抗氧化剂 油脂氧化
油脂氧化原理
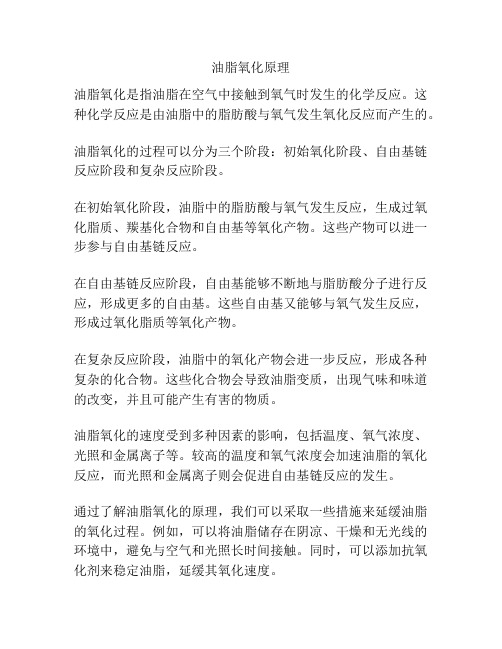
油脂氧化原理
油脂氧化是指油脂在空气中接触到氧气时发生的化学反应。
这种化学反应是由油脂中的脂肪酸与氧气发生氧化反应而产生的。
油脂氧化的过程可以分为三个阶段:初始氧化阶段、自由基链反应阶段和复杂反应阶段。
在初始氧化阶段,油脂中的脂肪酸与氧气发生反应,生成过氧化脂质、羰基化合物和自由基等氧化产物。
这些产物可以进一步参与自由基链反应。
在自由基链反应阶段,自由基能够不断地与脂肪酸分子进行反应,形成更多的自由基。
这些自由基又能够与氧气发生反应,形成过氧化脂质等氧化产物。
在复杂反应阶段,油脂中的氧化产物会进一步反应,形成各种复杂的化合物。
这些化合物会导致油脂变质,出现气味和味道的改变,并且可能产生有害的物质。
油脂氧化的速度受到多种因素的影响,包括温度、氧气浓度、光照和金属离子等。
较高的温度和氧气浓度会加速油脂的氧化反应,而光照和金属离子则会促进自由基链反应的发生。
通过了解油脂氧化的原理,我们可以采取一些措施来延缓油脂的氧化过程。
例如,可以将油脂储存在阴凉、干燥和无光线的环境中,避免与空气和光照长时间接触。
同时,可以添加抗氧化剂来稳定油脂,延缓其氧化速度。
激发油脂氧化的基本因素和抗氧化剂的基本类型
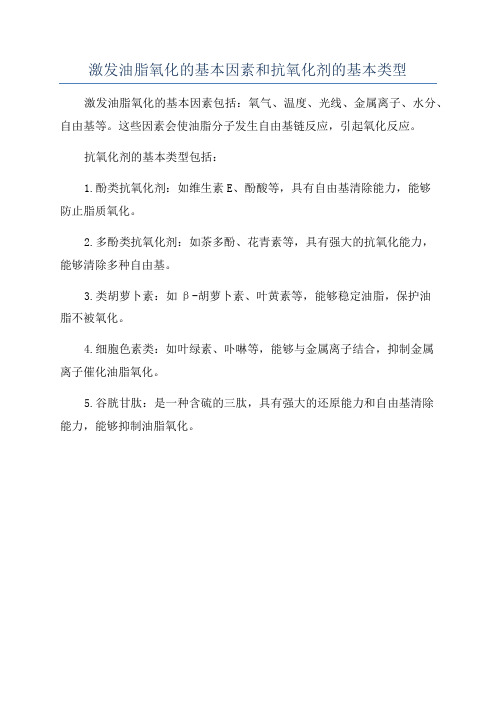
激发油脂氧化的基本因素和抗氧化剂的基本类型
激发油脂氧化的基本因素包括:氧气、温度、光线、金属离子、水分、自由基等。
这些因素会使油脂分子发生自由基链反应,引起氧化反应。
抗氧化剂的基本类型包括:
1.酚类抗氧化剂:如维生素E、酚酸等,具有自由基清除能力,能够
防止脂质氧化。
2.多酚类抗氧化剂:如茶多酚、花青素等,具有强大的抗氧化能力,
能够清除多种自由基。
3.类胡萝卜素:如β-胡萝卜素、叶黄素等,能够稳定油脂,保护油
脂不被氧化。
4.细胞色素类:如叶绿素、卟啉等,能够与金属离子结合,抑制金属
离子催化油脂氧化。
5.谷胱甘肽:是一种含硫的三肽,具有强大的还原能力和自由基清除
能力,能够抑制油脂氧化。
简述油脂氧化的机理及影响因素
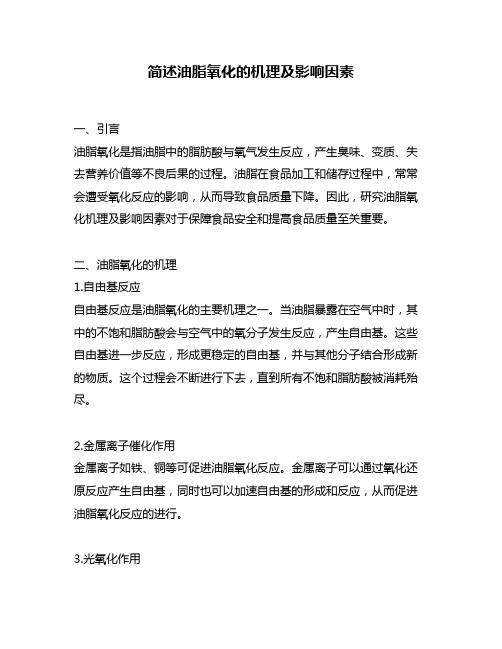
简述油脂氧化的机理及影响因素一、引言油脂氧化是指油脂中的脂肪酸与氧气发生反应,产生臭味、变质、失去营养价值等不良后果的过程。
油脂在食品加工和储存过程中,常常会遭受氧化反应的影响,从而导致食品质量下降。
因此,研究油脂氧化机理及影响因素对于保障食品安全和提高食品质量至关重要。
二、油脂氧化的机理1.自由基反应自由基反应是油脂氧化的主要机理之一。
当油脂暴露在空气中时,其中的不饱和脂肪酸会与空气中的氧分子发生反应,产生自由基。
这些自由基进一步反应,形成更稳定的自由基,并与其他分子结合形成新的物质。
这个过程会不断进行下去,直到所有不饱和脂肪酸被消耗殆尽。
2.金属离子催化作用金属离子如铁、铜等可促进油脂氧化反应。
金属离子可以通过氧化还原反应产生自由基,同时也可以加速自由基的形成和反应,从而促进油脂氧化反应的进行。
3.光氧化作用油脂暴露在光线下时,其中的不饱和脂肪酸会与空气中的氧分子发生反应,这个过程称为光氧化作用。
这个过程会导致油脂中的营养物质丢失以及产生有害物质。
三、影响油脂氧化的因素1.温度温度是影响油脂氧化的最主要因素之一。
随着温度升高,油脂中的不饱和脂肪酸分子更容易与空气中的氧分子发生反应,从而导致油脂更快地变质。
2.水分水分也会影响油脂氧化。
当油脂中含有水分时,水分会促进自由基反应,并且使金属离子催化作用更加明显。
3.金属离子金属离子是促进油脂氧化反应的重要因素之一。
铁、铜等金属离子在油脂中存在时,会加速自由基的形成和反应,从而促进油脂氧化反应的进行。
4.光照油脂暴露在光线下时,其中的不饱和脂肪酸会与空气中的氧分子发生反应,这个过程称为光氧化作用。
这个过程会导致油脂中的营养物质丢失以及产生有害物质。
5.抗氧化剂抗氧化剂可以减缓油脂氧化反应。
抗氧化剂能够与自由基结合,从而阻止它们进一步反应,并保护油脂不被氧化。
四、结论综上所述,油脂氧化是食品加工和储存过程中常见的问题。
了解油脂氧化机理及影响因素对于提高食品质量、保障食品安全具有重要意义。
第3章抗氧化剂
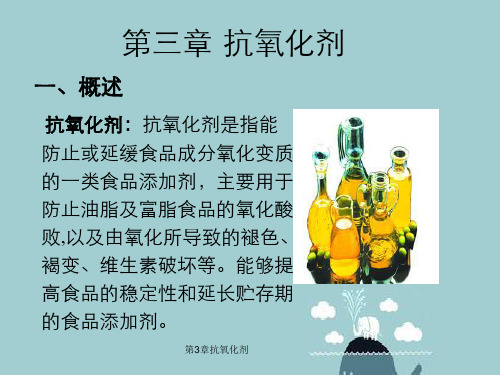
以AH代表抗氧化剂 AH+ROO·→ROOH+A· AH+ R· → RH + A· 抗氧化剂的自由基A·没有活性,它不能引
起链式反应,却能参与一些终止反应。 如:A·+ A·→AA
A·+ROO·→ROOA
第3章抗氧化剂
⑵氧清除剂 抗氧化剂极易被氧化,可与空气中的氧
首先反应,保护食品。 抗氧化剂只能阻碍氧化作用的过程,以
第三章 抗氧化剂
一、概述
抗氧化剂:抗氧化剂是指能 防止或延缓食品成分氧化变质 的一类食品添加剂,主要用于 防止油脂及富脂食品的氧化酸 败,以及由氧化所导致的褪色、 褐变、维生素破坏等。能够提 高食品的稳定性和延长贮存期 的食品添加剂。
第3章抗氧化剂
氧化剂应具备条件
具有抗氧化作用物质很多,但用于食品 的抗氧化剂应具备以下特点: ⑴安全卫生,抗氧化剂本身及分解产物 都无毒无害;便于分析检测。 ⑵对食品的感官性质不产生明显的影响, 能够与食品共存,性能稳定。 ⑶抗氧化效果良好,低浓度有效,使用 方便,价格便宜。
第3章抗氧化剂
9
R2
OOH R2
b.亚油酸氢氢过氧化合物
R1 13 11 9 R2
光、热
或金属
R1
9 R2
R1
11
R2 R1 12
R2
O2 H (增殖期)
OOH
OOH
R1
R2 R1
第3章抗氧化剂
R2
(二)激发油脂氧化的因素
一些二价或多价,如Cu 2+、Zn2+、 Fe3+、Fe2+、Al3+、Pb2+等的金属离 子常可促进油脂氧化反应的进行,称这 些金属离子为助氧化剂。金属离子在油 脂氧化中通过下面三种方式发挥促进的 作用:
油脂的氧化酸败名词解释
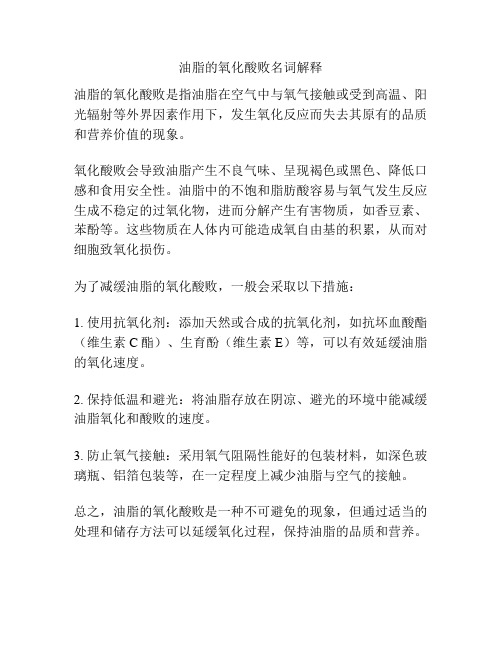
油脂的氧化酸败名词解释
油脂的氧化酸败是指油脂在空气中与氧气接触或受到高温、阳光辐射等外界因素作用下,发生氧化反应而失去其原有的品质和营养价值的现象。
氧化酸败会导致油脂产生不良气味、呈现褐色或黑色、降低口感和食用安全性。
油脂中的不饱和脂肪酸容易与氧气发生反应生成不稳定的过氧化物,进而分解产生有害物质,如香豆素、苯酚等。
这些物质在人体内可能造成氧自由基的积累,从而对细胞致氧化损伤。
为了减缓油脂的氧化酸败,一般会采取以下措施:
1. 使用抗氧化剂:添加天然或合成的抗氧化剂,如抗坏血酸酯(维生素C酯)、生育酚(维生素E)等,可以有效延缓油脂的氧化速度。
2. 保持低温和避光:将油脂存放在阴凉、避光的环境中能减缓油脂氧化和酸败的速度。
3. 防止氧气接触:采用氧气阻隔性能好的包装材料,如深色玻璃瓶、铝箔包装等,在一定程度上减少油脂与空气的接触。
总之,油脂的氧化酸败是一种不可避免的现象,但通过适当的处理和储存方法可以延缓氧化过程,保持油脂的品质和营养。
油脂的氧化及其抗氧化措施
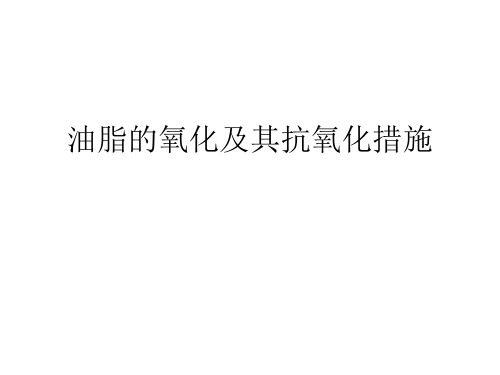
3.3 温度
温度的上升会促进氧化。油脂在100℃以下, 温度每上升10℃ 10℃, 温度的上升会促进氧化。油脂在100℃以下, 温度每上升10℃, 100℃以下 氧化速度便提高1 对动植物性的起酥油试验, 97. 氧化速度便提高1 倍。对动植物性的起酥油试验, 在97.8~110℃ 的平均值下, 温度每上升9℃ 氧化速度增加1 9℃, 的平均值下, 温度每上升9℃,氧化速度增加1 倍, 植物性起酥油 贮藏时21 63℃, 温度每上升16℃ 氧化速度增加1 倍等等。 21~ 16℃, 贮藏时21~63℃, 温度每上升16℃, 氧化速度增加1 倍等等。经 测定, 油脂在110℃时的氧化速度是97℃时的215 110℃时的氧化速度是97℃时的 测定, 油脂在110℃时的氧化速度是97℃时的215 倍。
油脂的氧化及其抗氧化措施
• • • • •
1.油脂 2.油脂的氧化机理 3.影响油脂氧化的因素 4.油脂氧化机理研究的几个核心问题 5.油脂氧化的抗氧化剂——TBHQ
1.油脂 油是不饱和高级脂肪酸甘油酯,脂肪是饱和高级脂肪酸甘油 油脂: 油脂
酯,都是高级脂肪酸甘油酯,是一种有机物。植物油在常温常压下一般 为液态,称为油,而动物脂肪在常温常压下为固态,称为脂,二者合称 为油脂。油脂均为混合物,无固定的熔沸点。油脂不但是人类的主要营 养物质和主要食物之一,也是一种重要的工业原料。 油脂中的碳链含碳碳双键 含碳碳双键时(即为不饱和脂肪酸甘油酯),主要 含碳碳双键 是低沸点的植物油;油脂中的碳链为碳碳单键 为碳碳单键时(即为饱和脂肪酸甘油 为碳碳单键 酯),主要是高沸点的动物脂肪。 • 油脂结构
2.1油烯底物(如不饱和油 活化的含烯底物( 活化的含烯底物 和空气中的氧在室温下,未经任何直接光照 未经任何直接光照,未 脂)和空气中的氧在室温下 未经任何直接光照 未 加任何催化剂等条件下的完全自发的氧化反应。 加任何催化剂等条件下的完全自发的氧化反应。 自动氧化是一个自由基连锁反应,它一般是按游 离基反应的机理进行的。油脂的变质,绝大部分 是由于脂类的自动氧化造成的。 • 油脂自动氧化三个过程:诱导 发展 终止。 诱导 终止
不同浓度抗氧化剂对油脂氧化的影响研究
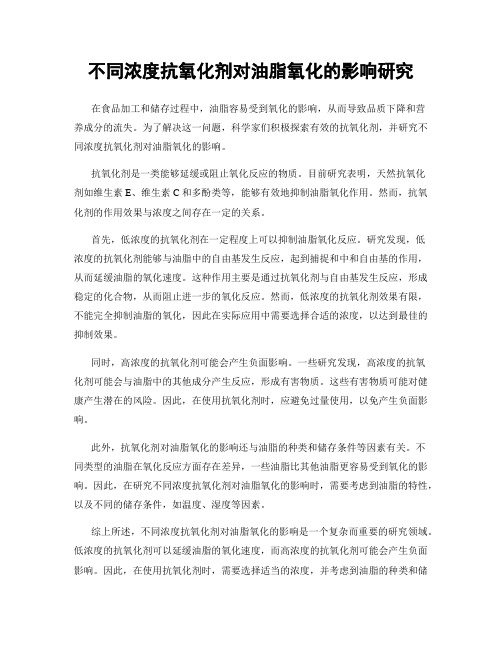
不同浓度抗氧化剂对油脂氧化的影响研究在食品加工和储存过程中,油脂容易受到氧化的影响,从而导致品质下降和营养成分的流失。
为了解决这一问题,科学家们积极探索有效的抗氧化剂,并研究不同浓度抗氧化剂对油脂氧化的影响。
抗氧化剂是一类能够延缓或阻止氧化反应的物质。
目前研究表明,天然抗氧化剂如维生素E、维生素C和多酚类等,能够有效地抑制油脂氧化作用。
然而,抗氧化剂的作用效果与浓度之间存在一定的关系。
首先,低浓度的抗氧化剂在一定程度上可以抑制油脂氧化反应。
研究发现,低浓度的抗氧化剂能够与油脂中的自由基发生反应,起到捕捉和中和自由基的作用,从而延缓油脂的氧化速度。
这种作用主要是通过抗氧化剂与自由基发生反应,形成稳定的化合物,从而阻止进一步的氧化反应。
然而,低浓度的抗氧化剂效果有限,不能完全抑制油脂的氧化,因此在实际应用中需要选择合适的浓度,以达到最佳的抑制效果。
同时,高浓度的抗氧化剂可能会产生负面影响。
一些研究发现,高浓度的抗氧化剂可能会与油脂中的其他成分产生反应,形成有害物质。
这些有害物质可能对健康产生潜在的风险。
因此,在使用抗氧化剂时,应避免过量使用,以免产生负面影响。
此外,抗氧化剂对油脂氧化的影响还与油脂的种类和储存条件等因素有关。
不同类型的油脂在氧化反应方面存在差异,一些油脂比其他油脂更容易受到氧化的影响。
因此,在研究不同浓度抗氧化剂对油脂氧化的影响时,需要考虑到油脂的特性,以及不同的储存条件,如温度、湿度等因素。
综上所述,不同浓度抗氧化剂对油脂氧化的影响是一个复杂而重要的研究领域。
低浓度的抗氧化剂可以延缓油脂的氧化速度,而高浓度的抗氧化剂可能会产生负面影响。
因此,在使用抗氧化剂时,需要选择适当的浓度,并考虑到油脂的种类和储存条件,以达到最佳的抑制效果。
未来的研究可以进一步深入探讨不同类型抗氧化剂和油脂的相互作用机制,以实现更好的抗氧化效果。
油脂自动氧化的机制及其控制油脂氧化是油脂及油基食品败坏
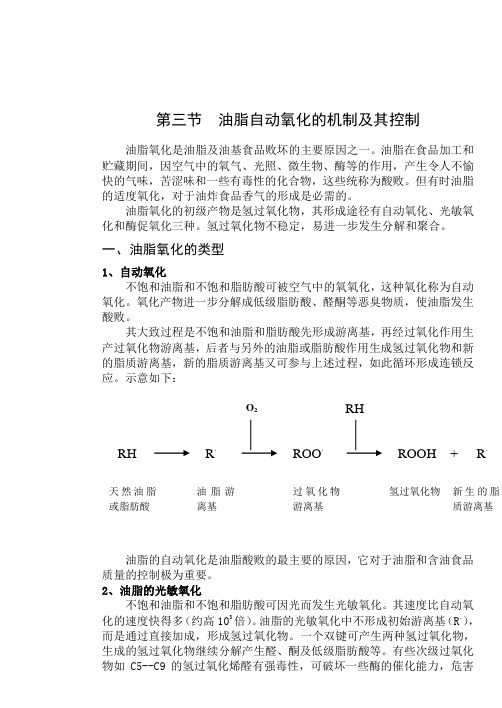
第三节 油脂自动氧化的机制及其控制油脂氧化是油脂及油基食品败坏的主要原因之一。
油脂在食品加工和贮藏期间,因空气中的氧气、光照、微生物、酶等的作用,产生令人不愉快的气味,苦涩味和一些有毒性的化合物,这些统称为酸败。
但有时油脂的适度氧化,对于油炸食品香气的形成是必需的。
油脂氧化的初级产物是氢过氧化物,其形成途径有自动氧化、光敏氧化和酶促氧化三种。
氢过氧化物不稳定,易进一步发生分解和聚合。
一、油脂氧化的类型1、自动氧化不饱和油脂和不饱和脂肪酸可被空气中的氧氧化,这种氧化称为自动氧化。
氧化产物进一步分解成低级脂肪酸、醛酮等恶臭物质,使油脂发生酸败。
其大致过程是不饱和油脂和脂肪酸先形成游离基,再经过氧化作用生产过氧化物游离基,后者与另外的油脂或脂肪酸作用生成氢过氧化物和新的脂质游离基,新的脂质游离基又可参与上述过程,如此循环形成连锁反应。
示意如下:油脂的自动氧化是油脂酸败的最主要的原因,它对于油脂和含油食品质量的控制极为重要。
2、油脂的光敏氧化不饱和油脂和不饱和脂肪酸可因光而发生光敏氧化。
其速度比自动氧化的速度快得多(约高103倍)。
油脂的光敏氧化中不形成初始游离基(R .),而是通过直接加成,形成氢过氧化物。
一个双键可产生两种氢过氧化物,生成的氢过氧化物继续分解产生醛、酮及低级脂肪酸等。
有些次级过氧化物如C5--C9的氢过氧化烯醛有强毒性,可破坏一些酶的催化能力,危害RH R .ROOH 天然油脂或脂肪酸 油脂游离基 过氧化物游离基 氢过氧化物 R . + 新生的脂质游离基性极大。
3、酶促氧化脂肪在酶参与下发生的氧化反应,称为酶促氧化。
油脂在酶的作用下氧化产生的中间产物也是一些氢过氧化物。
以上各种途径生成的氢过氧化物均不稳定,当体系中的浓度增至一定程度时,就开始分解。
可能发生的反应之一是氢过氧化物单分子分解为一个烷氧基和一个羟基游离基,烷氧基游离基的进一步反应生成醛、醇或酮等。
醛、醇或酮等这些小分子具有令人不愉快的气味即哈喇味,导致油脂酸败。
食用油抗氧化剂
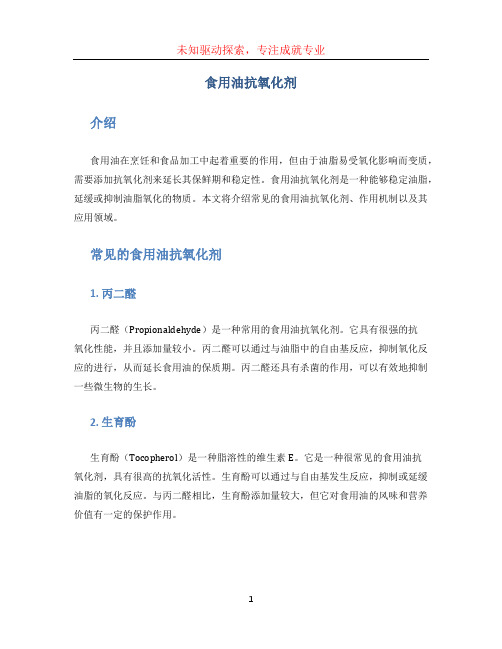
食用油抗氧化剂介绍食用油在烹饪和食品加工中起着重要的作用,但由于油脂易受氧化影响而变质,需要添加抗氧化剂来延长其保鲜期和稳定性。
食用油抗氧化剂是一种能够稳定油脂,延缓或抑制油脂氧化的物质。
本文将介绍常见的食用油抗氧化剂、作用机制以及其应用领域。
常见的食用油抗氧化剂1. 丙二醛丙二醛(Propionaldehyde)是一种常用的食用油抗氧化剂。
它具有很强的抗氧化性能,并且添加量较小。
丙二醛可以通过与油脂中的自由基反应,抑制氧化反应的进行,从而延长食用油的保质期。
丙二醛还具有杀菌的作用,可以有效地抑制一些微生物的生长。
2. 生育酚生育酚(Tocopherol)是一种脂溶性的维生素E。
它是一种很常见的食用油抗氧化剂,具有很高的抗氧化活性。
生育酚可以通过与自由基发生反应,抑制或延缓油脂的氧化反应。
与丙二醛相比,生育酚添加量较大,但它对食用油的风味和营养价值有一定的保护作用。
3. 亚硫酸氢钠亚硫酸氢钠(Sodium bisulfite)是一种无色结晶,具有很强的抗氧化性能。
亚硫酸氢钠可以与氧气中的自由基发生反应,抑制氧化反应的进行。
此外,亚硫酸氢钠还具有阻断有机物质氧化的作用,可以保持食用油的色泽和风味。
抗氧化剂的作用机制食用油抗氧化剂可以通过多种途径发挥其抗氧化的作用。
下面是一些常见的作用机制:1. 自由基捕捉抗氧化剂能够与油脂中的自由基发生反应,从而有效地抑制或延缓氧化反应的进行。
自由基是引起油脂氧化的主要原因之一,抗氧化剂通过与自由基结合,降低其活性,从而保护油脂的稳定性。
2. 金属离子螯合一些抗氧化剂具有与金属离子结合的能力,形成稳定的络合物。
金属离子可以促进油脂的氧化反应,而抗氧化剂通过螯合金属离子,阻断其参与氧化反应,从而延缓油脂的氧化进程。
3. 阻断链反应氧化反应通常是一个自由基链反应过程。
抗氧化剂可以通过与链反应中的自由基反应,从而阻断氧化反应的进行。
通过阻断链反应,抗氧化剂可以有效地延缓油脂的氧化进程。
油脂氧化的原理

油脂氧化的原理油脂氧化是指由于油脂与空气中的氧发生反应而引起的化学变化。
油脂氧化是一个复杂的过程,涉及多种反应和产物的生成。
下面将详细介绍油脂氧化的原理。
油脂是由甘油和脂肪酸通过酯化反应形成的化合物,其主要成分是三酸甘油酯。
这种三酸甘油酯是由三个脂肪酸分子与一个甘油分子通过酯键结合而成的。
由于甘油酯的分子结构中含有大量的不饱和脂肪酸,使得油脂易受氧化影响。
油脂氧化反应是一种复杂的过程,主要包括自由基链反应、自氧化反应和多种氧化产物的生成。
自由基链反应是油脂氧化的主要反应途径。
当油脂与空气中的氧发生接触时,油脂中的不饱和脂肪酸发生氧化反应,产生自由基。
这些自由基接着与其他不饱和脂肪酸分子反应,形成新的自由基,从而引发自由基链反应。
自氧化反应是指油脂中的不饱和脂肪酸与氧发生直接反应,而不需要通过自由基中间体。
这种反应是较慢的反应途径,但在存在一定条件下会起到重要作用。
例如,当油脂中的氧分压升高时,自氧化反应的速率会显著增加。
在油脂氧化过程中,会生成多种氧化产物。
其中,主要包括醛、酮、酸、过氧化物、羟基化合物等。
醛和酮是由于不饱和脂肪酸氧化产生的产物,具有较强的挥发性和刺激性。
酸是由于不饱和脂肪酸发生氧化反应形成的,会降低油脂的酸值。
过氧化物是由于不饱和脂肪酸与氧直接反应形成的,具有较强的氧化性和活性。
羟基化合物是由于油脂中的不饱和脂肪酸与氧发生反应,形成的具有亲水性的产物。
油脂氧化的速率受到多种因素的影响。
其中最重要的是温度、氧分压、水分、金属离子和抗氧化剂等。
温度是影响油脂氧化速率的关键因素,温度升高会加速油脂的氧化反应。
氧分压是影响油脂氧化速率的另一个重要因素,氧分压升高会促进氧与油脂中的不饱和脂肪酸发生反应。
水分是影响油脂氧化速率的关键因素之一,水分的存在会加速油脂的氧化反应。
金属离子是影响油脂氧化速率的关键因素之一,金属离子会促进自由基反应的发生。
抗氧化剂能够抑制油脂的氧化反应,延缓油脂的老化。
油脂氧化酸败的名词解释
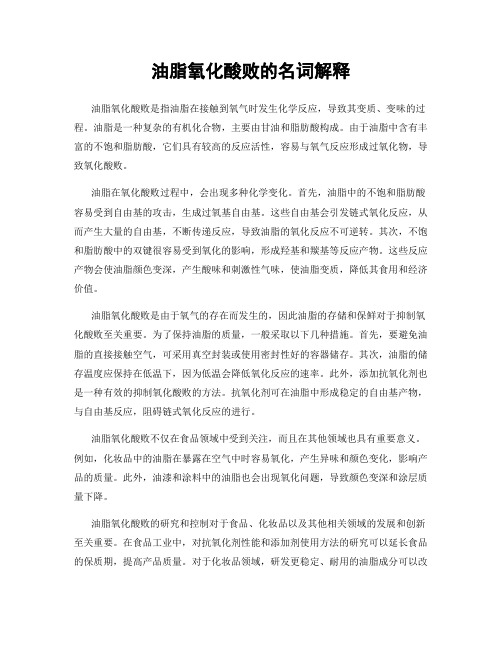
油脂氧化酸败的名词解释油脂氧化酸败是指油脂在接触到氧气时发生化学反应,导致其变质、变味的过程。
油脂是一种复杂的有机化合物,主要由甘油和脂肪酸构成。
由于油脂中含有丰富的不饱和脂肪酸,它们具有较高的反应活性,容易与氧气反应形成过氧化物,导致氧化酸败。
油脂在氧化酸败过程中,会出现多种化学变化。
首先,油脂中的不饱和脂肪酸容易受到自由基的攻击,生成过氧基自由基。
这些自由基会引发链式氧化反应,从而产生大量的自由基,不断传递反应,导致油脂的氧化反应不可逆转。
其次,不饱和脂肪酸中的双键很容易受到氧化的影响,形成羟基和羰基等反应产物。
这些反应产物会使油脂颜色变深,产生酸味和刺激性气味,使油脂变质,降低其食用和经济价值。
油脂氧化酸败是由于氧气的存在而发生的,因此油脂的存储和保鲜对于抑制氧化酸败至关重要。
为了保持油脂的质量,一般采取以下几种措施。
首先,要避免油脂的直接接触空气,可采用真空封装或使用密封性好的容器储存。
其次,油脂的储存温度应保持在低温下,因为低温会降低氧化反应的速率。
此外,添加抗氧化剂也是一种有效的抑制氧化酸败的方法。
抗氧化剂可在油脂中形成稳定的自由基产物,与自由基反应,阻碍链式氧化反应的进行。
油脂氧化酸败不仅在食品领域中受到关注,而且在其他领域也具有重要意义。
例如,化妆品中的油脂在暴露在空气中时容易氧化,产生异味和颜色变化,影响产品的质量。
此外,油漆和涂料中的油脂也会出现氧化问题,导致颜色变深和涂层质量下降。
油脂氧化酸败的研究和控制对于食品、化妆品以及其他相关领域的发展和创新至关重要。
在食品工业中,对抗氧化剂性能和添加剂使用方法的研究可以延长食品的保质期,提高产品质量。
对于化妆品领域,研发更稳定、耐用的油脂成分可以改善产品的使用寿命和品质。
此外,对于其他领域如医药、农业等,研究油脂氧化酸败的机理和控制方法,有助于推动相关产品的创新和发展。
总结而言,油脂氧化酸败是指油脂在与氧气接触时发生的化学反应,导致油脂变质、变味的过程。
食品中油脂氧化与抗氧化机制的研究
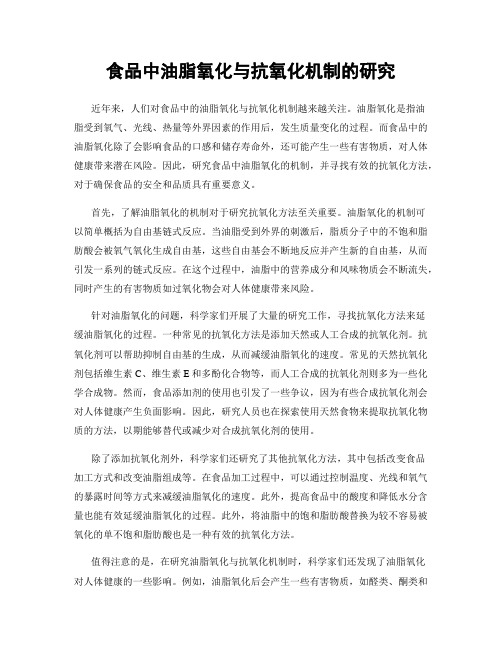
食品中油脂氧化与抗氧化机制的研究近年来,人们对食品中的油脂氧化与抗氧化机制越来越关注。
油脂氧化是指油脂受到氧气、光线、热量等外界因素的作用后,发生质量变化的过程。
而食品中的油脂氧化除了会影响食品的口感和储存寿命外,还可能产生一些有害物质,对人体健康带来潜在风险。
因此,研究食品中油脂氧化的机制,并寻找有效的抗氧化方法,对于确保食品的安全和品质具有重要意义。
首先,了解油脂氧化的机制对于研究抗氧化方法至关重要。
油脂氧化的机制可以简单概括为自由基链式反应。
当油脂受到外界的刺激后,脂质分子中的不饱和脂肪酸会被氧气氧化生成自由基,这些自由基会不断地反应并产生新的自由基,从而引发一系列的链式反应。
在这个过程中,油脂中的营养成分和风味物质会不断流失,同时产生的有害物质如过氧化物会对人体健康带来风险。
针对油脂氧化的问题,科学家们开展了大量的研究工作,寻找抗氧化方法来延缓油脂氧化的过程。
一种常见的抗氧化方法是添加天然或人工合成的抗氧化剂。
抗氧化剂可以帮助抑制自由基的生成,从而减缓油脂氧化的速度。
常见的天然抗氧化剂包括维生素C、维生素E和多酚化合物等,而人工合成的抗氧化剂则多为一些化学合成物。
然而,食品添加剂的使用也引发了一些争议,因为有些合成抗氧化剂会对人体健康产生负面影响。
因此,研究人员也在探索使用天然食物来提取抗氧化物质的方法,以期能够替代或减少对合成抗氧化剂的使用。
除了添加抗氧化剂外,科学家们还研究了其他抗氧化方法,其中包括改变食品加工方式和改变油脂组成等。
在食品加工过程中,可以通过控制温度、光线和氧气的暴露时间等方式来减缓油脂氧化的速度。
此外,提高食品中的酸度和降低水分含量也能有效延缓油脂氧化的过程。
此外,将油脂中的饱和脂肪酸替换为较不容易被氧化的单不饱和脂肪酸也是一种有效的抗氧化方法。
值得注意的是,在研究油脂氧化与抗氧化机制时,科学家们还发现了油脂氧化对人体健康的一些影响。
例如,油脂氧化后会产生一些有害物质,如醛类、酮类和羟基脂肪酸等。
油脂自动氧化的机制及其控制

油脂自动氧化的机制及其控制油脂是一种由脂肪酸和甘油酯构成的混合物,常见于食品和化妆品中。
油脂自动氧化是指在空气中或储存时,油脂会与氧气接触并发生氧化反应的过程。
这一过程会导致油脂的氧化质量下降,出现氧化变质、变色、变味等现象。
因此,控制油脂的自动氧化对于保持其质量和延长其使用寿命非常重要。
第一步是引发反应,即活性氧的生成。
油脂中存在的活性氧可通过多种途径生成,如自由基引发的氧化、光氧化、酶催化等。
其中,自由基引发的氧化是最为常见的机制。
当油脂中存在氧气和自由基时,它们会发生反应生成过氧化物自由基,这是引发油脂氧化的关键步骤。
第二步是传递反应,即自由基与其他油脂分子发生反应。
在这个过程中,自由基会攫取其他分子的氢原子,产生不稳定的自由基中间体。
这一过程会形成新的自由基,并导致氧化反应的连锁增加。
第三步是扩散反应,即氧化产物在油脂中的扩散和演化。
在氧化反应进行的过程中,大量的氧化产物会逐渐形成,如过氧化脂质、醛类、酮类等。
这些氧化产物会导致油脂质量下降,引起氧化变质的现象。
为了控制油脂的自动氧化,可以采取以下几种措施:1.阻断引发反应。
通过添加抗氧化剂来阻止自由基的生成,减少氧化反应的开始。
常见的抗氧化剂有硫酸盐类、亚硫酸盐类和合成抗氧化剂等。
抗氧化剂可以中和自由基,降低其活性。
2.阻止传递反应。
通过控制油脂中的过氧化物自由基的生成和消除来减少自由基的数量,以防止连锁反应的进行。
这可以通过添加金属螯合剂、活性吸附剂等来达到目的。
3.阻碍扩散反应。
通过控制氧气的接触、降低温度、减少光照等方式来降低氧化反应的速率。
此外,包装油脂时可以选择透氧性较低的包装材料,减少氧气的进入油脂中。
4.使用抗氧化性能良好的油脂。
一些油脂本身具有较好的抗氧化性能,如鳄梨油、橄榄油等。
选择这些油脂作为原料可以降低油脂自动氧化的发生率。
综上所述,控制油脂自动氧化的机制主要涉及引发反应、传递反应和扩散反应三个步骤。
通过选择适当的抗氧化剂、控制氧气接触和温度等方式可以有效降低油脂自动氧化的发生率,延长油脂的使用寿命。
油脂的氧化及其抗氧化措施
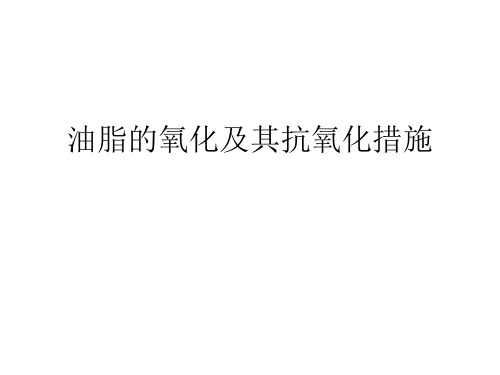
R·+O2→ROO· ROO·+RH→ROOH+ R· ROOH→RO·+·OH 2ROOH→R·+ ROO·+H2O RO·+RH→ROH+ R· ·OH+ RH→ROH+ R·
3.4 水分活度(Aw)
油脂食品中少量的水分活度(Aw<0.2) 被认为有益于油脂的稳定 性, 油脂从极低的水分活度Aw 开始氧化速度随着水分的增加而降低, 这是由于这部分水能与脂肪氧化的自由基反应中的氢过氧化物形成氢键, 氢键可以保护氢过氧化物的分解, 因此可以降低过氧化物分解时的初 速度同时, 催化氧化作用的微量金属水合后降低了氧化活性, 从而阻 碍了氧化的进行。但当水分活度不断增大(0.2<Aw<0.8), 增加 的水分增加了氧的溶解度,也使脂肪大分子肿胀, 暴露了更多的氧化 部位, 加速了氧化。当水分活度继续增大(Aw>0.8) 后, 水对催 化剂的稀释降低了催化效力和反应物浓度, 氧化速度降低。
二次产物的形成:分解和进一步聚合反应在进行,形成低分子 产物如醛、酮、酸、醇和高分子化合物。氧化酸败划分的这几 个阶段并无绝对界线,只不过在某一阶段,以哪个反应为主,在 其量上哪个反应占优势。
2.1油脂的光氧化
光氧化作用也是油脂氧化作用的组成部分。氧分子有两种存在能量 状态,一是单线态,即激发态氧分子(1O2);另一是三线基态氧分子 (3O2)。油脂中的色素会强烈吸收邻近的可见光或紫外光,发生光氧 化作用,这也包括有光敏剂存在下与氧的反应所引起的氧化反应
油脂氧化与抗氧化
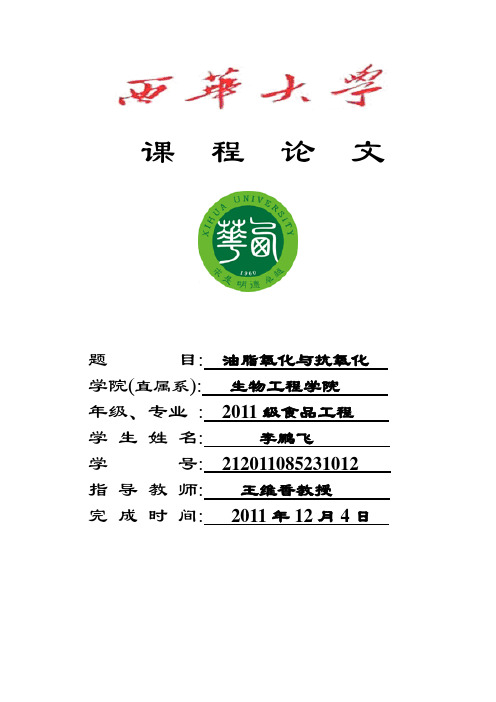
课程论文题目:油脂氧化与抗氧化学院(直属系):生物工程学院年级、专业:2011级食品工程学生姓名:李鹏飞学号:212011085231012 指导教师:王维香教授完成时间:2011年12月4日目录1 油脂的氧化的机理 (1)1.1油脂的自动氧化 (1)1.1.1 自动氧化 (1)1.1.2 自动氧化的特征 (1)1.1.3 自动氧化的过程 (1)1.2影响油脂氧化速率的因素 (5)1.3重要脂类氧化的测定方法 (7)1.3.1过氧化值 (7)1.3.2硫代巴比妥酸值(TBA) (7)1.3.3活性氧法(AOM) (7)1.3.4史卡尔(Schaal)温箱实验 (8)1.3.5 色谱法 (8)1.3.6感官评定 (8)2 脂类的抗氧化(ANTI-OXIDANT) (8)2.1脂类抗氧化机理 (8)2.2影响抗氧化剂抗氧化效果的因素 (9)2.3主要抗氧化剂 (9)2.4 抗氧化的增效作用 (12)2.5抗氧化剂的选择 (12)3体会 (13)脂类自动氧化与抗氧化摘要:食品在加工、储存以及精制过程中,脂类发生了复杂的化学变化,产生许多新的化合物,有的可改善食品品质,但有的则生成有害的物质,对食品的色泽、风味、营养价值产生不良影响。
脂类的有自动氧化,热氧化,酶促氧化等,本文以油脂的自动氧化的机理和实例解析为基础,探讨脂类的抗氧化工艺。
1油脂的氧化的机理1.1 油脂的自动氧化1.1.1 自动氧化自动氧化作用是脂类分子与氧分子之间的反应,是脂类氧化变质的主要原因。
脂类的自动氧化是一个自由基连锁反应,诱导期中启动自由基的诱发剂可能是脂氧酶、光氧化,但多数为过渡金属离子。
氧化酸败的过程通常可分为四个阶段。
油脂的诱导期是油脂氧化稳定性的标志,影响脂类氧化速度的因素很多,主要是抗氧化剂、金属及脂类本身的不饱和度,抗氧化剂的加入能延长脂类的诱导期。
1.1.2 自动氧化的特征大量证据表明,脂类的自动氧化是典型的游离基反应历程,凡是能干扰游离基反应的化学物质,都将具有明显的抗氧化作用,延缓氧化反应的速度;光和产生游离基的物质对反应起催化作用,氢过氧化物ROOH产率高;光引发氧化反应时量子产率超过1;纯底物时,有较长的诱导期。
油脂氧化osi
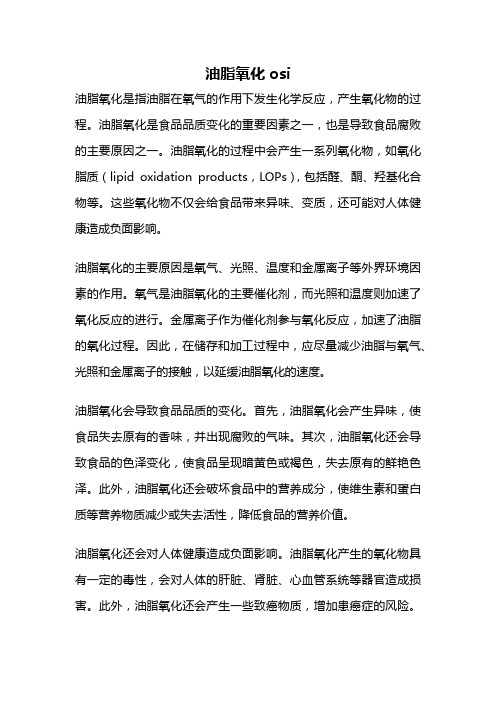
油脂氧化osi油脂氧化是指油脂在氧气的作用下发生化学反应,产生氧化物的过程。
油脂氧化是食品品质变化的重要因素之一,也是导致食品腐败的主要原因之一。
油脂氧化的过程中会产生一系列氧化物,如氧化脂质(lipid oxidation products,LOPs),包括醛、酮、羟基化合物等。
这些氧化物不仅会给食品带来异味、变质,还可能对人体健康造成负面影响。
油脂氧化的主要原因是氧气、光照、温度和金属离子等外界环境因素的作用。
氧气是油脂氧化的主要催化剂,而光照和温度则加速了氧化反应的进行。
金属离子作为催化剂参与氧化反应,加速了油脂的氧化过程。
因此,在储存和加工过程中,应尽量减少油脂与氧气、光照和金属离子的接触,以延缓油脂氧化的速度。
油脂氧化会导致食品品质的变化。
首先,油脂氧化会产生异味,使食品失去原有的香味,并出现腐败的气味。
其次,油脂氧化还会导致食品的色泽变化,使食品呈现暗黄色或褐色,失去原有的鲜艳色泽。
此外,油脂氧化还会破坏食品中的营养成分,使维生素和蛋白质等营养物质减少或失去活性,降低食品的营养价值。
油脂氧化还会对人体健康造成负面影响。
油脂氧化产生的氧化物具有一定的毒性,会对人体的肝脏、肾脏、心血管系统等器官造成损害。
此外,油脂氧化还会产生一些致癌物质,增加患癌症的风险。
因此,食用含有氧化油脂的食品可能会对人体健康产生不良影响。
为了延缓油脂氧化的速度,可以采取一些措施。
首先,储存油脂时应尽量避免阳光直射,以减少光照对油脂的影响。
其次,应尽量减少油脂与空气接触的时间和表面积,可以使用密封容器储存油脂,避免油脂暴露在空气中。
此外,还可以通过添加抗氧化剂来延缓油脂氧化的速度。
常用的抗氧化剂有维生素E、维生素C、硫代硫酸钠等。
这些抗氧化剂可以捕捉自由基,阻止油脂氧化反应的进行。
油脂氧化是食品品质变化的重要因素之一,也是导致食品腐败的主要原因之一。
油脂氧化会导致食品出现异味、变质,降低食品的色泽和营养价值。
此外,油脂氧化还会对人体健康造成负面影响。
油脂氧化osi

油脂氧化osi油脂氧化(Oxidative Stability Index, OSI)是一种用于评估油脂抗氧化性能的指标。
在食品加工和储存中,油脂容易受到氧化的影响,从而导致食品品质下降甚至变质。
因此,对油脂的抗氧化性能进行评估和监测具有重要意义。
油脂氧化是指油脂中的脂肪酸与氧发生反应,产生过氧化物等氧化产物的过程。
氧化不仅会使油脂的口感、香气和色泽发生变化,还会导致有害物质的产生,对人体健康造成潜在威胁。
因此,了解油脂的氧化稳定性十分重要。
油脂的氧化稳定性可以通过测定其氧化指数来评估。
氧化指数是指油脂在特定条件下开始发生氧化反应所需的时间。
而油脂氧化指数(OSI)则是一种常用的评估油脂抗氧化性能的方法。
为了测定油脂的OSI,通常采用的是Rancimat法。
该方法通过将油脂样品加热至一定温度,然后以一定速率通入空气,通过测定样品中氧化产物的生成速率来计算OSI值。
OSI值越高,表示油脂的抗氧化性能越好。
油脂的氧化过程是一个复杂的化学反应过程。
在油脂中,主要存在的是不饱和脂肪酸,它们容易受到氧化的攻击。
氧化反应的过程中,自由基的生成是一个重要的步骤。
自由基是一种高度活跃的化学物质,它们会与油脂中的不饱和脂肪酸发生反应,产生过氧化物等氧化产物。
为了延缓油脂的氧化过程,可以采取一系列的措施。
首先,要尽量减少油脂与空气的接触,避免氧化反应的发生。
其次,可以添加抗氧化剂来抑制氧化反应的进行。
常见的抗氧化剂有维生素E、维生素C等。
此外,还可以通过调节油脂的酸度、水分含量等来提高其氧化稳定性。
油脂氧化对食品的品质和口感有着重要影响。
当油脂发生氧化反应时,会产生一系列的挥发性物质和有机酸,导致食品变质。
此外,氧化反应还会导致食品中的营养物质的损失,进一步降低食品的营养价值。
对于食品加工和储存过程中的油脂选择,了解其氧化稳定性是非常重要的。
通过测定油脂的OSI值,可以评估其抗氧化性能,为食品加工和储存提供科学依据。
油脂化学性质
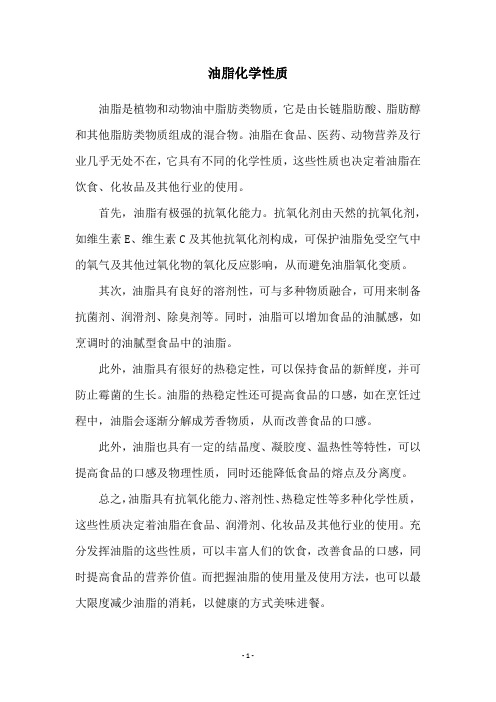
油脂化学性质
油脂是植物和动物油中脂肪类物质,它是由长链脂肪酸、脂肪醇和其他脂肪类物质组成的混合物。
油脂在食品、医药、动物营养及行业几乎无处不在,它具有不同的化学性质,这些性质也决定着油脂在饮食、化妆品及其他行业的使用。
首先,油脂有极强的抗氧化能力。
抗氧化剂由天然的抗氧化剂,如维生素E、维生素C及其他抗氧化剂构成,可保护油脂免受空气中的氧气及其他过氧化物的氧化反应影响,从而避免油脂氧化变质。
其次,油脂具有良好的溶剂性,可与多种物质融合,可用来制备抗菌剂、润滑剂、除臭剂等。
同时,油脂可以增加食品的油腻感,如烹调时的油腻型食品中的油脂。
此外,油脂具有很好的热稳定性,可以保持食品的新鲜度,并可防止霉菌的生长。
油脂的热稳定性还可提高食品的口感,如在烹饪过程中,油脂会逐渐分解成芳香物质,从而改善食品的口感。
此外,油脂也具有一定的结晶度、凝胶度、温热性等特性,可以提高食品的口感及物理性质,同时还能降低食品的熔点及分离度。
总之,油脂具有抗氧化能力、溶剂性、热稳定性等多种化学性质,这些性质决定着油脂在食品、润滑剂、化妆品及其他行业的使用。
充分发挥油脂的这些性质,可以丰富人们的饮食,改善食品的口感,同时提高食品的营养价值。
而把握油脂的使用量及使用方法,也可以最大限度减少油脂的消耗,以健康的方式美味进餐。
- 1 -。
- 1、下载文档前请自行甄别文档内容的完整性,平台不提供额外的编辑、内容补充、找答案等附加服务。
- 2、"仅部分预览"的文档,不可在线预览部分如存在完整性等问题,可反馈申请退款(可完整预览的文档不适用该条件!)。
- 3、如文档侵犯您的权益,请联系客服反馈,我们会尽快为您处理(人工客服工作时间:9:00-18:30)。
ORIGINAL PAPERInfluence of Polydimethylsiloxane on the Formationof 4-Hydroxynonenal in Soybean Oil at Frying TemperatureJose A.Gerde •Earl G.Hammond •Pamela J.WhiteReceived:14September 2010/Revised:7February 2011/Accepted:15February 2011/Published online:5April 2011ÓAOCS 2011Abstract Soybean oils treated with 25or 100ppb poly-dimethylsiloxane (PDMS)and a control with no PDMS were heated at 180°C for 48h.The decomposition of linoleate and tocopherols was monitored,as well as the changes in concentration of 4-hydroxynonenal (HNE).HNE was rap-idly formed at the beginning of the heating period in the control and in the oil containing 25ppb PDMS.HNE reached a maximum concentration of 0.033l mol/g oil and then began to slowly decline.The maximum HNE concen-tration was attained at 18h in the control oil compared with 28h in the oil with 25-ppb PDMS.In the oil with 100-ppb PDMS the HNE increased very slowly during the first 32h after which the HNE increased faster,reaching 0.033l mol/g oil at 46h and showed no decline during the 48h heating period.Thus,PDMS had a beneficial effect in preventing the formation of the toxic HNE.Keywords 4-Hydroxynonenal ÁPolydimethylsiloxane ÁSoybean oil ÁFrying ÁTocopherolIntroductionDuring frying,oils are exposed to oxygen and high tem-peratures.These stressful conditions are especially criticalfor frying oils such as soybean oil that are rich in poly-unsaturated esters.Polyunsaturated fatty acid oxidation products include aldehydes,ketones,alcohols and hydro-carbons.Linoleic acid,a fatty acid making up about 50%of ordinary soybean oil,can be degraded during frying to 4-hydroxy-2-(E )-nonenal (HNE)[1].The physiological role of HNE has been studied extensively [2].It can react spontaneously with glutathione (GSH)[2],which is abun-dant in the cells of the gastrointestinal tract where it serves as a defense against oxidative stress.This HNE-mediated reduction of GSH concentration may impair the body’s defenses against oxidative compounds [3].DNA and pro-tein synthesis also have been reported to be affected by HNE at concentrations between 1and 50l M [4].Polydimethylsiloxane (PDMS)is a silicon-based polymer used by the fried-food industry as an antifoaming agent during frying.Previous studies have demonstrated a protective effect of PDMS in frying oils when used in amounts greater than that necessary to form a monolayer of PDMS in the air–oil inter-face [5,6].The effect of PDMS on the formation of HNE in oils rich in linoleic acid has not been evaluated.The objective of this paper was to study the influence of PDMS on the for-mation and degradation of HNE,and to measure the duration of PDMS’s protective effect at frying temperature.Experimental ProceduresMaterialsThe soybean oil was refined,bleached,and deodorized and treated with citric acid (Golden Chef,Archer Daniels Midland Company,Decatur,IL,USA).The PDMS was food-grade Dow Corning 200with a viscosity of 350cSt (Dow Corning Co.,Midland,MI,USA).P.J.White (&)Department of Food Science and Human Nutrition,Iowa State University,E262Lagomarcino Hall,Ames,IA 50011-1060,USA e-mail:pjwhite@J.A.Gerde ÁE.G.HammondDepartment of Food Science and Human Nutrition,Iowa State University,2312Food Sciences Building,Ames,IA 50011-1061,USAJ Am Oil Chem Soc (2011)88:1503–1510DOI 10.1007/s11746-011-1811-2TreatmentsA stock solution containing100ppm of PDMS in hexane was prepared,and appropriate amounts were added to 100950mm crystallizing dishes(Pyrex,Corning Inc., Corning,NY,USA),and the solvent was evaporated.Two replicates of all treatments were prepared.Two hundred grams of soybean oil was added to each container giving an initial surface to volume ratio of0.36cm-1.The treatments were heated simultaneously to180°C and held for48h. Oil aliquots of2.5mL were removed every2h for further analysis and immediately frozen by immersion in liquid nitrogen,and stored in glass vials at–80°C.The oil removed was not replaced.Fatty Acid CompositionOil aliquots were converted to fatty acid methyl esters (FAME)according to Hammond[7].The FAME were analyzed by gas chromatography using a Hewlett-Packard 5890Series II chromatograph with aflame ionization detector and split/splitless injector.The column was15m with a0.25mm90.2l mfilm of SP-2330(Supelco, Bellefonte,PA,USA).The chromatographic conditions were the same as those for Gerde et al.[6]:injector tem-perature,230°C,detector temperature,230°C,oven tem-perature program,150–180°C at5°C/min with no holding time.The carrier gas(He)was set at5.4mL/min,the auxiliary gasflow(He)was19.4mL/min.Hydrogenflow was13.9mL/min,and airflow426mL/min.The split ratio was24:1.The disappearance of methyl linoleate(18:2)was monitored by using the amount of methyl palmitate present in the oil as an internal standard and the linoleate to pal-mitate ratios(18:2/16:0)were calculated and transformed to l mol18:2/g FAME.The natural logarithm of the concen-trations was computed and plotted versus time.Linear or bilinear equations,if points of change in slope were present, werefitted to pseudofirst-order kinetics for the degradation of18:2,and the rate constants were calculated before and after the change of slopes(k1and k2)[6].Tocopherol ConcentrationOil aliquots were accurately weighed and diluted with hexane to obtain0.1g/mL solutions.The concentrations of the various tocopherols were determined by injecting 20l L of the solution in a Beckman Coulter System Gold HPLC(Beckman Coulter Inc.,Fullerton,CA,USA) equipped with a25cm94.6mm5l60A˚LiChrosorb Silica column(ES Industries Chromega Columns,West Berlin,NJ,USA)and a photo diode array detector set at 292nm.The elution solvent was isopropanol:hexane(5:95 v/v)at aflow rate of0.7mL/min.The tocopherol concentrations were expressed in ppm using external standards for quantification.It was assumed that the dis-appearance of the tocopherols followedfirst order kinetics, and the natural logarithms of the tocopherol concentrations were plotted versus time.Linear equations,or bilinear equations if there were changes in slope,werefitted,and the rate constants were calculated before and after the changes in slope(k1and k2)[6].HNE ConcentrationThe HNE concentration in the oil was measured as descri-bed by LaFond[8].After adding a known amount of 4-hydroxy-9,9,9-d3-non-2E-enal(HNE-d3)(Cayman Chemical Co.,Ann Arbor,MI,USA),the oil aliquots were extracted twice for20min with10mL water to remove the HNE,and the combined water extracts were treated with 1mL of1%O-(2,3,4,5,6-pentafluorobenzyl)hydroxyl-amine hydrochloride(PFBHA,Sigma-Aldrich Corp.,Saint Louis,MO,USA)solution in methanol to derivatize the aldehyde group.The PFBHA solution also contained0.1% tert-butyl hydroxytoluene(BHT)to minimize oxidation. The reaction mixture was sonicated at room temperature for 1h and extracted twice with10mL pentane.After evapo-rating the pentane under a gentle stream of nitrogen,the extracts were treated with200l L of N,O-Bis(trimethyl-silyl)trifluoroacetamide(BSTFA)and trimethylchlorosi-lane(TMCS,Sigma-Aldrich Corp.,Saint Louis,MO,USA) (9:1v/v)and heated at90°C in sealed tubes to derivatize the alcohol group.Then the reaction mixtures were diluted to1mL with methylene chloride,and the samples were analyzed by gas chromatography-electron impact mass spectrometry(GC–MS).The GC–MS was an Agilent6890 (Agilent Technologies Inc.,Santa Clara,CA,USA)con-nected to a Micromass GCT Time of Flight(TOF)mass spectrometer(Waters Corp.,Milford,MA,USA).The GC separation was done using a30m9 0.25mm90.25l mfilm DB-5MS capillary column(Ag-ilent Technologies Inc.,Santa Clara,CA,USA)with a1-m guard column(uncoated,Agilent Technologies Inc.,Santa Clara,CA,USA).The chromatographic parameters were: injector temperature,260°C and oven temperature pro-gram,100°C for1min,100–240°C at8°C/min,and 240–300°C at25°C/min with afinal holding time of 3.1min.The carrier gas(helium)flow was1.0mL/min. The mass spectrometer conditions were:2scans/s,ioni-zation mode,positive electron impact,function type,TOF MS,and mass range45–600.The m/z(mass to charge ratio)352,226,and242fragments were used to identify the HNE peaks and the m/z352,229,and245were used to identify the HNE-d3peaks.Because the m/z352fragment is common to HNE and HNE-d3,only the areas of the integrated peaks corresponding to the m/z226and242fragments and the m/z229and245fragments were used for HNE and HNE-d3quantification,respectively.The con-centration of HNE and HNE-d3standard solutions were monitored spectrophotometrically by evaporating the sol-vent of an aliquot of the standard solution,re-dissolving it in water,and reading it in the spectrophotometer at k=223nm.The concentration was calculated by using the molar extinction coefficient of HNE in water at k=223nm e=13,750to verify the concentrations reported by the supplier[9,10].Kinetics Model ParametersThe equation parameters were estimated by using Graph-Pad Prism software version4.03for Windows(GraphPad Software,San Diego,CA,USA)and all the regression curvesfitted had an R2[0.9.Statistical AnalysisThe estimated kinetic coefficients were analyzed by using analysis of variance(ANOVA)with the SAS9.1software mixed-models procedure(SAS Institute Inc.,Cary,NC, USA).Comparisons were evaluated by contrasts using Tukey’s modification at a level of significance of a=0.05 unless otherwise indicated[11].Results and DiscussionDegradation of18:2and TocopherolsFor both the25-and100-ppb PDMS treatments,there was an increase in slope(rate of degradation)in the plots of ln [18:2]versus time during the heating of the oil(Fig.1)in agreement with previously reported data[6].The times of change in the rate of degradation(T)were calculated,and the rates before and after this time were compared(Table1). The rates after T were different from the rates before T at this significance level(p\0.065).For the control oil,no change in rate was observed.The rate after T for the25-ppb PDMS treatment was not significantly different from that of thecontrol oil.But for the100ppb PDMS treatment,the rate of 18:2degradation after T remained lower than that of the control oil.T was later for the100ppb PDMS treatment than for the25ppb PDMS treatment at just over significance level of a=0.05(p=0.055).This change in slopes of18:2 degradation may be controlled by the disappearance of tocopherols at PDMS concentrations greater than the monolayer concentration[6].For c-tocopherol disappearance,there was a change in rate only in the100-ppb PDMS treatment(Fig.2),and no change in rate was detected for the25-ppb PDMS treatment.The rate after T in the100-ppb PDMS treatment was greater than the rates of c-tocopherol in the control or 25-ppb PDMS-treated oils(Table2).The degradation of d-tocopherol showed rate changes for both the25-and100-ppb PDMS-treated oils(Table2) in agreement with previously reported results[6].The T occurred later in the100-ppb PDMS treatment than in the 25-ppb PDMS treatment.For the25ppb PDMS treatment, the rate after T was not different from the rate of the degradation of d-tocopherol in the control oil(Fig.2).For the100ppb PDMS treatment,the rate after T wasgreater Fig.1Decrease of the natural logarithm of the[linoleate]in two replications of control soybean oil containing no polydimethylsilox-ane(PDMS)and in soybean oil treated with25-or100-ppb PDMS during heating at180°C and the curves generated from the mean of the parameters of the respectivefitted curvesthan in both the control and the 25-ppb PDMS-treated oils.Table 2shows that c -tocopherol degraded faster than d -tocopherol,in agreement with previous reports [6,12].Interfering compounds co-eluted with a -tocopherol,so its rate of disappearance could not be determined.4-Hydroxynonenal FormationFor the control oil,the HNE concentration rapidly increased during the first 14h of heating,then slowed,reaching a maximum concentration of 0.034l mol/g oil at *18h heating (Fig.3).For the 25-ppb PDMS oil,the increase in HNE concentration was less steep,and the maximum,0.033l mol/g oil,occurred at 28h (Fig.3).For both treat-ments,there was a slow decrease in the HNE concentration with time after reaching the maximum.For the 100-ppb PDMS treatment,the increase in HNE concentration was much slower than in the other treatments during the first 32h heating.After 32h the HNE concentration increased faster,and after 46h of heating,reached levels comparable to the maxima found for the other treatments (0.033l mol/g oil)(Fig.4).There was no perceptible decline in HNE concen-tration during the 48h the oil was heated.HNE is formed as a product of linoleate degradation,but it may further degrade by additional reactions.Assuming pseudo first-order kinetics for these degradations:Table 1The time of change in rates (T )and rates of linoleate destruction (1st order kinetics)in a control soybean oil with no PDMS added and in soybean oil with 25or 100ppb PDMS heated at 180°C PDMStreatment (ppb)Mean T (h)Mean rate (k 18:2)Before change (k 1)Afterchange (k 2)0(control)–0.0154x 0.0154x 2517y 0.0108a,y 0.0146b,x 10029x0.0031a,z0.0064b,ya,bDifferent superscripts in the same row of columns 3and 4indicate significant differences at p B 0.065x–zDifferent superscripts in the same column indicate significant differences at p B0.055Fig.2Decrease of the natural logarithm of the [c -tocopherol]and [d -tocopherol]in tworeplications of control soybean oil containing nopolydimethylsiloxane (PDMS)and in soybean oil treated with 25-or 100-ppb PDMS during heating at 180°C and the curves generated from the mean of the parameters of the respective fitted curves18:2À!k 18:2HNE +other productswhere k 18:2could be k 1or k 2,depending on the stage of degradation of 18:2[6].18:2À!k fHNE À!k ddegradation products d 18:2½dt ¼Àk 18:218:2½ ð1Þd HNE ½dt ¼Àk f 18:2½ Àk d HNE ½ ð2Þ18:2½ ¼18:2½ 0e Àk 18:2tð3ÞHNE ½ ¼HNE ½ 0e Àk d t þk f 18:2½ 0k d Àk 18:2ðe Àk 18:2tÀe Àk d t Þð4ÞFor the control oil and the 25ppb (the monolayer concentration)treatments [HNE]0was 0,as measured in the unheated oil,with the model for those treatments described by Eq.5.HNE ½ ¼k f 18:2½ 0k d Àk 18:2ðe Àk 18:2tÀe k d t Þð5ÞAlthough there was a slight change in slope during heating of the 25-ppb PDMS treatment,to simplify the model,degradation of 18:2was assumed to follow a pseudo first-order kinetics with a constant slope k 18:2=0.0134.Based on this assumption,the kinetic parameters of the degradation of 18:2and the formation and degradation of HNE in the control oil and the 25ppb PDMS oil are presented in Table 3and the data points,with the curves generated from the mean of the parameters of the respective fitted curves,in Figs.3and 4.In the treatment with 100-ppb PDMS,the model is more complicated so the data was fitted by dividing the plot into several intervals (Fig.4).At the beginning of the heating period (before 4h zone I),there was an abrupt increase in the HNE concentration.After this period,there was a linear increase in the concentration of HNE for approximately 32h (zone II).At this point there was a transition zone (zone III),where the velocity of the reaction increased until the concentration of HNE followed kinetics similar to that of the control oil (38–48h,zone IV).In zone I,the rapid increase in HNE concentration probably resulted from the decomposition of peroxides formed during the temperature rise in the oil,coupled with the protective effect at higher temperatures of ample amounts of PDMS that reduced the destruction of HNE to very low levels.The initial period lasted 4h in this study because that was the first aliquot analyzed after the system reached 180°C.During the second stage (zone II)the concentration of HNE increased at a very low rate (Fig.4).Because of the very low concentration of HNE and the comparatively highTable 2The time of changes in rates (T )and rates of destruction of c -and d -tocopherol in a control soybean oil with no PDMS added and in soybean oil with 25or 100ppb PDMS heated at 180°CTreatment (ppb PDMS)Mean T (h)Mean rate before change (k 1)Mean rate after change (k 2)c -Tocopherol0Control –0.2306x 0.2306y 25–0.2279x 0.2279y 100320.0590a,y 0.3724b,x d -Tocopherol0Control –0.1424x 0.1424y 2513y 0.0835a,y 0.1536b,y 10037x0.0287a,z0.1963b,xa,b Different superscripts in the same row of columns 3and 4indicate significant differences at p B 0.05x–zDifferent superscripts in the same column indicate significant differences at p B 0.05within each tocopheroltypeFig.3Change in HNE concentration (l mol/g)in two replications of control soybean oil with no PDMS added and soybean oil with 25-ppb PDMS heated at 180°C and the curve generated from the mean of the parameters from the respective fitted curvesconcentration of 18:2,the probability of oxygen or a free radical attacking HNE instead of 18:2was very low.Thus,it was assumed that,during this period,the degradation of HNE should be very small compared to the rate of for-mation of HNE.To compare the rest of the parameters with the models obtained for the control and the 25-ppb PDMS treated oils,the time (t )and the initial concentration of HNE ([HNE]0)were adjusted to consider 4h the initial point of zone II.Thus,t =t -4h and [HNE]0was the [HNE]measured in the initial point of zone II (4h after the start of heating).Likewise,the degradation of 18:2that occurred in zone I was taken into account;therefore,the initial concentration of 18:2([18:2]0)was the concentration of 18:2after heating the oil for 4h.Zone III was likely a transition zone between zones II and IV,in which the system adjusted to a lower PDMS level similar to that of the unprotected soybean oil.Also during this period,the changes in the rate of degradation of tocopherols and 18:2occurred.For zone IV (Fig.4)it was again necessary to consider the changes that occurred in the system before the starting time and to adjust the parameters appropriately:t =t -38,[HNE]0and [18:2]0were the concentrations of HNE and 18:2at t =38h,respectively.In zone II of the 100-ppb PDMS treatment both k f and k d were smaller than they were in zone IV,and both k f and k d were smaller than those in the control oil and the 25-ppb PDMS treatment.In zone IV k f for the 100-ppb PDMS treatment was not different from the k f in the control ortheFig.4Change in HNEconcentration (l mol/g)in two replications of soybean oil treated with 100-ppb PDMS heated at 180°C.The chart was divided into four zones,designating the different stages of HNE concentration evolution to simplify curve fitting.Details of zones II and IV data points with their corresponding fitted curves are presentedTable 3Mean kinetic parameters for the formation of HNE in soybean oil with 0,25or 100ppb PDMS held at 180°C100ppb PDMS 25ppb PDMSControlZone II t =t -4hZone IV t =t -38h [18:2]0a 1,771l mol/g 1,531l mol/g 1,807l mol/g 1,807l mol/g k 18:2a 0.00310.00640.0134b 0.0154Rate of HNE formation (k f ) 1.598E-7e 2.310E-6cd 1.720E-6d 3.262E-6c Rate of HNE degradation (kd )0.0082e 0.0529d 0.0656d 0.1295c kf /k 18:25.19E-5e3.524E-4c1.300E-4de2.121E-4cda [18:2]0and k 18:2were obtained from the curves representing degradation of 18:2versus time and fixed during the fitting of the other parameters b18:2degradation in 25ppb PDMS was considered to be linear to simplify the calculationsc–eDifferent superscripts in the same row indicate significant differences at p B 0.0525-ppb PDMS treatments.But k d of the100-ppb PDMS treatment was less than that of the control oil,but similar to that of the25-ppb PDMS treatment(Table3).The kinetic constant for the formation reaction of HNE k f,is a function of k18:2.Therefore,the ratio of k f/k18:2is a measure of the proportion of HNE formed compared to other18:2degradation products.This ratio was greater in zone IV and in the control oil than in zone II,probably because of the effect of PDMS on the rate of oxygen uptake by the oil[13].After the PDMS degraded to a level where it could no longer maintain a low oxygen concentration in the oil(zone IV),the formation of oxygenated degradation products,such as HNE,would have been favored,thus explaining the similarity of k f/k18:2for the control oil and the100-ppb PDMS treatment during zone IV.The production of HNE in heat-abused oils has been previously studied[1,14].In one study,soybean oil was heated at185°C in a round bottomflask while air was bubbled through the oil.The HNE increased rapidly in the beginning followed by a decrease after reaching a maxi-mum concentration.The maximum HNE concentration (0.27l mol/g oil)occurred after6h heating[14].In another study,pure methyl linoleate was heated at185°C and the maximum HNE concentration occurred at3h heating(0.54l mol/g FAME)followed by a decrease[1]. These values are considerably higher than those found in the current study,but show similar trends.Bubbling air through the oil would produce much better oxygenation, leading to higher levels of HNE in the early stages when there would be abundant amounts of linoleate.Another study reported the presence of0.021l mol HNE/g oil after heating soybean oil in an open beaker for8h with constant air bubbling[15].This value is much closer to the values obtained in the current study.The change in the kinetic constants of the disappearance of both tocopherols and18:2and in the formation and degradation of HNE for the three treatments suggests that the protective effect of PDMS is lost after heating for several hours.PDMS has been reported to depolymerize and degrade by a free radical mechanism when heated to high temperatures(290°C in the presence of air)[16],and this may occur slowly at180°C.ConclusionsThe protective effect against oxidation by PDMS was confirmed in soybean oil.To be effective,the concentration of PDMS seemingly must be greater than the calculated monolayer concentration,which,under our conditions,was about25ppb of the oil.In soybean oil with100ppb PDMS,the slopes of c-and d-tocopherol and18:2degra-dation decreased compared to the control,and changes in rates occurred during the heating period.The PDMS con-centration also influenced the formation and degradation of HNE.To facilitate the analysis of the formation of HNE in soybean oil with100-ppb PDMS,the data was divided into four intervals(zones I,II,III,and IV).The times at which the changes in rates for18:2and tocopherol degradation occurred were close to or within the transition zone III.The change in slopes of18:2degradation may be controlled by the disappearance of tocopherols at PDMS concentrations greater than the monolayer concentration.However,the increase in the rate of formation of HNE in zone IV of the 100-ppb PDMS treatment may have occurred because of PDMS degradation.Thus,an increase in the uptake of oxygen may be the reason for the greater k f/k18:2ratio in zone IV of the100-ppb PDMS treatment than in either the 25-ppb PDMS treatment or in the control oil. Acknowledgment The authors would like to thank Sean Fond, John P.Jerrell,Keith R.Cadwallader,and William E.Artz(Univer-sity of Illinois at Urbana-Champaign)for providing the method for the HNE analysis.Our research was funded by the United States Department of Agriculture(USDA)through a Special Grant to the Nutrition and Wellness Research Center(NWRC)at Iowa State University(Project No.2008-3411519372).References1.Han HI,Csallany AS(2009)Formation of toxic a,b-unsaturated4-hydroxy-aldehydes in thermally oxidized fatty acid methyl esters.J Am Oil Chem Soc86:253–2602.Esterbauer H,Schaur RJ,Zollner H(1991)Chemistry and bio-chemistry of4-hydroxynonenal,malonaldehyde and related aldehydes.Free Radic Biol Med11:81–1283.Kanner J(2007)Dietary advanced lipid oxidation end productsare risk factors to human health.Mol Nutr Food Res 51:1094–11014.Esterbauer H(1993)Cytotoxicity and genotoxicity of lipid-oxi-dation products.Am J Clin Nutr57:779S–786S5.Freeman IP,Padley FB,Sheppard WL(1973)Use of silicones infrying oils.J Am Oil Chem Soc50:101–1036.Gerde JA,Hammond EG,White PJ(2011)Influence of poly-dimethylsiloxane on the degradation of soybean oil at frying temperature.J Am Oil Chem Soc.doi:10.1007/s11746-011-1810-37.Hammond EG(1991)Organization of rapid analysis of lipids inmany individual plants.In:Linskens HF,Jackson JF(eds) Modern methods of plant analysis,new series,vol12.Essential oils and waxes.Springer,New York,pp321–330Fond SI,Jerrell JP,Cadwallader KR,Artz WE(2011)For-mation of4-hydroxy-2-(E)-nonenal in a corn-soy oil blend:a controlled heating study using a french fried potato model.J Am Oil Chem Soc.doi:10.1007/s11746-010-1738-zng J,Celotto C,Esterbauer H(1985)Quantitative determina-tion of the lipid peroxidation product4-hydroxynonenal by high-performance liquid chromatography.Anal Biochem150:369–378 10.Esterbauer H,Weger W(1967)Action of aldehydes on normaland malignant cells3:synthesis of homologues of4-hydroxy-2-alkenals,2.Monastshefte fur Chemie98:1994–200011.Littel RC,Stroup WW,Freund RJ(2002)SAS for linear models,4th edn.SAS Institute,Cary12.Barrera-Arellanos D,Ruiz-Mendez V,Velasco J,Marquez-RuizG,Dobarganes C(2002)Loss of tocopherols and formation of degradation compounds at frying temperatures in oils differing in degree of unsaturation and natural antioxidant content.J Sci Food Agric82:1696–170213.Gerde JA,Hammond EG,White PJ(2011)Influence of poly-dimethylsiloxane on the oxygen concentration of oils at various temperatures.J Am Oil Chem Soc.doi:10.1007/s11746-011-1762-714.Seppanen CM,Csallany AS(2002)Formation of4-hydroxy-nonenal,a toxic aldehyde in soybean oil at frying temperature.J Am Oil Chem Soc79:1033–103815.Seppanen CM,Csallany AS(2001)Simultaneous determinationof lipophilic aldehydes by high-performance liquid chromatog-raphy in vegetable oil.J Am Oil Chem Soc78:1253–1260 16.Camino G,Lomakin SM,Lazzari M(2001)Polydimethylsilox-ane thermal degradation.Part 1.Kinetic aspects.Polymer 42:2395–2402。