V),there exists someδ>0 such that Bδ(x)⊆f−1(V).Then there exists a positive integer N such that x n∈Bδ(x) for all n>N.It follows that f(x n)∈V=Bε(f(x))for all n>N.Consequently, lim n→∞f(x n)=f(x).3⇒4:Let A be a subset of X.If y∈f(A),then there exists x∈A such that y=f(x).Since x∈A,there exists a sequence(x n)n=1,2,...of A such that lim n→∞x n=x. By statement3we have lim n→∞f(x n)=f(x).It follows that y=f(x)∈f(A).This shows f(A)⊆f(A).4⇒5:Let F be a closed subset of Y,and let A:=f−1(F).By statement4we have f(A)⊆⊆F=F.It follows that A⊆f−1(F)=A.Hence,A is a closed subset of X.5⇒1:Let a∈X andε>0.Consider the closed set F:=Y\Bε(f(a)).By statement5,f−1(F)is a closed subset of X.Since a/∈f−1(F),there exists someδ>0 such that Bδ(a)⊆X\f−1(F).Consequently,ρ(x,a)<δimpliesτ(f(x),f(a))<ε.So f is continuous at a.This is true for every point a in X.Hence,f is continuous on X.As an application of Theorem4.1,we prove the Intermediate Value Theorem for continuous functions.7Theorem 4.2.Suppose that a,b ∈I R and a <b .If f is a continuous function from [a,b ]to I R ,then f has the intermediate value property,that is,for any real number d between f (a )and f (b ),there exists c ∈[a,b ]such that f (c )=d .Proof.Without loss of any generality,we may assume that f (a )<d <f (b ).Since the interval (−∞,d ]is a closed set,the set F :=f −1((−∞,d ])={x ∈[a,b ]:f (x )≤d }is closed,by Theorem 4.1.Let c :=sup F .Then c lies in F and hence f (c )≤d .It follows that a ≤c <b .We claim f (c )=d .Indeed,if f (c )<d ,then by the continuity of f we could find r >0such that c <c +r <b and f (c +r )<d .Thus,we would have c +r ∈F and c +r >sup F .This contradiction shows f (c )=d .The following theorem shows that a continuous function maps compact sets to compact sets.Theorem 4.3.Let f be a continuous function from a metric space (X,ρ)to a metric space (Y,τ).If A is a compact subset of X ,then f (A )is compact.Proof.Suppose that (G i )i ∈I is an open cover of f (A ).Since f is continuous,f −1(G i )is open for every i ∈I ,by Theorem 4.1.Hence,(f −1(G i ))i ∈I is an open cover of A .By thecompactness of A ,there exists a finite subset {i 1,...,i m }of I such that A ⊆∪m k =1f−1(G i k ).Consequently,f (A )⊆∪mk =1G i k .This shows that f (A )is compact.Theorem 4.4.Let A be a nonempty compact subset of a metric space (X,ρ).If f is a continuous function from A to the real line I R ,then f is bounded and assumes its maximum and minimum.Proof.By Theorem 4.3,f (A )is a compact set,and so it is bounded and closed.Let t :=inf f (A ).Then t ∈f (A )=f (A ).Hence,t =min f (A )and t =f (a )for some a ∈A .Similarly,Let s :=sup f (A ).Then s ∈f (A )=f (A ).Hence,s =max f (A )and s =f (b )for some b ∈A .A function f from a metric space (X,ρ)to a metric space (Y,τ)is said to be uni-formly continuous on X if for every ε>0there exists δ>0(depending on ε)such that τ(f (x ),f (y ))<εwhenever ρ(x,y )<δ.Clearly,a uniformly continuous function is continuous.A function from (X,ρ)to (Y,τ)is said to be a Lipschitz function if there exists a constant C f such that τ(f (x ),f (y ))≤C f ρ(x,y )for all x,y ∈X .Clearly,a Lipschitz function is uniformly continuous.8Example.Let f and g be the functions from the interval(0,1]to the real line I R given by f(x)=x2and g(x)=1/x,x∈(0,1],respectively.Then f is uniformly continuous, while g is continuous but not uniformly continuous.Theorem4.5.Let f be a continuous function from a metric space(X,ρ)to a metric space(Y,τ).If X is compact,then f is uniformly continuous on X.Proof.Letε>0be given.Since f is continuous,for each x∈X there exists r x>0suchthatτ(f(x),f(y))<ε/2for all y∈B rx (x).Then(B rx(x))x∈X is an open cover of X.Since X is compact,Theorem3.3tells us that there exists a Lebesgue numberδ>0for this open cover.Suppose y,z∈X andρ(y,z)<δ.Then{y,z}⊆B rx(x)for some x∈X. Consequently,τ(f(y),f(z))≤τ(f(y),f(x))+τ(f(x),f(z))<ε/2+ε/2=ε.This shows that f is uniformly continuous on X.9。
数学分析课件

连续性
01 02
连续性的定义
连续性是函数的一种性质,它描述了函数在某一点处的变化情况。如果 函数在某一点处的左右极限相等且等于该点的函数值,则函数在该点处 连续。
连续性的性质
连续性具有一些重要的性质,如局部保序性、介值定理等。这些性质在 数学分析中有着广泛的应用。
03
连续性的判定
判定一个函数是否连续,可以通过计算该函数的左右极限并检查它们是
否相等来实现。此外,还可以利用连续性的性质进行判定。
导数
导数的定义
导数是函数的一种性质,它描述了函 数在某一点处的切线斜率。导数的定 义包括函数在某一点的导数和函数在 某区间的导数。
导数的性质
导数的计算
计算导数的方法有很多种,如直接法、 乘积法则、复合函数求导法则等。这 些方法可以帮助我们计算一些复杂的 导数表达式。
电子工程
在电子工程中,数学分析用于信号处理、图像处 理和通信系统设计。
计算机科学
在计算机科学中,数学分析用于算法设计、数据 分析和人工智能等领域。
06 数学分析的习题与解答
CHAPTER
习题的选择与解答
精选习题
选择具有代表性的数学分析题目,涵盖各个知识点,难度适中, 适合学生巩固所学内容。
详细解答
极限的计算方法
计算极限的方法有很多种,如直接代入法、分解因式法、等价无穷小替换法、洛必达法则 等。根据不同的情况选择合适的方法可以简化计算过程。
导数问题
导数的定义
导数描述了函数在某一点处的切线斜率,是函数局部性质的一种体现。导数可以分为一阶导数、二阶导数等,高阶导 数可以用来研究函数的拐点、凸凹性等性质。
03 数学分析的定理与证明
《数学分析》PPT课件

当n无限增大时,xn 无限接近于1.
8
数列的极限
研究数列{1 (1)n1 }当 n 时的变化趋势. n
当n无限增大时, xn无限接近于1.
“无限接近”意味着什么?
如何用数学语言刻划它.
|
xn
1|
(1 (1)n1
1)1 n
1 n
xn 1 可以要多么小就多么小,只要n充分大,
则要看 xn 1小到什么要求.
n
yn
b,
且 a b, 则存在 N , 当 n N时,有 xn yn .
26
• Thm 3.6 若对任意正整数 n, 有xn yn ,
且
lim
n
xn
a,
lim
n
yn
b,
则 a b.
• Remark
(1)因为数列的前有限项不影响数列的 极限,故上不等式的条件可减弱为:
“若 N 0,
当 n N 时,xn yn ”;
积仍为无穷大;
∗ 用无零值有界变量去除无穷大仍为无穷大.
例 求 lim ( x 1 x) x
解 lim ( x 1 x) x
32
无穷小与无穷大
注 (1) 无穷大是变量,不能与很大的数混淆; (2)切勿将 lim f ( x) 认为极限存在.
x x0
(3) 无穷大与无界函数的区别: 它们是两个不同的概念. 无穷大一定是无界函数, 但是无界函数 未必是某个过程的无穷大.
9
数列的极限
|
xn
1 |
1 n
给定
1 100
,
由
1 n
1, 100
只要 n 100时,有
xn
1
1, 100
数学分析课件华东师大版

数学分析课件华东师大版
汇报人:
目录
• 引言 • 数学分析基础 • 导数与微分 • 积分学 • 无穷级数 • 多元函数微积分
01
引言
课程简介
01
数学分析是数学专业的一门基础 课程,主要研究实数、函数、极 限、连续性、可微性和积分等概 念及其性质。
02
通过学习数学分析,学生可以掌 握数学的基本原理和方法,培养 逻辑思维能力、抽象思维能力和 解决问题的能力。
总结词
理解无穷级数的定义和性质是掌握无穷级数的基础。
详细描述
无穷级数是数学分析中的一个重要概念,它是由无穷多个数按照一定的规则排列组成的数列。无穷级数具有一些 重要的性质,如线性性质、可加性、可乘性和收敛性等。这些性质在无穷级数的运算和证明中有着广泛的应用。
无穷级数的收敛性判别法
总结词
掌握无穷级数的收敛性判别法是判断无穷级数收敛性的关键。
定积分的计算
牛顿-莱布尼兹公式
分部积分法
牛顿-莱布尼兹公式是计算定积分的常 用方法,它通过求不定积分的原函数 (即不定积分),然后利用原函数计 算定积分。
分部积分法是另一种计算定积分的方 法,通过将两个函数的乘积进行求导 ,将定积分转化为容易计算的积分。
换元法
换元法是一种常用的计算定积分的方 法,通过改变定积分的积分变量或积 分区间,将复杂的积分转化为容易计 算的积分。
极限的性质
极限具有唯一性、局部有界 性、局部保序性、迫近性等 性质。
连续函数的性质
连续函数具有局部有界性、 局部保序性、迫近性等性质 。
偏导数与全微分
偏导数的定义
如果一个函数在某个点的某个 自变量的偏导数存在,则称该 函数在该点关于该自变量可偏
数学分析PPT课件第四版华东师大研制--第8章-不定积分(1)可编辑全文
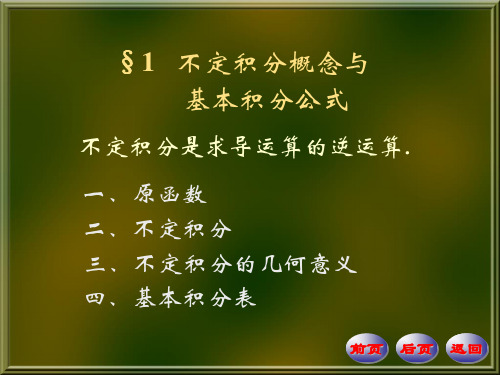
例6 求sec xdx.
解
(解法一)
sec xdx
cos x
cos2 x
dx
d(sin x)
1 sin2 x
1 ln 1 sin x C. 2 1 sin x
(解法二) sec
xdx
sec x(sec x tan sec x tan x
x)
dx
d(sec x tan x sec F (x)是 f (x) 的一个原函数, 则称 y = F (x) 的图
像是 f (x) 的一条积分曲线.
所有的积分曲线都是
y
y F(x) C
由其中一条积分曲线
y F(x)
沿纵轴方向平移而得 到的.
( x0 , y0 )
O
x
前页 后页 返回
满足条件 F( x0 ) y0 的原函数正是在积分曲线中 通过点( x0 , y0 )的那一条积分曲线. 例如, 质点以匀速 v0 运动时, 其路程函数
法则. 定理 8.3 (不定积分的线性运算法则)
若函数 f 与 g 在区间 I 上都存在原函数, k1, k2为
任意常数, 则 k1 f k2 g 在 I上也存在原函数, 且
( k1 f ( x) k2g( x) )dx k1 f ( x)dx k2 g( x)dx.
例1 p( x) a0 xn a1 xn1 an1x an , 则
s(t) v0 dt v0 t C.
若 t0 时刻质点在 s0 处, 且速度为 v0, 则有 s (t ) v0(t t0 ) s0 .
前页 后页 返回
四、基本积分表
由基本求导公式可得以下基本积分公式:
1. 0dx C.
2. 1dx dx x C. 3. xdx x1 C ( 1, x 0).
《数学分析》课件 (完整版)
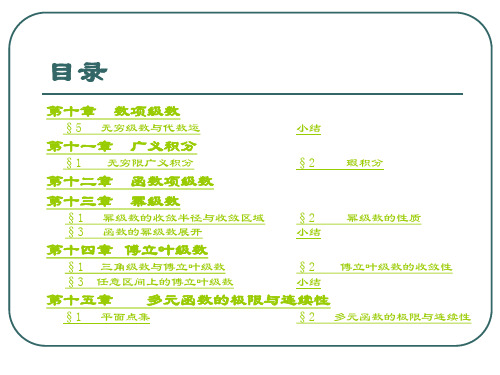
§1 无穷限广义积分
定积分的两个限制
积分区间的有界性 被积函数的有界性 实践中,我们却经常要打破这两个限制。如:关于级数收敛的Cauchy积分判别法;概率统计中,随机变量的空间通常是无限的;第二宇宙速度;物理中的 函数;量子运动;‥‥‥
无穷限积分的定义
设函数 在 有定义,在任意有限区间 上可积。若 存在,则称之为 在 上的广义积分,记为 此时亦称积分 收敛;若 不存在,则称积分 发散。
P.S. 为一符号,表示的是一无穷积分;而当它收敛时,还有第二重意义,可用来表示其积分值。
1. 2. 当 , 均收敛时,定义 显然, 的值与 的选取无关。
类似地,我们可以给出其它无穷积分的定义:
特别地,我们若可利用Taylor公式,求得
则
时 收敛, 时 发散, 时,只能于 时推得 收敛。
Question
我们将参照物取为幂函数 ,而有了上述的比较判别法;那么,将参照物取为指数函数 ,结果又如何呢? 无穷限的广义积分有着与级数非常类似的比较判别法,都是通过估计其求和的对象大小或收敛于0的速度而判断本身的敛散性;而且,我们还有Cauchy积分判别法,使某些级数的收敛与某些无穷限积分的收敛等价了起来。那么,是否可以将关于级数中结论推广至无穷限积分中来呢?某些结论不能推广的原因是什么呢?
1. 结合律
对于收敛级数,可任意加括号,即
2. 交换律
仅仅对于绝对收敛的级数,交换律成立 而对于条件收敛的级数,是靠正负抵消才可求和的,故重排后结果将任意。可见,绝对收敛才是真正的和。
定理 10.19 若级数 绝对收敛,其和为 ,设 为 的任意重排,则 亦绝对收敛,且和仍为
第十章 数项级数
§5 无穷级数与代数运算 有限和中的运算律,如结合律,交换律,分配律,在无穷和中均不成立。具体地,我们有下面的一些结论。
数学分析课件绪论
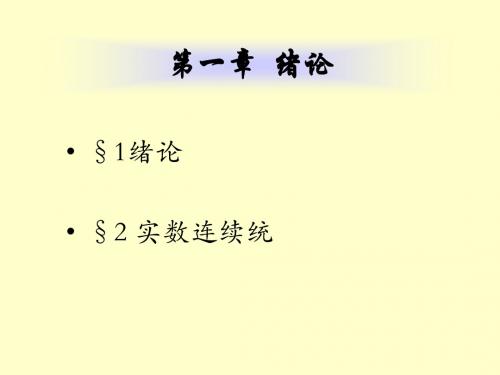
事实上:在整个有理数系中到处都是空隙。从而有理数 系不能刻画连续量。即不能作为连续量的数学表示。 那么,用什么量来表示连续量呢?
思路:扩充有理数系,使新数系是“连续的”
问题:如何扩充?
一个直接的想法:在有理数系中加进一些数, 让它们对应于那些空隙,即把数轴上的空隙都填 满了,这样就可以得到一个“连续”的数系。
•与原来有理数的运算是否一致? 大小关系是否不变? •填多少? •怎么算填满了?
问题:如何判断一个数系是连续的? (即:找出连续量的数学特征。)
Dedekind的名著《连续性与无理数》中写道: “经过长期徒劳的思考,我终于发现,它的实 质是很平凡的。直线上的一点,把直线分成 左右两部分,连续性的本质就在于返回去: 把直线分割成左右两部分,必有唯一的分点”
量
最小的单位,不能分。 离散量 例如:位移: 2、在数学上的表示:正整数:1, 2, 3…… 千米 米cmmm微米纳米…… 或者说:正整数是离散量的数学模型。 1、没有最小的单位,无限可分
连续量 2、连续量的数学表示? 要能进行运算--需要数系的概念
• 集合:把某些东西作为一个整体,称为一个集合, 其中的每一个东西称为这个集合的一个元素. 常见的数集:正整数集,整数集,有理数集, 实数集, 等. • 数系:定义了若干种运算的数集,这些运算满 足一定的规律. 例如:正整数集 N+ 及其上的加 法和乘法运算和在一起构成了正整数系. 1、运算; 2、数集S对运算的封闭性;
7 2 例如: 3 3 3
7 写成 3.6666 3
7 3 0.6666 3
对有尽小数,我们用循环为 0 的有尽小数表示, 而不用循环节为 9的无穷小数表示。
例: 记
1 而不用 0.5 0.50000 是有尽小数, 2
数学分析ppt课件
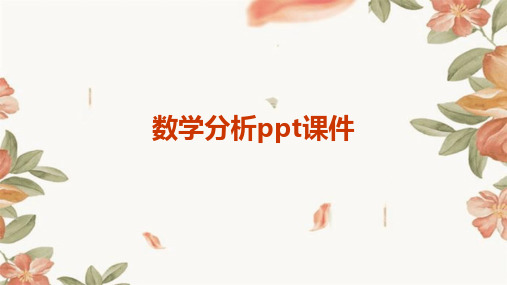
有限覆盖定理
总结词
有限覆盖定理是实数完备性定理中的另一个 重要结论,它涉及到实数集的覆盖问题。
详细描述
有限覆盖定理说明,任意一个开覆盖${(a_n, b_n)}$的实数集都可以被有限个开区间覆盖 。换句话说,对于任意一个实数集$S$,都 存在有限的开区间${(a_1, b_1), (a_2, b_2), ldots, (a_n, b_n)}$,使得$S subseteq cup_{i=1}^{n} (a_i, b_i)$。这个定理在证 明紧空间的性质和实数完备性中起到了关键 作用。
3
实数系中的基本运算
实数系中可以进行加法、减法、乘法和 除法等基本运算,这些运算具有交换律 、结合律、分配律等性质。此外,实数 系中还可以定义绝对值、最大值、最小 值等概念。
极限理论
01
极限的定义
极限是数学分析中的一个基本概念,它描述了当自变量趋向某一值时,
函数值的变化趋势。极限的定义包括数列极限和函数极限两种形式。
详细描述
介绍向量值函数和空间曲线的定义,通过实例说明向量值函 数和空间曲线的性质,并解释其在数学分析中的重要性和应 用。
06
实数完备性定理
区间套定理
总结词
区间套定理是实数完备性定理中的一个 重要组成部分,它描述了闭区间套的性 质。
VS
详细描述
区间套定理指出,如果存在一个闭区间套 ,即一列闭区间${[a_n, b_n]}$,满足 $a_n < b_n$且$a_n < a_{n+1} < b_{n+1} < b_n$(对任意$n$),则该区 间套中至少存在一个实数。这个定理在数 学分析中有着广泛的应用,例如在证明连 续函数的性质和极限理论中。
17-1 数学分析全套课件
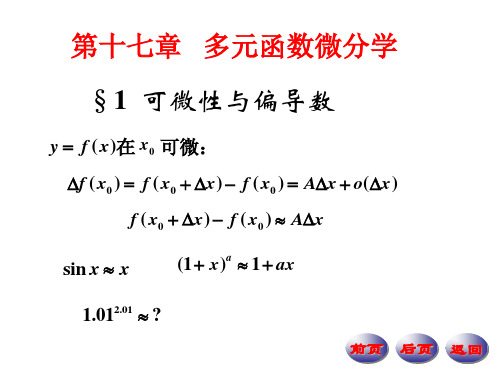
z
P•
O
dh Q•
S
y x
前页 后页 返回
例1 试求抛物面 z a x2 b y2 在点 P( x0, y0, z0 ) 处 的切平面方程与法线方程,其中 z0 a x02 b y02 .
例2 曲面z ( x2 y2 ) 3 在何处的切平面平行
于平面2x 2 y z 0,写出切平面方程
y0 )
fx ( 0x0,, y0x)2x
x2 y2
在 (0, 0)的连续性与可微型
y
2f
y ( 0x0 , y0
前页
)y
后页
0
返回
本次课内容
z f ( x, y) 在 ( x0 , y0 )可微定义 z f ( x0 x, y0 y) f ( x0, y0 )
A x B y o( ),
(1) fx ( x0 , y0 )与 fx ( x0 , y0 ) 存在
(2) lim (x,y )(0,0)
f ( x0 ,
y0 )
fx ( x0 , y0 )x x2 y2
f y ( x0 ,
y0 )y
0
f ( x, y) f ( x0, y0 ) f x ( , y) ( x x0 ) f y ( x0,) ( y y前0 )页. 后页 返回
前页 后页 返回
定理 17.4 曲面 z f ( x, y) 在点 P( x0, y0, f ( x0, y0 )) 存在不平行于 z 轴的切平面的充要条件是: 函数 f 在点 P0( x0 , y0 ) 可微. 此时,切平面方程为
z z0 fx ( x0, y0 )( x x0 ) f y ( x0, y0 )( y y0 ),
f
数学分析华东师大版上第一章ppt课件
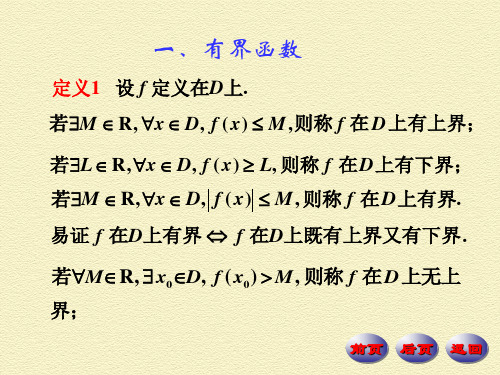
是奇函数
y1 =
1 (ex -e- x ) 的反 2
函数,从而由奇函数的图象性质可知它也是奇函
数.
前页 后页 返回
四、周期函数
定义4 设 f 为 D 上定义的函数. 若 0, 使 x D 必有x D,且 f ( x ) f ( x), 则称 f 为周期函数, 为 f 的一个周期.
一、有界函数
定义1 设 f 定义在D上. 若M R, x D, f ( x) M ,则称 f 在 D上有上界;
若L R,x D, f ( x) L, 则称 f 在D上有下界; 若M R,x D, f ( x) M , 则称 f 在 D上有界. 易证 f 在D上有界 f 在D上既有上界又有下界. 若M R, x0D, f ( x0) M, 则称 f 在 D 上无上 界;
x2n1 1
0
x2n1 2
或
x2n1 1
0
x22n1,
这证明了 y2n1 在 R 上严格增.
前页 后页 返回
例5 易证函数 y [ x]在 R上是增函数, 但非严格 增.
y
3 2 1
2 1 O 1 2 3 4 x
1 2
前页 后页 返回
定理1.2 设 y f ( x), x D为严格增函数,则 f 必 有反函数 f 1,且 f 1在其定义域 f (D)上也是严格 增函数. 类似地, 严格减函数 f 必有反函数 f 1, 且 f 1在其 定义域上也是严格减函数. 证 设 f 在 D 上严格增, 则 y f (D)只有一个 x D, 使 f (x) y. 事实上,若 x1 x2, 使 f ( x1) y f ( x2 ), 则与 f
前页 后页 返回
若L R,x0D, f ( x0) L, 则称 f 在D上无下界; 若MR, x0D, f ( x0) M , 则称 f 在 D上无界.
数学分析 第一章ppt
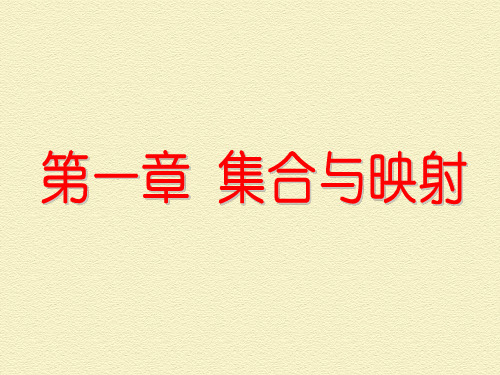
(1) y log(x 1) arctan 1 cos x
2
(2) y f ( x)
g ( x)
, 其中f ( x) 0, g ( x)为初等函数
3
(3) y 1 x x x
2
2016/8/27
解:
( 1 )初等函数 (2)初等函数 因为y f ( x)
2016/8/27
2
; 对于
(5)对于反正弦函数 y arcsin x和反余弦函数
( 例1:求函数y log (x1 ) 16 x )的定义域。
2
解:
16 x 2 0 x 1 0 x 1 1
?
1 x 2或2 x 4 定义域为: D (1, 2) (2, 4)
2016/8/27
解:
x ( 1 )y 与y x是两个不同的函数 ,因为前者 x 的定义域为 (,0) (0,), 后者的定义域为 ( , ),两个函数定义域不 同.
2
(2) y lg( x )与y 2 lg x是两个不同的函数,
2
因为前者的定义域为 (,0) (0,), 后者的定义域为( 0, ),两个函数定 义域不同 .
S X \ S,其中S是X的一个子集
C X
有限集与无限集
若集合S由n个元素组成,n是确定的非负整数,则称
集合S为有限集。
不是有限集的集合称为无限集,前面所说的N,Z,Q,R 都是无限集。
无限集:可列集合不可列集
可列集:若一个无限集上的元素可以按某种规律排成一个序列,或者可以表示成 { } 如 正整数集
(4)反余切函数arc cot x
数学分析课件
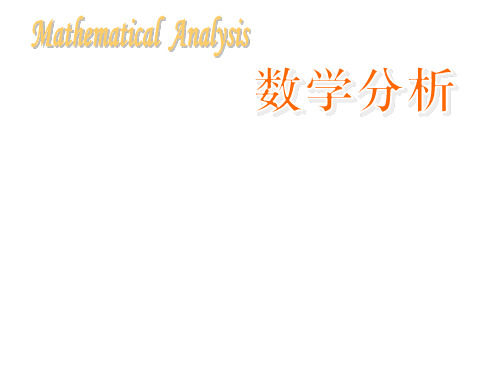
对于一个给定函数来说,局部与整体是 一个事物的两个方面,二者是对立的统一。
因此,微分与积分具有密切关系,积分 问题是由函数的局部性质研究整体性质。建 立二者关系的桥梁是
微积分基本定理——牛顿-莱布尼茨公式。
极限:人类认识无限的必要手段
由于生理的原因,人类只能看到有限时 间、有限范围内的事物;只能判断、测量在 一定时间段内事物的变化量与平均变化速度。 要认识无限变化的事物,要确定事物瞬时变 化的情况等,极限是一个有效工具。
2020/1/23
我 的 教 学 观
2
我的教学观
鱼 渔道 知识 方法 思想 What How Why
SZU
Introduction
1 数学分析的产生与发展 2 数学分析的对象、工具与内容 3 如何学习数学分析
SZU
1
数学分析的产生与发展
The Invention and Development of Mathematical Analysis
3
如何学习数学分析
How to Study Mathematical Analysis
……
也有更多的不能具体通过代数式表示、 但却具有实际意义的函数,以及一般的 抽象函数。
微积分:研究连续性变化
连续性变化的情况涉及到每一个瞬间, 涉及到“无穷小”的时间段内的变化情况, 人类是无法精确捕捉到的。如何研究?
动画片如何表现连续动作? 切片!很短时间内的一种静止画面。
“微小的差异”是微分积分的奥秘!
The Objects, Tools, and Contents of Mathematical Analysis
对象:函数 内容:微分、积分,以及连接微分
与积分的桥梁——微积分基 本定理。 工具:极限
(完整版)数学分析全套课件(华东师大)
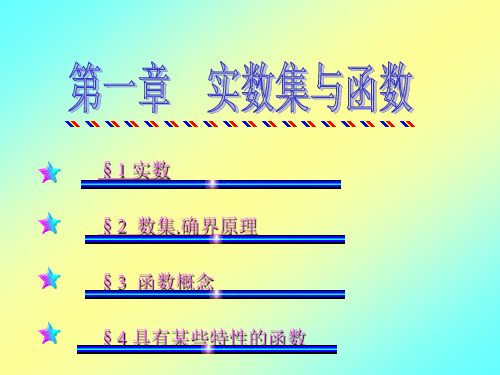
证明
由于x
<
y, 故存在非负整数n,使得x n
< yn.令r
1 2
(xn
yn
)
则r为有理数,且有x xn < r < yn y,即得x < r < y.
例2 设a,b R,证明: 若对任何正数e有a < b e ,则a b.
证明 用反证法.假若结论不成立 ,则根据实数的有序性
有a > b.令e a - b,则e为正数且a b e , 这与假设 a < b e矛盾.从而必有a b.
§3 函数概念
1.函数概念
❖定义
设数集DR, 则称映射f : D R为定义在D上的函数, 通常简记为
yf(x), xD, 其中x称为自变量, y称为因变量, D称为定义域, 记作Df, 即DfD.
说明:
记为函号了数f叙的和述记f(x方号)的便是区可, 常别以用:任前记意者号选表“取示f(的x自), 变除x量了Dx用”和或f因“外变y, 还量f(可xy)之,用x间“D的g””对来 应表、法示“则 定F”义,、而在“后D者”上表等的示,函此与数时自, 函这变数时量就应x对记理应作解的y为函g由(数x它)、值所.y确F定(x的)、函y数f(x.)
的集合, RR常记作R2.
3.实数集 ❖两个实数的大小关系
• 定义1
给定两个非负实数
x a0.a1a2 Lan L, y b0 .b1b2 Lbn L,其中a0 ,b0为非负整数, ak ,bk (k 1,2,L)为整数,0 ak 9,0 bk 9. 若有ak bk , k 1,2,L,则称x与y相等,记为x y;
称有理数xn a0.a1a2 Lan为实数x的n 位不足近似,